Abstract
Detonation nanodiamonds (NDs) are emerging as delivery vehicles for small chemical drugs and macromolecular biotechnology products due to their primary particle size of 4 to 5 nm, stable inert core, reactive surface, and ability to form hydrogels. Nanoprobe technology capitalizes on the intrinsic fluorescence, high refractive index, and unique Raman signal of the NDs, rendering them attractive for in vitro and in vivo imaging applications. This review provides a brief introduction of the various types of NDs and describes the development of procedures that have led to stable single-digit-sized ND dispersions, a crucial feature for drug delivery systems and nanoprobes. Various approaches used for functionalizing the surface of NDs are highlighted, along with a discussion of their biocompatibility status. The utilization of NDs to provide sustained release and improve the dispersion of hydrophobic molecules, of which chemotherapeutic drugs are the most investigated, is described. The prospects of improving the intracellular delivery of nucleic acids by using NDs as a platform are exemplified. The photoluminescent and optical scattering properties of NDs, together with their applications in cellular labeling, are also reviewed. Considering the progress that has been made in understanding the properties of NDs, they can be envisioned as highly efficient drug delivery and imaging biomaterials for use in animals and humans.
Introduction
Elemental carbon has six electrons with an electronic configuration of 1s2 2s2 2p2 in its ground state. The two electrons of the 1s orbital are core electrons, while the other four are valence electrons. Sp2 hybridization of carbon atoms leads to the formation of the two-dimensional planar hexagonal structure of graphite. In this type of arrangement, the central carbon is linked to three other carbon atoms by σ-bonds, with the remaining electron of the pz orbital forming a delocalized cloud of π electrons over the graphitic structure. Sp3 hybridization of carbon atoms forms a rigid diamond structure with tetrahedral symmetry, where all the four valence electrons of the carbon atom each form a σ bond with the neighboring carbon atoms. The general lack of free electrons in the bulk structure of diamond accounts for their inertness. However, the surface structure of the material is different from the bulk, due to the relaxation at the surface and the need to terminate the bonds. Thus, the terminating structure on diamond surfaces involves univalent species such as H or OH (hydrogen or hydroxyl) or any deliberate terminating groups for both nano and larger gem diamonds (synthetic and natural).
Nanodiamonds (NDs) have attracted a great deal of scientific and technological interest due to their unique structural, chemical, biological, mechanical, and optical properties. Based upon their primary particle sizes, Shenderova and McGuire classified NDs into nanocrystalline particles (1 to ≥150 nm), ultrananocrystalline particles (2 to 10 nm), and diamondoids (1 to 2 nm).Citation1 Ultrananocrystalline diamond particles are of particular interest for biomedical applications and focus has been largely placed on detonation NDs with a primary particle size of 4 to 5 nm.Citation2 shows the classification of diamonds based on synthetic production methods. The types of diamonds include chemical vapor deposition diamonds, high-pressure high-temperature diamonds, and detonation NDs.
Figure 1 A schematic representation of nanodiamond types based on their methods of synthesis and applications.
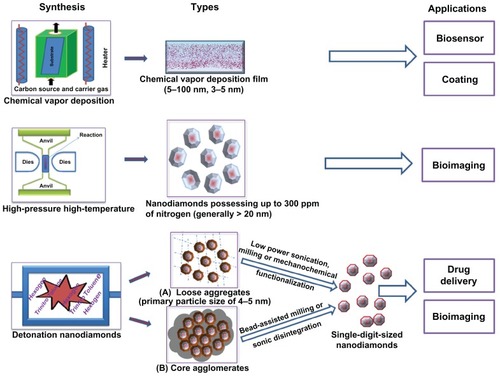
Deposition of carbon vapors on a diamond or non-diamond substrate leads to the formation of diamond films;Citation3 the nanocrystalline and ultrananocrystalline types of these are of major interest, due to their superior mechanical and wear resistant properties. Depending on the growth precursors (hydrogen-rich and carbon-poor precursors) and other deposition parameters such as biasing voltage, surface temperature, and film pressure, the grain size of nanocrystalline diamond films can vary from 5 to 100 nm.Citation4,Citation5 Ultrananocrystalline diamond films with a much smaller grain size of 3 to 5 nm are usually synthesized under hydrogen-poor and argon-rich conditions.Citation6–Citation8 In addition to the difference in grain size, these two types of diamond films also differ in their sp2 carbon content. Nanocrystalline diamond films grown in a methane-rich environment possess a sp2-bonded carbon structure of up to 50%, which decreases drastically to less than 0.1% with decrease in the amount of methane.Citation9 For the ultrananocrystalline diamond films, the sp2-bonded carbon fraction ranges from 2% to 5%.Citation9 Scanning transmission electron microscopic images of nanocrystallineCitation4 and ultrananocrystallineCitation10 diamond films are shown in .
Figure 2 Scanning electron microscopic image of (A) nanocrystallineCitation4 and (B) ultrananocrystallineCitation10 diamond films grown on silicon substrate.
A is reprinted with permission from: Philip J, Hess P, Feygelson T, et al. Elastic, mechanical, and thermal properties of nanocrystalline diamond films. J Appl Phys. 2003;93(4):2164–2171,Citation4 Copyright (2003), American Institute of Physics. B is reprinted with permission from: Sumant AV, Grierson DS, Gerbi JE, Carlisle JA, Auciello O, Carpick RW. Surface chemistry and bonding configuration of ultrananocrystalline diamond surfaces and their effects on nanotribological properties. Phys Rev B Condens Matter Mater Phys. 2007;76(23):235429-1–235429-11,Citation10 Copyright (2007) by the American Physical Society.
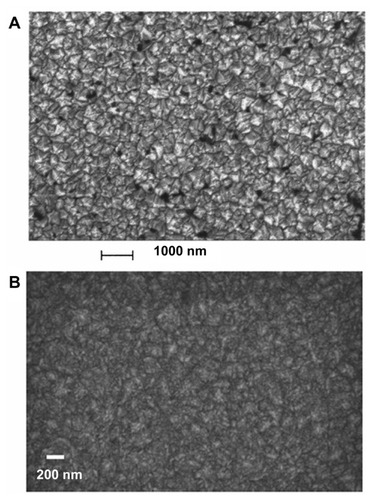
For coating biomedical implants, ultrananocrystalline diamond films are emerging as substrates superior to those of silicon, platinum, and quartz.Citation11,Citation12 This advantage is due to their low cytotoxicity, dense structure, high surface roughness, sp2 structural boundaries and surface dangling bonds.Citation11,Citation12 These films also exhibit the desired characteristics for producing micro-electro-mechanical devices due to their robustness, high Young’s modulus, high acoustic velocity, high resistance to fracture, and low friction coefficient (~0.01 to 0.1).Citation13–Citation16 Similarly, the high stability, superior electrochemical properties, selectivity in binding biological materials, and biocompatibility of the nanocrystalline diamond films have opened up their potential in the development of biosensors.Citation17,Citation18
Synthetic diamonds produced by a high-pressure high-temperature technique could contain up to 100 to 300 ppm of nitrogen.Citation19–Citation22 High-energy irradiation of these diamond particles causes damage by knocking a diamond carbon atom out of the structure, creating vacancies (V).Citation23 Upon thermal annealing, these vacancies move closer to the nitrogen (N) centers to form nitrogen-vacancy (N-V) color defect centers.Citation23 These centers are responsible for the emission of fluorescence.Citation24 Hence, high-pressure high-temperature synthetic diamonds have been widely explored as imaging agents in cellular models.Citation19,Citation22,Citation25 However, the high production cost and the general inability to produce diamond particles smaller than 20 nm are significant barriers to their use in biomedical sciences.
The third category of diamonds, detonation NDs, also known as “ultradispersed diamonds,” are synthesized relatively inexpensively on a large scale by the detonation of carbon explosives with a CmHnNoOp composition.Citation26 The explosive mixtures are required to have an overall negative oxygen balance in the detonation chamberCitation26 to prevent the complete combustion of the carbon into its gaseous products. Generally, a mixture of trinitrotoluene and hexogen in a mass ratio ranging from 40:60 to 70:30 is utilized.Citation27 A shock wave produced by the detonator compresses and heats the composition mixture, leading to its explosion.Citation26 A large amount of energy is released during this exothermic process, which consequently raises the temperature in the detonation chamber to 3000°C–4000°C and the pressure to 20–30 GPa, to favor the synthesis of the diamond particles ().Citation28,Citation29 After the detonation is complete, a rapid cooling is achieved, either by using an inert cooling gas (dry synthesis) or water (wet synthesis).Citation29 The cooling of the detonation products is crucial to minimize transformation of the diamond phase into graphitic forms, in accordance with the phase diagram ().Citation28,Citation29 The average primary particle size of NDs generated by this technique is ~4 to 5 nm ().Citation28,Citation30
Figure 3 Phase diagram for carbon depicting the pressure and temperature requirements for synthesis of detonation nanodiamond (1); cooling profile of products produced by wet detonation synthesis (2) and dry detonation synthesis (3).Citation29
Notes: “TD” represents Debye temperature.
Reproduced courtesy of IOP Publishing Ltd, from: Baidakova M, Vul A. New prospects and frontiers of nanodiamond clusters. J Phys D Appl Phys. 2007;40(20):6300–6311.
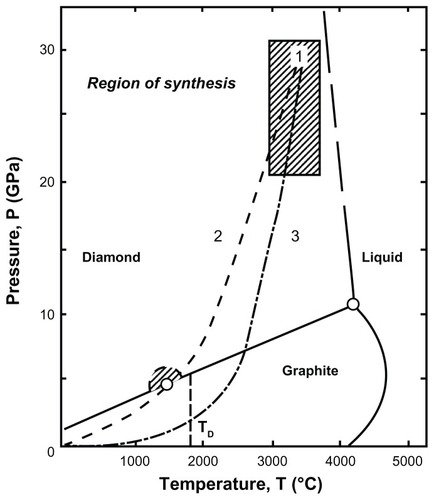
Figure 4 High-resolution transmission electron microscopic image of detonation nanodiamonds.
Note: Image is courtesy of Bogdan Palosz, IHPP, Warsaw, Poland.
