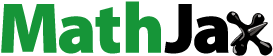
Abstract
A new layered organic–inorganic nanocomposite material with an anti-parkinsonian active compound, L-3-(3,4-dihydroxyphenyl) alanine (levodopa), intercalated into the inorganic interlayers of a Zn/Al-layered double hydroxide (LDH) was synthesized using a direct coprecipitation method. The resulting nanocomposite was composed of the organic moiety, levodopa, sandwiched between Zn/Al-LDH inorganic interlayers. The basal spacing of the resulting nano-composite was 10.9 Å. The estimated loading of levodopa in the nanocomposite was approximately 16% (w/w). A Fourier transform infrared study showed that the absorption bands of the nanocomposite were characteristic of both levodopa and Zn/Al-LDH, which further confirmed intercalation, and that the intercalated organic moiety in the nanocomposite was more thermally stable than free levodopa. The resulting nanocomposite showed sustained-release properties, so can be used in a controlled-release formulation. Cytotoxicity analysis using an MTT assay also showed increased cell viability of 3T3 cells exposed to the newly synthesized nanocomposite compared with those exposed to pure levodopa after 72 hours of exposure.
Introduction
Levodopa has remained the gold standard symptomatic replacement therapy for Parkinsonism, a progressive neurodegenerative disease, over the past 40 years. However, long-term treatment is often complicated by dyskinesia, a form of motor dysfunction, which is attributed to the discontinuous and intermittent delivery of levodopa to the brain, resulting in nonphysiological pulsatile stimulation of striatal dopamine receptors.Citation1,Citation2 Dyskinesia is observed in 40% of patients with Parkinson disease after 5 years (the honeymoon period) of levodopa use and in over 80% of patients after 10 years of using levodopa.Citation2
Tailored nanoparticles are good drug delivery agents because of their sustained-release and controlled-release properties. It is widely believed that reducing the pulsatile stimulation of dopaminergic neurons will reduce the risk of levodopa-induced dyskinesia.Citation2
Use of nanotechnology in the medical science field and in nanomedicine has reached an advanced stage, where much research has been conducted on the synthesis of nanoparticles that can be used as delivery tools for anticancer agents, central nervous system tumor agents,Citation3,Citation4 antibiotics,Citation5 and nonsteroidal anti-inflammatory drugsCitation6 in gene delivery and imaging studies.Citation7,Citation8
Layered double hydroxides (LDHs), also known as hydrotalcite-like compounds or anionic clays, are a broad class of inorganic lamellar compounds with a high capacity for anion intercalation. The chemical composition of LDHs is expressed by the general formula
where M2+ and M3+ are divalent and trivalent metal cations, respectively. These hydroxides are derived from brucite [Mg(OH)2], and they can be found in nature or be synthesized in a laboratory via coprecipitation method. The interlayer region contains various amounts of water, which is hydrogen-bonded to the hydroxide layers and/or to the interlayer anions.Citation9 A characteristic feature of LDH is weak bonding between the interlayer anions and hydroxide sheets that enables exchange of anions.Citation10
LDHs are less toxic than other conventional drug carriers and are generally biocompatible, making them an acceptable alternative for drug deliveryCitation11 Anionic drugs, such as levodopa, which replace the interlayer anions lying between the two positive metal hydroxide sheets, enable a controllable ion-exchange mechanism. pH dependency and the ability for controlled-release are other advantages of using LDHs as drug delivery systems. Further, the positively charged outer layer of the nanohybrid delivery system, which can attract a negatively charged cell membrane, enables easy penetration of LDH into cells. This type of research has not as yet focused on neurodegenerative diseases. Hence, the aim of this study was to intercalate levodopa into LDH for the treatment of Parkinson’s disease.
Materials and methods
Materials
L-3-(3,4-dihydroxyphenyl) alanine (levodopa, 99% purity) was purchased from Sigma-Aldrich (St Louis, MO, USA). Other chemicals, including Zn(NO3)2 ⋅ 6H2O and Al(NO3)3 ⋅ 9H2O, were of analytical grade and used without further characterization. Deionized water was used throughout the experiment, unless stated otherwise.
