Abstract
Introduction
This work aimed to develop chitosan-coated cubosomal nanoparticles intended for nose-to-brain delivery of paliperidone palmitate. They were compared with standard and cationic cubosomal nanoparticles. This comparison relies on numerous classical in vitro tests and powder deposition within a 3D-printed nasal cast.
Methods
Cubosomal nanoparticles were prepared by a Bottom-up method followed by a spray drying process. We evaluated their particle size, polydispersity index, zeta-potential, encapsulation efficiency, drug loading, mucoaffinity properties and morphology. The RPMI 2650 cell line was used to assess the cytotoxicity and cellular permeation. An in vitro deposition test within a nasal cast completed these measurements.
Results
The selected chitosan-coated cubosomal nanoparticles loaded with paliperidone palmitate had a size of 305.7 ± 22.54 nm, their polydispersity index was 0.166 ± 0.022 and their zeta potential was +42.4 ± 0.2 mV. This formulation had a drug loading of 70% and an encapsulation efficiency of 99.7 ± 0.1%. Its affinity with mucins was characterized by a ΔZP of 20.93 ± 0.31. Its apparent permeability coefficient thought the RPMI 2650 cell line was 3.00E-05 ± 0.24E-05 cm/s. After instillation in a 3D-printed nasal cast, the fraction of the injected powder deposited in the olfactory region reached 51.47 ± 9.30% in the right nostril and 41.20 ± 4.59% in the left nostril, respectively.
Conclusion
The chitosan coated cubosomal formulation seems to be the most promising formulation for nose-to-brain delivery. Indeed, it has a high mucoaffinity and a significantly higher apparent permeability coefficient than the two other formulations. Finally, it reaches well the olfactory region.
Introduction
Paliperidone palmitate (PP) is a second-generation antipsychotic drug (SGAs)Citation1 used in the treatment of schizophrenia.Citation2 Antipsychotic drugs have several systemic side effects (sedation, weight gain, orthostatic hypotension or extrapyramidal effectsCitation1,Citation3). Such adverse events are responsible for poor patient compliance, which usually leads to non-adherence to the therapeutic scheme. Indeed, a non-adherence of about 50% is estimated for schizophrenia.Citation4 Despite the reduction of the extrapyramidal effects brought by the SGAs, the increase in therapeutic adherence to the treatment of schizophrenia remains a considerable challenge.Citation1
PP is also a poorly water-soluble drug,Citation3,Citation5 commonly used via the intramuscular way (Xeplion® or Invega Sustenna®),Citation5,Citation6 belonging to BCS class II.Citation7 Hence, it is necessary to increase its solubility and bioavailability by using formulation strategies or finding another administration way.
Nose-to-brain (N2B) delivery is a non-invasive route that aims to reach the brain by bypassing the blood-brain barrier (BBB).Citation1,Citation8–13 From the intra-neuronal pathway, the drug enters the brain via the olfactory bulb (through the olfactory nerve) or the brain stem (through the trigeminal nerve),Citation12–14 which innerves the whole nasal cavity,Citation15 by axonal transport. The extra-neuronal pathway in olfactory epitheliumCitation9,Citation10,Citation12,Citation13 is based on para- or transcellular mechanisms.Citation16 After cellular uptake, the drug reaches the subarachnoid space and reaches the brain via the cerebrospinal fluid.Citation13 The olfactory route allows a faster passage than from the trigeminalCitation1,Citation13 one. For instance, Ganger et al evaluated the duration of the transport between 1,5h to 6h and 17h to 56h through the olfactory and trigeminal nerves pathways, respectively.Citation17 Therefore, despite the relatively small area of the olfactory region (between 1.5 and 10% of the total area of the nasal cavity),Citation18 this work aims to develop N2B formulations of PP that, combined with the appropriate device, could reach significantly the olfactory region.Citation19,Citation20
An interesting new nanocarrier for PP could be the cubosomal nanoparticles (CNPs). These particles have a size range (between 10 and 500 nmCitation21,Citation22) adapted for N2B delivery. CNPs are non-lamellar liquid crystalline nanoparticles (NPs) that self-assemble by nanoprecipitation in an excess of water,Citation23,Citation24 with two production methods: the so-called Top-down and Bottom-up methods.Citation21,Citation25 The Bottom-up method has many advantages when compared with the Top-down method. This method allows working with lower energy inputs (thanks to the use of a hydrotrope) and, thus, encapsulate thermo-sensitives drugs to produce smaller and more stable NPs. Its simplicity makes it also more suitable for large scale production.Citation22–24 CNPs are composed of a bicontinuous lipid cubic phase with a single lipid bilayer that forms a continuous periodic membrane lattice structure with two interwoven water channels giving rise to the pores (5–10 nm.Citation21,Citation22,Citation25,Citation26 These NPs present several advantages like biocompatibility, bioadhesivness and biodegradability. They also have a broader lipidic internal structure than liposomes, hence a higher drug loading. CNPs can also encapsulate hydrophilic, hydrophobic and amphiphile drugs; they are easy to produce and offer enzymatic protection.Citation21,Citation24,Citation27 Finally, their fundamental constituents: amphiphilic lipid (phytantriol, monoolein (MO) or glycerylmonooleate), steric stabilizers and water compoundsCitation21,Citation22,Citation25,Citation28 are all FDA-approved for human use. The interest for CNPs intended for N2B delivery has been recently reported. Indeed, Elsenosy et al developed cubo-gels with Duloxetine intended for N2B delivery. They demonstrated a brain bioavailability 1.96 times higher for their cubo-gel than the Duloxetine solution after an intranasal administration.Citation29 Eissa et al formulated a cubosomes-based in situ gel loaded with granisetron. They reported a maximum serum concentration of drugs (Cmax) 1.90 times higher in rat brains with their cubosomal formulation (869.4 ng/mL) than with a Granisetron solution (457.2 ng/mL).Citation30
The objective of this work is the development of dry chitosan-coated cubosomes and the comparison of their efficiency for N2B delivery with standard and cationic CNPs. Indeed, the literature describes many NPs coated with chitosan (e.g PLGA NPs,Citation31,Citation32 liposomes,Citation20,Citation33 nanostructured lipid carrierCitation34) but no cubosomes. Dry formulations were used because their efficacity to reach the brain, in comparison with liquid formulations, was extensively reported.Citation19,Citation35–37
A reference formulation was inspired by Von Halling et al, which only used the MO to form the CNPs.Citation38 Their work was based on the research of Spicer et al about the formulation of dry powder precursors of liquid cubic crystalline NPs.Citation39 The second formulation was based on the addition of cholesterol (CHO) and a cationic lipid to produce positively charged CNPs. On one side, the cholesterol consolidates the lipid bilayer structure and reduces of thermal fluctuation of the CNPs.Citation26,Citation40 Moreover, the charged lipid swells the internal structure of the CNPs and increases the water-channels diameters.Citation26,Citation40 For this second formulation, 1,2-Dioleoyloxy-3-trimethylammonium propane chloride (DOTAP) was used as cationic lipid to allow a good affinity with the negatively charged mucins in the nasal mucosa due to electrostatic interactions.Citation1,Citation41 Finally, for the third formulation, 1,2-Dioleoyl-sn-glycero-3-phosphoserine (DOPS) was used as anionic lipid. To generate positively charged CNPs to provide good interaction with the mucins, the anionic CNPs were coated with chitosan to finally obtain positively charged CNPs. Moreover, as reported in the literature, chitosan is an efficient permeation enhancer for N2B delivery.Citation42–46 In particular, it can open the tight junction (TJs) by dehydrating the epithelial cells and thus increase the paracellular transport in the olfactory epithelium.Citation10,Citation45,Citation46 This is a crucial factor in increasing the absorption of drugs. Also, the chitosan has mucoadhesive proprieties and can increase the residence time of the formulation in the olfactory region.Citation10,Citation45,Citation46 A recent study by Ahmad et al demonstrated the efficacity of PLGA-NPs coated with chitosan for brain targeting. Indeed, the Cmax in the brain was 1.33 times higher for their PLGA-NPs coated with chitosan than for the drug suspension (5235.028 ± 5.00 and 3943.23 ± 5.77 ng/mL, respectively) after an intranasal administration in rats.Citation31 Thus, our work focused on developing a formulation using CNPs as an efficient carrier to encapsulate our lipophilic drug and chitosan to promote the permeation between the nasal epithelia. As described below, to cover the whole process of N2B delivery, we also used a nasal cast as a new tool for nasal in vitro assessment to map the pharmaceutic forms in the nasal cavities.Citation47
Methods
Preparation and Characterization of the Formulations
Preparation of the Cubosomal Nanoparticles Liquid Formulations
The standard CNPs (SCNPs) were prepared using the Bottom-up method. Briefly, 5.33 mg/mL of MO (Danisco A/S, Denmark) were dissolved in ethanol and dispersed in an aqueous phase at a ratio of 1:3 v/v.Citation38 Dextran (Pharmacosmos, Denmark) and Kolliphor® P407 (BASF, Germany) (4.32 mg/mL and 173 μg/mL, respectively) were previously dissolved in MilliQ water. The resulting formulation was mixed for 15 minutes by moderate magnetic stirring.