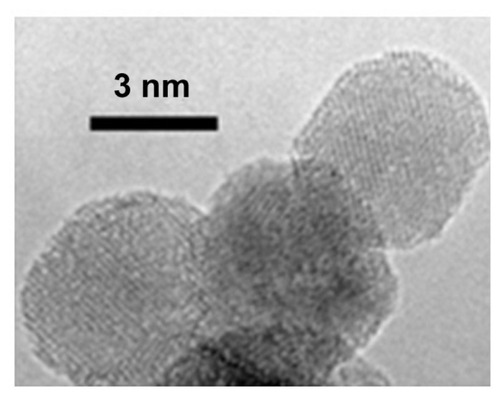
Although the primary particle size of NDs obtained by the detonation technique is well suited for biomedical studies, the detonation products need to be extensively purified. Depending on the materials and matrices present during their production, detonation NDs can contain oxides and carbides, including those of iron, chromium, silicon, calcium, copper, potassium, titanium, and sulfur, in addition to carbon soot.Citation26 To remove surface metallic impurities, NDs are treated with classic acidic treatments consisting of sulfuric acid and its mixtures with nitric acid or potassium dichromate.Citation31 A hydrofluoric acid and nitric acid combination has also been used to eliminate metallic contaminants from the particles.Citation32 The oxidization and subsequent removal of sp2-bonded carbon structures, present either in amorphous or graphitic forms, is achieved by the use of liquid oxidizers such as sodium peroxide, a chromium trioxide and sulfuric acid mixture, or a nitric acid and hydrogen peroxide mixture.Citation31 Thermal oxidation process uses temperatures of 400°C–430°C to allow the oxidation of sp2-bonded carbon species in the air, with negligible alteration in sp3-bonded carbon structures.Citation33 These temperature requirements for the selective oxidation of sp2-bonded carbon species were confirmed by Pichot et al.Citation32 The oxidation of NDs at a high temperature using air containing ozone is another approach that results in the removal of the majority of sp2-hybridized carbon structures.Citation34 The ozone-air treatment, also known as the “gas phase treatment,” is ecological and efficient, as the purification of NDs is achieved without using the corrosive liquid oxidizers.Citation34
Although the size, shape and surface properties of NDs are determined by the nature of explosion and purification conditions, their basic structure follows a core and shell model.Citation2 The diamond carbon forms the inert core and the surface shell is partially comprised of graphitic structures.Citation30,Citation35,Citation36 In addition, a wide variety of functional groups such as carboxyl, hydroxyl, lactone, anhydride, ketone, and ether can be present on the surface of these ND particles.Citation36,Citation37 X-ray diffraction is one of the most utilized techniques for characterizing NDs, including in terms of their size, structure, and composition.Citation38–Citation40 Upon annealing the NDs at 1500°C for 10 minutes in a vacuum, the diamond X-ray diffraction peak disappears with the emergence of sp2 carbon structure peak.Citation41 The crystal lattice parameters and hence the quality of NDs can vary depending upon the synthesis conditions, as determined by X-ray diffraction studies.Citation38
The economical large-scale production of detonation NDs provided considerable impetus to technological applications. For example, the antifriction properties of NDs and their soot make them ideal candidates as wear-protective additives.Citation42 The stability of these particles at extreme temperatures had led to applications in composite manufacturing.Citation43 The large surface area of NDs is suitable for adsorbing biomolecules, presenting them as an attractive material for isolating proteinsCitation44,Citation45 and pathogenic microorganisms.Citation46 However, it was not until the identification and characterization of single-digit-sized NDs in the dispersion medium that NDs were applied in biomedical sciences.
NDs in the biomedical field
The application of NDs in biological sciences requires their stable dispersion in a formulation medium, ability to conjugate with biomolecules, inherent biocompatibility, and ability to penetrate target tissues and cells.
Dispersion of NDs
Detonation NDs possess a strong propensity to aggregation when dispersed in a liquid formulation medium, hindering their biomedical applications. Several attempts to explain the aggregation phenomenon have focused on surface forces. The harsh conditions in the detonation chamber could create dangling bonds on the ND surfaces.Citation47 These free electron surfaces are sufficiently reactive to form functional groups, which then interact via various intermolecular surface forces such as van der Waals and dipole–dipole interactions, and hydrogen bonding.Citation47 In addition, these surface groups can also react to create covalent bonds between the primary particles,Citation48 producing core aggregates. Another study suggests that electrostatic forces induced by the oppositely charged facets of polyhedral shaped NDs are the primary reason for the creation of aggregates.Citation49 This finding was supported later with the aid of electron microscopy and computer simulations.Citation50 Other than the surface forces, the “graphitic soot-like structures” around the particles can also bind individual ND particles together into core aggregates ().Citation35,Citation36
Conventional techniques of disintegration, such as milling, emulsification, and low power sonication, have been ineffective in breaking down the tightly bound core aggregates of NDs to less than 10 nm.Citation35 Therefore, in an attempt to achieve primary-sized NDs, milling was carried out using an excessive amount of 100 μm silica beads.Citation35 Single-digit-sized NDs were obtained after an hour of sonication of the diluted milled suspension and the stability of the resulting aqueous colloid was maintained for a year.Citation35 However, reconstitution with water after drying the nanodispersion resulted in reaggregation into aggregates of 2.3 μm.Citation35 Bead-assisted sonic disintegration using zirconia beads has also gained interest for breaking the ND aggregates in aqueous medium to single-digit-sized particles.Citation51 This mechanical technique involves the combination of shear forces induced by beads with their acceleration during cavitation created by intense ultrasonic waves. These primary-sized NDs exhibited very good colloidal stability in polar solvents such as water and dimethyl sulfoxide (DMSO).Citation51 However, contamination from the beads and sonotrode material itself can be a barrier for these technologies,Citation35,Citation52 in addition to the structural alterations.Citation53
Bead-assisted millingCitation51,Citation54 or sonicationCitation52 yielded clear, but dark brown nanodispersions of diamond particles. It should be noted that sonication creates micro bubbles in liquid media, which implode violently, generating a local temperature of ~1900 K at the bubble–liquid interface.Citation55 The shock waves produced during high-power sonication cause high-speed collision of the beads with ND particles, which can lead to the conversion of the diamond carbon into its graphitic form, according to the diamond–graphitic phase transition phenomenon occurring at temperatures greater than 1200 K.Citation56 Similarly, a temperature of 1800 K can be attained during milling collisions when the speed of the blades is between 0.1 and 10 cm/s.Citation54 This could explain the black coloration of the colloids observed after high-speed milling or high-power bead-assisted probe sonication.
Mechanochemical treatment, which involves the breaking of ND aggregates by mechanical energy and the concurrent loading of electrolytes or surfactants onto a newly created surface, was also explored to achieve stable dispersions with a noticeable size reduction.Citation57 However, a primary dispersed particle size of less than 10 nm was not achieved.Citation57 Ultracentrifugation, a contamination-free procedure, resulted in NDs of 4 nm that formed a stable colloidal dispersion in water.Citation58 The yield of single-digit-sized particles was only 1% after centrifugation of the ND suspension at an acceleration of 2.80 × 105g for 40 minutes.Citation58 Increasing the centrifugation time to 240 hours at an acceleration of 2.0 × 104g did not improve the yield significantly, generating 6% of NDs with an average size of 8 nm.Citation58 Hence, there is still a need for the development of easy and feasible contamination-free techniques that can result in disaggregation of NDs at the laboratory scale.
Surface modification of NDs
Together, the nano size, larger surface area, and potential for purification with oxidizing agents make NDs a viable candidate for surface functionalization. summarizes approaches used in the past to introduce various functionalities on the surface of NDs.Citation48,Citation59–Citation66 One of the earliest modifications of the ND surface involved the generation of surface radicals, which then acted as substrates for synthesizing carboxylic acid-Citation59 and dicarboxylic acid-Citation60 functionalized NDs. For a high surface loading, it is essential to achieve surface uniformity. Reactions with hydrogen,Citation59,Citation60 chlorine,Citation61 and fluorineCitation62 have been explored to attain surface homogeneity and reactivity enhancement. The surfaces of chlorinated NDs were further modified with hydroxyl, amine, and carbon fluoride groups.Citation61 Finally, reactions of NDs with alkyllithium, ethylenediamine, and glycine ethyl ester hydrochloride generated alkyl-, amino-, and glycine-substituted NDs, respectively.Citation62
Figure 5 A schematic representation of approaches used for modification of nanodiamond surfaces.
Abbreviations: R.T., room temperature; R, alkyl group; Ar, aromatic group; THF, tetrahydrofuran; DMAP, (dimethylamino)pyridine; EDC, (1-ethyl-3-[(3-dimethylamino)propyl]carbodiimidehydrochloride).
