Abstract
Purpose
Pretargeting of biomarkers with nanoparticles in molecular imaging is promising to improve diagnostic specificity and realize signal amplification, but data regarding its targeting potential in magnetic resonance (MR) imaging are limited. The purpose of this study was to evaluate the tumor angiogenesis targeting efficacy of the anti-αvβ3 antibody guided three-step pretargeting approach with magnetoliposomes.
Methods
Polyethylene glycol-modified and superparamagnetic iron oxide-encapsulated magnetoliposomes with and without biotin were synthesized and characterized. The cytotoxicity of both probes was evaluated using the methyl thiazdyl tetrazolium assay, and their cellular uptake by mouse macrophage was visualized using Prussian blue staining. Three-step pretargeting MR imaging was performed on MDA-MB-435S breast cancer-bearing mice by intravenous administration of biotinylated anti-αvβ3 monoclonal antibodies (first step), followed by avidin and streptavidin (second step), and by biotinylated magnetoliposomes or magnetoliposomes in the targeted or nontargeted group, respectively (third step). The specificity of αvβ3 targeting was assessed by histologic examinations.
Results
The developed magnetoliposomes were superparamagnetic and biocompatible as confirmed by cell toxicity assay. The liposomal bilayer and polyethylene glycol modification protected Fe3O4 cores from uptake by macrophage cells. MR imaging by three-step pretargeting resulted in a greater signal enhancement along the tumor periphery, occupying 7.0% of the tumor area, compared with 2.0% enhancement of the nontargeted group (P < 0.05). Histologic analysis demonstrated the targeted magnetoliposomes colocalized with neovasculature, which was responsible for the MR signal decrease.
Conclusion
These results indicate that our strategy for MR imaging of αvβ3-integrin is an effective means for sensitive detection of tumor angiogenesis, and may provide a targetable nanodelivery system for anticancer drugs.
Introduction
Tumor angiogenesis, which exhibits the structure and functional abnormalities of blood vessels, plays an important role in tumor growth and development.Citation1 In the progress of angiogenesis, the expression of αvβ3-integrin is upregulated in activated neovascular endothelial cells and several tumor cells, but not in mature, quiescent endothelial cells and normal cells, which makes αvβ3-integrin a highly selective biomarker for tumor angiogenesis.Citation2 Therefore, molecular imaging for overexpression of integrin αvβ3 would facilitate early detection of cancer, evaluation of tumor progression, and cancer therapeutic response.
Much research has focused on the development of diagnostic nanoprobes targeted to αvβ3-integrin receptors to image tumor vasculature by single-photon emission computed tomography,Citation3 positron emission tomography,Citation4 optical imaging,Citation5 or ultrasound.Citation6 Although these imaging modalities are effective and sensitive, the low spatial resolution restricts the exact localization of the expression sites. Magnetic resonance (MR) imaging has been recognized as a powerful diagnostic technique to provide high spatial resolution and excellent soft-tissue contrast. Nevertheless, MR imaging suffers from its major inadequacy – low sensitivity – thus, contrast agents with high affinity and selectivity are necessary for targeting in MR imaging.
Superparamagnetic iron oxide nanoparticles (SPIONs), which can produce predominant T2 relaxation effects on T2 images and especially T2* weighted sequences (negative contrast), have widely been applied to cellular and molecular MR imaging.Citation7,Citation8 They can be effective in nanomolar concentrations due to their high susceptibility;Citation9 thus, they overcome the disadvantage of the low sensitivity of conventional contrast agents. Bare SPIONs generally have hydrophobic surfaces with a large surface area to volume ratio. They tend to aggregate and quickly be taken up by macrophages and accumulated in the reticuloendothelial system (RES) after injection.Citation10 One possible approach to avoid capture by RES is synthesis of new contrast agents coated with biocompatible materials. Liposomes have been extensively used as drug carriers, which can be defined as spherical, self-closed structures formed by concentric lipid bilayers with an aqueous phase inside.Citation11 Liposomes entrapping SPIONs in the aqueous lumen exhibit prolonged circulation times and more favorable pharmacokinetic distribution properties.Citation12 However, after being coated with nonmagnetic materials, the relaxivity of the contrast agents is lowered, which results in a decrease of sensitivity.
The pretargeting approach based on the avidin–biotin system, exploiting the high specificity and strong affinity (Ka = 10−15 mol/L) of avidin (or streptavidin [SA]) for biotin, may provide a strategy to improve the sensitivity and specificity of MR imaging. The feasibility of the three-step procedure has been demonstrated in cancer imaging and radioimmunotherapy.Citation13,Citation14 The target-specific biotinylated antibodies are injected first to localize onto tumors, followed by avidin, and finally biotinylated effector molecules are administered to bind to tumor pretargeting antibodies. Also, avidin/SA has four sites which can conjugate with biotin, and the avidin–biotin binding is essentially irreversible;Citation15 thus, this approach provides an amplification of the signal from the tumor with high specificity. Nevertheless, there is, to the authors’ knowledge, no report so far that has successfully characterized tumor angiogenesis via the MR-imaging strategy.
In the present study, polyethylene glycol (PEG)-modified and SPIONs-based magnetoliposomes (ML) were prepared (). The synthesis, characterization, cytotoxicity assay, and cellular uptake by mouse macrophage of ML are reported. Furthermore, the targeting potential of the antibody-guided three-step pretargeting approach to detect angiogenesis was investigated by MR imaging in vivo and histologic examinations.