For the preparation of the cationic and chitosan-coated cubosomal nanoparticles liquid formulations, several proportions of lipid mixtures were prepared by the thin lipid film hydration method to evaluate their influence on the physicochemical properties of the resulting cubosomes. Briefly, the lipid mixtures were composed of 70:25:5, 60:30:10, 50:35:15 and 40:40:20%MOL of MO, CHO (Sigma-Aldrich, USA) and charged lipids (DOTAP or DOPS, cationic and anionic lipids, respectively) (Lipoid GmbH, Germany). The lipids were introduced into a round-bottomed opaque flask and dissolved by a mixture of organic solvents (dichloromethane:methanol 50:50 v/v). Except for the blank formulations, the PP (Biochem Partner, China) was previously dissolved in the organic solvent-lipid mixture.
The organic solvents were removed within 20 minutes using a Rotavapor R-205 (Büchi Labortechnik, Flawil, Switzerland) at 60°C to produce a thin lipid film at the internal surface of the round-bottom flask. The pressure was set at 250 mmHg for 10 minutes and 150 mmHg for the next 10 minutes.
Finally, the Bottom-up method was used to generate the CNPs. This process relies on the lipid film dissolution by ethanol and, then, by the precipitation of the cubosomes by adding of an excess of an aqueous phase. In this work, Kolliphor® P407 (10% w/w of lipid mixture) was dissolved in MilliQ water to stabilize the colloidal system.
A further coating step of the anionic CNPs with chitosan followed this procedure to produce the chitosan-coated CNPs (ChCNPs) liquid formulation, this procedure was followed by a further step of coating the anionic CNPs with chitosan low molecular weight (50–190 kDa, 75–85% deacetylated, Sigma-Aldrich, USA). A solution of 10% w/v of chitosan was prepared in 1% v/v acetic acid solution. Then, this solution was added drop by drop under vigorous magnetic stirring for 10 minutes in the previously formed anionic liquid formulation to obtain a final product with 0.1% w/v of chitosan. So, the anionic CNPs were positively charged thanks to the chitosan coating.
Preparation of the Powder Formulations Using Spray Drying
The spray drying was performed with a Mini Spray-Dryer B-290 (Büchi, Switzerland) equipped with a 0.7 mm diameter nozzle and a high-performance cyclone. The inlet temperature was 130°C; the spray gas flow was 357 L/h; the flow rate was fixed at 3 mL/min; the aspirator rate reached 100%. Ten milliliters of each liquid formulation were dried in triplicate.
After spray-drying, each powder formulation was dispersed in Milli-Q water at 1 mg/mL and briefly sonicated using a VCX 500 probe sonicator (Vibra-Cell; Sonics and Materials, Newton, CT, USA).
Then, the particle size (PS) (z-average, nm), the polydispersity index (PDI), and the zeta potential (ZP, mV) of the cubosomes were evaluated using dynamic light scattering (DLS) and electrophoretic mobility (Zetasizer® Nano ZS, Malvern Ltd., Malvern, England). Size measurements were performed with PS semi-micro disposable cuvettes (DTS0012) and zeta potential evaluation with disposable folded capillary cells (DTS1070). The temperature during the analysis was 25°C. All the experiments were repeated in triplicate and expressed as the mean ± SD (n=3). The pH of each reconstitution was determined using the pH-meter (VWR® pHenomenal® pH/mV/°C Meter, Bench, pH 1100L/1100LB).
Finally, the particle size distribution (PSD), the production yield and the percentage of the residual moisture of each powder formulation (SCNPs, CCNPs and ChCNPs) were compared without and with the use of 25% w/w of l-leucine (LL) (vegetal L-leucine, Sigma-Aldrich, USA). The PSD of the microparticles at the exit of the UDS device (Aptar Pharma, Le Vaudreuil, France) was measured via laser diffraction using a Spraytec apparatus in an open bench system with a 100 mm lens (Malvern® Instrument, Malvern, UK). The analysis parameters were: test duration of 300 ms, actuation distance of 7 cm and data acquisition rate of 2500 Hz. Size distribution was expressed in terms of first decile (Dv10), median diameter (Dv50), last decile (Dv90), and span value (experiments were done in triplicate).
The production yield (%) for the spray drying process was calculated as follows (EquationEquation 1(1) ):
where Wr is the mass of powder collected (mg) after drying, and Wi is the mass of components contained in the initial liquid formulation introduce in the spray drier (mg). The residual moisture (% w/w) of each powder formulation was evaluated by thermogravimetric analysis (TGA) using a TGA Q500 (TA Instruments, New Castle, Delaware, USA). The temperature cycle ranged between 35 and 150°C with a heat rate of 10°C/ min. The percentage of residual moisture was determined with TA Instrument Universal Analysis 2000 software by measuring the weight variation (% w/w).
Determination of Maximal Drug Loading Capacity and the Entrapment Efficiency
We added several amounts of pure PP to the SCNPs, CCNPs, and ChNPs liquid formulations to evaluate the maximal drug loading of each powder formulation. EquationEquation 2(2) gives the maximal drug loading capacity (LC) of the cubosomes (%):
where WPP is the mass of PP (mg) and WMO is the mass of MO (mg). EquationEquation 3(3) gives the encapsulation efficiency (EE) of the cubosomes/:
where WPPi is the initial mass of PP introduced in the formulation (mg) and WPPf is the mass of PP in the powder formulation after spray drying (mg).
We used an HPLC-UV method to quantify PP from the cubosomes. Five milligrams of each powder formulation were dissolved in a mixture of acetonitrile, ethanol and MilliQ water solution (45:45:10 v/v, respectively). The solutions were filtered through 0.45 μm filters (Sortorius®) and filled in 2 mL amber vials for HPLC analysis. As described by Manini et al, mobile phase A (100% v/v of acetonitrile) and mobile phase B (aqueous solution of trifluoroacetic acid at pH 2) were used at a ratio of 70/ 30 A/B (v/v). The flow rate was 1 mL/min for 20 min, and the wavelength was 278 nm. The retention time of PP was 8.0 min.Citation48 The pH of the mobile phase was evaluated by using a VWR® pHenomenal® pH/mV/°C Meter.
Powder X-Ray Diffraction
The powder formulations were evaluated by powder X-ray diffraction (PXRD) using an X-ray diffractometer (D8 Advance Eco Bruker). The measurement system was a one-dimensional silicon detector (LynxEye XE-T, Bruker) using Cu-K radiation (1.54 Å; 40 kV x 25 mA). Data were collected over the angular range of 1–40° 2θ, with a step size of 0.02°, a time step of 2 s and a variable divergence slit of 8 mm. The percentage of amorphous form was calculated as 100% minus the crystalline phase content in the powder, as determined using the surface area ratio method.
Characterization of the Morphology of the Nanoparticles
The cubosomal morphology of the optimized formulations was observed by transmission electron microscopy (TEM). 0.5 µL of a sample (1mg/mL in Milli-Q water) was placed onto the center of a commercial 3–5-layer graphene grid with a Lacey-C support (EMS). Then, a top grid allowed the droplet to expand and fill the nano-pockets while van der Waals forces joined the two grids to seal the system.
The system was placed in a single-tilt holder and introduced in a TECNAI G2 super-twin with a LaB6 filament, 30 µm CA2 aperture, operated at 200 kV. We worked in TEM mode using a Cheetah- MEDIPIX 3 direct detection camera (Amsterdam Scientific Instruments, Hollands) located in the 35 mm port of the microscope to obtain high-quality pictures using an extremely low electron flux (around 10−3 e−.Å−1.s−1). An objective aperture of 30 µm optimized the contrast.
Scanning Electron Microscope Analysis
The morphology of the microparticles was studied by scanning electron microscope (SEM) analysis using a Hitachi SU8020 ultra-high-resolution microscope (Hitachi, Tokyo, Japan). The particles were coated with gold (35 mA for 4.5 min at 1 mbar under argon) before analysis. The images were processed using ImageJ software (National Institutes of Health, USA).
Plume Angle and Ejection Velocity
To evaluate the angle and the velocity of the plume from the UDS device (Aptar Pharma, Le Vaudreuil, France), a high-speed camera was used (IDT Motion Pro Y3, Integrated Design Tools, Pasadena, CA, USA with a Nikon AF micro-Nikkor 60 mm f/2.8 D lens, Nikon, Tokyo, Japan) (n=3). The parameters were: aperture of 16, acquisition time of 100 µs, and acquisition frequency of 3,000 Hz.