![Figure 5 A schematic representation of approaches used for modification of nanodiamond surfaces.Abbreviations: R.T., room temperature; R, alkyl group; Ar, aromatic group; THF, tetrahydrofuran; DMAP, (dimethylamino)pyridine; EDC, (1-ethyl-3-[(3-dimethylamino)propyl]carbodiimidehydrochloride).](/cms/asset/2934650a-c46f-49fb-8e58-0a639da34b0a/dijn_a_37348_f0005_c.jpg)
Surface functionalization has also emerged as a novel treatment for reducing aggregate sizes of NDs. Functionalization with long alkyl chains reduced the particle size from 15 μm to 150–450 nm.Citation63 The long alkyl chain-modified NDs showed an enhanced dispersibility in organic solvents compared with their pristine form.Citation63 Similarly, surface modification with fluorine contributed to size reduction from 1930 to 160 nm.Citation62 A noticeable size reduction of the micrometer-sized pristine NDs to ~50 nm was also achieved upon reduction of the ND surface groups with borane.Citation65 After being grafted with alkyl silane, these surface-modified NDs were used to synthesize biotinylated NDs with a surface loading of 1.45 mmol/g.Citation65 Lysine molecules attached to the surface of NDs reduced the aggregate size from 1281 to 21 nm.Citation66 The resulting lysine-functionalized NDs with a surface loading of 1.7 mmol/g showed a considerably better dispersibility in water compared with carboxylated NDs.Citation66
Biocompatibility studies of NDs
The intrinsic biocompatibility of detonation NDs has been supported by the results of a number of studies;Citation67–Citation72 however, some studies have refuted this claim by arguing that NDs can induce toxic responses under certain conditions.Citation73,Citation74
The biocompatibility of acid- or base-purified NDs of 2 to 10 nm was first demonstrated in neuroblastoma, macrophage, keratinocyte, and PC-12 cells.Citation67 By considering the mitochondrial activity as a key determinant of cellular viability, NDs up to a concentration of 100 μg/mL were reported not to be cytotoxic over a 24-hour incubation period.Citation67 In addition, NDs showed higher biocompatibility than carbon black, and single- and multi-walled carbon nanotubes.Citation67,Citation68 When incubated for 24 hours with NDs at the concentration range of 25 to 100 μg/mL, no significant cell death occurred in neuroblastoma cells.Citation68 Similarly, alveolar macrophages maintained the cellular viability upon exposure for 24 hours to NDs at a concentration of 25 μg/mL.Citation68 In addition to mitochondrial function, the inflammatory activity of cells has also been a key determinant of the toxic effects of nanomaterials.Citation72 There was no significant increase in the gene expression of inflammation biomarkers, such as interleukin-6, tumor necrosis factor-alpha and nitric oxide synthase, in murine macrophages after incubation with a 100 μg/mL concentration of NDs.Citation72 In addition, no significant attenuation in the gene expression level of Bcl-x, which generally diminishes with an increased risk of apoptosis, endorsed the intrinsic biocompatibility of these diamond nanoparticles.Citation72
Various studies have demonstrated that the toxicity of NDs can vary depending upon their surface chemistry,Citation74,Citation75 the type of cell line,Citation74 and the composition of the treatment medium.Citation73 The amine-terminated NDs exhibited a higher toxicity (~22% cell death) than hydroxyl-terminated NDs (~11%) and carboxyl group-terminated NDs (~7%) in human embryonic kidney cells at a concentration of up to 200 μg/mL.Citation75 The carboxylated NDs did not show any significant genotoxic effects in mouse embryonic fibroblastsCitation76 but induced DNA damage in mouse embryonic stem cells,Citation74 although to a lesser extent than what occurred with multi-walled carbon nanotubes.Citation77 In another instance, a 100% viability of human cervical cancer (HeLa) cells was observed after incubating them with 0.1–100 μg/mL NDs in a medium containing serum for 24 hours.Citation73 In the absence of serum, this viability dropped dramatically to almost 0% within 6 hours of dosage with 50 μg/mL NDs.Citation73 Similar behavior has been also observed with other nanoparticles such as carbon blackCitation78 and silica.Citation79 Although there is no clear explanation of the serum-dependent cytotoxicity of nanoparticles so far, several hypotheses have been put forth. It is believed that serum can have a protective effect on the cells by coating the surface of nanoparticles that are thought to have inherent cytotoxic effects.Citation73,Citation78,Citation80 While, on one hand, serum is found to enhance the dispersion stability of the nanoparticles,Citation73,Citation78,Citation81 on the other, serum promotes aggregation.Citation79 The cellular internalization of nanoparticles was observed to be higher in the absence of serum than in its presence,Citation78,Citation79 which might be one of the reasons for the lower cellular toxicity of nanoparticles when incubated with serum. Another study found that nanoparticles could adsorb the essential micronutrients from the medium, hence indirectly produce cytotoxic effects by depleting the cellular structures of essential nutrients.Citation82 Finally, the serum proteins themselves are essential for the growth of the cell, thus their absence can have a negative effect on overall cell proliferation. However, it is important to note that NDs did not show significant toxicity in some studies, even when incubated with cells in medium lacking serum.Citation67,Citation68
In addition to the cellular studies, some in vivo studies have been conducted to evaluate the toxicity of NDs. The intravenous administration of 125 mg of modified NDs did not cause any deaths in rabbits.Citation83 Neither the red blood cell count nor the hemoglobin level of the rabbits decreased after 15 minutes of administering 50 mg of modified NDs intravenously.Citation83 However, 48 hours after the injection, the levels of biochemical molecules such as total bilirubin, triglyceride, low-density lipoprotein, aspartate aminotransferase, and γ-glutamyltranspeptidase altered significantly.Citation83 In another study, an oral administration of 0.002 to 0.05 wt% of ND hydrosols to mice instead of water for 6 months showed no significant abnormalities in growth or organs weight.Citation43 The NDs exhibited low pulmonary toxicity and were cleared from the lungs after 90 days of intratracheal instillation in imprinting control region (ICR)-strain mice.Citation84 Furthermore, the clearance of XenoFluorTM 750-labeled NDs from all the body organs of wild-type FVB/N (mouse strain) mice occurred in 3 to 10 days after tail vein injection at a dose of 120 μg.Citation85
In summary, NDs have shown biocompatibility in various cell lines and some animal models with minimal or no cytotoxicity, demonstrating their potential for biomedical applications. However, the purity, surface chemistry, and dimensions of particles should be considered carefully. More comprehensive and long-term animal studies need to be conducted to verify the safety of NDs before proceeding to human clinical trials.
Cellular uptake of NDs
A number of studies have demonstrated the ability of NDs to internalize into cells.Citation19,Citation25,Citation67,Citation69–Citation71,Citation86–Citation88 Faklaris et al demonstrated that NDs having an average size of 46 nm internalize into HeLa cells predominantly by clathrin-mediated endocytosis after 2 hours of incubation.Citation88 In addition to the clathrin-mediated endocytic pathways, NDs of 100 nm were found to be internalized by cancer and stem cells after 4 hours of incubation via macropinocytosis.Citation76 The intracellular localization of the NDs suggests that these nanoparticles have the potential to be employed for the intracellular delivery of therapeutic molecules. While the NDs used in these studies showed only perinuclear localization with no translocation to the nucleus,Citation76,Citation88 fenton-treated NDs were detected in the nucleus of HeLa cells.Citation89 The internalization of NDs into cells could be dependent upon their surface characteristics,Citation89 incubation time, and other physiochemical parameters such as size, shape, and aggregation. Nuclear internalization of NDs opens up the possibility of their use as delivery agents for nucleic acids. summarizes the prominent applications of NDs in the biomedical field.
Applications of NDs as drug delivery agents
The large surface area of biocompatible NDs, which have several surface functionalities, is ideal for conjugating various biochemical entities. The suitability of the carrier can be projected from its loading capacity as well as from its ability to protect and retain the inherent therapeutic effects of the attached entities. A high loading capacity allows a high concentration of payload to be delivered while using less of the delivery agent itself. In parallel to the loading capacity, the release of payload from the carrier is also important. The feasibility of being able to tune the release of cargo from the carrier is advantageous in developing novel applications of these delivery agents, such as controlled- and sustained-release delivery. Diamond nanoparticles are being investigated globally for improving the intracellular delivery of small molecules and biotechnological products, with a major focus on chemotherapeutic agents. Although some studies have made use of the ND surfaces to bind drugs via chemical bonding,Citation90,Citation91 the majority have focused on physical adsorption procedures.Citation72,Citation73,Citation85,Citation92 This simple process of loading cargo onto the carrier via physical interactions avoids the use of complex chemical reactions, which, in addition to being high cost, can also affect the therapeutic activity of the attached entity by causing structural alterations.Citation93 The use of NDs as a small-molecule delivery agent, using chemotherapeutic drugs as model drugs, has been exemplified by various studies.
Delivery of small molecules
In 2007, the suitability of NDs to act as a delivery agent of doxorubicin hydrochloride (DOX) was evaluated.Citation72 The investigation was based on the rationale that the surface carboxylic and hydroxylic groups of detonation NDs can interact efficiently with the amine groups of DOX via ionic forces when dispersed in aqueous medium.Citation72 The surface loading of DOX on NDs increased from 0.5 to 10 wt% upon addition of 1% sodium chloride solution to their aqueous dispersion, and the removal of salt favored the release of DOX.Citation72 NDs loaded with DOX were suggested to assemble in the form of loose clusters, such that a certain amount of DOX adsorbed on the NDs surface resides within the cavity of the cluster ().Citation72 This strategy of drug entrapment in loose aggregates of NDs could provide an advantage by minimizing the systemic adverse effects of naked DOX.Citation72 Thus, ND-based delivery systems could overcome the limitation to the use of high concentrations of chemotherapeutic drugsCitation94 in cancer treatments. In addition, the lower cytotoxicity of the ND-DOX composite in mouse macrophages and human colorectal cancer cells compared with bare DOX in a 48-hour period could be beneficial in sustained drug release.Citation72
Figure 7 A schematic diagram representing the binding of detonation nanodiamonds with (A) small molecules,Citation72,Citation73 (B) proteins,Citation73 (C) plasmid DNA,Citation66,Citation109 and (D) siRNA.Citation110
©2012 Dove Medical Press. Adapted with permission from Kaur R, Chitanda JM, Michel D, et al. Lysine-functionalized nanodiamonds: synthesis, physiochemical characterization, and nucleic acid binding studies. Int J Nanomedicine. 2012;7:3851–3866.
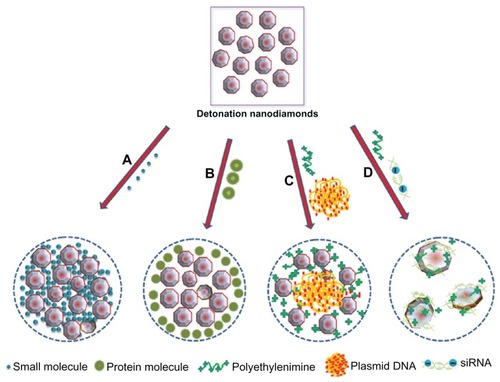
Another study, published in 2010, proposed NDs as efficient multifunctional delivery agents for the chemotherapeutic drug, 10-hydroxycamptothecin (HCPT).Citation73 Similar to the previous study by Huang et al,Citation72 NDs were able to adsorb the drug onto their surfaces via simple physical forces.Citation73 However, in this case, the surface loading of HCPT was considerably enhanced to 50 wt% from 0.4 wt% with an increase in pH of the HCPT solution from 7 to 8.2, rather than by means of salination.Citation73 The NDs released HCPT slowly into the phosphate-buffered saline medium over a period of 5 days, with only 38% release observed in the first 24 hours.Citation73 In addition, the ND-HCPT complex showed almost 2.5 times higher cytotoxicity in HeLa cells than the chemotherapeutic activity of HCPT alone, which was credited to the ND-triggered intracellular delivery of HCPT.Citation73 Bovine serum albumin adsorbed on the surface of NDs rapidly (<1 hour) while HCPT showed a gradual adsorption (>120 hours).Citation73 Based on this differential adsorption, a model suggesting porous clusters of NDs was developed in which small molecules diffuse inside the clusters () and large molecules adsorb only on the outer surface of the NDs ().Citation73 Hence, NDs can facilitate the intracellular delivery of both small chemotherapeutic drugs and large therapeutic biomolecules.Citation73
Detonation NDs have also been utilized to increase the aqueous dispersibility of hydrophobic drugs. Poorly water soluble chemotherapeutic drugs such as purvalanol A, with therapeutic activity against liver cancer,Citation95 and 4-hydroxytamoxifen, with a high potential to treat breast cancer,Citation96,Citation97 are soluble in the polar, organic solvents DMSO and ethanol, respectively.Citation92 The use of nonaqueous solvents limits the parenteral administration of these formulations in clinically relevant settings. However, when formulated with NDs, the aqueous dispersibility of purvalanol A and 4-hydroxytamoxifen was enhanced markedly.Citation92 By adsorbing the drug on their surfaces, NDs considerably reduced particle size and increased the zeta potential of these drugs in water, promoting their dispersibility and, potentially, cellular uptake.Citation92 Similar to the previous studies, the NDs preserved the therapeutic activity of the drugs, as demonstrated by DNA fragmentation and 3-(4,5-Dimethylthiazol-2-yl)-2,5-diphenyltetrazolium bromide (MTT) assay for ND-4-hydroxytamoxifen and ND-purvalanol A composites, respectively.Citation92 These findings suggest that NDs could play an important role in designing injectable formulations of water insoluble drugs.
