Abstract
Background
The purpose of this study was to investigate the influences of nanoscale wear particles derived from titanium/titanium alloy-based implants on integration of bone. Here we report the potential impact of titanium oxide (TiO2) nanoparticles on adhesion, migration, proliferation, and differentiation of mesenchymal stem cells (MSC) from the cellular level to the molecular level in the Wistar rat.
Methods
A series of TiO2 nanoparticles (14 nm, 108 nm, and 196 nm) were synthesized and characterized by scanning electron microscopy and transmission electron microscopy, respectively.
Results
The TiO2 nanoparticles had negative effects on cell viability, proliferation, and the cell cycle of MSC in a dose-dependent and size-dependent manner. Confocal laser scanning microscopy was used to investigate the effects of particle internalization on adhesion, spreading, and morphology of MSC. The integrity of the cell membrane, cytoskeleton, and vinculin of MSC were negatively influenced by large TiO2 nanoparticles.
Conclusion
The Transwell migration assay and a wound healing model suggested that TiO2 nanoparticles had a strong adverse impact on cell migration as particle size increased (P < 0.01). Furthermore, alkaline phosphatase, gene expression of osteocalcin (OC) and osteopontin (OPN), and mineralization measurements indicate that the size of the TiO2 nanoparticles negatively affected osteogenic differentiation of MSC.
Introduction
Titanium and its alloys are widely used as bone implants in the clinical setting, mainly because of their good biocompatibility and mechanical properties.Citation1,Citation2 Nevertheless, owing to their inherent surface inertness, titanium-based implants can only integrate with the surrounding bone tissue via mechanical interlocking, and the ability to do this is closely related to surface topography at the microscale or nanoscale.Citation3,Citation4 Titanium substrates with a nanorough surface have been shown to influence cell adhesion, proliferation, and differentiation of osteoblasts.Citation5 Guida et al confirmed that microscale or nanoscale modification of titanium substrates enhanced proliferation and synthesis of the extracellular matrix in human bone marrow-derived mesenchymal stem cells (MSC) and expression of osteoprotegerin.Citation6 Annunziata et al also demonstrated that nanostructured titanium surfaces enhance adhesion and differentiation of human bone marrow-derived MSC.Citation7 Thus, fabrication of titanium substrates with desirable topography has been a focus in the development of high quality titanium implants in recent decades. With the rapid development of nanotechnology, surface engineering of titanium using nanotopography to regulate cell behavior is now attracting much research attention.Citation8,Citation9 In our previous research, we confirmed that hierarchically microstructured or nanostructured surfacesCitation10 and nanostructured titanium substratesCitation11 could efficiently regulate the proliferation and differentiation of MSC.
However, in long-term clinical application (eg, titanium-based hip joint implants), microscale and/or nanoscale wear particles can be generated at the implant/bone interface due to corrosion, aseptic loosening, and prosthesis friction.Citation12,Citation13 On the other hand, composite bone prostheses containing titanium nanoparticles may be released directly at the implant/bone interface.Citation14 Previous studies have confirmed that microscale wear debris in the form of titanium particles released from a titanium implant occur in vivo, resulting in adverse effects, ie, significant attenuation of osteoblast function, increased osteoclast recruitment, and decreased bone integration.Citation15,Citation16
Given the worldwide concerns about nanotoxicity in biomaterials, the potential cytotoxicity of titanium nanoparticles has also been investigated in different cell lines. Previous studies have demonstrated that titanium nanoparticles have negative effects on cells, including inflammatory reactions in endothelial cells,Citation17 damage to the DNA of human lymphoblastoid cells,Citation18 and induction of micronuclei and apoptosis in Syrian hamster embryo fibroblasts.Citation19 Other reports have shown that titanium dioxide (TiO2) nanoparticles delivered to the abdominal cavity and liver in mice cause brain injury and liver damage, respectively.Citation20,Citation21 Wang et al also confirmed that TiO2 nanoparticles are potentially toxic to major organs and cause damage to the knee joints in rabbits due to their ability to catalyze oxidative DNA damage.Citation22
To be able to confirm further the feasibility of development of surface nanostructured titanium-based bone implants, there is an urgent need for increased knowledge about how titanium nanoparticles affect biological function in cells involved in bone formation. In a previous study, we established a correlation between the cytotoxicity of TiO2 nanoparticles and different particle sizes on a sub-200 nm scale in respect to osteoblasts.Citation23 In this study, we investigated the interaction between TiO2 nanoparticles and MSC. Although a previous study investigated the interaction between nanoparticles and neural stem cells,Citation24 the effects of titanium nanoparticles on the biological behavior of bone marrow-derived MSC have not yet been reported. The rationale for choosing bone marrow-derived MSC was that the head of a titanium-based hip joint must be inserted into the medullary cavity of a long bone for fixation in clinical application, and the medullary cavity contains plentiful MSC. At this site, titanium implants can interact with MSC directly. In our previous study,Citation23 we investigated the influence of different-sized titanium nanoparticles on the biological behavior of osteoblasts.
The objective of this study was to use titanium nanoparticles of different sizes mimicking the wear debris potentially loosening from a joint implant and to investigate how titanium nanoparticles influence the biological behavior of MSC, including cell adhesion, migration, proliferation, and differentiation.
Materials and methods
Materials
Reagents used in this study, including fluorescein isothiocyanate (FITC), alkaline phosphatase (ALP), a bicinchoninic acid assay kit, Hoechst 33258, dexamethasone, L-ascorbate-2-phosphate, 1,25-dihydroxy vitamin D3, b-glycerol phosphate, and alizarin red-S, were purchased from Sigma-Aldrich Chemical Co (St Louis, MO, USA). Rhodamine-phalloidin was purchased from Invitrogen Co (Carlsbad, CA, USA). A CCK-8 assay kit was provided by Dojindo Laboratories (Kumamoto, Japan). Vinculin antibody was purchased from Santa Cruz Biotechnology Co (Santa Cruz, CA, USA). DyLight 649 goat anti-mouse secondary antibody was supplied by ZSGB-Bio Co (Beijing, People’s Republic of China). Other reagents were sourced from Oriental Chemical Co (Chongqing, People’s Republic of China).