Figure 1 Schematic representation of a multimodal SPION-based biotinylated magnetoliposome.
Abbreviations: SPIONs, superparamagnetic iron oxide nanoparticles; DSPG, 1,2-Distearoyl-sn-glycero-3-phospho-rac-glycerol, sodium salt; Biotin-PEG2000-DSPE, 1,2-distearoyl-sn-glycero-3-phosphoethanol-amine-N- [biotinyl (polyethylene glycol)2000]; Rhodamine-DHPE, Lissamine™ rhodamine B 1,2-dihexadecanoyl-sn-glycero-3-phosphoethanolamine, triethylammonium salt.
![Figure 1 Schematic representation of a multimodal SPION-based biotinylated magnetoliposome.Abbreviations: SPIONs, superparamagnetic iron oxide nanoparticles; DSPG, 1,2-Distearoyl-sn-glycero-3-phospho-rac-glycerol, sodium salt; Biotin-PEG2000-DSPE, 1,2-distearoyl-sn-glycero-3-phosphoethanol-amine-N- [biotinyl (polyethylene glycol)2000]; Rhodamine-DHPE, Lissamine™ rhodamine B 1,2-dihexadecanoyl-sn-glycero-3-phosphoethanolamine, triethylammonium salt.](/cms/asset/40cf7397-58d4-4bc5-8a16-b00ca90db7a4/dijn_a_38678_f0001_c.jpg)
Materials and methods
Materials
1,2-Distearoyl-sn-glycero-3-phospho-rac-glycerol, sodium salt (DSPG) was purchased from Lipoid GmbH (Ludwigshafen, Germany). 1,2-distearoyl-sn-glycero-3-phosphoethanol-amine-N- [biotinyl (polyethylene glycol)2000] (Biotin-PEG2000-DSPE) and 1,2-distearoyl-sn-glycero-3 -phosphoethanolamine-N-(methoxy [polyethylene glycol]-2000) (DSPE-PEG-2000) were obtained from Avanti Polar Lipids (Alabaster, AL, USA). Lissamine™ rhodamine B 1,2-dihexadecanoyl -sn-glycero-3- phosphoethanolamine, triethylammonium salt (Rho-DHPE) was from Life Technologies (Carlsbad, CA, USA). 2-(Tris (hydroxymethyl)methylamino) ethane-1-sulphonic acid (TES), methyl thiazdyl tetrazolium (MTT), dimethyl sulfoxide, and paraformaldehyde were from Sigma-Aldrich (St Louis, MO, USA). Cholesterol was purchased from Pubo Chemical Co, Ltd (Guangzhou, China). Streptavidin was obtained from Ruisi Biological Reagents Co, Ltd (Guangzhou, China). Ferric chloride (FeCl3 · 6H2O), ferrous sulfate (FeSO4 · 7H2O), concentrated hydrochloric acid (28% NH4 · OH in water solution), and lauric acid were purchased from Guangzhou Chemical Reagents Co, Ltd (Guangzhou, China). All other chemicals were of analytical grade.
Preparation of SPIONs
Synthesis of SPIONs was done according to the literature,Citation16 with slight modifications. In general, the synthesis proceeded as follows: appropriate amounts of FeSO4 · 7aq (2.78 g) and FeCl3 · 6aq (5.4 g) were mixed at a 1:2 molar ratio and transferred to a three-necked flask, to which 15 mL of ammonia water was added gradually under vigorous stirring. The reaction was maintained at 60°C for 15 minutes to obtain Fe3O4 nanoparticles (NPs). The black precipitates were isolated by applying a permanent magnet to the Fe3O4 NPs, then washing with 50 mL of ammonia/water (5/95, v/v) three times in sequence, then the particles were combined with 1 g of lauric acid (dissolved in 50 mL of ammonia/water [5/95, v/v]), reacted at 90°C for several minutes, and finally lauric acid-stabilized SPIONs were obtained.
Preparation of ML
Liposomes were prepared by a thin lipid film hydration method. DSPG, cholesterol, and biotin-PEG2000-DSPE with a molar ratio of 3:2:0.15 were dissolved in chloroform. Nonbiotinylated control liposomes contained DSPG, cholesterol, and PEG2000-DSPE at a molar ratio of 3:2:0.15. As a fluorescent marker, 0.2 mol% of Rho-DHPE was added. The solvent was removed by rotary evaporation, followed by additional drying under nitrogen. The dry lipid film was hydrated in 10 mL TES (5 microM, pH 7.0). Subsequently, the mixture was dispersed by ultrasonic cleaner (200 W, 10 minutes) and then sonicated by ultrasonic disintegrator (150 W, 30 minutes). The phospholipid vesicle was purified by centrifugation at 37°C for 20 minutes at 2500 g/minute.
ML and biotinylated ML (Bt-ML) were prepared from lauric acid-stabilized SPIONs (0.5 mL; Fe concentration 22 mg/mL), which were dialyzed (molecular weight cut-off: 10,000) at 37°C for 3 days in the presence of phospholipid vesicles (10 mL containing 0.15 mmol phospholipid) with regular changes of the buffer (5 microM TES, PH 7.0) by a self-assembly procedure to form lipid-coated SPIONs.