The efficacy of NDs to deliver chemotherapeutic drugs was examined recently in animal models for the first time.Citation85 In addition to generalized toxicity, large particle size, and poor water solubility, chemotherapeutic drugs also trigger acquired and intrinsic chemoresistance in tumor cells.Citation98 Among various other factors, overexpression of P-glycoprotein on the cellular membranes, causing premature efflux of the drug from cells, is the leading cause for the development of chemoresistance in mammalian tumor cells.Citation99,Citation100 This challenge could be addressed by using a delivery system that not only enhances the uptake of the chemotherapeutic drugs but also retains them in the cancer cells for a longer period. The study revealed that DOX bound to the ND surface was significantly more toxic towards DOX-resistant mouse LT2-Myc liver and 4T1 mammary tumor models than bare DOX.Citation85 The high chemotherapeutic efficacy of the ND-DOX composite was attributed to ND-mediated DOX retention in tumor cells as determined by fluorescence microscopy and quantitative analysis.Citation85 Moreover, long-term treatment showed the superiority of the DOX bound to ND over the bare DOX in preventing tumor growth.Citation85 NDs not only circumvented the premature efflux of DOX from tumor cells but also improved the adverse effects of naked DOX by significantly reducing myelosuppression and early mortality.Citation85 In addition, NDs increased the circulation half time of DOX from 0.83 to 8.43 hours, which further validated their sequestering behavior proposed in earlier studies.Citation72,Citation73,Citation85
In summary, formulation of chemotherapeutic drugs with NDs improves the pharmaceutical properties of the agents by providing high surface loading, improved aqueous dispersibility, sustained tunable release, and enhanced retention in chemoresistant cells. These properties are due to the hydrophilic functional group-enriched surface, large surface-to-volume ratio, ability to form loose clusters, improved cellular delivery, and biocompatibility of the NDs. Hence, NDs are suitable platforms on which to build nanoparticles for overcoming some of the major deficiencies of the chemotherapeutic drugs.
In addition to small molecules, NDs have shown potential as delivery agents for protein, DNA, and RNA.
Delivery of biotechnology products
The potential of NDs as a targeted protein-delivery vehicle was evaluated in a pH-dependent system.Citation101 By means of physical adsorption, NDs achieved a considerably high surface loading of bovine insulin (~80%) in pH-neutral water at a weight ratio of 1:4 of insulin:NDs.Citation101 Similar to a previous study,Citation92 the aggregation properties (size, zeta potential and polydispersity index) of the insulin improved after interacting with NDs.Citation101 This suggests that NDs have the ability to facilitate uniform-sized complex formation.Citation101 Further, the release of insulin from the surface of the NDs was almost 20 times higher when at a pH of 10.5 than when in a neutral pH medium.Citation101 The applicability of ND-bound insulin was verified by MTT assay in serum-starved murine macrophages.Citation101 A higher cellular viability was observed with sodium hydroxide-treated ND-insulin than with neutral pH-treated ND-insulin.Citation101 Similar results were obtained by measuring the gene expression of insulin 1 and granulocyte colony stimulating factor in serum-starved mouse adipocytes.Citation101 Hence, formulations of insulin can be designed with NDs to target the recovery of injured tissuesCitation102 that have a basic pH due to bacterial growth.Citation101,Citation103
Although there is compelling evidence to support the utilization of NDs as drug delivery agents for small molecules, their potential as gene delivery vectors has been less widely explored. The major obstacle to the use of naked nucleic acids as therapeutics is their inefficient cellular delivery. Physicochemical characteristics such as high negative surface charge and large molecular weight are barriers in the efficient cellular internalization of the DNACitation104,Citation105 and small interfering RNA (siRNA).Citation106,Citation107 While DNA is too large for cellular uptake, the relatively small size of siRNA also poses an additional challenge to its cellular delivery, as particles having a molecular weight of less than 50 kDa are susceptible to excretion through glomerular filtration.Citation106,Citation108 Zhang et al investigated NDs as a platform on which to build enhanced plasmid DNA (pDNA) delivery systems ().Citation109 At first, they coated NDs with polyethyleneimine 800 (PEI800) then allowed them to interact with luciferase pDNA via electrostatic forces.Citation109 NDs were found to enhance the transfection efficiency of the polymer by 70-fold at a 15:1 weight ratio of ND-PEI800:pDNA in HeLa cells, which might be due to ND-mediated cellular and nuclear uptake of the pDNA.Citation109 Similar to DNA delivery, the ND-based complex (ND-PEI800) was used to deliver anti-green fluorescent protein siRNA to breast cancer cells expressing green fluorescent protein ().Citation110 Compared with the PEI-siRNA, the ND-PEI800:siRNA complex at a weight ratio of 3:1 showed an almost twofold higher knockdown in cellular green fluorescent protein expression.Citation110 In the presence of serum in the treatment medium, the ND-PEI800:siRNA complex showed better knockdown in green fluorescent protein expression as well as lower cytotoxicity than LipofectamineTM, which is a gold standard for in vitro delivery of nucleic acids.Citation110 Therefore, NDs have the potential to enhance the transfection ability of polymers while remaining biocompatible with the cell lines.
However, so far, there have been limited studies of the applications of NDs as drug delivery agents. More studies are needed to verify their potential and to translate their use as a delivery agent into clinical applications.
NDs as bioimaging agents
Fluorescence of the NDs
Based upon the presence or lack of nitrogen impurities in the crystal lattice structure, the natural diamonds are classified as type I or type II, respectively.Citation111 The arrangement of nitrogen impurities is used to subclassify type I diamonds into type Ia and type Ib.Citation112 While type Ib contain atomically dispersed, single substitutional nitrogen impurities (C-centers), type Ia diamonds comprise an aggregated form of nitrogen.Citation112 The latter are categorized further into type IaA, having two aggregated nitrogen atoms (A-center); type IaB, having platelets and four aggregated nitrogen atoms surrounding the nearest lattice vacancy (B-center); and type IaA/B, possessing the characteristics of both type IaA and type IaB.Citation112
Besides natural diamonds, synthetic diamonds manufactured in high-pressure high-temperature conditions are also known to contain a high amount of nitrogen in the form of impurities.Citation113 The natural diamonds have mainly aggregated nitrogen defects (type Ia), while synthetic high-pressure high-temperature diamonds are predominantly embellished with single substitutional nitrogen centers (type Ib).Citation114 Irradiation damage of type Ib diamond crystals creates intrinsic defects, such as vacancies, which, upon thermal annealing, move towards nitrogen centers (N) and become trapped within to form N-V color defect centers.Citation23 Generally, irradiation of these diamond particles is carried out by high-energy electron (~2 MeV)Citation115–Citation117 or proton (~3 MeV)Citation69,Citation118 beam using Van de Graaff or tandem particle accelerators, respectively. These sources generate vacancies but are difficult to install in usual pharmaceutical or biomedical laboratory settings due to their high cost, complicated setup, and safety issues.Citation19 Hence, to increase the feasibility of the wider production of fluorescent NDs, a moderate scale-energy helium-ion (40 keV) beam emanating from a radio frequency positive-ion source was proposed.Citation19 Even though a much lower energy source was used in this irradiation process, none of the essential fluorescence spectral features were lost, apart from a decrease in fluorescence intensity, when compared with the fluorescence spectra generated by 3 MeV energy proton beam-irradiated particles.Citation19 This method not only benefited the laboratories having ordinary infrastructure in producing fluorescent NDs but also increased the yield of fluorescent NDs by creating a higher number of vacancies compared with electron beam- and proton beam-irradiated NDs.Citation19,Citation118,Citation119
After 2 hours of annealing at 800°C, irradiated synthetic type Ib NDs mainly generated two types of N-V defect centers: neutral nitrogen vacancy (N-V)0, with zero phonon line at 575 nm, and negatively charged nitrogen vacancy (N-V)−, with zero phonon line at ~638 nm.Citation19,Citation88,Citation116 Among all the color centers, (N-V)− dominate the fluorescence spectra of type Ib diamonds.Citation23,Citation117 This defect center absorbs light photons at ~560 nm and fluoresces brightly at ~700 nm with a quantum efficiency close to 1.Citation25,Citation69,Citation120 As such, these centers offer characteristically bright red fluorescence to diamond particles upon excitation with green-yellow light,Citation22 hence have found applications for high-pressure high-temperature NDs in imaging studies.Citation19,Citation25
The extreme photostability of (N-V)− defect centersCitation121,Citation122 provides a greater advantage to NDs to emerge as an excellent candidate for long-term cellular imaging over commonly used fluorophores. Upon continuous exposure to a 100 W lamp power for 480 minutes, the red fluorescent NDs (100 nm) remained stable with no observed photobleaching whereas similarly sized red fluorescent polystyrene nanospheres photobleached within the first 30 minutes of photoexcitation.Citation69 In another instance, no photobleaching occurred in both 35 and 100 nm red fluorescent NDs when photoexcited with 8 kW/cm2 power density of light for 5 minutes.Citation25 In contrast, under the same photoexcitation conditions, the fluorescence of Alexa Fluor® 546 dye conjugated to DNA faded completely in 12 seconds.Citation25 Additionally, the fluorescence of red NDs was found to be significantly brighter than that emitted by Alexa Fluor 546.Citation25 The evidence of photostability has also been determined for a single 7 nm red fluorescent diamond nanocrystal that did not show any sign of photobleaching over 30 seconds.Citation123 In addition to resistance to photobleaching, the fluorescence of NDs (35 and 100 nm in size) was also found to be immune to photoblinking on a time scale of 1 millisecond.Citation25 Moreover, due to the deep location of (N-V)− centers in the lattice structure of NDs,Citation124,Citation125 the fluorescence remains unaffected by surface alterations, as evidenced by NDs functionalized with carboxylic acid,Citation69 polylysine,Citation25 and transferrinCitation126 groups. Therefore, the intrinsic photostability of NDs opens avenues for carrying out chemical modification of their surface for specific imaging applications.