Synthesis
Zn/Al-LDH (the nanolayer) was synthesized in nitrate form by adding a solution of sodium hydroxide (1 molar) dropwise to a solution of zinc and aluminum nitrate, with a molar ratio of 2:1, in deionized water under a nitrogen atmosphere while vigorously stirring until a pH of 7.0 was reached. The mixture was aged in an oil bath for 18 hours at 70°C. The white precipitate obtained was centrifuged washed three times with deionized water, and dried in an oven overnight at 70°C. The resulting Zn/Al-LDH was used in a cytotoxicity study and compared with its nanocomposite and levodopa.
Levodopa-Zn/Al-LDH (the nanocomposite) was synthesized using a direct coprecipitation method. In brief, a solution of levodopa (0.08 molar) was added to a Zn(NO3)2 ⋅ 6H2O and Al(NO3)3 ⋅ 9H2O solutions, at a ratio of 2:1, under constant stirring in the presence of a sustained nitrogen supply at room temperature, and the pH was adjusted to 7.0 using 1.0 molar NaOH. The experiment was protected from direct sunlight exposure because of the sensitivity of levodopa to light. The mixture was aged at 70°C in an oil bath for 18 hours and then centrifuged filtered washed with deionized water three times, and dried in an oven overnight.
Controlled-release study
Drug release profiles were determined at room temperature using phosphate-buffered saline at pH 4.8 and 7.4. Approximately 300 mg of the nanocomposite was added to 500 mL of the medium. The accumulated amount of levodopa released into the solution was measured at preset time intervals and at λmax = 280 nm using an ultraviolet-visible spectrophotometer (Lambda 35, Perkin-Elmer, Boston, MA, USA).
Cell culture
3T3 cells (fibroblasts) were purchased from the American Type Culture Collection ((ATCC), Manassas, VA, USA), cultured in RPMI 1640 medium supplemented with 10% fetal bovine serum, L-glutamine 15 mmol/L, penicillin 100 U/mL, and streptomycin 100 μg/mL, and were then incubated in 5% CO2 at 37°C. Cells at 80%–90% confluence were used for seeding and treatment.
Seeding and treatment
For the cytotoxicity study, cells were seeded in a 96-well plate at a density of 0.5 × 105 cells/mL (100 μL/well) and allowed 24 hours to attach before treatment. A stock solution containing 10 mg/mL of the nanocomposite, the nanohybrid and levodopa in phosphate-buffered saline was made, diluted in RPMI 1640 complete medium to the desired concentration, and subjected to sonication before being added to the cells. Cell treatment was conducted using a series of dilutions ranging from 150 μg/mL to 0 μg/mL (the control), and readings were taken 72 hours after treatment using a microplate reader (EL 800×, Bio-Tek Instruments Inc, Winooski, VT, USA) after adding MTT (3-(4,5-dimethylthiazol-2-yl)-2,5-diphenyltetrazolium bromide). This assay is dependent upon reduction of the tetrazolium salt by mitochondrial dehydrogenase in viable cells, which forms a blue formazan product (Mosmann 1983).Citation12 In brief, 20 μL of MTT solution (5 mg/mL in phosphate-buffered saline) was added to each well, and the plates were kept in an incubator in 5% CO2 at 37°C for 3 hours and centrifuged at 1000 rpm for 5 minutes. The supernatant was discarded and 100 μL of dimethyl sulfoxide was added per well. After keeping this mixture in the dark at room temperature for 30 minutes, absorbance was measured at a wavelength of 570 nm. Experiments were conducted in triplicate, and the cytotoxicity was calculated as:
Characterization
Powder X-ray diffraction patterns were recorded in the 2°−60° range on a diffractometer (XRD-6000, Shimadzu, Tokyo, Japan) using CuKα radiation (λ = 1.5418 Å) at 30 kV and 30 mA, with a dwell time of 4 degrees per minute. The Fourier transform infrared spectra of the materials were recorded at 400–4000 cm−1 using a Thermo Nicolet Nexus FTIR (model Smart Orbit) (International Equipment Trading Ltd. Vernon Hills, IL, USA). The chemical compositions of the samples were analyzed for zinc and aluminum ion content using inductively coupled plasma atomic emission spectrometry (Optima 2000DV, Perkin-Elmer) under standard conditions. An elemental analyzer was used for C, H, N, and S analyses (CHNS-932, LECO Corporation, Saint Joseph, MI, USA). Thermogravimetric and differential thermogravimetric analyses (Mettler Toledo, Columbus, OH, USA) were carried out at a heating rate of 10°C per minute from 20° to 1000°C under a nitrogen atmosphere (N2 flow rate of 50 mL per minute). A field emission scanning electron microscope (Nova™ NanoSEM 230, FEI Company, Hillsboro, OR, USA) was used to determine the surface morphology of the samples.