Mucoaffinity Test
A stock solution (400 μg/mL) was prepared by dissolving 20.0 mg of porcine-stomach mucins (type II, Sigma-Aldrich, USA) in 50.0 mL of MilliQ water. In addition, the stock solution was diluted with MilliQ water to provide four concentrations of mucins, namely 80, 160, 240, 320, and 400 μg/mL (Sm1, Sm2, Sm3, Sm4, and Sm5, respectively). The concentration of mucins was fixed to be in the detection range found when using the previously described method based on DLS. Each liquid formulation was reconstituted by mixing forty milligrams of the corresponding powder formulation in 20 mL of MilliQ water.
The evaluation method of the interaction between the mucin and the CNPs was based on the method of Wong et al.Citation49 One milliliter of each mucin solution (Sm1, Sm2, Sm3, Sm4 and Sm5) and one milliliter of each reconstituted liquid formulations (SCNPs, CCNPs and ChCNPs) were mixed and incubated for 30 min in 37°C at 600 rpm.
The ZP of each solution was evaluated by DLS (Zetasizer® Nano ZS, Malvern Ltd., Malvern, England), along with the ΔZP to evaluate the mucoaffinity of each kind of CNPs (see EquationEquation 4)(4) .
Where ZPi is the initial ZP (mv) of the CNPs and ZPf is the ZP (mv) of the CNPs after contact with mucin solution (Sm5). Each experiment was realized in triplicate and expressed by mean ± SD.
In vitro Cellular Study
RPMI2650 Cell Culture
The RPMI 2650 cell line is a common choice for an epithelial in vitro model for nasal permeation studies.Citation50–52 Indeed, this cell line produces mucus,Citation50 expresses TJs,Citation52,Citation53 and grows in multilayer leading to appropriate transepithelial electrical resistance (TEER) values (between 75–150 Ωcm2 and 90–180 Ωcm2 for RPMI 2650 cell line and excised human nasal mucosa, respectively).Citation50,Citation52,Citation53 The RPMI 2650 cells (ECACC, Sigma-Aldrich, USA) were cultured in a 75 cm2 polystyrene cell-culture flask under standard conditions in an incubator at 37 °C and 5% v/v of CO2. The culture medium (MEM with phenol red, 10% heat-inactivated fetal bovine serum (FBS), 1% Pen/Strep solution, 1% L-glutamine and 1% NEAA, Thermo™ Fisher Scientific, USA) was changed every couple of days. The cells were detached when confluence reached about 80% by treating with trypsin-EDTA at 37°C. Then, the cells were seeded into a new 25 cm2 polystyrene cell-culture flask at a 1:10 ratio.
As explained by Gonçalves et al, the RPMI 2650 cells were seeded on permeable Thincert® (TC-PTP, 0.4 μm, 4.52 cm2, Thermo™ Fisher Scientific, USA) at a density of 1.5×105 cells/cm2. The cells were cultured in a liquid-covered culture (LCC) for 8 days. The insert culture medium (MEM without phenol red, 10% heat-inactivated fetal bovine serum (FBS), 1% Pen/Strep solution, 1% L-glutamine and 1% NEAA) was changed every 2 or 3 days. After, the insert was lifted to the air-liquid interface (ALI) and cultured for 14 more days.Citation50 As reported by Wengst et al, the TEER value is significantly higher for the RPMI 2650 cells in ALI conditions compared to LCC conditions.Citation52 Their results, coupled with light microscopy analysis, demonstrate that, in LCC conditions, the cells grow in clusters with free spaces between them.Citation52 On the other hand, the ALI conditions increased the aerobic exposition, which correlates with epithelial barrier formation.Citation53
The TEER was recorded using an epithelial Volt/Ohm meter EVOM2® (World Precision Instruments, Sarasota, USA). The value of the blank insert was subtracted from the raw data and normalized for the surface area (4.52 cm2). For TEER routine evaluations, 2 mL of fresh insert culture medium were added to the apical side and 3 mL to the basal side. Cells were left for 30 min before measurements. A TEER value higher than 75 Ωcm2 (mimicking the TEER of the human nasal mucosaCitation52) was targeted to start the permeation study.
Alcian Blue Assay
The secretion of mucus on the surface of the mono/multilayer of RPMI 2650 cells was observed using Alcian blue (Thermo™ Fisher Scientific, USA) dye as described by Gonçalves et al.Citation50
Images were acquired with an Olympus BX60 microscope (Olympus, Hamburg, Germany), equipped with a JVC tk-c1381 color video camera and an Olympus U-CMAD-2 C-Mount Adapter Ring, supported by the analySIS®docu image-processing software (Olympus).
The images were analyzed by ImageJ (V2.1.0, NIH) with the Color Inspector 3D V2.5 (Kai Uwe Barthel; Internationale MedieiInformatik, Berlin, Germany) plugin. To this end, each image was first transformed into 8-bit RGB. The average value over the whole picture of the blue component was then divided by the sum of the three channels (blue, red, and green). The resulting ratio allowed us to quantify the mucus produced by the cells.
Permeation Study
The study started after 22 days of culture to get the desired TEER value. A Krebs-Ringer buffer (KRB) was prepared to perform this permeation study. First, the cells were rinsed three times with KRB, and 1.0 mL was added to the apical side and 2.0 mL to the basolateral side. The plates were kept in an incubator (37°C, 5% CO2) for 30 min. After, around 3 milligrams of each powder (pure PP, SCNPs, CCNPs, and ChCNPs) were deposed on the apical side. Each powder was introduced into three different inserts to perform the test in triplicate and diffused for three hours. The apparent permeability coefficient (Papp) was calculated for each powder formulation by the following equation and expressed in cm/sCitation50,Citation54 (EquationEquation 5(5) ):
where (µg/mL) is the concentration of PP in the basolateral compartment at the end of the experiment,
(µg/mL) is the initial apical concentration of PP, V (mL) is the volume of the basolateral compartment, A is the surface area (cm2) and
is the duration of the experiment (s).
The Transport Enhancement Ratio (TER) of the formulation compared to the pure PP was calculated from the Papp values (EquationEquation 66 ):
where Papp(formulation) corresponds to the Papp of SCNPs, CCNPS, and ChCNPs formulations and where Papp(PP) corresponds to the Papp of PP.
The TEER was measured again 24 hours after the experiment to evaluate the integrity of the epithelium cells.
LDH Cytotoxicity Assay
An LDH assay was also carried out with the Cayman chemical kit (Item no 601,170) to measure the cytotoxicity of the three powder formulations. Cells were placed in a 96-well plate with a density of 50,000 cells/mL and allowed to settle for one hour. The cells were then treated with the formulations and analyzed following the kit manufacturer’s instructions. The absorbance at 490 nm (A490) was measured and the percentage of cytotoxicity was expressed by the following equation:
Deposition Study in a Nasal Cast
Nasal Cast Conception
We used the 3D-printed nasal replica (called “nasal cast”) described by Rigaut et al to assess the deposition profile of our formulations.Citation47 The selected patient has normal anatomy without any anatomical disorder. The nasal cast is a new in vitro test to evaluate drug deposition in a nasal cavity. The human nasal cavity is composed of tortuous and complex structures and varies between patients (e.g. sex, ethnicity, age or disease state). Thus, evaluating the olfactory targeting in vitro represents a pivotal element before the expensive in vivo test. Indeed, although nasal casts are not yet a regulatory test, a recent study by Williams et al validated this model as a suitable in vitro tool to assess in vivo deposition of a nasal product.Citation55
Briefly, from the CT scan of a patient, the nasal cavities were separated from the body via the grey value: darker pixels are air, and lighter pixels are parts of the body. It resulted in a series of black-and-white images that could be assembled and smoothed to re-create the 3D model of the nasal cavity. Then, this model was segmented into five pieces to evaluate the spatial distribution of the spray in the cavity. The nasal cast was printed with a Form3 printer (Formlabs, Somerville, MA, USA) using Formlabs Clear resin, except for the nostrils, which were printed with Formlabs Flexible 80A resin to allow a better insertion of the devices. After printing, each part of the nasal cast was washed with isopropanol and UV-cured according to Formlabs guidelines.
Deposition Test
Each part of the nasal cast was coated with an artificial mucus based on a thermosensitive gel (liquid under 10°C and swells around 18°C) composed of 25% w/w of Poloxamer® 407 mixed into a simulated nasal electrolyte solution (SNES).Citation47,Citation56 We introduced an exact amount of around 20 mg of powder into the device since the maximal dose for nasal delivery is 25 mg per nostril.Citation37,Citation57 A previous study allowed us to determine the optimal parameters to target the olfactory zone.Citation47 The insertion angle was fixed with a direct aim of the olfactory zone (sagittal angle of 39° on the right and 29° on the left, and coronal angle of 20° on the right and 5° on the left, both pointing inwards). There was no concomitant inspiratory flow, and the powder was injected with a UDS device (Aptar Pharma, Le Vaudreuil, France). The device was weighed before and after the injection to evaluate the administered dose. After instillation, we washed each part of the nasal cast with a mixture of acetonitrile, ethanol, and MilliQ water (45:45:10% v/v). Each recovery solvent was quantified by an HPLC-UV method to determine the percentage of powder in each part. Here, since the target is N2B delivery, the analysis was focused on the olfactory deposition (i.e. the deposition in the uppermost piece of the middle region).