Furthermore, the red fluorescent NDs could be more advantageous than organic fluorophores in obtaining bright contrast images in cells. The absorption and emission wavelength of cellular endogenous components such as flavins, collagens, lipofuscins, and coenzymes, ranging from ~280 to 630 nm,Citation127,Citation128 coincides with most of the organic fluorophores used for cellular imaging.Citation129 Thus, it provides an undesirable, strong background to the fluorescence signal. However, fluorescent NDs can act as an ideal candidate in this case, as emission of the dominant color center of NDs, (N-V)−, occurs above 690 nm,Citation25,Citation69 well separated from the cellular autofluorescence region. In addition, the average fluorescence lifetime of NDs is 17 nanoseconds,Citation25,Citation123 which is considerably longer than the lifetime of fluorescent organic dyes (<5 nanoseconds)Citation130 and most of the cellular endogenous fluorophores (<6 nanoseconds).Citation127 Hence, by time gating,Citation131 it is possible to collect the fluorescence of the NDs alone with minimal background cellular autofluorescence, thereby providing high-contrast imaging of NDs in the cells, which is not feasible with most of the commonly used organic fluorophores.Citation25 lists the advantages of using red fluorescent NDs as a fluorescent tag for in vitro or in vivo tracking applications.
Table 1 Highlights of the unique fluorescence properties of nanodiamonds
Similar to the N-V defect centers of type Ib synthetic diamonds, the nitrogen-vacancy-nitrogen (N-V-N) or H3 defect centersCitation24,Citation132 in type Ia diamond nanocrystals can be created by irradiating them with a high-energy (3 MeV) proton or a medium-energy (40 keV) helium-ion beam followed by annealing at 800°C.Citation87 H3 defect centers have a zero phonon line at 503 nm, with absorption and fluorescence emission at 470 and 530 nm, respectively.Citation22 Therefore, type Ia NDs with H3 centers are known as “green fluorescent NDs.”
Other than the natural and high-pressure high-temperature NDs containing nitrogen impurities, researchers are also trying to understand the fluorescence characteristics of detonation NDs. Considering the actual composition of the explosive mixture (~20 mass% of nitrogen) used to synthesize these particles, they might contain a high concentration of nitrogen,Citation133 either in their core structure or in the form of surface defects. Initially, these nanoparticles were suggested to be devoid of C-centers and believed to be unsuitable for imaging applications.Citation21 The absence of zero phonon lines corresponding to the N-V centers in irradiated and annealed detonation NDs also suggested that structural defects were the origin of their fluorescence.Citation134 Z-contrast scanning transmission microscopy, high-resolution transmission electron microscopy, and electron energy loss spectroscopy identified nitrogen in the core structure of detonation NDs.Citation135 Recently, Vlasov et al detected stable N-V color centers in detonation NDs greater than 30 nm after their irradiation and thermal annealing treatment.Citation133 While the occurrence of N-V color centers in the bulk structure of detonation NDs of less than 10 nm has been questioned in various studies,Citation136,Citation137 Smith et al showed the presence of these centers in single-digit-sized irradiation-damaged and annealed NDs.Citation138 Moreover, N-V color centers have also been discovered in nonirradiated 5 nm detonation NDs.Citation139 The NDs synthesized by the detonation technique are shown to possess up to 3 at% of nitrogen impurities.Citation133,Citation140
NDs, due to their bright fluorescence, high photostability with sufficiently long lifetime and excellent biocompatibility, are emerging as efficient and safe candidates for cellular imaging.
In 2007, Fu et al explored the fluorescent properties of a single 100 nm red fluorescent ND to examine the binding pattern of negatively charged DNA with a positively charged ND.Citation25 First, the molecules of T4 DNA labeled with TOTO-1 fluorophore were allowed to interact electrostatically with polyL-lysine-coated carboxylated fluorescent NDs in a buffer.Citation25 The diluted solution containing a single fluorescent ND and DNA was then added into a microchannel of a coverglass plate terminated with amino groups, followed by DNA stretching using microchannel-combing methodology.Citation25 After laser excitation of the sample at 514 nm, fluorescence was detected using a wide-field epifluorescence microscope equipped with 545 to 605 nm and 675 to 685 nm wavelength channels.Citation25 By overlapping the fluorescence emitted from the TOTO-1 dye and the ND, detected by the shorter wavelength channel, with that of ND alone, detected by the longer wavelength channel, it was discovered that the DNA interacts with the positively charged single ND through a wrapping arrangement ().Citation25 Furthermore, by using the fluorescence intensity of the ND as a control, it was observed that over a period of 13.9 seconds, a 35 nm ND travels within a 1 × 1 μm2 area close to the nucleus of HeLa cells.Citation25 To compare the photostability of TOTO-1 dye with fluorescent ND, samples were excited for 40 seconds at 514 nm.Citation25 No sign of photobleaching was observed in the ND, whereas the dye started fading within the first 5 seconds.Citation25 This study shows that a single fluorescent ND can function as tracking agent and probe to investigate the interaction between biomolecules.
Figure 8 Overlay image (far right) demonstrating the wrapping of TOTO-1 dye-labeled T4DNA (green color, V-shape) around the surface of a single polyL-lysine-coated fluorescent nanodiamond (red color).
Reprinted with permission from: Fu CC, Lee HY, Chen K, et al. Characterization and application of single fluorescent nanodiamonds as cellular biomarkers. Proc Natl Acad Sci U S A. 2007;104(3):727–732.Citation25 Copyright (2007) National Academy of Sciences, USA.
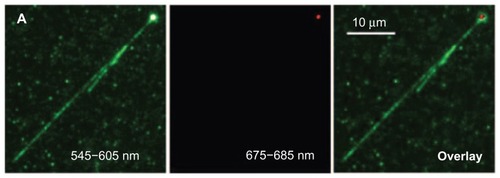
In 2009, Weng et al investigated the uptake mechanism of transferrin in HeLa cells using the fluorescent properties of NDs as a biomolecule label.Citation126 They oxidized 100 nm red fluorescent NDs and conjugated transferrin to their surfaces through an amide linkage to form ND-transferrin conjugates.Citation126 These bioconjugates were then incubated for an hour at a concentration of 10 μg/mL with HeLa cells overexpressing transferrin receptors.Citation126 To further verify the findings, ND-transferrin complexes were added to HeLa cells in which their transferrin receptors were pre-saturated by adding an excess of transferrin.Citation126 Upon excitation of the treated HeLa cell samples using a 514.5 nm laser source, confocal fluorescence images were obtained by collecting fluorescence from 663 to 738 nm, thereby excluding most of the cellular autofluorescence background and providing high-contrast images of NDs in cells ().Citation126 While the successful internalization of transferrin was observed in the HeLa cells overexpressing transferrin receptors, no noticeable uptake occurred in transferrin-receptor pre-saturated HeLa cells, as evidenced by the absence of ND fluorescence emission in the latter.Citation126 Hence, by using the fluorescence of NDs as a marker, it was confirmed that uptake of transferrin in HeLa cells occurs through receptor-mediated endocytosis.Citation126 Moreover, 100 nm NDs did not interfere in the binding of transferrin to its receptor and subsequent cellular uptake.Citation126 Moreover, it was verified that the fluorescence of the NDs originates from the lattice color defect center rather than surface defects, as the surface conjugation of NDs with transferrin and the transferrin-ND–transferrin receptor interactions had no adverse effects on their fluorescence properties.Citation126 Hence, this result shows that NDs can act as an efficient label to examine the cellular uptake mechanism of various biomolecules.
Figure 9 Images of a single HeLa cell treated with ND-transferrin complex: (A) bright-field image, (B) confocal scanning image obtained by collecting all the fluorescence emissions above a wavelength of 550 nm, and (C) confocal scanning image obtained by collecting only the fluorescence emissions at wavelengths of 663–738 nm.
Note: A 514.5 nm laser was used as excitation source.
Reprinted from: Weng MF, Chiang SY, Wang NS, Niu H. Fluorescent nanodiamonds for specifically targeted bioimaging: application to the interaction of transferrin with transferrin receptor. Diam Relat Mater. 2009;18(2–3):587–591.Citation126 Copyright (2008), with permission from Elsevier.
Abbreviation: ND, nanodiamonds.
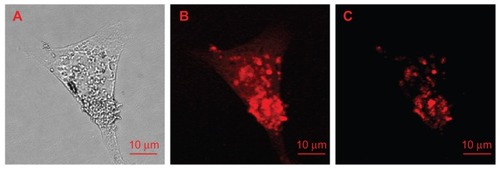
In addition to the various explorations of the red fluorescence of type Ib irradiated/annealed synthetic NDs as cellular biomarkers,Citation25,Citation115,Citation126 in 2009, Mkandawire et al proposed the use of untreated detonation NDs, emitting green fluorescence, for labeling live cells.Citation141 Immunoconjugated ND complexes were prepared by covalently coupling actin and mitochondrial-targeted antibodies to the surface of oxidized NDs.Citation141 The actin antibody-ND and mitochondria antibody-ND immunoconjugates were then allowed internalization by HeLa cells using maltotriose-shelled fourth-generation dendrimers.Citation141 Using the green fluorescence of the NDs as a marker, live-cell fluorescence microscopic images of the treated cells, collected at 535/40 nm wavelength, revealed selective targeting of actin and mitochondria by actin antibody-ND and mitochondria antibody-ND immunoconjugates, respectively.Citation141 With the rational selection of an antibody, NDs could also be designed to image the specific structures of the cells.Citation141
Further to the in vitro studies, in 2010, Mohan et al traced the movement of bare and bioconjugated red fluorescent NDs in Caenorhabditis elegans (), a hermaphrodite with an optically transparent body.Citation142 The worms were deprived of Escherichia coli, and then fed bare and dextran-/bovine serum albumin-conjugated carboxylated NDs.Citation142 The location of NDs in the worms was determined by differential interference and wide-field epifluorescence microscopic images, obtained by collecting the emission from the sample above a wavelength of 600 nm upon excitation with green-yellow light (510–560 nm).Citation142 The bare NDs were found to be localized in the lumen of the worm 12 hours after administration, with no signs of intestinal absorption.Citation142 However, the excretion of these NDs took place within an hour when the worms were fed E. coli.Citation142 In contrast to the bare NDs, a major fraction of the bioconjugated NDs was taken up by the intestinal cells of the worm and remained there for 24 hours, despite E .coli feeding.Citation142 The high intestinal absorption of bioconjugated NDs compared with bare NDs was believed to be due to enhanced dispersibility of the NDs as a result of surface functionalization and/or triggering of certain cellular uptake pathways.Citation142 No photobleaching or photoblinking of NDs was observed, even after 48 hours of continuous excitation with light.Citation142 Furthermore, observation of the lifespan, brood size, reactive oxygen species, and stress response level demonstrated high biocompatibility of the NDs.Citation142 This study shows the potential for the use of the fluorescent properties of NDs in long-term tracking of biomolecules in organisms and as tools for marking human organelles.Citation142
Figure 10 (I) Images of Caenorhabditis elegans: (A) an untreated worm with labeled organ morphology; worms after oral administration of bare nanodiamonds (NDs) for (B) 2 hours and (C) 12 hours; worms fed with bare NDs for 2 hours with subsequent administration of Escherichia coli for (D) 20 minutes (E) and 40 minutes. (B–E) upper show epifluorescence images; lower show epifluorescence and differential interference contrast merged images. (II) Epifluorescence and differential interference contrast merged images of Caenorhabditis elegans: worms after oral administration of (A) dextran-conjugated NDs, (B) bovine serum albumin-conjugated NDs, and worms exposed to Escherichia Coli after administration of (C) dextran-conjugated NDs and (D) bovine serum albumin-conjugated NDs.