Synthesis and characterization of TiO2 nanoparticles
A series of TiO2 nanoparticles was synthesized with a hydrothermal method.Citation23 The morphological features of the TiO2 nanoparticles were characterized by field emission scanning electron microscopy (FEI Nova 400 Nano SEM, Philips, Eindhoven, The Netherlands) and transmission electron microscopy (TECNAI-10, Phillips), respectively.
Cell culture
MSC were isolated from the bone marrow of the femur and tibia of a Wistar rat (weight 150–200 g) using a technique reported previously.Citation25 The MSC were cultured in a 75 cm2 flask containing 5 mL of Dulbecco’s Modified Eagle’s Medium supplemented with 10% bovine serum (Gibco, Grand Island, NY, USA) at 37°C in a 5% CO2 atmosphere. The cell culture medium was changed on the first day and thereafter every 2 days. After reaching confluence, the cells were detached with 0.25% trypsin in 1 mM tetrasodium EDTA, centrifuged, and resuspended in complete medium for reseeding in new culture flasks. The complete medium contained osteogenic supplements, ie, L-ascorbate-2-phosphate 50 mg/mL, dexamethasone 0.1 mM, b-glycerol phosphate 10 mM, and 1,25-dihydroxy vitamin D3 10 nM. Cells at the third passage were used for our experiments.
Cell viability assay
The viability of MSC was examined using the CCK-8 assay kit. Briefly, the cells were cultured in a 24-well plate with or without (control) TiO2 particles at an initial seeding density of 2 × 104 cells/cm2. For investigation of dose-dependent effects, MSC were incubated with different concentrations of TiO2 nanoparticles (0.05, 0.10, and 0.20 mg/mL) for 48 hours. For investigation of time-dependent effects, MSC were incubated with TiO2 0.10 mg/mL nanoparticles for 3, 7, and 14 days. CCK-8 kit reagents were added to each well according to the manufacturer’s instructions. Absorbance was measured using a microplate reader (model 550, Bio-Rad Laboratories, Hercules, CA, USA) at a wavelength of 450 nm (n = 6).
Cell cycle assay using flow cytometry
The cells were cultured in a 24-well plate at an initial cell seeding density of 1 × 105 cells/cm2. TiO2 nanoparticles were added into each well when cell confluence reached around 70%, and the final concentration of nanoparticles was adjusted to 0.10 mg/mL. After culture for one, two, and three days, the cells were washed twice with phosphate-buffered solution and centrifuged. The cells were then fixed with 70% ethanol at 4°C for one hour and washed with phosphate-buffered solution. Next, the treated cells were resuspended with propidium iodide solution 0.05 mg/mL containing RNase and incubated in the dark at room temperature for 30 minutes. The DNA content of the cells was then analyzed using a flow cytometer (EPICS XL-MCL, Beckman Coulter, Fullerton, CA, USA).
Particle internalization assay
FITC-TiO2 nanoparticles were constructed in a manner reported elsewhereCitation26 and their translocation was investigated. Briefly, 15 mg of TiO2 nanoparticles were suspended in 1 mL of distilled water and treated with ultrasonication at room temperature for 45 minutes. The sample was then centrifuged to remove the aqueous solution. Next, 0.4 mL of dopamine 1 mM solution was added with stirring for 10 minutes. After removal of the free dopamine by centrifugation, the resulting dopamine-modified TiO2 nanoparticles were further reacted with FITC to yield FITC-labeled TiO2 nanoparticles. Free FITC molecules were removed by centrifugation. FITC-labeled TiO2 nanoparticles were resuspended in phosphate-buffered solution for further experiments. Cells were cocultured with or without (control) FITC-TiO2 nanoparticles for 24 hours. Internalization of the TiO2 nanoparticles was investigated by confocal laser scanning microscopy (TCS SP5, Leica Microsystems, Wetzlar, Germany).
Cell adhesion
MSC were cultured with 0.10 mg/mL of FITC-TiO2 nanoparticles for 12 and 24 hours, respectively. The cells were washed with phosphate-buffered solution three times and fixed with 4% paraformaldehyde at 4°C for 30 minutes. The treated cells were then permeabilized with 0.25% Triton X-100 for 10 minutes and washed with phosphate-buffered solution three times. Next, the cells were incubated with 5% bovine serum albumin/phosphate-buffered solution at 37°C for one hour. Subsequently, mouse monoclonal antibody against vinculin (1:200) was added and incubated at 4°C overnight. Next, DyLight 649 goat anti-mouse secondary antibody (1:100) was added and incubated at 37°C for a further hour. Subsequently, the cells were stained with 5 U/mL of rhodamine-phalloidin at 4°C overnight and counterstained with 10 μg/mL of Hoechst 33258 at room temperature for 5 minutes. Finally, the cells were mounted with 95% glycerinum and visualized by confocal laser scanning microscopy.
Wound healing
Wound healing experiments were performed for quantitative investigation of the migration of MSC when stimulated by TiO2 nanoparticles of different sizes. MSC were incubated without (control) or with the different TiO2 nanoparticles (0.10 mg/mL) at 37°C for 4 hours. The initial cell seeding density was 1 × 105 cells/cm2. A linear wound was made by scratching with a cell scraper. The cells were washed twice with phosphate-buffered solution and incubated with fresh medium for another 12 and 16 hours, respectively. Cells were then visualized by confocal laser scanning microscopy.
Transwell assay
A further cell migration assay was performed as reported elsewhere.Citation27 MSC were cultured with TiO2 nanoparticles (0.10 mg/mL) for 8 hours. After detaching with trypsin, the treated cells were seeded into a Millicell (Greiner Bio-One, Frickenhausen, Germany) containing pores 8 μm in diameter at an initial seeding density of 1 × 105 cells/cm2 suspended in Dulbecco’s Modified Eagle’s Medium containing 1% fetal bovine serum. Next, the Millicell was put into the well of a 24-well plate containing 20% fetal bovine serum in Dulbecco’s Modified Eagle’s Medium. The cells were allowed to migrate at 37°C for another 8 hours. After staining with crystal violet (0.1 wt%), the migrated cell clones were recorded using an optical microscope. The cell number was counted at 12 different areas, and the data were averaged from three parallel experiments and normalized by data from the control group.