Biotinylation of monoclonal antibodies (MoAb)
Anti-αvβ3 monoclonal antibodies (Biolegend, San Diego, CA, USA) were biotinylated with Sulfo-NHS-LC-Biotin following the manufacturer’s (Pierce, Rockford, IL, USA) protocol. After purification by ultrafiltration with an Amicon Ultra-15 Centrifugal Filter Unit with a 10 kDa membrane from EMD Millipore (Billerica, MA, USA), the final biotin/antibody ratio was ≈4 as determined by the HABA method (Pierce).
Characterization
The size and morphology of the synthesized NPs were observed using a transmission electron microscope ([TEM] H-7650; HITACHI, Tokyo, Japan) operating at 200 kV. ML and Bt-ML were stained with 1% phosphotungstic acid for negative staining to distinguish their shell from the core, but SPIONs were not. The mean size distribution of the particles was determined using a Malvern Zeta-sizer 3000HS (Malvern Instruments, Malvern, UK) operating at 632.0 nm. X-ray diffraction (XRD) was performed using a XRD system (D-MAX2200 VPC; Rigaku Corporation, Tokyo, Japan) equipped with Cu/Ka radiation at a scanning rate of 4°/minute in a 2 theta range of 10°~70° at 40 kV and 30 mA. The loading content of iron in the particles was determined using an atomic absorption spectrophotometer (Z-5000; HITACHI).
Magnetic property measurements
The NPs were prepared in 0.01, 0.02, 0.03, 0.04, and 0.05 microM of Fe concentrations. The T2 of these solutions were determined on a 3.0T MR system (Signa Excite; General Electric, Milwaukee, WI, USA). A T2 mapping sequence (TR: 3000 ms, TE: 20, 40, 60, and 80 ms, matrix 512 × 160, field of view 200 mm) was used to measure transverse relaxation time. Images of the various solutions were analyzed by defining regions of interest (ROI) in each test tube. Relaxivity (r2) was calculated through the curve-fitting of T2 (s−1) vs the Fe concentration (microM).
A vibration sample magnetometer (MPMS XL-7 magnetometer; Quantum Design, Inc, San Diego, CA, USA) was used to characterize the magnetic properties of the particles. The hysteresis of the magnetization was recorded at 300 K obtained under a circulate magnetic field ranged between +1 and −1 Tesla.
Cell culture
All the cell lines were gifts from the Research Center of Clinical Medicine in Nanfang Hospital (Guangzhou, China). Both breast cancer cell lines of MDA-MB- 435S (αvβ3-integrin positive) and MCF-7(αvβ3 negative) cells were grown in Dulbecco’s modified Eagle’s medium, and RAW264.7 in RPMI-1640 supplemented with 10% fetal bovine serum, penicillin (100 U/mL), and streptomycin (100 U/mL) in a humidified incubator at 37°C with 5% CO2 in the air.
Cytotoxicity assay
In vitro cytotoxicity of the NPs was assessed using the MTT assay of MDA-MB-435S and MCF-7. Briefly, the cells were seeded in a 96-well plate at the density of 4 × 104 cells/well for 48 hours, then the culture medium was replaced with a 200 μL medium containing ML or Bt-ML (at 200, 400, 800, 1200, 1600, and 2000 μM Fe) and kept incubated for 20 hours. Subsequently, 50 μL of MTT solution (5 mg/mL) was added to the wells and incubated for 4 hours in a humidified atmosphere prior to the addition of 150 μL of dimethyl sulfoxide into each well to dissolve the formazan crystals, and gently shaken for 10 minutes so that complete dissolution was achieved. The reaction mixture on each well of the 96-well culture plate was measured using the enzyme-linked immunosorbent assay reader BIOTEK ELX800 at a wavelength of 570 nm. The control group contained cells and cell culture medium without NPs.
Visualization of the cellular uptake by Prussian blue staining
RAW264.7 cells were seeded onto the 6-well plate with 5 × 105 cells/well and incubated for 24 hours. Then the supernatant was removed and 2 mL of the full RPMI-1640 culture medium containing SPIONs, ML, and Bt-ML (Fe concentration of 50 μg/mL) was added to the RAW264.7 cells, respectively. After incubation at 37°C for 2 hours, the supernatant was removed and cells were washed three times with phosphate-buffered saline. Then, the cells were fixed by 2 mL of 4% paraformaldehyde solution for 20 minutes. After fixation, the cells were stained with a filtrated and fresh prepared potassium ferrocyanate solution (mixture of equal volume of 4% potassium ferrocyanate with 4% hydrochloric acid) for 30 minutes at 37°C causing a deep blue complex, rewashed, and then counterstained with nuclear fast red for 5 minutes. The wells without NPs were used as blank.
In vivo MR imaging
All procedures were approved by the animal center of Southern Medical University (Guangzhou, China). Tumor xenografts with 1 × 107 MDA-MB-435S cells were implanted into the right caudal mammary fat pad of female mice (NOD/SCID; Huafukang Company, Beijing, China). Six to 8 weeks after implantation when the tumor reached 0.8–1.2 cm in diameter, the animals were randomized into two groups: targeted Bt-ML and nontargeted ML group for MR scan (n = 5 per group).