Notes: Blue arrows indicate internalization of NDs in intestinal cells; yellow arrow indicate localization of NDs in lumen of worm.
Reprinted with permission from: Mohan N, Chen CS, Hsieh HH, Wu YC, Chang HC. In vivo imaging and toxicity assessments of fluorescent nanodiamonds in Caenorhabditis elegans. Nano Lett. 2010;10(9):3692–3699.Citation142 Copyright (2010) American Chemical Society.
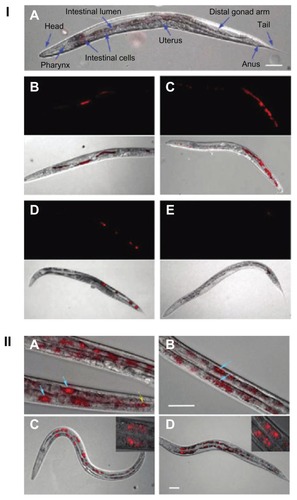
To date, there are a number of publications showing the utilization of ND fluorescence for imaging, mostly in the cells and less commonly in translucent organisms. However, further studies need to be carried out to demonstrate the usefulness of NDs as imaging agents in animals and humans. Imaging organisms that do not possess translucent membranes could pose a challenge.
Elastic and inelastic light scattering imaging
In addition to the fluorescence, NDs have a refractive index of 2.42, thus possess characteristic optical scattering properties.Citation124 This property was explored by Smith et al by obtaining differential interference contrast microscopic images of 55 nm NDs internalized into 3T3 mammalian epithelial cells.Citation143 Based upon the differences in refractive indices, the Rayleigh light scattering of diamond nanocrystals is 300 times higher compared to cellular structures of the same size.Citation144 This offers an advantage in using NDs for obtaining high-contrast imaging in the cells. In addition to elastic scattering, NDs are also capable of scattering light inelastically, hence are Raman active.Citation145,Citation146 The phonon mode of the diamond carbon shows a sharp and distinguished Raman signal at 1332 cm−1,Citation147 providing an opportunity for spectroscopic characterization and mapping of NDs in the cells. Raman spectroscopy is a noninvasive and nontoxic technique, therefore has the potential to be used for the detection and imaging of NDs in biological samples. Also, Raman spectroscopic measurements do not require complicated sample preparation and conditions that are hard to maintain, such as vacuum. Therefore, in addition to the fluorescent tag, NDs can also be used as an optical scattering nanoprobe in cellular studies.
In 2007, Perevedentseva et al used NDs as nanobioprobes to observe biomolecule–bacterial interactions in vivo, using the unique Raman signal of NDs as a detection marker.Citation145 Physical adsorption of lysozymes was performed on carboxylated NDs and the resultant carboxylated ND-lysozyme complex was then allowed to interact with E. coli.Citation145 The optical microscope located the E. coli, while the confocal Raman spectrometer mapped the Raman signals from the NDs.Citation145 As the lysozyme was adsorbed onto the surface of the NDs, the overlay of optical and Raman images revealed the interaction sites of the lysozyme with E .coli ().Citation145 This dual imaging provided a simple method through which to observe the interaction of molecules with living cells.Citation145 Furthermore, there was no reduction in the bactericidal activity of lysozymes upon adsorption onto the NDs’ surface, proving that the NDs did not interfere with the therapeutic activity of the attached entity.Citation145
Figure 11 Lysozymal interaction sites revealed with Escherichia coli: (I) carboxylated nanodiamonds (NDs) and Escherichia coli, (II) carboxylated ND-lysozyme complex and Escherichia coli. (A) Optical images identifying Escherichia coli; (B) confocal Raman mapping identifying NDs present in the square area outlined in optical image; (C) merging of the optical image with Raman mapping, demonstrating the interaction of the lysozymes with Escherichia coli.Citation145
Reproduced from: Perevedentseva E, Cheng CY, Chung PH, Tu JS, Hsieh YH, Cheng CL. The interaction of the protein lysozyme with bacteria E. coli observed using nanodiamond labelling. Nanotechnology. 2007;18(31):315102,Citation145 courtesy of IOP Publishing Ltd.
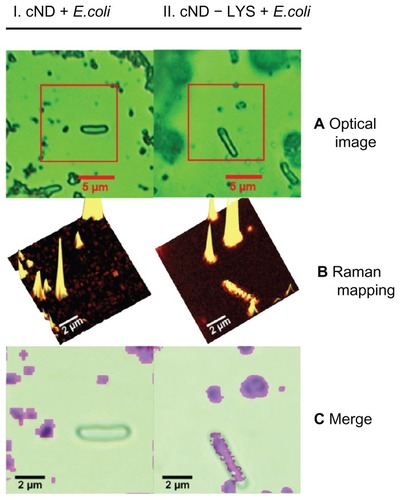
In 2007, the same group investigated the use of NDs to predict the location of growth-hormone receptors in human lung cancer cells by identifying the sp3 carbon Raman signals of NDs.Citation146 Growth hormone-ND complexes were created by carboxylating and covalently linking 100 nm NDs to the growth hormone.Citation146 When mapped by confocal Raman spectrophotometry, the NDs bound to growth hormone were located on the surface of cells, while the unconjugated carboxylated NDs were internalized into the cells.Citation146 These observations verified the extracellular location of the growth-hormone receptor using the Raman signal of the ND as a label.Citation146 This study provided a relatively easy and direct method of locating receptors at the cellular level. This method could be beneficial in investigating the receptor-mediated internalization of biomolecules and diagnosing diseases such as cancers involving the alteration in expression of certain receptors.Citation146
Conclusion
Continued investigation and understanding of disaggregation methods, surface properties, functionalization, biocompatibility, cellular fate, fluorescence, and the optical scattering characteristics of NDs have opened new horizons for their applications in the biomedical field. NDs have shown great potential to emerge as a platform for delivering drugs into biological systems due to their biocompatibility, high loading capacity, and ability to cross cellular membranes. These particles have imparted sustained-release and hydrophilic characteristics to water insoluble drugs, and have opened up the possibilities for systemic administration of highly effective, hydrophobic chemotherapeutic molecules. While N-V defect centers are responsible for conferring fluorescence to NDs, the scattering properties originate from their high refractive index and Raman optical activity. These properties provide imaging capabilities to NDs for their detection in the cell. The multifunctional characteristics of NDs can be utilized to improve the delivery of drugs in conjunction with imaging their fate in biological systems, which can revolutionize studies in the area of life sciences. This unique biomaterial could be further explored as a gene delivery vehicle and a label to identify the biomolecular targets of drugs.
Acknowledgments
The authors thank Dr Ramaswami Sammynaiken (Saskatchewan Structural Sciences Centre, University of Saskatchewan) and Dr Ronald E Verrall (Department of Chemistry, University of Saskatchewan) for their valuable suggestions for improving the manuscript.
Disclosure
The authors report no conflicts of interest in this work.
References
- ShenderovaOAMcGuireGTypes of nanocrystalline diamondShenderovaOAGruenDMUltrananocrystalline Diamond: Synthesis Properties and ApplicationsNew York, NYWilliam Andrew200679114
- MochalinVNShenderovaOHoDGogotsiYThe properties and applications of nanodiamondsNat Nanotechnol201271112322179567
- GracioJJFanQHMadalenoJCDiamond growth by chemical vapour depositionJ Phys D Appl Phys201043374017
- PhilipJHessPFeygelsonTElastic, mechanical, and thermal properties of nanocrystalline diamond filmsJ Appl Phys200393421642171 Available at: http://dx.doi.org/10.1063/1.1537465
- ZhouDGruenDMQinLCMcCauleyTGKraussARControl of diamond film microstructure by Ar additions to CH4/H2 microwave plasmasJ Appl Phys199884419811989
- QinLCZhouDKraussARGruenDMTEM characterization of nanodiamond thin filmsNanostructured Materials1998104649660
- EspinosaHDPengBProrokBCFracture strength of ultrananocrystalline diamond thin films – identification of Weibull parametersJ App Phys200394960766084
- AngadiMAWatanabeTBodapatiAThermal transport and grain boundary conductance in ultrananocrystalline diamond thin filmsJ Appl Phys20069911114301114306
- ButlerJESumantAVThe CVD of nanodiamond materialsChemical Vapor Deposition2008147–8145160
- SumantAVGriersonDSGerbiJECarlisleJAAucielloOCarpickRWSurface chemistry and bonding configuration of ultrananocrystalline diamond surfaces and their effects on nanotribological propertiesPhys Rev B Condens Matter Mater Phys20077623235429-1235429-11 Available from http://link.aps.org/doi/10.1103/PhysRevB.76.235429Accessed 24 August 2012.