Total intracellular protein content
MSC were cultured without or with TiO2 nanoparticles (0.10 mg/mL) in Dulbecco’s Modified Eagle’s Medium with 10% fetal bovine serum for 7 and 14 days, respectively. The initial cell seeding density was 2 × 104 cells/cm2. At prescribed time intervals, the cells were lysed using 1% Triton X-100 with three freeze-thaw cycles. Total protein content in the cell lysates was determined spectrophotometrically using a commercially available kit (bicinchoninic acid Sigma-Aldrich). The light absorbance of the samples was measured at 570 nm with the Bio-Rad 550 spectrophotometric microplate reader. Total intracellular protein (expressed as mg) synthesized by the cells was determined from a standard curve of absorbance versus the known concentration of albumin run in parallel experiments.
Alkaline phosphatase activity
Cells were incubated with 0.10 mg/mL TiO2 particles for 7 and 14 days, respectively. The initial seeding density was 2 × 104 cells/cm2. Cells were then collected and lysed as mentioned above. The supernatant was used for determining ALP activity, with paranitrophenyl phosphate as a substrate. Absorbance at 405 nm was measured using a spectrophotometer in a 96-well microplate reader (Bio-Rad 550). ALP activity (expressed as nmol of p-nitrophenol converted per minute) was normalized by total intracellular protein synthesis and expressed as mol p-nitrophenol per minute per mg protein. The ALP activity of MSC cultured without TiO2 particles was used as the control.
Mineralization
Cell mineralization was quantitatively measured after alizarin red-S staining elution as described elsewhere.Citation28 Briefly, MSC were incubated with TiO2 particles (0.10 mg/mL) for 15 days at an initial seeding density of 5 × 104 cells/cm2. The cells were washed with phosphate-buffered solution and fixed with 2% glutaraldehyde at 4°C for 20 minutes. The samples were then washed three times in distilled water and stained with 40 Mm alizarin red-S (pH 4.1) with shaking at room temperature for 20 minutes. Next, 400 μL of 10% acetic acid was added to each well and incubated for another 30 minutes with shaking. The cell monolayer was then scraped with a cell scraper and transferred into a 1.5 mL tube with 10% acetic acid. Samples were heated up to 85°C for 10 minutes and centrifuged at 20,000 g for 15 minutes. Subsequently, 300 μL of the supernatant was transferred into a new tube and neutralized with 200 μL of 10% ammonium hydroxide. The absorbance of supernatant (100 μL) was measured at a wavelength of 405 nm using a spectrophotometric microplate reader (Bio-Rad 680). Cell mineralization was finally normalized for protein content.
Reverse transcription polymerase chain reaction
Cells were collected after culture for 14 days. The total RNA in the cells was extracted according to the instructions on an RNA extract kit (Bio-Tek Instruments Inc., Winooski, VT, USA). Aliquots of total RNA (5 mL) were reverse transcribed and a 5 mL cDNA sample was amplified. The following oligonucleotide primer pairs related to the targeted RNA were used in this study: β-actin, 5′-ATATCGCTGCGCTGGTCGTC-3′ and 5′-AGGATGGCGTGAGGGAGAGC-3′; osteocalcin, 5′-TGGCCCTGACTGCATTCTGC-3′ and 5′-GCTGTGCCGTCCATACTTTCG-3′; and osteopontin, 5′-CCTCTGAAGAAACGGAT GACT-3′ and 5′-CTGTGTGTTTCCACGCTT-3′. The polymerase chain reaction conditions were as follows: first cycle at 94°C for 5 minutes, followed by 35 cycles at 94°C for 30 seconds, 56°C for 30 seconds, and 72°C for 30 seconds, and the final cycle at 72°C for 10 minutes. The amplified cDNA was analyzed by gel electrophoresis with 1.5% agarose gel.
Statistical analysis
All data are expressed as the mean ± standard deviation. The statistical analysis was performed using the Student’s t-test and one-way analysis of variance at confidence levels of 95% and 99% (OriginPro, version 7.5).
Results
Synthesis and characterization of TiO2 nanoparticles
TiO2 nanoparticles of different sizes were home-synthesized by a hydrothermal methodCitation29 and characterized by scanning and transmission electron microscopy, respectively. Three types of TiO2 nanoparticles with average particle sizes of 14 nm, 108 nm, and 196 nm were synthesized by simply changing the ratios of peptizing agents, titanium precursor solution (titanium butoxide), and reaction time. Scanning and transmission electron microscopic images () show that the TiO2 nanoparticles had a relatively uniform morphology with a narrow size distribution.
Cell viability and proliferation assay
A CCK-8 kit was used to investigate the influence of TiO2 nanoparticles on the biological behavior of MSC, ie, their potential cytotoxicity. First, we investigated the dose-dependent effects of TiO2 nanoparticles on the viability of MSC. The results show that high concentrations of TiO2 nanoparticles (0.10 and 0.20 mg/mL) had strong adverse effects on the viability of MSC ().
Figure 2 Dose-dependent effects of various nanoparticles on cell viability after culture for 2 days. The initial cell seeding density was 2 × 104 cells/cm2.
Abbreviation: ABS, absorbance.