The mice were subjected to injections via the tail vein with 150 μg biotinylated anti-αvβ3 MoAb over 2 minutes (first step). After 36 hours, 1 mg of cold avidin (Santa Cruz Biotechnology, Santa Cruz, CA, USA) was injected over 2 minutes, followed by an additional 0.5 mg of cold streptavidin 10 minutes later (second step). Two hours later, ML or Bt-ML was injected via the tail vein at a dose of 80 μmol Fe/kg (third step). Mice were anesthetized with an intraperitoneal injection of pentobarbital sodium (60 mg/kg) 10 minutes before the MR studies. MR imaging was performed before and 2 hours post-injection with a 3.0T MR system (Signa Excite) equipped with a mouse-imaging coil. Coronal T2-weighted SE (TR/TE = 4000/85 ms, FOV = 12 cm, matrix = 320 × 224, NEX = 4, thickness/interval = 2.5/0.2 mm).
The pixel intensities from the T2-weighted images were analyzed with MATLAB software (The Math-Works, Natick, MA, USA).Citation17,Citation18 In each baseline and 2 hours post-injection T2-weighted image slice, a ROI was manually placed around the tumor edge on each baseline slice, and the standard deviation (SD) of the average tumor signal at baseline was calculated. Subsequently, serial images were spatially coregistered using a cross-correlation routine, and the tumor ROI mask was copied to each time point. At 2 hours post-injection, pixels with an MR signal intensity decrease ≤3 SD below the baseline tumor signal were considered significant. The enhanced fraction within the whole tumor for each individual mouse was determined by the number of enhanced pixels divided by the number of total pixels within the tumor.
Fluorescence immunohistochemistry and Prussian blue staining
After MR imaging, animals were sacrificed following anesthesia and cervical dislocation. Tumors were resected, and quickly frozen in an optimal cutting temperature compound for immunohistochemistry. Frozen tumor tissue slices (5 μm thick) were fixed in acetone for 10 minutes, and then dried in the air for 30 minutes. Nonspecific binding of antibodies was blocked by incubation with donkey serum for 30 minutes at room temperature. The sections were incubated overnight with rat anti-mouse CD31 monoclonal antibody (BD Pharmingen, San Jose, CA, USA), then washed and incubated with fluorescein isothiocyanate (FITC) goat anti-rat secondary antibody (Jackson ImmunoResearch, West Grove, PA, USA). 4,6′-diamidino-2-phenylindole (Molecular Probes, Eugene, Oregon, USA) was used for cell nuclei staining.
Sections were also stained with Prussian blue according to standard clinical pathology protocols.
Statistical analysis
Statistical analysis was performed using a two-tailed unpaired Student’s t-test at the P < 0.05 level. All data were expressed in the form of mean ± SD. All tests were performed using SPSS version 13.0 (IBM Corporation, Armonk, NY, USA).
Results
Characterization of ML
TEM images () show NPs in a dry state. From the TEM images (), the diameter of spherical SPIONs was estimated to be 13.6 nm. After incorporation of SPIONs with liposomes, an about 4 nm thick amorphous edge around the SPIONs was observed. We also measured hydrodynamic diameters and distribution of ML using a dynamic light scattering (DLS) instrument. The particle sizes, hydrodynamic diameters, and polydispersity indices are summarized in . Pure SPIONs had a particle size of 86.9 nm with a polydispersity index of 0.146. The incorporation of SPIONs into the liposomes resulted in increased particle size (120~129 nm) due to the formation of coating layers of liposomes. As shown in , the particle size determined by TEM was smaller compared with the particle size of the same particles obtained from DLS measurements. These results confirm the presence of a liposome hairy layer on the particle surface, which increases the value of the average hydrodynamic diameter during the DLS measurements and which is collapsed onto the particle surface during the drying of the TEM sample. It should also be remembered that while TEM provides the number-average particle size, DLS gives the zeta-average, which is sensitive to large-size particles.
Table 1 Physical characteristics of nanoparticles
Figure 2 Transmission electron microscopy images of (A) SPIONs, (B) ML, and (C) Bt-ML.
Abbreviations: SPIONs, superparamagnetic iron oxide nanoparticles; ML, magnetoliposomes; Bt-ML, biotinylated magnetoliposomes.
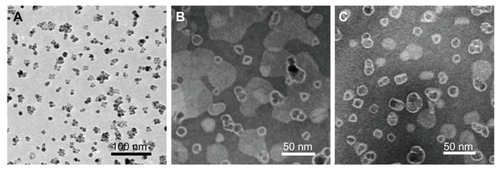
XRD patterns of the synthesized particles were analyzed to determine the crystal phase of the SPIONs and ML (). All of the particles (SPIONs, ML, and Bt-ML) showed diffraction peaks at 30.1°, 35.5°, 43.1°, 53.4°, 57.0°, and 62.6°, due to face-centered cubic lattice structures 220, 311, 400, 422, 440, and 511 which are characteristic peaks of the Fe3O4 crystal structure. Additionally, there are no peaks at 31° corresponding to γ-Fe2O3 and α-Fe2O3 for 210 and 213 in XRD patterns, supporting the purity of synthesized iron oxide nanoparticles. The results indicated that liposomal encapsulation of the magnetic cores did not make significant changes in the crystal phase of Fe3O4.