Statistical Analysis
We used the one-way ANOVA test was used to demonstrate the significance of each factor for the comparison of CNPs with or without LL, the TER and the Papp comparison in the permeation study and for the comparison in the deposition test with the left and the right side. Student’s t-test was used to study the difference in the mucoaffinity test. All statistical tests were performed by using GraphPad (Prism 9, San Diego, USA) and the difference between means was considered significant with a p-value lower than 0.05.
Results
Characterization of the Formulations
Spray-Dried Powder Properties
We compared the characteristics of the positively charged cubosomes (cationic CNPs (CCNPs) and ChCNPs) intended for N2B delivery to those of SCNPs that are created without CHO or a charged lipid to evaluate the potential efficacity.
The first step for the two charged blank CNPs (CCNPs and anionic CNPs) was to select the correct proportion of lipids to obtain stable and compatible NPs for N2B delivery. Indeed, it is necessary to formulate anionic CNPs as an intermediate step to coat them afterwards with chitosan by electrostatic interaction to obtain the ChCNPs. All test lipid mixtures gave NPs with a PS and a ZP suitable for N2 delivery. But only the mixture of 60:30:10%mol of MO, CHO, and an ionized lipid leads to a narrow PSD (i.e. PDI value lower than 0.3).
As mentioned previously, for N2B delivery, a positive surface charge of NPs increases their interaction and their affinity with the negatively-charged mucins of the nasal mucosa.Citation1,Citation10 So, the selected anionic CNPs were coated with chitosan to obtain a final positive charged CNPs called ChCNPs. The summary of the selected blank CNPs with their characteristics is shown in .
Table 1 Particles Size (PS) (Nm), Polydispersity Index (PDI) Value and Zetapotential (ZP) (mV) of Selected Blank Standard Cubosomal Nanoparticles (SCNPS), Cationic Cubosomal Nanoparticles (CCNPS), Anionic Cubosomal Nanoparticle (Anionic CNPS), and Chitosan-Coated Cubosomal Nanoparticles (ChCNPS). Results are Expressed by Mean ± SD
The two positively charged CNPs were characterized by a positive ZP (+60.1 ± 1.2 and +34.4 ± 1.2 mV for CCNPs and ChCNPs respectively) while the SCNPs were characterized by a negative ZP. Since a ZP higher than +20 mV or lower than −20mV is known to be favorable for long-term stability,Citation10 the two positively charged CNPs are more stable than the SCNPs.
NPs with a PS lower than 200 nm can diffuse through olfactory neurons.Citation42,Citation58,Citation59 All blank CNPs were characterized by a particle size lower than 200 nm except for the ChCNPs (see ), which had a PS of 249.8 ± 15.9 nm. However, the benefits of chitosan as a permeation enhancer should offset this slight deviation in size.Citation10,Citation60,Citation61 Indeed, Raj et al demonstrated a higher dopamine content in the brain after an intranasal administration of Pramipexole loaded in chitosan NPs (despite a PS of 292 ± 8.80 nm) versus a solution of the same drug (97.38 ± 3.91 ng/g tissue and 81.61 ± 4.44 ng/g tissue respectively).Citation62 Finally, in our study, all blank CNPs demonstrated a narrow particle size distribution which a PDI value lower than 0.3,Citation63 except the SCNPs with a slightly higher value of 0.353 ± 0.014, which should not have any consequence on their suitability.
During the spray drying process, a sticky powder in the cyclone was observed. Indeed, a high residual moisture content promotes the formation of liquid bridges between particles which solidify after drying to generate agglomerates.Citation64–67 Moreover, a high residual moisture content reduces the glass transition temperature (Tg). Therefore, when the experimental temperature is higher than the Tg of the material, it becomes sticky (rubbery state) and adheres to the cyclone.Citation65,Citation66 Adding LL in a range between 10–20% w/w increases the yield of production and the flowability of the particles, and reduces the residual moisture and the caking effect.Citation64,Citation68–71 As LL is a hydrophobic amino acid, it will dry faster than the other formulation compounds, which could create a shell around the drug.Citation65 Indeed, droplet drying is characterized by two distinct steps: the constant-rate period and the falling-rate period. The materials characterized by a high Peclet number, such as LL, tend to concentrate onto the droplet surface as evaporation is faster than their diffusion.Citation65 Preliminary studies were performed to determinate the optimal LL content to improve the powder PSD (data not shown), to increase the spray-drying yield and to decrease the residual moisture content of the dry powders. To evaluate the influence of LL, its percentage was fixed at 25% w/w in each formulation ().
Table 2 Size Distribution Was Expressed in Terms of First Decile (Dv10), Median Diameter (Dv50), Last Decile (Dv90), and Span Value. The Yield (%) and Residual Moisture Content (%) Were Expressed for Standard Cubosomal Nanoparticles (SCNPs), Cationic Cubosomal Nanoparticles (CCNPs), and Chitosan-Coated Cubosomal Nanoparticles (ChCNPs) Blank Formulation with or Without l-Leucine (LL). Results are Expressed by Mean ± SD
As shown in , the yield was similar, regardless of the presence of LL (higher than 70% w/w). Although, in the absence of LL, the residual moisture content was higher than 2% w/w, while the addition of the amino acid allowed us to obtain a lower percentage. Therefore, the yield was slightly overestimated for the powders that were not based on LL.
These results illustrated the anti-hygroscopic effect of LLCitation64,Citation66,Citation67,Citation69,Citation72 and could explain the decrease of powder agglomerates. Indeed, the LL increased the moisture resistance of the spray-dried particles by its surface enrichment. This, in turn, reduced the thickness of the liquid on the surface of the particles and reduced the liquid bridges between them.Citation67,Citation73 It allowed both improving the deagglomeration and the flowability of the powder.
The deagglomeration effect is illustrated by the Dv50 value of each powder formulation measured at the exit of the UDS device. Indeed, all powder formulations containing LL were characterized by a Dv50 smaller than the powder without LL (14.49 ± 0.63 μm, 14.34 ± 0.82 μm, and 12.65 ± 0.37 μm for SCNPs-LL, CCNPs-LL, and ChCNPs-LL, respectively and 78.42 ± 14.61 μm, 61.02 ± 9.30 μm and 43.76 ± 12.04 μm for SCNPs, CCNPs and ChCNPs, respectively).
The Dv50 of our LL-containing powders ranged between 13 and 20 µm. Such diameters are known to promote olfactory zone impaction and thus the passage to the brain.Citation74–76
Determination of the Maximal Drug Loading Capacity and the Encapsulation Efficiency
We studied the maximal LC (EquationEquation 2(2) ) of each CNPs and checked their EE (EquationEquation 3
(3) ) by introducing several quantities of PP (fixed percentage shown in ) and analyzing the effect on the PSD of the CNPs. The maximal LC selected is 10%, 5%, and 70% for the SCNPs, CCNPs, and ChCNPs, respectively, because these LCs gave the lowest PDI value for each CNPs formulation and thus, guaranteed a narrow PSD with a PS suitable for the N2B delivery (). The CCNPs and SCNPs formulations provided the lowest LC, 5% and 10% w/w, respectively, while the LC of the ChCNPs formulation reached 70% w/w.
Table 3 Loading Capacity (LC) (%), Particles Size (PS) (Nm), Polydispersity Index (PDI), pH, and Encapsulation Efficiency (EE) (%) of Each Selected Standard Cubosomal Nanoparticles (SCNPS), Cationic Cubosomal Nanoparticles (CCNPS), and Chitosan-Coated Cubosomal Nanoparticles (ChCNPS). And Zeta-Potential (ZP) (mV) of Each Selected CNPs Formulation. Results are Expressed by Mean ± SD
The PP is a strongly basic drug (pKa1 8.2 and pKa2 2.6),Citation77 and the pH of liquid pre-formulations was around 4.5 for each formulation, leading to positively-charged PP. So, electrostatic repulsion between the positively charged PP and the positively charged lipid during the production process could explain the lowest LC obtained for the CCNPs. In contrast, there was an attraction between the drug and the negatively-charged CNPs during the production process (SCNPs and ChCNPs before chitosan coating). For SCNPs and ChCNPs, the difference between the LC (10% and 70% w/w, respectively) could be explained by the cubosomal structure of ChCNPs: CHO stiffens the bilayer, and the anionic lipid (DOPS) electrostatically swells the structure, which allowed increasing the LC.Citation40,Citation78,Citation79
Finally, the EE of each selected formulation is higher than 95% (98.3 ± 1.8%, 98.0 ± 0.4% and 99.7 ± 0.1% for SCNPs, CCNPs, and ChCNPs, respectively) and they are stable, given their ZP value (), which should be ideally either above +20 mV or below −20 mV.Citation10
Powder X-Ray Diffraction
To evaluate the crystallinity and the stability of our powder formulations, the PXRD profiles of pure PP, dextran and CNPs were studied (). The CNPs formulations were mostly amorphous () due to the presence of dextran ().