Results and discussion
Powder X-ray diffraction
The X-ray diffraction patterns of levodopa intercalated into Zn/Al-LDH and of free levodopa are shown in , respectively. As shown in , the (003), (006), and (009) reflections were found at 2θ values of approximately 8.06°, 16.04°, and 24.52°, respectively. As reported in the literature, the (003) reflection for the precursor Zn/Al-NO3 LDH appears at 9.98°, indicating a d spacing of 8.90 Å.Citation13 The shifting of this reflection toward low 2θ (2θ = 8.06°, corresponding to a d spacing of 10.9 Å) for the nanocomposite indicates intercalation of levodopa into the interlayer galleries of the LDH. The characteristic reflections of levodopa, shown in , were absent from the X-ray diffraction pattern of the nanocomposite, suggesting that levodopa was intercalated into the interlayer galleries rather than absorbed at the surface. The broader (003) reflection peak in might have been caused by the formation of a secondary phase due to cointercalation of the counter ion (NO3−).
Spatial orientation of intercalated levodopa
shows the three-dimensional molecular size of levodopa, which was obtained using ChemOffice 2008 software (Cambridge, MA, USA). The long and short axes (x axis and y axis, respectively) and molecular thickness (z axis) of levodopa were calculated, giving values of 10.4 Å, 7.1 Å, and 6.2 Å, respectively. The X-ray diffraction pattern shows a basal spacing (d) of 10.9 Å for the nanocomposite. The thickness of the Zn/Al-NO3 LDH layer was 4.8 Å.Citation14 Therefore, the gallery height of LDH after the intercalation processes was 6.1 Å (10.9–4.8 Å). This gallery height of the nanocomposite, 6.1 Å, is similar to the thickness axis of levodopa (6.2 Å). This result indicates that the levodopa anions are accommodated as an alternate monolayer (see ).
Infrared spectroscopy
The Fourier transform infrared spectra for pristine levodopa and levodopa intercalated into the Zn/Al interlayer are shown in . The spectrum for levodopa () shows many intense, sharp absorption peaks that arise from the different functional groups present in the molecules, ie, the primary amine, carboxylic acid, benzene ring, and hydroxyl groups.
Figure 3 Fourier transform infrared spectra of free levodopa (A) and levodopa-Zn/Al-layered double hydroxide nanocomposite (B).
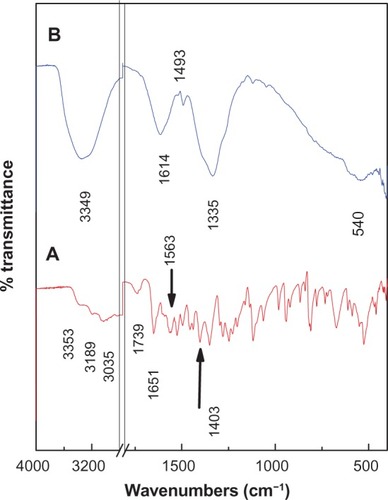
The carboxylic group for levodopa shows three absorption peaks: 1245 cm−1 for the C−O stretching, 1739 cm−1 for the C═O bond, and a broad band at 1400–1440 cm−1for the O−H bending vibration. The bands at 3353, 3189, and 3035 cm−1 can be attributed to the ν(N−H), ν(O−H), and ν(Ar−H) vibrations, respectively. The primary amine shows absorption peaks at 1651 cm−1and 1563 cm−1 that can be attributed to N−H bending, while the peaks between 1064 cm−1 and 1200 cm−1 are due to C−N stretching, and the peak at approximately 800 cm−1 indicates N−H (oop) bending. The band at 1496 cm−1 can be attributed to the C═C bond in the benzene ring.