Magnetic property measurements
The T2 relaxivity of the NPs at 3.0T MR was examined (). The results showed that the MR imaging signal intensity of the NPs decreased with the increase of Fe concentration. The NPs were well-fitted by a line within the analyzed range of iron concentration (0.01–0.05 microM), thus exhibiting the typical property of SPIONs in shortening T2 relaxation time. The specific relaxivity values of SPIONs, ML, and Bt-ML were found to be 0.722 × 103, 0.611 × 103, and 0.675 × 103 microM−1 s−1, respectively.
Figure 4 Magnetic property measurements of nanoparticles: (A) T2-weighted MR images and (B) magnetic behavior of SPIONs, ML, and Bt-ML.
Abbreviations: MR, magnetic resonance; SPIONs, superparamagnetic iron oxide nanoparticles; ML, magnetoliposomes; Bt-ML, biotinylated magnetoliposomes.
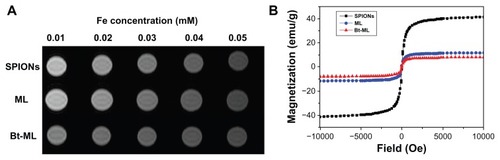
The magnetic property of SPIONs, ML, and Bt-ML was evaluated by vibration sample magnetometer to confirm the feasibility and sensitivity as MR imaging nanoprobes (). Typical hysteresis curves regarding superparamagnetic behaviors were observed. The magnetization decreased from plateau value to zero when magnetic field intensity decreased, which implied the NPs would respond well to magnetic fields without any permanent magnetization. The specific saturation magnetism σs at 1T for ML and Bt-ML were 11.7 emu/g and 8.05 emu/g, which were smaller than the value for the SPIONs (41.2 emu/g). The decrease of the magnetization of such coated particles might be due to the coating of nonmagnetic liposomes on the magnetic nanoparticles and/or the decrease in their iron oxide content.
Cytotoxicity assay
In order to investigate the cytotoxicity of the novel nanoparticles carrier, both MDA-MB-435S and MCF-7 were incubated with ML and Bt-ML, and the cytotoxicity was assessed using MTT assay. Cell viability was apparently unaltered in the entire test dosage range from 200 to 2000 μM Fe as depicted in . This result indicated that ML and Bt-ML are biocompatible and low-toxic at the given Fe concentration range (200–2000 μM).
Figure 5 In vitro cell viability of (A) MDA-MB-435S and (B) MCF-7 cells incubated with ML and Bt-ML with different iron concentrations determined by MTT assay.
Abbreviations: MDA-MB-435S, human breast cancer cells; MCF-7, hormone dependent breast cancer cells; ML, magnetoliposomes; Bt-ML, biotinylated magnetoliposomes; MTT, methyl thiazdyl tetrazolium.
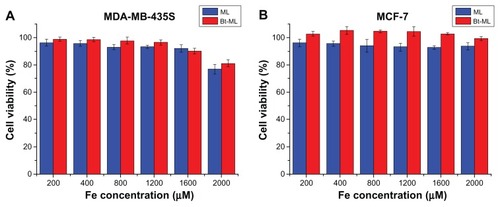
NP uptake by macrophages
In order to evaluate the capacity of ML to evade capture by macrophage cells in vitro, the cellular uptake of NPs in RAW264.7 cells was visualized by Prussian blue staining (). Compared with the control group without contrast agents (), RAW264.7 cells incubated with SPIONs were stained in intensive blue color under 50 μg/mL of the Fe concentration in culture medium. Blue areas or spots could be seen in almost every cell (). In comparison, the RAW264.7 cells incubated with ML or Bt-ML showed a little weak blue color appearance (). The result indicated that SPION entrapment into liposomes and modification by PEG could dramatically decrease the internalization of the NPs by macrophage cells, which allows more contrast agents access to the target vasculature.
Figure 6 Prussian blue staining micrographs of macrophage cells (RAW264.7). (A) Control without contrast agent, and cells treated with (B) SPIONs, (C) ML, and (D) Bt-ML.
Note: Blue stain density reflects the level of SPIONs accumulation within cells.
Abbreviations: SPIONs, superparamagnetic iron oxide nanoparticles; ML, magnetoliposomes; Bt-ML, biotinylated magnetoliposomes.
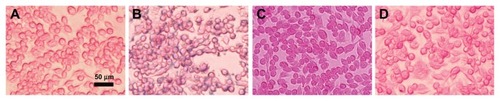
In vivo MR imaging
The in vivo MR imaging was performed before injection and 2 hours post-injection (). The pre-contrast images also contained some enhanced pixels, as their signal intensities were below the threshold. For mice receiving Bt-ML, the spatial distribution of enhanced pixels was heterogeneous and mainly in the periphery of the tumor. For mice receiving ML, pixel signal enhancement was much less pronounced and scattered within the tumor rim. The enhanced fraction in tumors was 7.0% ± 1.8% (P < 0.05) at 2 hours post-injection of targeted Bt-ML, while only a 2.0% ± 1.1% decrease was observed in the nontargeted group (). Additionally, we found high signal reductions in the liver at 2 hours post-injection of Bt-ML and ML, respectively.