Figure 1 (A) XRPD profile of the three powder formulations: standard cubosomal nanoparticles (SCNPs), cationic cubosomal nanoparticles (CCNPs), and chitosan-coated cubosomal nanoparticles (ChCNPs), the vertical red lines correspond to the peaks of chitosan extracted to ICDD databaseCitation81; (B) XRPD profile of pure paliperidone palmitate (PP), l-leucine, monoolein and, dextran. The percentage of amorphous form was indicated on the right of the curve.
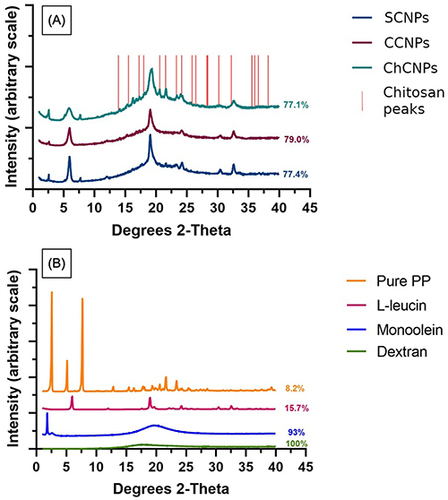
In each powder formulation, the peaks of LL are at 6° and 19° 2θ Citation80 and 30° and 32.5° 2θ. The main peaks of crystallinity of PP at 2° and 7.5° 2θ were observed for the SCNPs and ChCNPs powders. For the CCNPs powder, only the typical peak at 2° 2θ was observed. It could be the consequence of the low concentration of PP in the CCNPs powder formulation.
In , the PXRD profile of chitosan extracted to the ICDD databaseCitation81 (vertical red lines) suggested that the two peaks at 20.3° and 21.4° 2θ for the ChCNPs formulation correspond to the chitosan coating. Indeed, these two peaks are missing in the two other formulations which did not contain chitosan.
Characterization of the Morphology of the Cubosomes
The morphology of each CNPs was performed with TEM analysis. The SCNPs were nearly spherical with polyangular irregular shapes (). In previous studies, Elsenosy et al, as well as Nasr et al, already observed that loaded cubosomes usually lose their cubic shape.Citation29,Citation82 In contrast, although they were also loaded with PP, the CCNPs and ChCNPs preserved their cubic structure ( and ). The addition of cholesterol in both formulations may explain this observation. Indeed, before the TEM analysis, the powder was reconstituted by sonication in Milli Q water. This process is known to generate heat stress that may change the cubosomal shape. We may assume that cholesterol reduced thermal fluctuation and consolidated the lipid bilayer structure responsible for the cubosomal structure.Citation26,Citation40
Figure 2 TEM image of (A) standard cubosomal nanoparticles (SCNPs), (B) cationic cubosomal nanoparticles (CCNPs), and (C) chitosan-coated cubosomal nanoparticles (ChCNPs). It corresponds to the final product loading with paliperidone palmitate.
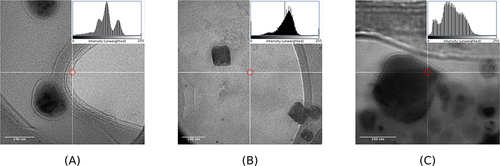
Surprisingly, the mean diameter observed by TEM was lower than by DLS. Eissa et alCitation30 and Nasr et alCitation82 made similar observations. In contrast to the DLS measurements which were performed after the dispersion of the powder in water, TEM analysis was done in a vacuum on the dry powders themselves. Such difference may explain our observations.
Scanning Electron Microscope Analysis
The SEM showed that using the LL as an excipient for spray drying process induces corrugated particlesCitation65,Citation83 (see ). Indeed, LL has a high Péclet number which causes a surface enrichment during the spray drying process, which results in these corrugated particles.Citation65 By creating a shell around the dried particles, LL reduced the water vapor escaping during the spray drying process, inducing an increase vapor pressure. At the end of the process, the vapor pressure was decreased and yielded corrugated particles.Citation73
Figure 3 SEM images of corrugated microparticles. (A) standard cubosomal nanoparticles powder formulation with l-leucin, (B) cationic cubosomal nanoparticles powder formulation with l-leucin, and (C) chitosan-coated cubosomal nanoparticles powder formulation with l-leucin.
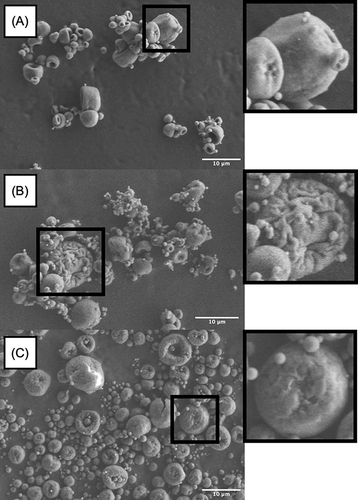
For dry powder inhalers (DPIs), a high deposition performance seems to correlate with the corrugated surface of the powder. It is principally due to the reduction of agglomeration and the reduction of the interparticular forces.Citation65
Plume Angle and Ejection Velocity
All powder formulations were characterized by a plume angle lower than 30° when instilled from the UDS (20.13 ± 2.65°, 18.87 ± 1.66°, and 17.73 ± 0.65° for the SCNPs, CCNPs, and ChCNPs, respectively). These values follow those previously observed using similar UDS and caffeine as a drug model (16.8° ± 2.4°).Citation47 A narrow plume angle seems to decrease the impaction in the nasal valve, increasing the postnasal-valve deposition.Citation84–86 For powder formulation, the plume angle is only affected by the administration device.Citation47 Indeed, the main factors influencing the plume of liquid sprays (viscosity, surface tension, and actuation force)Citation87 are fixed for dry sprays since the propellant gas is always the same (air in our case), and the actuation force set by the design of the device.
The ejection velocity of each powder formulation (49.53 ± 7.58 m/s, 42.40 ± 2.31 m/s, and 47.28 ± 4.33 m/s for the SCNPs, CCNPs and ChCNPs, respectively) was at least twice higher than the one observed with a liquid formulation (around 15 m/sCitation88). A high ejection velocity reduces the influence of air friction on the microparticles.Citation47 Therefore, for a given patient and a given administration side, the particle trajectories are only influenced by the device orientation.Citation47
Mucoaffinity Test
In addition to proteins such as albumin or immunoglobulins, the nasal mucus contains 95% water, less than 1% lipids, 2% mucin, and 1% of other salts.Citation17,Citation58,Citation89 Due to sialic and sulfonic acids, mucins are responsible for negative charges in the nasal mucosa.Citation17,Citation90–92 The use of positively charged NPs increases the affinity, the residence time in the nasal mucosa and the bioavailability of the drug.Citation10
As mentioned previously, the ZP of each formulation upon contact with solutions of mucin at different concentrations was evaluated. A sharp decrease of the ZP from 14.2 ± 0.3 to −18.8 ± 4.6 mV was observed when the SCNPs were placed upon contact with Sm1, in comparison with the initial solution. Then, the ZP becomes independent of the mucin concentration and equal to the ZP of the mucin solution (see ). Such results confirmed that there was no electrostatic interaction between the SCNPs and the mucins. That seemed to be correlated with the poor positive charge of these NPs. As illustrated in , the ZP of the CCNPs and the ChCNPs decreased with an increase in the mucin concentration. Moreover, the ΔZP was twice higher for the CCNPs than the ChCNPs ().
Figure 4 (A) Influence of the mucin concentration on the zetapotential (ZP) of standard cubosomal nanoparticles (SCNPs), cationic cubosomal nanoparticles (CCNPs), and chitosan-coated cubosomal nanoparticles (ChCNPs); (B) Comparison of the +ΔZP of the CCNPs and ChCNPs. The results are expressed by mean ± SD. A statistical Student’s t-test concluded a significant difference between the ΔZP of the two formulations ***p-value < 0.001). +ΔZP corresponds to the difference between the initial ZP (mv) of the cubosomal nanoparticles (ZPi) and the ZP of the cubosomal nanoparticles after contact with mucin solution (ZPf). ΔZP = ZPi - ZPf.