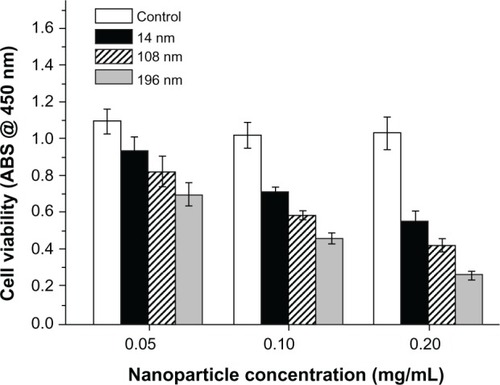
Second, we investigated the effects of TiO2 nanoparticle size on cell proliferation (). MSC were cultured with TiO2 nanoparticles (0.10 mg/mL) for 3, 7, and 14 days. The results show that the negative effect increased significantly with increasing particle size. After culture for 3 days with TiO2 nanoparticles 196 nm in size, relative cell viability decreased to 51.73% compared with the control group. Relative cell viability decreased further to 36.39% and 34.92% after culture for 7 and 14 days, respectively. Over all time intervals, the TiO2 nanoparticles showed a clear tendency for size-dependent inhibition of growth (P < 0.01).
Figure 3 Viability of mesenchymal stem cells incubated with various TiO2 nanoparticles for different time periods.
Notes: The initial cell seeding density was 2 × 104 cells/cm2. Error bars represent the mean ± standard deviation for n = 6, **P < 0.01.
Abbreviation: ABS, absorbance.
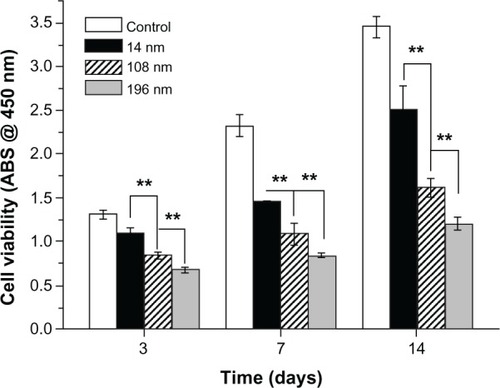
Third, we investigated the influence of different-sized TiO2 nanoparticles on cell division by flow cytometry to show the cell cycle during proliferation. Previous research has suggested that metal nanoparticles might potentially induce oxidative stress, leading to intracellular changes and alterations in the cell cycle.Citation30 In this study, we measured the change in percent proliferation index (percent synthesis phase of DNA [%S] + percent post-synthesis phase of DNA [%G2]) in the cell cycle to indicate the effects of the TiO2 nanoparticles on MSC.
The percentage of TiO2 nanoparticle-treated MSC in G1 phase increased when compared with that of the control after culture for one, two, and three days, respectively. Accordingly, the percent proliferation index (%S + %G2) of MSC treated with TiO2 nanoparticles decreased after culture for one, two, and three days (). Further, inhibition of proliferation of the TiO2 nanoparticles was also dependent on their particle size at each time interval. In particular, the percent proliferation index of MSC treated with 196 nm TiO2 nanoparticles decreased 2.51-fold compared with controls after culture for 3 days. This result indicates that the change in percent proliferation index for the MSC was mediated mainly by the size of the TiO2 nanoparticles. This finding was consistent with a previous study showing that polystyrene nanoparticle size and charge had a pronounced effect on mitotic spindle formation and chromosomes during cell division.Citation31 Moreover, other studies have demonstrated that DNA strand breaks were related to cell cycle arrest and cell death in epithelial cells treated with TiO2 nanoparticles and copper dioxide nanoparticles.Citation32,Citation33
Table 1 Cell cycle analysis. Cells were incubated without (control) or with various titanium dioxide nanoparticles for one, two, and three days, respectively
Particle internalization and cell adhesion
To identify the location of TiO2 nanoparticles within cells and the effect of particle size on adhesion of MSC, FITC-TiO2 particles (green), vinculin (focal adhesion protein, cyan), actin (cytoskeleton) filaments (red), and cell nuclei (blue) were visualized by confocal laser scanning microscopy. MSC cultured without (control) or with FITC-TiO2 nanoparticles of different sizes were observed after culture for 12 and 24 hours (), respectively. Large amounts of FITC-TiO2 nanoparticles (108 nm and 196 nm) were observed to accumulate in MSC (green). Moreover, the digested nanoparticles were only distributed in the cytoplasm rather than the nuclei of MSC. The same phenomenon was also observed in previous studies of neural stem cellsCitation24 and fibroblasts.Citation34
Figure 4 (A) Confocal microscopic images of mesenchymal stem cells incubated without (control) or with FITC-TiO2 particles (0.10 mg/mL) for 12 and 24 hours, respectively. FITC-TiO2 particles (green), vinculin (cyan), actin filaments (red), and cell nuclei (blue). Scale bar = 20 μm. (B) Relative fluorescence intensity analysis of vinculin. Average fluorescence intensity per cell was normalized to the control sample.
Notes: Error bars represent the mean ± standard deviation for n = 50, **P < 0.01.
Abbreviation: FITC, fluorescein isothiocyanate.
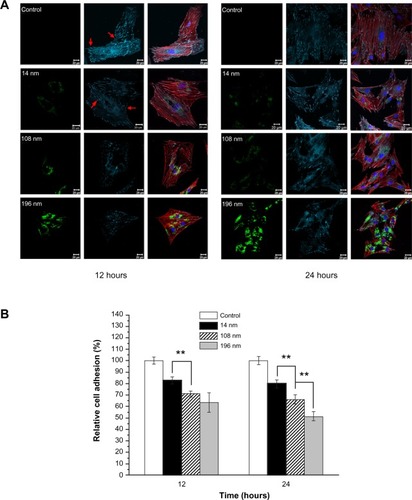
Adhesion is the first response of the cell when it comes into contact with a biomaterial, and plays an essential role in regulation of the subsequent biological behavior of cells. Previous research indicates that the adaptor protein of vinculin is a key regulator of focal adhesion, which is activated by binding of talin.Citation35 Compared with the controls, a distinct difference was observed regarding cell adhesion (vinculin spots, arrows) and the cytoskeletons of MSC treated with TiO2 nanoparticles. The larger the size of the TiO2 nanoparticles, the lower the amount of vinculin that was present (). A similar tendency was also observed for cell spreading, indicated by distribution of actin within cells.