Figure 7 MR detection of the tumors via the anti-αvβ3 antibody guided three-step pretargeting approach. (A) T2-weighted MR images of tumor-bearing mice before and 2 hours after injection of targeted Bt-ML and nontargeted ML, respectively. Enhanced pixels within the tumors were color coded in red. (B) Enhanced fraction of the tumor region before and after 2 hours after injection of contrast agents. (C) Schematic representation of the antibody-guided three-step pretargeting approach.
Abbreviations: MR, magnetic resonance; Bt-ML, biotinylated magnetoliposomes; ML, magnetoliposomes.
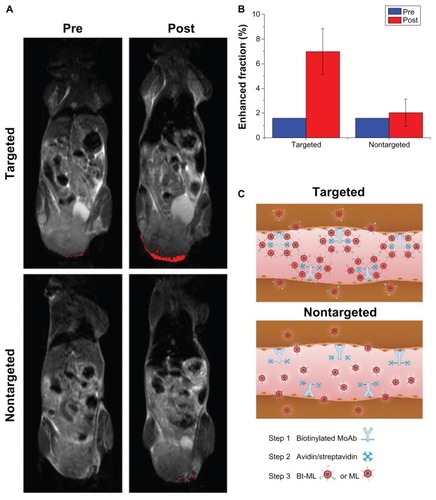
Fluorescence immunohistochemistry and Prussian blue staining
Ex vivo fluorescence microscopy was used to investigate the binding location of NPs inside tumor tissues. Rhodamine fluorescence from αvβ3-targeted Bt-ML was colocalized with the angiogenic endothelial cells within tumor tissues, and mainly distributed to the periphery of the tumor (). Little fluorescence was also observed outside the vessel wall or lumen. In contrast, fluorescence from ML was only found in the interstitial space of the tumor but not colocalized with endothelial cells ().
Figure 8 Fluorescence microscopy images of tumor tissues. (A–C) Bt-ML colocalizes with the angiogenic vasculature from the tumor periphery; (D–F) ML is seen beyond the vasculature.
Notes: Liposomal fluorescence from rhodamine (red) is shown in the left panel. Endothelium stained using an antimouse CD31 antibody (green) is shown in the middle panel. Color overlays with nuclei counterstained DAPI (blue) are shown in the right panel.
Abbreviations: DAPI, 4,6′-diamidino-2-phenylindole; Bt-ML, biotinylated magnetoliposomes.
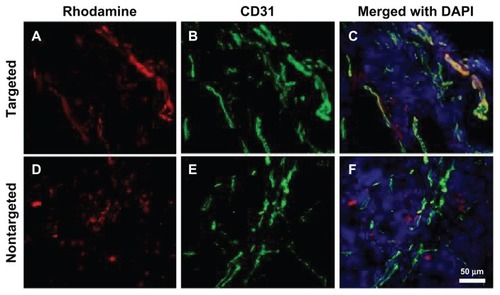
To further verify the accumulation of the SPION encapsulated NPs in the tumor tissue, Prussian blue staining was carried out as shown in . In good agreement with fluorescence immunohistochemistry, blue spots of Bt-ML showed vascular localization. In the nontargeted group, only a little ML was observed with an unspecific distribution. The neovascular spatial distribution seen microscopically, was consistent with the contrast enhancement patterns of angiogenesis observed in MR imaging.
Discussion
In the present study, we demonstrated that the antibody-guided three-step pretargeting approach was highly effective for molecular imaging of angiogenesis in vivo. SPION-based ML are sensitive and biocompatible contrast agents for detection of αvβ3-integrin overexpressing on angiogenic endothelium. MR signal enhancement revealed the peripheral spatial distribution of neovasculature, which was highly correlated to the histologic characteristics of tumors.
To enable targeted imaging of tumor angiogenesis, highly efficient NPs coated with biocompatible polymers are desirable.Citation19 ML, with a high payload of NPs and biocompatibility, have been studied as bone marrow MR contrast agents.Citation20 In the present study, we prepared SPION-encapsulated ML with superparamagnetism and high molar relaxivity. Our results demonstrated that even at the Fe concentration of 2000 μM, the cell viability evaluated by the MTT assay still remained above 75%, which is important for further biological applications. Besides, after liposomal coating, the capture of NPs by mouse macrophage cells was significantly reduced. Incorporation of PEG into lipid bilayers not only protects against possible unwanted interactions with plasma protein or neighboring cells,Citation21 but also can be functionalized with a distal moiety to target biomarkers,Citation22 consequently allowing more ML to accumulate at the targeted sites.