The Fourier transform infrared spectrum of the levodopa intercalated into Zn/Al-LDH is shown in . The spectrum of the nanocomposite shows the characteristic bands of pure levodopa, which indicate that the levodopa anions were intercalated into the interlayer galleries of LDH. Some of the bands are slightly shifted. For example, the position of the band for the C═C bond shifted from 1496 cm−1 for free levodopa to 1493 cm−1 for the nanocomposite, and the band at 1118 cm−1 for the C−N bond shifted to 1120 cm−1; these shifts are due to the interaction between the levodopa anion and the interlayers as a result of the intercalation process. The appearance of a new broad peak at 1614 cm−1 for the nanocomposite can be assigned to the asymmetric stretching vibration of the COO− group.Citation14 The band at 1335 cm−1 is due to the nitrate anion, which may not be completely removed from the interlayers during the intercalation process.Citation15
Elemental analysis
Elemental (CHNS) and inductively coupled plasma analyses were conducted to determine the organic and inorganic compositions of the nanocomposite. As expected, the levodopa-nanocomposite contained both organic and inorganic constituents. This result indicates that the levodopa was incorporated into the Zn/Al-LDH inorganic interlayers.
The percentage loading of levodopa in the nanocomposite is 16.00%, as shown in . The C/N ratio for free levodopa is 8.09, and this value is higher than the C/N ratio of the nanocomposite, possibly indicating that the nitrate anion was not completely removed from the interlayers during the intercalation process. This result was confirmed by the presence of a very broad peak at low 2θ in the X-ray diffraction pattern. From the elemental chemical analysis and thermogravimetric studies, the empirical formula for the nanocomposite can be proposed as: be
Table 1 Elemental chemical compositional for free levodopa and its nanocomposite
Thermal analysis
The thermal behavior of the compounds before and after intercalation of levodopa into Zn/Al-LDH was examined using thermogravimetric and derivative thermogravimetric analysis. The thermograms of levodopa and the nanocomposite are shown in . The free levodopa () thermal decomposition exhibited two weight loss events above 270°C. The first sharp event occurred at 288°C with a weight loss of 42.0%, which was due to the decomposition of levodopa. The second weight loss at 381°C–666°C can be attributed to the combustion of levodopa.
Figure 4 Thermogravimetric and differential thermogravimetric thermograms of levodopa (A) and levodopa-nanocomposite (B).
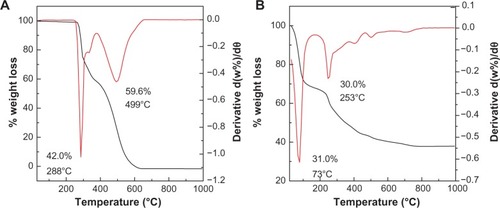
After the intercalation process, the thermal decomposition characteristics of the resulting product were different from those of the precursor. The thermal decomposition of the nanocomposite was characterized by two weight loss events, one at 35°C–162°C with a weight loss of 31.0%, due to the removal of the external surface-adsorbed and interlayer water molecules, and a second event at 162°C–960°C with a weight loss of 30.0%. This second weight loss was due to dehydroxylation of the layers and decomposition of levodopa.
Surface properties
In , the surface morphologies of the Zn/Al-nanolayer (A and B) and levodopa-nanocomposite (C) are shown. The micrographs were obtained using field emission scanning electron microscopy at (A) 8000×, (B) 15,000×, and (C) 30,000× magnifications. Zn/Al-LDH and the nanocomposite show nonuniform, irregular agglomerates with compact and nonporous plate-like structures, which are commonly observed for LDH and its nanocomposites.