The amount of vinculin in MSC treated with 14 nm TiO2 nanoparticles was significantly greater (P < 0.01) than that in MSC treated with 108 nm TiO2 nanoparticles, and yet greater (P < 0.01) in MSC treated with 196 nm TiO2 nanoparticles after culture for 24 hours (). The average amount of vinculin in MSC treated with 196 nm TiO2 nanoparticles decreased to 63.4% of control levels after culture for 12 hours, and decreased further to 51.3% of control levels after culture for 24 hours. These results suggest that TiO2 nanoparticle size strongly affects adhesion of MSC, which is consistent with previous reports.Citation16,Citation36
Cell migration
Focal adhesion of cells generally has a close relationship with cell migration.Citation37 To investigate the effect of TiO2 nanoparticles on cell migration, a wound healing (scrape migration) assay was performed, as described elsewhere.Citation38 After culture for 12 hours, many cells treated with 14 nm TiO2 nanoparticles migrated to the center of the wound field, whereas few cells treated with 108 nm TiO2 nanoparticles and even fewer cells treated with 196 nm TiO2 nanoparticles did so ().
Figure 5 Wound healing in mesenchymal stem cells incubated without (control) or with different TiO2 particles (0.10 m/mL) shown by confocal laser scanning microscopy.
Notes: Scale bar = 100 μm. The initial cell seeding density was 1 × 105 cells/cm2.
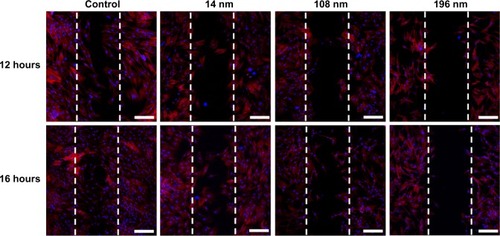
To investigate further the effects of TiO2 nanoparticles on cell migration, a matrigel invasion assay was performed using Transwell chambers. shows representative images for control MSC and those treated with TiO2 nanoparticles. Quantitative analysis showed that treatment with TiO2 nanoparticles significantly decreased migration of MSC. Moreover, the relative migration of MSC treated with 14 nm TiO2 nanoparticles was significantly greater (P < 0.01) than that of MSC treated with 108 nm TiO2 nanoparticles, and yet higher (P < 0.01) than migration of MSC treated with 196 nm TiO2 nanoparticles (). Our results suggest that TiO2 nanoparticle size strongly affects migration of MSC, and are consistent with those of a previous study of hydroxyapatite nanoparticles.Citation39
Figure 6 Characterization of cell migration. (A) Qualitative cell migration assay shown by Transwell assay after culture for 8 hours. a: control; b: 14 nm; c: 108 nm; d: 196 nm; and (B) quantitative analysis of relative cell migration from A (n = 100).
Notes: The initial cell seeding density was 1 × 105 cells/cm2. Scale bar = 100 μm. **P < 0.01.
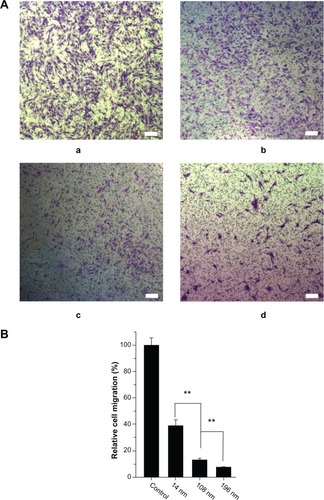
Cell differentiation
To investigate the effects of TiO2 nanoparticles on osteogenic differentiation of MSC, we firstly measured ALP activity in MSC treated with different-sized TiO2 nanoparticles (). ALP is an early mineralization-related protein marker for osteogenesis of osteoblasts.Citation40 MSC cultured with different-sized TiO2 nanoparticles showed significantly weaker (P < 0.01) ALP activity than the control group at all time points. After culture for 7 days, MSC treated with 14 nm and 108 nm TiO2 nanoparticles demonstrated significantly higher (P < 0.01) ALP activity than those treated with 196 nm TiO2 nanoparticles. After culture for 14 days, MSC treated with 14 nm TiO2 nanoparticles showed significantly more (P < 0.01) ALP activity than those treated with 108 nm and 196 nm TiO2 nanoparticles. Next, we measured mineralization ability in MSC treated with TiO2 nanoparticles of different sizes. The normalized mineralization demonstrated a trend similar to that for ALP activity after culture for 15 days ().
Figure 7 Alkaline phosphatase activity of mesenchymal stem cells cultured with different-sized TiO2 nanoparticles (0.10 mg/mL) for 7 and 14 days, respectively.
Notes: The initial cell seeding density was 2 × 104 cells/cm2. Error bars represent the mean ± standard deviation for n = 6, *P < 0.05, **P < 0.01.
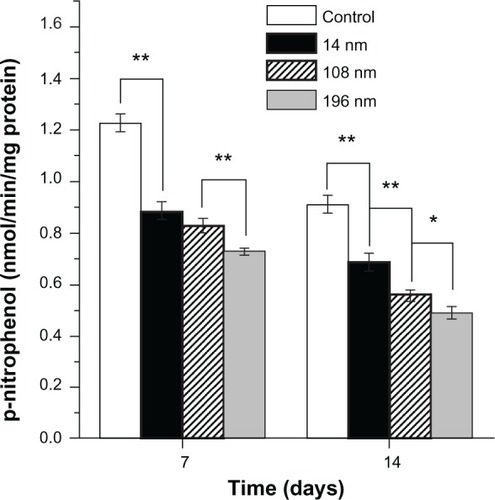
Figure 8 Quantification of cell mineralization. Mesenchymal stem cells were cultured without (control) or with different TiO2 particles (0.10 mg/mL) for 15 days.
Notes: The initial cell seeding density was 5 × 104 cells/cm2. Error bars represent the mean ± standard deviation for n = 6, **P < 0.01.