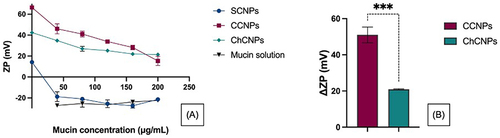
As previously described, the reconstituted liquid formulation had a pH of 4.574, 4.463 and 4.475 for SCNPs, CCNPs and ChCNPs, respectively. At this pH, the chitosan was positively charged due to the protonation of the amino group which generated an electrostatic interaction with the sulphate and the carboxyl group of the mucin.Citation90,Citation93,Citation94 The strong mucin electrostatic interaction with the CCNPs could easily be explained by the highly positively charged surface due to the incorporation of the cationic lipid. The molar concentration of DOTAP was higher than the one of the chitosan (6.2E-2 mole/g and 7.0E-4 mole/g of formulation for CCNPs and ChCNPs, respectively). Thus, there were more amino groups to interact with the mucins. This observation of increasing interaction with increasing amino groups is also supported by Mazzarino et al. In a recent study, they correlated a rise in the electrostatic interaction between the mucins and chitosan with the increase in the molar mass of the chitosan.Citation94
In vitro Cellular Study
Permeation Study
In our study, the main aim was to compare the mucoaffinity between SCNPs and cationic formulations (CCNPs and ChCNPs) and the ability of the ChCNPs to open the TJs. Indeed, the human nasal mucosa is characterized by goblet cells responsible for mucus secretion and by ciliated epithelium with TJs.Citation50,Citation52,Citation95,Citation96 Therefore, the RPMI 2650 cells were cultured in ALI condition from day 8 to day 22 to reach a TEER above 75 Ωcm2 in order to mimic the TEER of nasal mucosa as mainly reported.Citation50,Citation52
As previously described by Gonçalves et al, the Alcian blue assay was used to characterize mucin production by RPMI 2650 cells.Citation50 An increase in the RGBb ratio was observed after the 11th day (0.3290 ± 0.007 and 0.4002 ± 0.020 for the day 6 and the day 11, respectively) (). It correlated with the beginning of the culture in ALI condition (on the 8th day) and illustrated the start of mucin production. When the cells started to produce mucus, the drug permeation study was performed as soon as the TEER value was higher than 75 Ωcm.Citation2
Figure 5 +RGBb as a function of the culture duration (mean ± SD, n=3). LCC = liquid-covered culture and ALI = air-liquid interface cultivation. +RGBb (Red-Green-Blue) ratio corresponding to the average value over the whole picture of the blue component was then divided by the sum of the three channels.
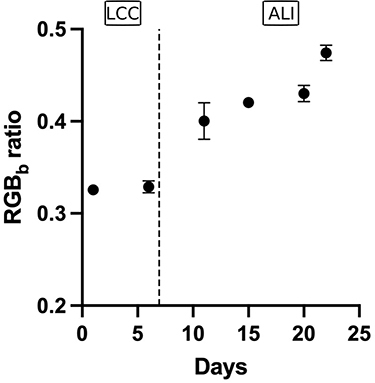
All the CNPs formulations were characterized by a significative higher Papp than pure PP, except for the SCNPs formulation (). Such data confirmed the interest in improving the mucoaffinity of the cubosomes with a cationic lipid (CCNPs) or adding a permeation enhancer such as chitosan to open the TJs (ChCNPs). The Papp coefficient was increased from 2.57E-08 ± 3.025E-08 cm/s for the pure PP to 2.23E-06 ± 0.46E-06 cm/s for the SCNPs, to 1.72E-05 ± 0.21E-05 cm/s for the CCNPs and finally to 3.00E-05 ± 0.24E-05 cm/s for the ChCNPs. The ChCNPs were characterized by a significantly higher TER (86.86 ± 17.97, 671.25 ± 79.82 and 1168.38 ± 93.90 for SCNPs, CCNPs and ChCNPs, respectively) due to their mucoaffinity and ability to open the TJs ().
Figure 6 (A) Apparent permeability coefficient through the RPMI 2650 cell line of standard cubosomal nanoparticles (SCNPs), cationic cubosomal nanoparticles (CCNPs), chitosan-coated cubosomal nanoparticles (ChCNPs), and of the pure paliperidone palmitate (PP). The y-axis is expressed in log 10. Each comparison has a p-value < 0.0001 ****Except between pure PP and SCNPs (no significant). (B) The Transport Enhancement Ratio (TER) of each formulation compared to the pure PP. ****p-value < 0.0001 and ***p-value < 0.001. The results are expressed by mean ± SD and are realized in triplicate. The comparison was realized by a one-way ANOVA statistical test.
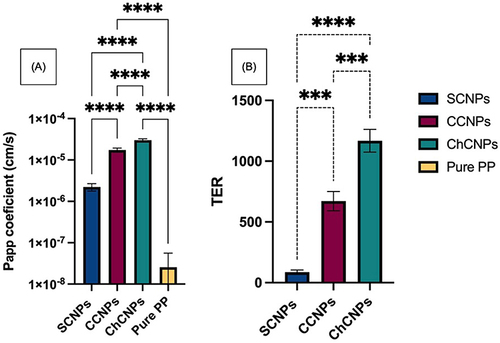
Several results are highlighted in the literature. First, it is well-known that the cationic NPs have a higher cellular uptake than the negatively charged NPs in commonly used cell lines.Citation97–100 However, in ex vivo studies, the results are more nuanced. Recent research conducted by Clementino et al demonstrated a higher initial (after 1 hour) sustained transport of their drug with their chitosan-coated NPs (their only positively charged formulation) across excised nasal mucosa of rabbits (3-fold and 18-fold lower for their two other negatively charged NPs).Citation100 Indeed, their chitosan-coated NPs are drug vehicles that firmly anchor in the nasal mucosa. It improves drug absorption by lower colloidal stability upon mucus contact and by the specific biodegradation carried out by enzymes.Citation100
On the other hand, another recent study compared the permeation of negatively and positively charged NPs through the olfactory mucosa.Citation101 Albarki et al concluded in better permeation with the negatively charged NPs.Citation101 They explained such results as their study was performed for one hour, although other studies were conducted for a longer period of time.Citation101 The work of Albarki et al contradicts Clementino et. al, but they only studied the effect of the charge while Clementino et al added chitosan, a permeation enhancer.Citation100 Moreover, the difference between the work of Albarki et al and previous studies could be due not only to the timescale of the experiments but also to the difference between cancerous and normal cells.Citation31
LDH Cytotoxicity Assay
The TEER value was unchanged before and 24h after the beginning of the permeation test. It confirmed the integrity of the cell barrier. In addition, the LDH cytotoxicity assay demonstrated the safety of the formulations except for the CCNPs. Indeed, we observed an increase in the percentage of cytotoxicity correlated with the positive surface charge (18.4 ± 1.3, 53.7 ± 3.2, and 32.2 ± 0.8% of cytotoxicity for SCNPs, CCNPs and ChCNPs respectively). However, the literature mainly described the cytotoxicity effect of the positively charged NPs in tumoral cells.Citation31,Citation102,Citation103 We observed a decrease in the percentage of cytotoxicity between the CCNPs and ChCNPs. It is probably due to the reduction of the positive surface charge. On the other hand, chitosan is extensively studied, and its cytotoxicity effect is negligible in normal cells.Citation31,Citation103 For instance, a recent in vivo safety study by Abas et al concluded no significant irritation either for the placebo or for the drug-loaded chitosan formulations.Citation33
Deposition Study in a Nasal Cast
We compared the percentage of olfactory deposition in the right and in the left cavities of the nasal cast for each CNPs dry formulation (). Coherently with our previous study on the same cast,Citation47 the percentage of olfactory deposition in the right side (52.91 ± 8.85%) was always larger than in the left side (35.80 ± 8.46%). The size of the olfactory region (11.4 cm2 and 10.5 cm2 for the right and the left side, respectivelyCitation47) could explain this difference. However, an ear, nose and throat (ENT) specialist of Erasme Hospital identified the CT scan of our patient as a non-pathologic nasal cavity; a perfect symmetry of the nasal cavity being inexistent in the population. Interestingly, also shows that the deposition in the olfactory region was statistically similar regardless of the CNPs powder. Therefore, it seemed that the anatomy of the nasal cavity had the most influence on the deposition than the physical properties of the powder with this combination system (UDS device with our powder formulation). Indeed, all formulations had a similar Dv50, plume angle, and ejection velocity. As previously described, the plume angle and the ejection velocity depend only on the device. The UDS device generates a narrow plume angle with a high ejection velocity and a direct aim at the olfactory region.
Figure 7 Percentage of the powder deposited on the olfactory region for the standard cubosomal nanoparticles (SCNPs), cationic cubosomal nanoparticles (CCNPs), and chitosan-coated cubosomal nanoparticles (ChCNPs) formulations in the left side and in the right side. Results are expressed by mean ± SD.
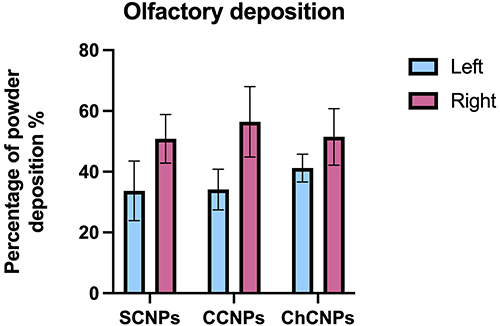
gives information on the general profile of deposition on both sides. A one-way ANOVA test concluded a significant difference between the left and the right side of the nose, the olfactory region, and the middle turbinates (p-value = 0.0106, < 0.0001, and <0.0001 respectively).