- BajajPAkinDGuptaAUltrananocrystalline diamond film as an optimal cell interface for biomedical applicationsBiomed Microdevices20079678779417530409
- ShiBJinQChenLAucielloOFundamentals of ultrananocrystalline diamond (UNCD) thin films as biomaterials for developmental biology: Embryonic fibroblasts growth on the surface of (UNCD) filmsDiam Relat Mater2009182–3596600
- KraussARAucielloOGruenDMUltrananocrystalline diamond thin films for MEMS and moving mechanical assembly devicesDiam Relat Mater2001101119521961
- AucielloOBirrellJCarlisleJAMaterials science and fabrication processes for a new MEMS technology based on ultrananocrystalline diamond thin filmsJ Phys Condens Matter20041616R539R552
- AucielloOSumantAVStatus review of the science and technology of ultrananocrystalline diamond (UNCD™) films and application to multifunctional devicesDiam Relat Mater2010197–9699718
- SumantAVAucielloOCarpickRWSrinivasanSButlerJEUltrananocrystalline and nanocrystalline diamond thin films for MEMS/NEMS applicationsMRS Bull2010354281288 Available from: http://journals.cambridge.org/action/displayAbstract?fromPage=online&aid=7944009
- HupertMMuckAWangJConductive diamond thin-films in electrochemistryDiam Relat Mater20031210–1119401949
- YangWAucielloOButlerJEDNA-modified nanocrystalline diamond thin-films as stable, biologically active substratesNat Mater20021425325712618788
- ChangYRLeeHYChenKMass production and dynamic imaging of fluorescent nanodiamondsNat Nanotechnol20083528428818654525
- SonnefraudYCucheAFaklarisODiamond nanocrystals hosting single nitrogen-vacancy color centers sorted by photon-correlation near-field microscopyOpt Lett200833661161318347726
- BoudouJPCurmiPAJelezkoFHigh yield fabrication of fluorescent nanodiamondsNanotechnology2009202323560219451687
- ChangHCDevelopment and use of fluorescent nanodiamonds as cellular markerHoDNanodiamonds: Applications in Biology and Nanoscale MedicineNew York, NYSpringer2009127150
- DaviesGLawsonSCCollinsATMainwoodASharpSJVacancy-related centers in diamondPhys Rev B Condens Matter19924620131571317010003356
- ClarkCDNorrisCAPhotoluminescence associated with the 1.673, 1.944 and 2.498 eV centres in diamondJournal of Physics: C Solid State Phys197141422232229
- FuCCLeeHYChenKCharacterization and application of single fluorescent nanodiamonds as cellular biomarkersProc Natl Acad Sci U S A2007104372773210.1073/pnas.060540910417213326
- DolmatovVYDetonation synthesis ultradispersed diamonds: properties and applicationsRussian Chemical Reviews2001707607626
- DolmatovVYSynthesis and post-synthesis treatment of detonation nanodiamondsShenderovaOAGruenDMUltrananocrystalline Diamond: Synthesis, Properties, and ApplicationsNew York, NYWilliam Andrew2006347377
- ShenderovaOAZhirnovVVBrennerDWCarbon nanostructuresCritical Reviews in Solid State and Materials Sciences2002273–4227356
- BaidakovaMVulANew prospects and frontiers of nanodiamond clustersJ Phys D Appl Phys200740206300631110.1088/0022-3727/40/20/S14.
- AleksenskiiAEBaidakovaMVVul’AYSiklitskiiVIThe structure of diamond nanoclustersPhy Solid State1999414668671
- ShenderovaOAHensSACDetonation nanodiamond particles processing, modification and bioapplicationsHoDNanodiamonds: Applications in Biology and Nanoscale MedicineNew York, NYSpringer201079116
- PichotVCometMFoussonEAn efficient purification method for detonation nanodiamondsDiam Relat Mater20081711322
- OsswaldSYushinGMochalinVKucheyevSOGogotsiYControl of sp2/sp3 carbon ratio and surface chemistry of nanodiamond powders by selective oxidation in airJ Am Chem Soc200612835116351164216939289
- PetrovIShenderovaOGrishkoVDetonation nanodiamonds simultaneously purified and modified by gas treatmentDiam Relat Mater2007161220982103
- KrügerAKataokaFOzawaMUnusually tight aggregation in detonation nanodiamond: identification and disintegrationCarbon200543817221730
- KruegerAThe structure and reactivity of nanoscale diamondJ Mater Chem2008181314851492
- JiangTXuKFTIR study of ultradispersed diamond powder synthesized by explosive detonationCarbon1995331216631671
- Yur’evGSDolmatovVYX-ray diffraction study of detonation nanodiamondsJournal of Superhard Materials2010325311328
- HawelekLBrodkaADoreJCHonkimakiVTomitaSBurianAStructural studies of nanodiamond by high-energy X-ray diffractionDiam Relat Mater2008177–1011861193
- DolmatovVYOn elemental composition and crystal-chemical parameters of detonation nanodiamondsJournal of Superhard Materials2009313158164
- MykhaylykOOSoloninYMBatchelderDNBrydsonRTransformation of nanodiamond into carbon onions: A comparative study by high-resolution transmission electron microscopy, electron energy-loss spectroscopy, x-ray diffraction, small-angle x-ray scattering, and ultraviolet Raman spectroscopyJ Appl Phys2005977074302-1074302-16
- DolmatovVYDetonation nanodiamonds in oils and lubricantsJournal of Superhard Materials20103211420
- SchrandAMHensSAShenderovaOANanodiamond particles: properties and perspectives for bioapplicationsCritical Reviews in Solid State and Materials Sciences2009341–21874
- BondarVSPozdnyakovaIOPuzyrAPApplications of nanodiamonds for separation and purification of proteinsPhysics of the Solid State2004464758760
- PurtovKVPuzyrAPBondarVSNanodiamond sorbents: new carriers for column chromatography of proteinsDokl Biochem Biophys2008419727418505161
- DolmatovVYApplications of detonation nanodiamondShenderovaOAGruenDMUltrananocrystalline Diamond: Synthesis, Properties, and ApplicationsNew York, NYWilliam Andrew2006477527
- PentecostAGourSMochalinVKnokeIGogotsiYDeaggregation of nanodiamond powders using salt- and sugar-assisted millingACS Appl Mater Interfaces20102113289329421043470
- KrügerALiangYJarreGStegkJSurface functionalisation of detonation diamond suitable for biological applicationsJ Mater Chem2006162423222328
- BarnardASSelf-assembly in nanodiamond agglutinatesJ Mater Chem2008183440384041
- ChangLYÔsawaEBarnardASConfirmation of the electrostatic self-assembly of nanodiamondsNanoscale20113395896221258697
- OzawaMInagumaMTakahashiMKataokaFKrügerAÔsawaEPreparation and behavior of brownish, clear nanodiamond colloidsAdv Mater200719912011206
- LiangYOzawaMKruegerAA general procedure to functionalize agglomerating nanoparticles demonstrated on nanodiamondACS Nano2009382288229619601635
- ÔsawaERecent progress and perspectives in single-digit nanodiamondDiam Relat Mater2007161220182022
- EidelmanEDSiklitskyVISharonovaLVA stable suspension of single ultrananocrystalline diamond particlesDiam Relat Mater20051411–1217651769
- SuslickKSHammertonDAClineREThe sonochemical hot spotJ Am Chem Soc19861081856415642
- AleksenskiiAEBaidakovaMVVul’AYDavydovVYPevtsovaYADiamond-graphite phase transition in ultradisperse-diamond clustersPhysics of the Solid State199739610071015
- XuXYuZZhuYWangBEffect of sodium oleate adsorption on the colloidal stability and zeta potential of detonation synthesized diamond particles in aqueous solutionsDiam Relat Mater2005142206212
- MoritaYTakimotoTYamanakaHA facile and scalable process for size-controllable separation of nanodiamond particles as small as 4 nmSmall20084122154215718989864
- TsubotaTTaniiSIdaSNagataMMatsumotoYChemical modification of diamond surface with various carboxylic acids by radical reaction in liquid phaseDiam Relat Mater2004134–810931097
- IdaSTsubotaTTaniiSNagataMMatsumotoYChemical modification of the diamond surface using benzoyl peroxide and dicarboxylic acidsLangmuir2003192396939698
- AndoTNishitani-GamoMRawlesREYamamotoKKamoMSatoYChemical modification of diamond surfaces using a chlorinated surface as an intermediate stateDiam Relat Mater199651011361142
- LiuYGuZMargraveJLKhabasheskuVNFunctionalization of nanoscale diamond powder: fluoro-, alkyl-, amino-, and amino acid-nanodiamond derivativesChem Mater2004162039243930
- KruegerABoedekerTDeagglomeration and functionalisation of detonation nanodiamond with long alkyl chainsDiam Relat Mater2008177–1013671370
- KruegerAOzawaMJarreGLiangYStegkJLuLDeagglomeration and functionalisation of detonation diamondPhysica Status Solidi (A): Applications and Materials2007204928812887
- KruegerAStegkJLiangYLuLJarreGBiotinylated nanodiamond: simple and efficient functionalization of detonation diamondLangmuir20082484200420418312008
- KaurRChitandaJMMichelDLysine-functionalized nanodiamonds: synthesis, physiochemical characterization, and nucleic acid binding studiesInt J Nanomedicine201273851386622904623
- SchrandAMHuangHCarlsonCAre diamond nanoparticles cytotoxic?J Phys Chem B200711112717201422
- SchrandAMDaiLSchlagerJJHussainSMOsawaEDifferential biocompatibility of carbon nanotubes and nanodiamondsDiam Relat Mater2007161221182123
- YuSJKangMWChangHCChenKMYuYCBright fluorescent nanodiamonds: no photobleaching and low cytotoxicityJ Am Chem Soc200512750176041760516351080
- LiuKKChengCLChangCCChaoJIBiocompatible and detectable carboxylated nanodiamond on human cellNanotechnology20071832325102
- ChaoJIPerevedentsevaEChungPHNanometer-sized diamond particle as a probe for biolabelingBiophys J20079362199220817513352
- HuangHPierstorffEOsawaEHoDActive nanodiamond hydrogels for chemotherapeutic deliveryNano Lett20077113305331417918903
- LiJZhuYLiWZhangXPengYHuangQNanodiamonds as intracellular transporters of chemotherapeutic drugBiomaterials201031328410841820692696
- XingYXiongWZhuLOsawaEHussinSDaiLDNA damage in embryonic stem cells caused by nanodiamondsACS Nano2011532376238421370893
- MarconLRiquetFVicogneDSzuneritsSBodartJFBoukherroubRCellular and in vivo toxicity of functionalized nanodiamond in Xenopus embryosJ Mater Chem2010203780648069
- LiuKKWangCCChengCLChaoJIEndocytic carboxylated nanodiamond for the labeling and tracking of cell division and differentiation in cancer and stem cellsBiomaterials200930264249425919500835
- ZhuLChangDWDaiLHongYDNA damage induced by multiwalled carbon nanotubes in mouse embryonic stem cellsNano Lett20077123592359718044946
- ZhuYLiWLiQEffects of serum proteins on intracellular uptake and cytotoxicity of carbon nanoparticlesCarbon200947513511358