To specifically target the desired biomarkers of tumors, contrast agents can potentially be conjugated with many varieties of ligands including antibodies,Citation23–Citation25 peptides,Citation26 and polysaccharides.Citation27 For example, antibody-conjugated paramagnetic liposomes have been used to target αvβ3-integrin for MR imaging-based visualization of angiogenesis.Citation23 However, antibodies will considerably increase the already large size of contrast agents, resulting in unfavorable biodistribution.Citation25
Compared to a directly labeled MoAb approach, an antibody-guided pretargeting approach, through a stable bridge of biotin–avidin, holds promise in improving the tumor/nontumor ratio.Citation28 Several pretargeting protocols using different multistep avidin–biotin methods have been proposed.Citation14,Citation29,Citation30 A method including MoAb-biotin/avidin/biotin described by Paganelli et alCitation14 obtained high selective tumor uptake of radionuclide with less systemic toxicity than covalent conjugates of MoAb. The three steps of antibody-guided pretargeting are as follows: (1) the localization of biotinylated monoclonal antibodies on targeted cells; (2) avidination of the pretargeted biotinylated antibodies and clearance of circulating biotinylated antibodies by an excess of cold avidin/SA; (3) postlabeling of the target by biotinylated contrast agents.Citation29 To exploit the targeted receptor-ligand mechanism for delivering SPION-based ML to the tumor region, the antibody-guided three-step pretargeting approach was used in our study (). Through the linkage of pretargeting biotinylated MoAb and avidin/SA, Bt-ML rapidly bound to target αvβ3-integrin with high specificity, resulting in a heterogeneous and more pronounced signal decrease in the rim of tumors compared to the nontargeted group. The signal was amplified for the reason that more than one molecule of avidin/SA can bind to a single biotinylated MoAb molecule localized on the tumor and that more than one biotinylated effector can bind to an avidin/SA molecule. Zhu et alCitation30 utilized PAMAM dendrimer-based contrast agents to target Her-2/neu receptors by a three-step pretargeting approach for MR imaging, but produced limited selective enhancement. This may be due to the binding sites of avidin being saturated by biotinylated monoclonal antibodies. In our study, the intratumoral distribution of αvβ3-integrin was sparse and much excessive avidin/SA was administered, so the possibility of saturation of avidin/SA by biotinylated MoAb was much less. The pronounced MR enhancement in the targeted group further demonstrated the success of our strategy. Additionally, in contrast with our in vitro experiments, strong signal reduction in the liver was observed for ML and Bt-ML at 2 hours post-injection, which indicates that a portion of contrast agents were taken up by RES. Various conditions (such as water dilution or plasma buffering) in vivo may affect properties of ML and the situation will be certainly different.Citation31
For validation of the MR imaging findings and to establish the exact site and mechanism of accumulation of the NPs, fluorescence microscopy and Prussian blue staining of the excised tumor were performed. Histologic results further demonstrated that through the pretargeting approach, Bt-ML targeted αvβ3-integrin colocalized with angiogenic endothelium of tumors in vivo, in agreement with previous reports.Citation32 The selective targeting of Bt-ML to vascular structures is responsible for the MR signal decrease. Besides, a diffuse pattern of fluorescence was also found in the interstitial space within the tumor for both tested NPs, which is most likely due to the enhanced permeability of the neovasculature and retention effect of NPs. These results agree with previous findings that RGD-functionalized bimodal probes enable image angiogenesis by characterization of αvβ3-integrin with a combination of MR imaging and fluorescence microscopy.Citation33
It should be noted that long-circulating NPs allow for prolonged time for extravasation from neovasculature with increased permeability.Citation34 Because peak concentration of NPs in tumors is 1–3 hours post-injection,Citation29 we performed MR imaging in vivo at 2 hours post-injection to improve the specificity of the tumor imaging. In our study, unspecific contrast enhancement was also observed in nontargeted tumors, but much less pronounced than the targeted group. Compared to specific localization, the nonspecific extravasation was significantly less extensive in fluorescent microscopy.
Pretargeting is a useful tool to improve sensitivity and specificity of molecular imaging. This approach may facilitate assessment of angiogenic activity and noninvasive evaluation of chemotherapeutic response. In addition, liposomes may be also used as delivery vehicles to encapsulate therapeutic drugs to avoid severe damage to healthy tissues, which makes target-specific theranostics possible.
Conclusion
In conclusion, we have prepared SPION-based ML with superparamagnetism, biocompatibility, and low cytotoxicity. Our results demonstrated that with an antibody-guided three-step pretargeting approach, ML were sensitive enough to allow for MR imaging detection of tumor angiogenesis. Additionally, the MR enhancement revealed the predominantly peripheral distribution of angiogenesis, which was highly consistent with the histologic distribution of αvβ3-targeted NPs. Thus, our strategy with liposomal carriers may provide a versatile platform for assessment of angiogenic activity at the molecular level and can be used as a target-specific anticancer drug delivery system.
Acknowledgments
We thank Yingxin Yu (Department of Immunology, Southern Medical University, China) for technical assistance in antibodies biotinylation. This research was supported in whole or in part by the National Natural Science Foundation of China (30870708, 81071146, and 81271642).
Disclosure
The authors report no conflicts of interest in this work.