Figure 8 Percentage of powder deposition in (A) nostril, (B) olfactory, (C) middle turbinates, (D) lower turbinates, (E) nasopharynx, and (F) post-nasal fraction in left and right side for combined formulations. Results are expressed by mean ± SD. A one-way ANOVA statistical test was realized to compare the powder deposition in the right and in left side in each part of the nasal cast. *Indicate a p-value <0.05 and ****Indicate a p-value < 0.0001.
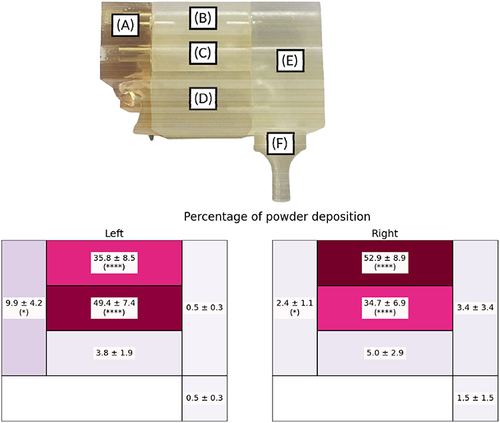
A higher deposition in the middle turbinates part than in the olfactory region was observed in the left nostril (49.4 ± 7.36 and 35.8 ± 8.46% for the middle turbinates region and the olfactory region respectively). Another interesting observation was the low deposition in the nasopharynx and post-nasal parts.
This deposition profile seemed to be favorable for brain targeting. Indeed, besides the olfactory nerves, another pathway to reach the brain after nasal instillation is through the trigeminal nerve which innerves the whole nasal cavity, except the post-nasal fraction.Citation15
Finally, our results correlated with the results of Williams et al, which validated their in vitro deposition with an in vivo study. Indeed, in their work, the majority of the powder was also found in the middle turbinates and the olfactory region in the nasal cast (about 40 and 35% for the middle turbinates and the olfactory region, respectively) and in the in vivo study (about 35 and 38% for the turbinates and the olfactory region, respectively).Citation55
Conclusion
The existing literature vastly underlines the potential of CNPs for hydrophobic drug administration and the advantageous nasal deposition of dry powder sprays compared to liquid sprays. So, our work focused on a dry cubosome formulation of PP intended for N2B delivery. To this end, we successfully developed three: one following the usual cubosome preparation method, and two innovative ones, incorporating cationic lipid or chitosan. After their comparison, we selected the ChCNPs. Indeed, the properties of these CNPs are adapted for N2B delivery in terms of PS, PDI and ZP. Finally, their Papp thought the RPMI 2650 cell line was 3.00E-05 ± 0.24E-05 cm/s. Since the pure PP has a Papp of 2.57E-08 ± 3.02E-08 cm/s, the formulation enables a 1000-fold increase in cell-diffusion efficiency. Furthermore, the powder deposition study in a 3D-printing nasal cast revealed that the powder mainly deposited in the olfactory region (51.47 ± 9.30% of olfactory deposition in the right side and 41.20 ± 4.59% of olfactory deposition in the left side, respectively). This good result arises from the combination of an appropriate Dv50 and an adapted administration device intended for N2B delivery via the production of a narrow plume angle and a high ejection velocity. These results in the nasal cast are encouraging, given the promising in vivo – in vitro correlation obtained by Williams et al.Citation55 However, an in vivo study remains necessary considering the discrepancies between cell lines and ex vivo permeation studies but also to determine if nasal irritation is low enough given the in vitro cytotoxicity results. Such studies will be the subject of a future paper. But, despite the absence of an in vivo study, the CNPs seem to be a great choice of nanocarrier for nasal administration. In the addition, the use of nasal cast as a new tool for in vitro evaluation for N2B formulation could be beneficial to make progress in nasal powder formulation studies.
Abbreviations
API, active pharmaceutical ingredient; ALI, air-liquid interface cultivation; BBB, blood-brain-barrier; CHO, cholesterol; CCNPs, cationic cubosomal nanoparticles; ChCNPs, chitosan-coated cubosomal nanoparticles; CNPs, cubosomal nanoparticles; DLS, dynamic light scattering; DPIs, drug powder inhalers; EE, encapsulation efficiency; ENT, the ear, nose and throat FBS, fetal bovine serum; KRB, Krebs-ringer buffer; LC, loading capacity; LCC, liquid-covered cultivation; LDH, lactate dehydrogenase; LL, l-leucin; MO, monoolein; N2B, nose-to-brain; NPs, nanoparticles; Papp, apparent permeation coefficient; PDI, polydispersity index; PP, paliperidone palmitate; PS, particles size; PSD, particle size distribution; PXRD, powder X-ray diffraction; SCNPs, standard cubosomal nanoparticles; SD, standard deviation; SEM, scanning electron microscopy; SGAs, second generation antipsychotic drugs; SNES, simulated nasal electrolyte solution; Tg, glass transition; TGA, thermogravimetric analysis; TEM, transmission electron microscopy; TER, transport enhancement ratio; TEER, transepithelial electrical resistance; TJs, thigh junction; UDS, Uni-dose system; ZP, zeta potential.
Ethics Statement
The studies involving human participants were reviewed and approved by the Ethics Committee of Erasme Hospital – ULB (P2022/075). The Ethics Committee waived the requirement of written informed consent for participation.
Disclosure
The authors declare that this study received equipment from AptarGroup. The equipment providers were not involved in the study design, collection, analysis, interpretation of data, the writing of this article or the decision to submit it for publication. The authors report no other conflicts of interest in this work.
Acknowledgments
The authors thank Tiriana Segato (4Mat, ULB, Brussels, Belgium) for her help during the PXRD investigation. The authors also thank Yoann Paint (MateriaNova, Mons, Belgium) for the SEM analysis. The authors thank Marc Vander Ghinst (Hôpital de Bruxelles (HUB), CUB Hôpital Erasme, department of Ear, Nose and Throat, and Cervico-facial surgery, Brussels, Belgium) for his expertise in nasal cavities.
AptarGroup (Aptar, Le Vaudreuil, France) kindly provided the unidirectional UDS devices.
This research was supported by the equipment of the Micro-mili platform.
References
- Tan MSA, Parekh HS, Pandey P, Siskind DJ, Falconer JR. Nose-to-brain delivery of antipsychotics using nanotechnology: a review. Expert Opin Drug Deliv. 2020;17(6):839–853. doi:10.1080/17425247.2020.1762563
- McCutcheon RA, Reis Marques T, Howes OD. Schizophrenia - an overview. JAMA Psychiatry. 2020;77(2):201–210. doi:10.1001/jamapsychiatry.2019.3360
- Citrome L. Paliperidone palmitate - review of the efficacy, safety and cost of a new second-generation depot antipsychotic medication. Int J Clin Pract. 2009;64(2):216–239. doi:10.1111/j.1742-1241.2009.02240.x
- Acosta FJ. Medication adherence in schizophrenia. World J Psychiatry. 2012;2(5):74. doi:10.5498/wjp.v2.i5.74
- Chue P, Chue J. A review of paliperidone palmitate. Expert Rev Neurother. 2012;12(12):1383–1397. doi:10.1586/ern.12.137
- Zingale E, Bonaccorso A, Carbone C, Musumeci T, Pignatello R. Drug nanocrystals: focus on brain delivery from therapeutic to diagnostic applications. Pharmaceutics. 2022;14(4):691. doi:10.3390/pharmaceutics14040691
- Maniyar AJ, Patel GM, Shelat PK, Lalwani AN. Development and characterization of thermosensitive intranasal gel containing paliperidone loaded microspheres. J Young Pharm. 2016;8(4):368–377. doi:10.5530/jyp.2016.4.12
- Emad NA, Ahmed B, Alhalmi A, Alzobaidi N, Al-Kubati SS. Recent progress in nanocarriers for direct nose to brain drug delivery. J Drug Deliv Sci Technol. 2021;64:102642. doi:10.1016/j.jddst.2021.102642
- Hussein NR, Omer HK, Elhissi AMA, Ahmed W. Advances in nasal drug delivery systems. In: Waqar Ahmed DA, Phoenix MJ editors. Advances in Medical and Surgical Engineering. Elsevier; 2020:279–311. doi:10.1016/B978-0-12-819712-7.00015-2
- Deruyver L, Rigaut C, Lambert P, Haut B, Goole J. The importance of pre-formulation studies and of 3D-printed nasal casts in the success of a pharmaceutical product intended for nose-to-brain delivery. Adv Drug Deliv Rev. 2021;113826. doi:10.1016/j.addr.2021.113826
- Wang Z, Xiong G, Tsang WC, Schätzlein AG, Uchegbu IF. Nose-to-brain delivery. J Pharmacol Exp Ther. 2019;370(3):593–601. doi:10.1124/jpet.119.258152
- Illum L. Is nose-to-brain transport of drugs in man a reality? J Pharm Pharmacol. 2010;56(1):3–17. doi:10.1211/0022357022539
- Selvaraj K, Gowthamarajan K, Karri VVSR. Nose to brain transport pathways an overview: potential of nanostructured lipid carriers in nose to brain targeting. Artif Cells Nanomed Biotechnol. 2017;46(8):1–8. doi:10.1080/21691401.2017.1420073
- Thorne RG, Emory CR, Ala TA, Frey WH. Quantitative analysis of the olfactory pathway for drug delivery to the brain. Brain Res. 1995;692(1–2):278–282.