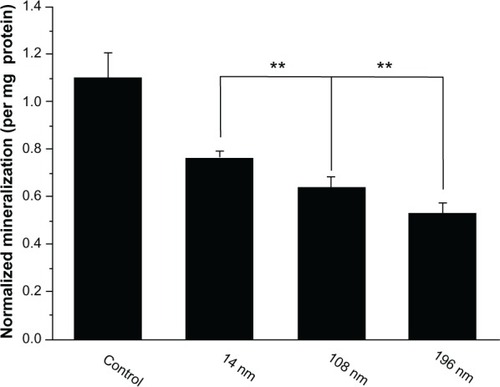
To investigate further the impact of TiO2 nanoparticles on differentiation of MSC, we characterized messenger RNA (mRNA) expression of osteocalcin and osteopontin by reverse transcription polymerase chain reaction. Osteocalcin and osteopontin are noncollagenous matrix proteins, which are regarded as mature osteoblast markers in MSC at a late stage.Citation41 We found that MSC treated with 196 nm TiO2 nanoparticles produced significantly less (P < 0.05 and P < 0.01) osteocalcin and osteopontin, respectively, than MSC treated with 14 nm and 108 nm TiO2 nanoparticles (). This result is consistent with the findings of a previous study showing that calcium phosphate-based particles of increasing size impaired osteogenic differentiation and bone matrix mineralization in MSC.Citation42 However, other research has confirmed that a high dose of zinc oxide nanoparticles is lethal to proliferating pluripotent MSC but has negligible toxicity in osteogenically differentiated MSC.Citation43 Nanohydroxyapatite and nanohydroxyapatite-poly(lactic-co-glycolic acid) (PLGA) composites promote osteogenic differentiation of human MSC.Citation44 In another study, iron oxide nanoparticles of different sizes and surface charge were found to regulate the differentiation of MSC.Citation45
Figure 9 Relative mRNA expression of osteocalcin and osteopontin analyzed by real-time polymerase chain reaction. The mesenchymal stem cells were cultured without or with different TiO2 particles (0.10 mg/mL) for 14 days.
Notes: The initial cell seeding density was 5 × 104 cells/cm2. Error bars represent the mean ± standard deviation for n = 5, *P < 0.05, **P < 0.01.
Abbreviations: mRNA, messenger RNA; OC, osteocalcin; OPN, osteopontin.
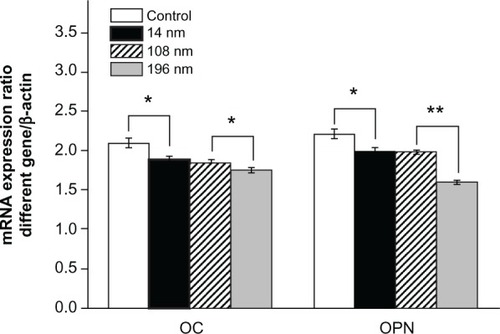
Discussion
In recent years, much effort has been made to improve the properties of nanostructured titanium implants.Citation3,Citation7,Citation8,Citation10 Nevertheless, nanoscale wear debris could potentially be generated during long-term implantation in the clinical setting, mainly because of abrasion and invasion of various enzymes and chemicals in an environment containing biological fluids. Therefore, investigation of the potential cytotoxicity of nanoscale debris released from a titanium implant is essential.
The head of a titanium joint implant not only comes into contact with osteoblasts derived from the surrounding bone tissue but also interacts with bone marrow stem cells in the long bone cavity. In a previous study,Citation23 we highlighted the size-dependent negative effects of different titanium nanoparticles on viability of osteoblasts, liver cells (L-02), and kidney cells (HEK 293), and investigated the size-dependent cellular uptake pathways and activation of matrix metalloproteinase 9. In this study, we used titanium nanoparticles (ie, a debris model) to investigate how titanium nanoparticles of different sizes affect adhesion and proliferation (ie, DNA synthesis), osteocalcin and osteopontin gene expression, and migration of MSC. Investigating the migration of MSC surrounding a titanium implant is of particular importance for understanding how MSC potentially contribute to osseous integration in implant/bone tissue.
Once wear debris (ie, TiO2 nanoparticle model) detaches from a titanium implant, it can affect the biological function of the surrounding cells in two ways, firstly by affecting protein adsorption around cells and secondly by being taken up directly by cells. The extent of this in both cases depends on particle size and nanotopography. However, the exact contribution of each factor needs to be investigated in more depth. Our interest was focused on the latter in this study.
Our results confirmed that the synthesized TiO2 nanoparticles (14 nm, 108 nm, and 196 nm) had a cytotoxic effect on MSC depending on their concentration (). This phenomenon could be interpreted as highly concentrated TiO2 nanoparticles producing oxidative stress as well as reactive oxygen species, leading to cell death.Citation34 Furthermore, we confirmed that growth and division of MSC were negatively affected in a size-dependent manner by the TiO2 nanoparticles ( and ) potentially via a mechanism causing intracellular acidity, thus inhibiting cell proliferation.Citation46
It is well known that uptake of nanoparticles can occur via different pathways in cells, depending on the nanoparticle dimensions. In a previous study, we confirmed that large TiO2 nanoparticles (74 nm and 196 nm) were taken up by osteoblasts mainly via clathrin-mediated and caveolae-mediated pathways, whereas small TiO2 nanoparticles (14 nm) were digested by osteoblasts through a caveolae-mediated pathway.Citation23 We assume that TiO2 nanoparticles were taken up by MSC in a similar manner. In our study, we found that greater amounts of 196 nm TiO2 nanoparticles accumulated within MSC than did 108 nm and 14 nm TiO2 nanoparticles (). Previously, Win et al demonstrated that Caco-2 cells took up much fewer 50 nm PLGA nanoparticles than particles with sizes of 200 and 500 nm.Citation47 Unlike the small (14 nm) TiO2 nanoparticles, high accumulation of large (196 nm) TiO2 nanoparticles within MSC led directly to more cytotoxicity, in turn affecting biological functions, such as migration, differentiation, and gene expression (–).