Release behavior of levodopa from the Zn/Al-levodopa nanocomposite
Typical release behavior profiles of levodopa from its nano-composite at different pHs are shown in , indicating that the release rate of levodopa from the nanocomposite is pH-dependent. The release rate at pH 7.4 is remarkably lower than that at pH 4.8. The percentage release of levodopa from the nanocomposite reaches approximately 74% within 2400 minutes when exposed to a pH of 4.8. When the pH is changed to 7.4, the release rate of levodopa from the nanocomposite is obviously lower. The time for 76% of the drug to be released from the nanocomposite is approximately 8600 minutes. This lower release rate of levodopa from the nanocomposite at pH 7.4 indicates that the levodopa-Zn/Al nanocomposite is indeed a potential drug delivery system. The difference in the release rates at pH 4.8 and pH 7.4 may be due to the difference in the release mechanism of levodopa from the nanocomposite.Citation16 At an acidic/lower pH of 4.8, Zn/Al-LDH begins to dissolve. At pH 7.4, the Zn/Al-LDH is more stable because of electrostatic interaction between the levodopa anions and the positively charged Zn/Al-LDH layers. Thus, the release mechanism at pH 4.8 should occur through both dissolution of the LDH layers and ion exchange, while the mechanism at pH 7.4 should occur via ion exchange with the ions in the buffer solution.Citation16
Release kinetics of levodopa from the Zn/Al-levodopa nanocomposite
To investigate the exact mechanism of levodopa release from the Zn/Al-LDH interlayer, the release profile data were fitted using mathematical models that describe various kinetics. The pseudo-first order kinetics mathematical model equation ln(qe − qt) = lnqe − k1t (qe and qt are the equilibrium release amount and the release amount at time t, respectively),Citation17 the pseudo-second order equation t/qt = 1/k2qe2 + t/qe18 and the parabolic diffusion equation (1 − Mt/Mo)/t = kt−0.5 + b (Mo and Mt are the drug content that remained in LDH at the release times of 0 and t, respectively)Citation19 were used.
The fitting results of the drug release profiles at pH 7.4 and 4.8 based on the three different kinetic models are shown in . The correlation coefficient (R2) is tabulated in . From , it can be seen that the release of levodopa from Zn/Al-LDH follows the pseudo-second order equation very well for both pH values, with satisfactory coefficients of 0.9988 (pH 7.4) and 0.9952 (pH 4.8). The rate constant for the pseudo-second order equation is 2.85 × 10−5 mg per minute and 4.29 × 10−5 mg per minute at pH 7.4 and pH 4.8, respectively (). The kinetic results obtained during this work are very similar to those from a kinetic study of the release of camptothecin from Mg/Al-LDHCitation16 and are also similar to those for perindopril erbumine intercalated into Zn/Al-LDH using the ion-exchange and coprecipitation methods.Citation20
Table 2 Correlation coefficients (R2), and rate constants (k) obtained by fitting the levodopa release data for the nanocomposite in solutions at pH 4.8 and 7.4
Cytotoxicity analysis of the nanocomposite, nanohybrid, and levodopa
Compared with the control, cell viability was found to decrease with increasing concentrations of levodopa, the nanolayer, and the nanocomposite. The 3T3 cell viability was reduced by approximately 40% when the concentration of levodopa was more than 50 μg/mL. For the nanolayer alone, cell viability was reduced by more than 40% when the concentration of the hybrid exceeded 100 μg/mL. We observed that the levodopa nanocomposite reached a higher cell viability compared with the carrier nanolayer or with the drug alone in a dose-dependent manner after 72 hours of exposure, as shown in .
Figure 8 In vitro cytotoxicity study of 3T3 cells after 72 hours of exposure to free levodopa, the nanolayer, and the levodopa nanocomposite.