References
- BergersGBenjaminLETumorigenesis and the angiogenic switchNat Rev Cancer20033640141012778130
- HoodJDChereshDARole of integrins in cell invasion and migrationNat Rev Cancer2002229110012635172
- Morales-AvilaEFerro-FloresGOcampo-GarcíaBEMultimeric system of 99 mTc-labeled gold nanoparticles conjugated to c[RGDfK(C)] for molecular imaging of tumor alpha(v)beta(3) expressionBioconjug Chem201122591392221513349
- XieHDiagaradjanePDeorukhkarAAIntegrin alphavbeta3-targeted gold nanoshells augment tumor vasculature-specific imaging and therapyInt J Nanomedicine2011625926921423588
- LanzardoSContiLBrioschiCA new optical imaging probe targeting alphaVbeta3 integrin in glioblastoma xenograftsContrast Media Mol Imaging20116644945822144022
- StreeterJEGessnerRCTsurutaJFeingoldSDaytonPAAssessment of molecular imaging of angiogenesis with three-dimensional ultrasonographyMol Imaging201110646046822201537
- BulteJWKraitchmanDLIron oxide MR contrast agents for molecular and cellular imagingNMR Biomed200417748449915526347
- Wahajuddin AroraSSuperparamagnetic iron oxide nanoparticles: magnetic nanoplatforms as drug carriersInt J Nanomedicine201273445347122848170
- YuMKJeongYYParkJDrug-loaded superparamagnetic iron oxide nanoparticles for combined cancer imaging and therapy in vivoAngew Chem Int Ed Engl200847295362536518551493
- GuptaAKGuptaMSynthesis and surface engineering of iron oxide nanoparticles for biomedical applicationsBiomaterials200526183995402115626447
- TorchilinVPRecent advances with liposomes as pharmaceutical carriersNat Rev Drug Discov20054214516015688077
- MulderWJStrijkersGJvan TilborgGAGriffioenAWNicolayKLipid-based nanoparticles for contrast-enhanced MRI and molecular imagingNMR Biomed200619114216416450332
- PaganelliGMagnaniPZitoFPre-targeted immunodetection in glioma patients: tumour localization and single-photon emission tomography imaging of [99 mTc]PnAO-biotinEur J Nucl Med19942143143218005155
- PaganelliGMagnaniPZitoFThree-step monoclonal antibody tumor targeting in carcinoembryonic antigen-positive patientsCancer Res19915121596059661933860
- DiamandisEPChristopoulosTKThe biotin-(strept)avidin system: principles and applications in biotechnologyClin Chem19913756256362032315
- De CuyperMJoniauMMagnetoliposomes. Formation and structural characterizationEur Biophys J19881553113193366097
- BolesKSSchmiederAHKochAWMR angiogenesis imaging with Robo4- vs alphaVbeta3-targeted nanoparticles in a B16/F10 mouse melanoma modelFASEB J201024114262427020585027
- van TilborgGAMulderWJvan der SchaftDWImproved magnetic resonance molecular imaging of tumor angiogenesis by avidin-induced clearance of nonbound bimodal liposomesNeoplasia200810121459146919048124
- LiWSuBMengSRGD-targeted paramagnetic liposomes for early detection of tumor: in vitro and in vivo studiesEur J Radiol201180259860621316892
- BulteJWde CuyperMDespresDFrankJAShort- vs long-circulating magnetoliposomes as bone marrow-seeking MR contrast agentsJ Magn Reson Imaging19999232933510077033
- EfremovaNVBondurantBO’BrienDFLeckbandDEMeasurements of interbilayer forces and protein adsorption on uncharged lipid bilayers displaying poly(ethylene glycol) chainsBiochemistry200039123441345110727239
- DingNLuYLeeRJFolate receptor-targeted fluorescent paramagnetic bimodal liposomes for tumor imagingInt J Nanomedicine201162513252022072885
- SipkinsDAChereshDAKazemiMRNevinLMBednarskiMDLiKCDetection of tumor angiogenesis in vivo by alphaVbeta3-targeted magnetic resonance imagingNat Med1998456236269585240
- HsiehWJLiangCJChiehJJIn vivo tumor targeting and imaging with anti-vascular endothelial growth factor antibody-conjugated dextran-coated iron oxide nanoparticlesInt J Nanomedicine201272833284222745546
- QiaoJLiSWeiLHER2 targeted molecular MR imaging using a de novo designed protein contrast agentPLoS One201163e1810321455310
- SchmiederAHCaruthersSDZhangHThree-dimensional MR mapping of angiogenesis with alpha5beta1(alpha nu beta3)-targeted theranostic nanoparticles in the MDA-MB-435 xenograft mouse modelFASEB J200822124179418918697838
- EspositoGGeninatti CrichSAimeSEfficient cellular labeling by CD44 receptor-mediated uptake of cationic liposomes functionalized with hyaluronic acid and loaded with MRI contrast agentsChem Med Chem20083121858186218988207
- SharkeyRMKaracayHCardilloTMImproving the delivery of radionuclides for imaging and therapy of cancer using pretargeting methodsClin Cancer Res20051119 Pt 27109s7121s16203810
- GoodwinDAMearesCFPretargeting: general principles; October 10–12, 1996Cancer199780Suppl 12267526809406724
- ZhuWOkollieBBhujwallaZMArtemovDPAMAM dendrimer-based contrast agents for MR imaging of Her-2/neu receptors by a three-step pretargeting approachMagn Reson Med200859467968518302223
- FrascioneDDiwokyCAlmerGUltrasmall superparamagnetic iron oxide (USPIO)-based liposomes as magnetic resonance imaging probesInt J Nanomedicine201272349235922661890
- ChenWJarzynaPAvan TilborgGARGD peptide functionalized and reconstituted high-density lipoprotein nanoparticles as a versatile and multimodal tumor targeting molecular imaging probeFASEB J20102461689169920075195
- MulderWJStrijkersGJHabetsJWMR molecular imaging and fluorescence microscopy for identification of activated tumor endothelium using a bimodal lipidic nanoparticleFASEB J200519142008201016204353
- KluzaEJacobsIHectorsSJDual-targeting of alphavbeta3 and galectin-1 improves the specificity of paramagnetic/fluorescent liposomes to tumor endothelium in vivoJ Control Release2012158220721422079810