The relatively low accumulation of small-sized (14 nm) TiO2 nanoparticles might be interpreted as meaning that small nanoparticles could escape more easily from endocytic vesicles in cells, thus resulting in limited cytotoxicity to MSC. Pan et al confirmed that 1.2 nm gold nanoparticles were markedly less cytotoxic than 1.4 nm gold nanoparticles, despite a three-fold higher concentration.Citation48 Another example was internalization of FITC-mesoporous silica nanoparticles that did not affect cell viability, proliferation, or differentiation potential of MSC.Citation49 An explanation for this is that FITC-mesoporous silica nanoparticles could escape endosomal vesicles in MSC and retain their architectonic integrity after internalization.
On the other hand, the physicochemical properties (chemistry and surface charge) of a nanoparticle could affect its escape mechanism and potential cytotoxicity.Citation50 A previous study showed that poly-L-lysine-functionalized PLGA nanoparticles could escape more rapidly and efficiently from endosomes than native PLGA nanoparticles and result in 10-fold higher intracellular delivery of an encapsulated model protein.Citation51
Although the positive effects of the nanotopography of biomaterials on cell function are well documented, for instance, cell density and cell adhesion are greatly enhanced by surface-nanostructured biomaterials,Citation52,Citation53 the present study implies that loss of such a nanoscale structure may have negative effects on cell and tissue function. Thus, ensuring the stability of a nanostructured entity is one of the major concerns when nanostructuring a biomaterial or exploiting a new nanostructured biomaterial, in particular inorganic materials.
Conclusion
In this study, the cytotoxicity of a series of TiO2 nanoparticles was systematically investigated from the cellular to the molecular level. We confirmed that TiO2 nanoparticles of different sizes have a negative impact on viability, adhesion, migration, proliferation, and differentiation of MSC in a size-dependent and dose-dependent manner. Accumulation of such knowledge might provide new insights into the development of novel nanostructured titanium implants.
Acknowledgments
This work was financially supported by the China Ministry of Science and Technology (973 Project 2009CB930000), Natural Science Foundation of Chongqing Municipal Government (CSTC, 2011JJJQ10004), Natural Science Foundation of China (11032012, 31170923, 51173216), National Key Technology R&D Program of the Ministry of Science and Technology (2012BAI18B04), Fok Ying Tung Education Foundation (121035), and the “111” Project (B06023).
Disclosure
The authors report no conflicts of interest in this work.
References
- LongMRackHJTitanium alloys in total joint replacement – a materials science perspectiveBiomaterials19981918162116399839998
- NiinomiMMechanical biocompatibilities of titanium alloys for biomedical applicationsJ Mech Behav Biomed Mater200811304219627769
- KuboKTsukimuraNIwasaFUenoTSaruwatariLAitaHCellular behavior on TiO2 nanonodular structures in a micro-to-nanoscale hierarchy modelBiomaterials200930295319532919589591
- ZhaoGRainesALWielandMSchwartzZBoyanBDRequirement for both micron- and submicron scale structure for synergistic responses of osteoblasts to substrate surface energy and topographyBiomaterials200728182821282917368532
- MendoncaGMendoncaDBSAragaoFJLCooperLFAdvancing dental implant surface technology – from micron- to nanotopographyBiomaterials200829283822383518617258
- GuidaLAnnunziataMRocciAContaldoMRulloROlivaABiological response of human bone marrow mesenchymal stem cells to fluoride-modified titanium surfacesClin Oral Implants Res201021111234124120497444
- AnnunziataMOlivaABuoscioloAGiordanoMGuidaAGuidaLBone marrow mesenchymal stem cell response to nano-structured oxidized and turned titanium surfacesClin Oral Implants Res201223673374021679255
- HoriNIwasaFTsukimuraNEffects of UV photofunctionalization on the nanotopography enhanced initial bioactivity of titaniumActa Biomater20117103679369121723964
- McNamaraLESjöströmTBurgessKESkeletal stem cell physiology on functionally distinct titania nanotopographiesBiomaterials201132307403741021820172
- ChenXYCaiKYLaiMZhaoLTangLLMesenchymal stem cell differentiation on hierarchically micro/nano-structured titanium substratesAdv Eng Mater2012145B217B223
- CaiKYLaiMYangWHSurface engineering of titanium with potassium hydroxide and its effects on the growth behaviors of mesenchymal stem cellsActa Biomater2010662314232119963080
- St PierreCAChanMIwakuraYAyersDCKurt-JonesEAFinbergRWPeriprosthetic osteolysis: characterizing the innate immune response to titanium wear-particlesJ Orthop Res201028111418142420872576
- GalvinALTipperJLInghamEFischerJNanometer size wear debris generated from crosslinked and non-crosslinked ultra high molecular weight polyethylene in artificial jointsWear2005259977983
- GerhardtLCJellGMBoccacciniARTitanium dioxide (TiO2) nanoparticles filled poly(D,L lactid acid) (PDLLA) matrix composites for bone tissue engineeringJ Mater Sci Mater Med20071871287129817211724
- ChoiMGKohHSKluessDEffects of titanium particle size on osteoblast functions in vitro and in vivoProc Natl Acad Sci U S A2005102124578458315755807
- SaldañaLVilaboaNEffects of micrometric titanium particles on osteoblast attachment and cytoskeleton architectureActa Biomater2010641649166019861182
- PetersKUngerREKirkpatrickCJGattiAMMonariEEffects of nano-scaled particles on endothelial cell function in vitro: studies on viability, proliferation and inflammationJ Mater Sci Mater Med200415432132515332593
- WangJJSandersonBJWangHCyto-and genotoxicity of ultrafine TiO2 particles in cultured human lymphoblastoid cellsMutat Res200762829910617223607