Notes: Using the graph as well as regression analysis, the IC50 (concentration of the drug required to induce 50% cell mortality) for the nanocomposite, nanolayer, and levodopa was 202 ± 3.55 μg/mL, 161 ± 6.42 μg/mL, and 73 ± 6.46 μg/mL, respectively (P < 0.005 for all three, showing statistical significance compared with the control).
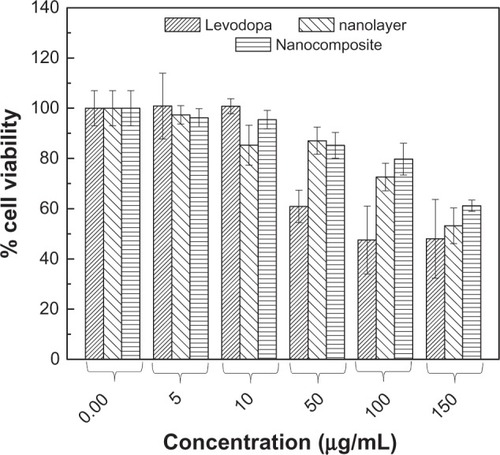
In vitro, cytotoxicity testing is an essential step in analyzing the health hazards of synthesized nanomaterials in terms of the cellular response to a toxicant for a biomedical application. Therefore, in the present study, we investigated the cytotoxic effect of the synthesized nanocomposite, nanohybrid, and pristine levodopa doses using a biological model with 3T3 fibroblast cells and an MTT assay.
It was found that exposure to the nanocomposite at a dose level of 5–150 μg/mL caused dose-dependent cytotoxicity in 3T3 cells, as shown in , but the same dose of pristine levodopa but more cytotoxicity after 72 hours of exposure compared with the nanocomposite. Findings by other researchers have demonstrated a similar pattern of toxicity in primary rat hepatocytes after exposure to allergic acid and its nanocomposite, ie, the allergic acid caused more toxicity compared with its nanocomposite using the same dose range after 6 hours of exposure.Citation21
Our results also demonstrated more toxicity in 3T3 cells following exposure to the nanolayer compared with exposure to the nanocomposite at the same doses after 72 hours. In previously reported studies, a decrease in the viability of human umbilical vein endothelial cells was observed following exposure to an LDH nanolayer compared with that following exposure to its nanocomposite, which was functionalized by poly(sulfobetaine).Citation22 Meanwhile, in another report, Chang liver cells exposed to an LDH nanolayer also exhibited a slight decrease in viability compared with those exposed to its nanocomposite containing cetirizine.Citation23 Our findings are supported by the above-mentioned studies using nanolayers and their corresponding nanocomposites. The results also indicate that our nanocomposite is likely to be a biocompatible product because it shows less cytotoxicity than pristine levodopa, which is very important for the potential use of the nanocomposite in an in vivo study.
Conclusion
Levodopa-Zn/Al-LDH nanocomposites were synthesized using the coprecipitation method with a basal spacing of 10.9 Å and 16.0% (w/w) loading of levodopa. The intercalation of levodopa into the interlayer spacing of LDH is shown by Fourier transform infrared spectra. The intercalated guest molecules of levodopa were found to be arranged in a monolayer manner between the inorganic interlayers. Release of levodopa from the nanocomposite was found to be governed by pseudo-second order kinetics, and the release time of levodopa from the nanocomposite at pH 7.4 was longer than that at pH 4.8. The cytotoxicity study also demonstrated a decrease in the toxicity potential of levodopa in a normal cell line following its intercalation into Zn/Al-LDH. Hence, successful intercalation of levodopa may improve drug delivery systems for the treatment of parkinsonism as well as decrease its potential toxicity to cells.
Acknowledgment
We would like to thank the Ministry of Science, Technology, and Innovation Malaysia for project funding under nanofund NND/NA/(I) TD11-010.
Disclosure
The authors report no conflicts of interest in this work.