- DrescherDOrts-GilGLaubeGToxicity of amorphous silica nanoparticles on eukaryotic cell model is determined by particle agglomeration and serum protein adsorption effectsAnal Bioanal Chem201140051367137321479547
- KittlerSGreulichCGebauerJSThe influence of proteins on the dispersability and cell-biological activity of silver nanoparticlesJ Mater Chem2010203512518
- CaseyADavorenMHerzogELyngFMByrneHJChambersGProbing the interaction of single walled carbon nanotubes within cell culture medium as a precursor to toxicity testingCarbon20074513440
- GuoLVon Dem BusscheABuechnerMYanAKaneABHurtRHAdsorption of essential micronutrients by carbon nanotubes and the implications for nanotoxicity testingSmall20084672172718504717
- PuzyrAPBaronAVPurtovKVNanodiamonds with novel properties: a biological studyDiam Relat Mater2007161221242128
- YuanYWangXJiaGPulmonary toxicity and translocation of nanodiamonds in miceDiam Relat Mater2010194291299
- ChowEKZhangXQChenMNanodiamond therapeutic delivery agents mediate enhanced chemoresistant tumor treatmentSci Transl Med201137373ra21
- FaklarisOGarrotDJoshiVDetection of single photoluminescent diamond nanoparticles in cells and study of the internalization pathwaySmall20084122236223918989862
- WeeTLMauYWFangCYHsuHLHanCCChangHCPreparation and characterization of green fluorescent nanodiamonds for biological applicationsDiam Relat Mater2009182–3567573
- FaklarisOJoshiVIrinopoulouTPhotoluminescent diamond nanoparticles for cell labeling: study of the uptake mechanism in mammalian cellsACS Nano20093123955396219863087
- MartínRÁlvaroMHeranceJRGarcíaHFenton-treated functionalized diamond nanoparticles as gene delivery systemACS Nano201041657420047335
- LiXShaoJQinYShaoCZhengTYeLTAT-conjugated nanodiamond for the enhanced delivery of doxorubicinJ Mater Chem2011212279667973
- LiuKKZhengWWWangCCCovalent linkage of nanodiamond-paclitaxel for drug delivery and cancer therapyNanotechnology2010213131510620634575
- ChenMPierstorffEDLamRNanodiamond-mediated delivery of water-insoluble therapeuticsACS Nano2009372016202219534485
- MohanrajVJChenYNanoparticles – a reviewTrop J Pharm Res200651561573
- SinhaRKimGJNieSShinDMNanotechnology in cancer therapeutics: bioconjugated nanoparticles for drug deliveryMol Cancer Ther2006581909191716928810
- GogaAYangDTwardADMorganDOBishopJMInhibition of CDK1 as a potential therapy for tumors over-expressing MYCNat Med200713782082717589519
- NayfieldSGKarpJEFordLGDorrFAKramerBSPotential role of tamoxifen in prevention of breast cancerJ Natl Cancer Inst19918320145014591920492
- FisherBCostantinoJPWickerhamDLTamoxifen for the prevention of breast cancer: current status of the National Surgical Adjuvant Breast and Bowel Project P-1studyJ Natl Cancer Inst200597221652166216288118
- LiangXJChenCZhaoYWangPCCircumventing tumor resistance to chemotherapy by nanotechnologyMethods Mol Biol201059646748819949937
- PeerDKarpJMHongSFarokhzadOCMargalitRLangerRNanocarriers as an emerging platform for cancer therapyNat Nanotechnol200721275176018654426
- ChidambaramMManavalanRKathiresanKNanotherapeutics to overcome conventional cancer chemotherapy limitationsJ Pharm Pharm Sci2011141677721501554
- ShimkunasRARobinsonELamRNanodiamond-insulin complexes as pH-dependent protein delivery vehiclesBiomaterials200930295720572819635632
- LiuYPetreacaMYaoMMartins-GreenMCell and molecular mechanisms of keratinocyte function stimulated by insulin during wound healingBMC Cell Biol200910119134226
- SchneiderLAKorberAGrabbeSDissemondJInfluence of pH on wound-healing: a new perspective for wound-therapy?Arch Dermatol Res2007298941342017091276
- WiethoffCMMiddaughCRBarriers to nonviral gene deliveryJ Pharm Sci200392220321712532370
- GaryDJPuriNWonYYPolymer-based siRNA delivery: perspectives on the fundamental and phenomenological distinctions from polymer-based DNA deliveryJ Control Release20071211–2647317588702
- WhiteheadKALangerRAndersonDGKnocking down barriers: advances in siRNA deliveryNat Rev Drug Discov20098212913819180106
- TakahashiYNishikawaMTakakuraYNonviral vector-mediated RNA interference: its gene silencing characteristics and important factors to achieve RNAi-based gene therapyAdv Drug Deliv Rev200961976076619386274
- van de WaterFMBoermanOCWouterseACPetersJGRusselFGMasereeuwRIntravenously administered short interfering RNA accumulates in the kidney and selectively suppresses gene function in renal proximal tubulesDrug Metab Dispos20063481393139716714375
- ZhangXQChenMLamRXuXOsawaEHoDPolymer-functionalized nanodiamond platforms as vehicles for gene deliveryACS Nano2009392609261619719152
- ChenMZhangXQManHBLamRChowEKHoDNanodiamond vectors functionalized with polyethylenimine for siRNA deliveryJ Phys Chem Lett201012131673171
- RamdasAKElectronic excitations in isotopically controlled diamonds: infrared and Raman spectroscopy of acceptor-bound holesNazaréMHNevesAJProperties, Growth and Applications of DiamondLondonINSPEC20012127
- KiflawiILawsonSCAggregates of nitrogen in diamondNazaréMHNevesAJProperties, Growth and Applications of DiamondLondonINSPEC2001130133
- VaijayanthimalaVChangHCFunctionalized fluorescent nanodiamonds for biomedical applicationsNanomedicine (Lond)200941475519093895
- MainwoodANitrogen and nitrogen-vacancy complexes and their formation in diamondPhys Rev B Condes Matter1994491279347940
- NeugartFZappeAJelezkoFDynamics of diamond nanoparticles in solution and cellsNano Lett20077123588359117975943
- TreussartFJacquesVWuEGacoinTGrangierPRochJFPhotoluminescence of single colour defects in 50 nm diamond nanocrystalsPhysica B Condens Matter2006376–377926929
- DaviesGHamerMFOptical studies of the 1.945 eV vibronic band in diamondProc R Soc Lond A Mat Phys Sci19763481653285298
- WeeTLTzengYKHanCCTwo-photon excited fluorescence of nitrogen-vacancy centers in proton-irradiated type Ib diamondJ Phys Chem A2007111389379938617705460
- LawsonSCFisherDHuntDCNewtonMEOn the existence of positively charged single-substitutional nitrogen in diamondJ Phys Condens Matter1998102761716180
- HuiYYChangYRMohanNLimTSChenYYChangHCPolarization modulation spectroscopy of single fluorescent nanodiamonds with multiple nitrogen vacancy centersJ Phys Chem A2011115101878188421332226
- GruberADräbenstedtATietzCFleuryLWrachtrupJvon BorczyskowskiCScanning confocal optical microscopy and magnetic resonance on single defect centersScience1997276532120122014
- CollinsATThomazMFJorgeMILuminescence decay time of the 1.945 eV centre in type Ib diamondJournal of Physics C: Solid State Physics1983161121772181
- TislerJBalasubramanianGNaydenovBFluorescence and spin properties of defects in single digit nanodiamondsACS Nano2009371959196521452865
- HuiYYChengCLChangHCNanodiamonds for optical bioimagingJ Phys D Appl Phys20104337374021
- GaliAFytaMKaxirasEAb initio supercell calculations on nitrogen-vacancy center in diamond: its electronic structure and hyperfine tensorsPhys Rev B20087715155206
- WengMFChiangSYWangNSNiuHFluorescent nanodiamonds for specifically targeted bioimaging: application to the interaction of transferrin with transferrin receptorDiam Relat Mater2009182–358759110.1016/j.diamond.2008.07.012
- KoenigKSchneckenburgerHLaser-induced autofluorescence for medical diagnosisJ Fluoresc1994411740
- AubinJEAutofluorescence of viable cultured mammalian cellsJ Histochem Cytochem19792713643220325
- LichtmanJWConchelloJAFluorescence microscopyNat Methods200521291091916299476
- Resch-GengerUGrabolleMCavaliere-JaricotSNitschkeRNannTQuantum dots versus organic dyes as fluorescent labelsNat Methods20085976377518756197
- DahanMLaurenceTPinaudFTime-gated biological imaging by use of colloidal quantum dotsOpt Lett2001261182582718040463
- DaviesGThe effect of nitrogen impurity on the annealing of radiation damage in diamondJ Phys C Solid State Phys197251725342542
- VlasovIIShenderovaOTurnerSNitrogen and luminescent nitrogen-vacancy defects in detonation nanodiamondSmall20106568769420108229
- BorjanovicVLawrenceWGHensSEffect of proton irradiation on photoluminescent properties of PDMS-nanodiamond compositesNanotechnology2008194545570121832790
- KvitAVZhirnovVVTylerTHrenJJAging effect and nitrogen distribution in diamond nanoparticlesComposites Part B Eng2004352163166
- VlasovIIBarnardASRalchenkoVGNanodiamond photoemitters based on strong narrow-band luminescence from silicon-vacancy defectsAdv Mater2009217808812
- BarnardASSternbergMCan we predict the location of impurities in diamond nanoparticles?Diam Relat Mater2007161220782082
- SmithBRInglisDWSandnesBFive-nanometer diamond with luminescent nitrogen-vacancy defect centersSmall20095141649165319334016
- BradacCGaebelTNaidooNObservation and control of blinking nitrogen-vacancy centres in discrete nanodiamondsNat Nanotechnol20105534534920383128
- TurnerSLebedevOIShenderovaOVlasovIIVerbeeckJVan TendelooGDetermination of size, morphology, and nitrogen impurity location in treated detonation nanodiamond by transmission electron microscopyAdv Funct Mater2009191321162124
- MkandawireMPohlAGubarevichTSelective targeting of green fluorescent nanodiamond conjugates to mitochondria in HeLa cellsJ Biophotonics200921059660619504515
- MohanNChenCSHsiehHHWuYCChangHCIn vivo imaging and toxicity assessments of fluorescent nanodiamonds in Caenorhabditis elegansNano Lett20101093692369910.1021/nl102190920677785
- SmithBRNiebertMPlakhotnikTZvyaginAVTransfection and imaging of diamond nanocrystals as scattering optical labelsJ Lumin20071271260263
- ColpinYSwanAZvyaginAVPlakhotnikTImaging and sizing of diamond nanoparticlesOpt Lett200631562562716570419
- PerevedentsevaEChengCYChungPHTuJSHsiehYHChengCLThe interaction of the protein lysozyme with bacteria E. coli observed using nanodiamond labellingNanotechnology2007183131510210.1088/0957-4484/18/31/315102
- ChengCYPerevedentsevaETuJSDirect and in vitro observation of growth hormone receptor molecules in A549 human lung epithelial cells by nanodiamond labelingAppl Phys Lett20079016163903
- KnightDSWhiteWBCharacterization of diamond films by Raman spectroscopyJ Mater Res198942385393