- RahmanQLohaniMDoppEEvidence that ultrafine titanium dioxide induces micronuclei and apoptosis in Syrian hamster embryo fibroblastsEnviron Health Perspect2002110879780012153761
- MaLLLiuJLiNOxidative stress in the brain of mice caused by translocated nanoparticulate TiO2 delivered to the abdominal cavityBiomaterials20103119910519783296
- DuanYMLiuJMaLLToxicological characteristics of nanoparticulate anatase titanium dioxide in miceBiomaterials201031589489919857890
- WangJXFanYBGaoYHuQHWangTCTiO2 nanoparticles translocation and potential toxicological effect in rats after intra-articular injectionBiomaterials200930274590460019500841
- CaiKYHouYHHuYCorrelation of the cytotoxicity of TiO2 nanoparticles with different particle sizes on a sub-200-nm scaleSmall20117213026303121919195
- LiuXYRenXFDengXYA protein interaction network for the analysis of the neuronal differentiation of neural stem cells in response to titanium dioxide nanoparticlesBiomaterials201031333063367020071024
- HuYCaiKYLuoZRegulation of the differentiation of mesenchymal stem cells in vitro and osteogenesis in vivo by microenvironmental modification of titanium alloy surfacesBiomaterials201233133515352822333987
- ChenJZhouHSantulliACWongSSEvaluating cytotoxicity and cellular uptake from the presence of variously processed TiO2 nanostructured morphologiesChem Res Toxicol201023587187920408587
- ZhangYYHuLYuDHGaoCYInfluence of silica particle internalization on adhesion and migration of human dermal fibroblastsBiomaterials201031328465847420701964
- LaiMCaiKYZhaoLChenXYHouYHYangZXSurface functionalization of TiO2 nanotubes with bone morphogenetic protein 2 and its synergistic effect on the differentiation of mesenchymal stem cellsBiomacromolecules20111241097110521381690
- LeeHYKaleGMHydrothermal synthesis and characterization of nano-TiO2Int J Appl Ceram Technol200856657665
- MahmoudiMAzadmaneshKShokrgozarMAJourneayWSLaurentSEffect of nanoparticles on the cell life cycleChem Rev201111153407343221401073
- LiuYLiWLaoFIntracellular dynamics of cationic and anionic polystyrene nanoparticles without direct interaction with mitotic spindle and chromosomesBiomaterials201132328291830321810539
- KangSJKimBMLeeYJChungHWTitanium dioxide nanoparticles trigger p53-mediated damage response in peripheral blood lymphocytesEnviron Mol Mutagen200849539940518418868
- KarlssonHLCronholmPGustafssonJMöllerLCopper oxide nanoparticles are highly toxic: a comparison between metal oxide nanoparticles and carbon nanotubesChem Res Toxicol20082191726173218710264
- PanZLeeWSlutskyLClarkRAPernodetNRafailovichMHAdverse effects of titanium dioxide nanoparticles on human dermal fibroblasts and how to protect cellsSmall20095451152019197964
- HumphriesJDWangPStreuliCGeigerBHumphriesMJBallestremCVinculin controls focal adhesion formation by direct interactions with talin and actinJ Cell Biol200717951043105718056416
- ThurnKTAroraHPauneskuTEndocytosis of titanium dioxide nanoparticles in prostate cancer PC-3M cellsNanomedicine20117212313020887814
- HuKJiLApplegateKTDanuserGWaterman-StorerCMDifferential transmission of actin motion within focal adhesionsScience2007315580811111517204653
- HuYCaiKYLuoZTiO2 nanotubes as drug nanoreservoirs for the regulation of mobility and differentiation of mesenchymal stem cellsActa Biomater20128143944822040682
- WangZXHouYHanWEffects of hydroxyapatite nanoparticles on apoptosis and invasion of human renal cell carcinoma 786-0 cellsChem Res Chinese Uni20112719498
- GundbergCMLookerACNiemanSDCalvoMSPatterns of osteocalcin and bone specific alkaline phosphatase by age, gender, and race or ethnicityBone200231670370812531565
- DalbyMJMcCloyDRobertsonMWilkinsonCDOreffoROOsteoprogenitor response to defined topographies with nanoscale depthsBiomaterials20062781306131516143393
- SaldañaLSánchez-SalcedoSIzquierdo-BarbaICalcium phosphate-based particles influence osteogenic maturation of human mesenchymal stem cellsActa Biomater2009541294130519114315
- TaccolaLRaffaVRiggioCZinc oxide nanoparticles as selective killers of proliferating cellsInt J Nanomedicine201161129114021698081
- LockJLiuHNanomaterials enhance osteogenic differentiation of human mesenchymal stem cells similar to a short peptide of BMP-7Int J Nanomedicine201162769277722114505
- JoJAokiITabataYDesign of iron oxide nanoparticles with different sizes and surface charges for simple and efficient labeling of mesenchymal stem cellsJ Control Release2010142346547319932720
- KwonSYLinTTakeiHAlterations in the adhesion behavior of osteoblasts by titanium particle loading: inhibition of cell function and gene expressionBiorheology2001382–316118311381173
- WinKYFengSSEffects of particle size and surface coating on cellular uptake of polymeric nanoparticles for oral delivery of anticancer drugsBiomaterials200526152713272215585275
- PanYNeussSLeifertASize-dependent cytotoxicity of gold nanoparticlesSmall20073111941194917963284
- HuangDMHungYKoBSHighly efficient cellular labeling of mesoporous nanoparticles in human mesenchymal stem cells: implication for stem cell trackingFASEB J200519142014201616230334
- GanQWangTCochraneCMcCarronPModulation of surface charge, particle size and morphological properties of chitosan-TPP nanoparticles intended for gene deliveryColloid Surf B Biointerfaces2005442–36573
- VasirJKLabhasetwarVQuantification of the force of nanoparticle-cell membrane interactions and its influence on intracellular trafficking of nanoparticlesBiomaterials200829314244425218692238
- SamarooHDLuJWebsterTJEnhanced endothelial cell density on NiTi surfaces with sub-macron to nanometer roughnessInt J Nanomedicine200831758218488418
- TranPWebsterTJEnhanced osteoblast adhesion on nanostructured selenium compacts for anti-cancer orthopedic applicationsInt J Nanomedicine20083339139618990948