References
- PoeweWAntoniniAZijlmansJCMBurkhardPRVingerhoetsFLevodopa in the treatment of Parkinson’s disease: an old drug still going strongClin Interv Aging2010522923820852670
- Aviles-OlmosIMartinez-FernandezRFoltynieTL-dopa-induced dyskinesias in Parkinson’s diseaseEur Neurol J20102291100
- ChoiSJOhJMChoyJHAnticancer drug-layered hydroxide nanohybrids as potent cancer chemotherapy agentsJ Phys Chem Solids2008695–615281532
- VergoniAVTosiGTacchiRVandelliMABertoliniACostantinoLNanoparticles as drug delivery agents specific for CNS: in vivo biodistributionNanomedicine20095436937719341816
- SilionMPopaMILisaGHritcuDNew hybrid compounds containing intercalated ciprofloxacin into layered double hydroxides: synthesis and characterizationRevue Roumaine de Chimie2008539827831
- Del ArcoMCebaderaEGutierrezSMg, Al layered double hydroxides with intercalated indomethacin: synthesis, characterization, and pharmacological studyJ Pharm Sci20049361649165815124221
- WongYMarkhamKXuZPEfficient delivery of siRNA to cortical neurons using layered double hydroxide nanoparticlesBiomaterials201031338770877920709387
- Flesken-NikitinAToshkovINaskarJToxicity and biomedical imaging of layered nanohybrids in the mouseToxicol Pathol2007356804810
- XuZPLuGQLayered double hydroxide nanomaterials as potential cellular drug delivery agentsPure Appl Chem200678917711780
- KovandaFJindovaEDousovaBKolousekDPlestilJSedlakovaZLayered double hydroxides intercalated with organic anions and their application in preparation of LDH/polymer nanocompositesActa Geodyn Geomater20096111119
- LiFJinLHanJWeiMLiCSynthesis and controlled release properties of prednisone intercalated Mg-Al layered double hydroxide compositeInd Eng Chem Res2009481255905597
- MosmannTRapid colorimetric assay for cellular growth and survival: Application to proliferation and cytotoxicity assaysJ Immunol Methods1983651–25683
- XiaSJNiZMXuQHuBXHuJLayered double hydroxides as supports for intercalation and sustained release of antihypertensive drugsJ Solid State Chem20081811026102619
- Al AliSHHAl-QubaisiMHusseinMZIsmailMZainalZHakimMNControlled release and angiotensin-converting enzyme inhibition properties of an antihypertensive drug based on a perindopril erbumine-layered double hydroxide nanocompositeInt J Nanomedicine201272129214122619549
- YaghiOMLiHT-Shaped molecular building units in the porous structure of Ag (4, 4′-bpy). NO3J Am Chem Soc19961181295296
- TynerKMSchiffmanSRGiannelisEPNanobiohybrids as delivery vehicles for camptothecinJ Control Release200495350151415023461
- DongLYanLHouW-GLiuS-JSynthesis and release behavior of composites of camptothecin and layered double hydroxideJ Solid State Chem2010183818111816
- HoYSOfomajaAEPseudo-second-order model for lead ion sorption from aqueous solutions onto palm kernel fiberJ Hazard Mater20061291–313714216188379
- KongXShiSHanJZhuFWeiMDuanXPreparation of glycy-l-tyrosine intercalated layered double hydroxide film and its in vitro release behaviorChem Eng J20101572–3598604
- Al AliSHHAl-QubaisiMHusseinMZZainalZHakimMNControlled release and angiotensin-converting enzyme inhibitor properties of an antihypertensive drug based on a perindopril erbumine-layered double hydroxide nanocompositeInt J Nanomedicine201272129214122619549
- HusseinMZAl AliSHZainalZHakimMNDevelopment of antiproliferative nanohybrid compound with controlled release property using ellagic acid as the active agentInt J Nanomedicine201161373138321796241
- XuFJPreparation and evaluation of well-defined hemocompatible layered double hydroxide-poly (sulfobetaine) nanohybridsJ Mater Chem201222301536215369
- Hussein-Al-AliSHAl-QubaisiMHusseinMZIsmailMZainalZHakimMNIn vitro inhibition of histamine release behavior of cetirizine intercalated into Zn/Al-and Mg/Al-layered double hydroxidesInt J Mol Sci20121355899591622754339