- Thorne RG, Pronk GJ, Padmanabhan V, Frey WH. Delivery of insulin-like growth factor-I to the rat brain and spinal cord along olfactory and trigeminal pathways following intranasal administration. Neuroscience. 2004;127(2):481–496. doi:10.1016/j.neuroscience.2004.05.029
- Illum L. Transport of drugs from the nasal cavity to the central nervous system. Eur J Pharm Sci. 2000;11(1):1–18.
- Gänger S, Schindowski K. Tailoring formulations for intranasal nose-to-brain delivery: a review on architecture, physico-chemical characteristics and mucociliary clearance of the nasal olfactory mucosa. Pharmaceutics. 2018;10(3):116. doi:10.3390/pharmaceutics10030116
- Bourganis V, Kammona O, Alexopoulos A, Kiparissides C. Recent advances in carrier mediated nose-to-brain delivery of pharmaceutics. Eur J Pharm Biopharm. 2018;128:337–362. doi:10.1016/j.ejpb.2018.05.009
- Nižić Nodilo L, Ugrina I, Špoljarić D, et al. A dry powder platform for nose-to-brain delivery of dexamethasone: formulation development and nasal deposition studies. Pharmaceutics. 2021;13(6):795. doi:10.3390/pharmaceutics13060795
- Salade L, Wauthoz N, Vermeersch M, Amighi K, Goole J. Chitosan-coated liposome dry-powder formulations loaded with ghrelin for nose-to-brain delivery. Eur J Pharm Biopharm. 2018;129:257–266. doi:10.1016/j.ejpb.2018.06.011
- Chaudhary K, Sharma D. Cubosomes: a Potential Drug Delivery System. Asian J Pharm Res Dev. 2021;9(5):93–101. doi:10.22270/ajprd.v9i5.981
- Thadanki M, Kumari PS, Prabha KS. Overview of cubosomes: a nano particle. Int J Res Pharm Chem. 2011;1(3):535–541.
- Spicer PT, Hayden KL, Lynch ML, Ofori-Boateng A, Burns JL. Novel process for producing cubic liquid crystalline nanoparticles (cubosomes). Langmuir. 2001;17(19):5748–5756. doi:10.1021/la010161w
- Karami Z, Hamidi M. Cubosomes: remarkable drug delivery potential. Drug Discov Today. 2016;21(5):789–801. doi:10.1016/j.drudis.2016.01.004
- Yaghmur A, Tran BV, Moghimi SM. Non-lamellar liquid crystalline nanocarriers for thymoquinone encapsulation. Molecules. 2020;25(1):1–14. doi:10.3390/molecules25010016
- Barriga HMG, Holme MN, Stevens MM. Cubosomes: the next generation of smart lipid nanoparticles? Angew Chemie Int Ed. 2019;58(10):2958–2978. doi:10.1002/anie.201804067
- Chountoulesi M, Perinelli DR, Pippa N, et al. Physicochemical, morphological and thermal evaluation of lyotropic lipidic liquid crystalline nanoparticles: the effect of stimuli-responsive polymeric stabilizer. Colloids Surfaces a Physicochem Eng Asp. 2020;595:124678. doi:10.1016/j.colsurfa.2020.124678
- Chong JYT, Mulet X, Waddington LJ, Boyd BJ, Drummond CJ. Steric stabilisation of self-assembled cubic lyotropic liquid crystalline nanoparticles: high throughput evaluation of triblock polyethylene oxide-polypropylene oxide-polyethylene oxide copolymers. Soft Matter. 2011;7(10):4768–4777. doi:10.1039/c1sm05181d
- Elsenosy FM, Abdelbary GA, Elshafeey AH, Elsayed I, Fares AR. Brain targeting of duloxetine hcl via intranasal delivery of loaded cubosomal gel: in vitro characterization, ex vivo permeation, and in vivo biodistribution studies. Int J Nanomedicine. 2020;15:9517–9537. doi:10.2147/IJN.S277352
- Eissa EM, Elkomy MH, Eid HM, et al. Intranasal delivery of granisetron to the brain via nanostructured cubosomes-based in situ gel for improved management of chemotherapy-induced emesis. Pharmaceutics. 2022;14:7. doi:10.3390/pharmaceutics14071374
- Ahmad S, Khan I, Pandit J, et al. Brain targeted delivery of carmustine using chitosan coated nanoparticles via nasal route for glioblastoma treatment. Int J Biol Macromol. 2022;221:435–445. doi:10.1016/j.ijbiomac.2022.08.210
- Fong SS, Foo YY, Saw WS, et al. Chitosan-coated-PLGA nanoparticles enhance the antitumor and antimigration activity of stattic – a STAT3 dimerization blocker. Int J Nanomedicine. 2022;17:137–150. doi:10.2147/IJN.S337093
- Abbas H, El Sayed NS, Youssef NAHA, et al. Novel luteolin‐loaded chitosan decorated nanoparticles for brain‐targeting delivery in a sporadic alzheimer’s disease mouse model: focus on antioxidant, anti‐inflammatory, and amyloidogenic pathways. Pharmaceutics. 2022;14(5):1–26. doi:10.3390/pharmaceutics14051003
- Yasir M, Zafar A, Noorulla KM, et al. Nose to brain delivery of donepezil through surface modified NLCs: formulation development, optimization, and brain targeting study. J Drug Deliv Sci Technol. 2022;75:103631. doi:10.1016/j.jddst.2022.103631
- Calmet H, Inthavong K, Eguzkitza B, Lehmkuhl O, Houzeaux G, Vázquez M. Nasal sprayed particle deposition in a human nasal cavity under different inhalation conditions. PLoS One. 2019;14(9):e0221330–e0221330. doi:10.1371/journal.pone.0221330
- Warnken ZN, Smyth HDCC, Davis DA, Weitman S, Kuhn JG, Williams RO. Personalized medicine in nasal delivery: the use of patient-specific administration parameters to improve nasal drug targeting using 3D-printed nasal replica casts. Mol Pharm. 2018;15(4):1392–1402. doi:10.1021/acs.molpharmaceut.7b00702
- Maaz A, Blagbrough IS, De Bank PA. In vitro evaluation of nasal aerosol depositions: an insight for direct nose to brain drug delivery. Pharmaceutics. 2021;13(7):1079. doi:10.3390/pharmaceutics13071079
- von Halling Laier C, Gibson B, van de Weert M, et al. Spray dried cubosomes with ovalbumin and Quil-A as a nanoparticulate dry powder vaccine formulation. Int J Pharm. 2018;550(1–2):35–44. doi:10.1016/j.ijpharm.2018.08.036
- Spicer PT, Small WB, Lynch ML, Burns JL. Dry powder precursors of cubic liquid crystalline nanoparticles (cubosomes). J Nanoparticle Res. 2002;4(4):297–311.
- Tyler AII, Barriga HMG, Parsons ES, et al. Electrostatic swelling of bicontinuous cubic lipid phases. Soft Matter. 2015;11(16):3279–3286. doi:10.1039/c5sm00311c
- Cone RA. Barrier properties of mucus. Adv Drug Deliv Rev. 2009;61(2):75–85. doi:10.1016/j.addr.2008.09.008
- Bhattamisra SK, Shak AT, Xi LW, et al. Nose to brain delivery of rotigotine loaded chitosan nanoparticles in human SH-SY5Y neuroblastoma cells and animal model of Parkinson’s disease. Int J Pharm. 2020;579. doi:10.1016/j.ijpharm.2020.119148
- Shiran MR, Akhtari J. Chitosan coating of anionic liposomes containing sumatriptan succinate: a candidate for nasal administration. Nanomed J. 2021;8(2). doi:10.22038/nmj.2021.08.002
- Yu S, Xu X, Feng J, Liu M, Hu K. Chitosan and chitosan coating nanoparticles for the treatment of brain disease. Int J Pharm. 2019;560:282–293. doi:10.1016/j.ijpharm.2019.02.012
- Aderibigbe BA, Naki T. Chitosan-based nanocarriers for nose to brain delivery. Appl Sci. 2019;9:11. doi:10.3390/app9112219
- Sandri G, Motta S, Bonferoni MC, et al. Chitosan-coupled solid lipid nanoparticles: tuning nanostructure and mucoadhesion. Eur J Pharm Biopharm. 2017;110:13–18. doi:10.1016/j.ejpb.2016.10.010
- Rigaut C, Deruyver L, Goole J, Haut B, Lambert P. Instillation of a dry powder in nasal casts: parameters influencing the olfactory deposition with uni- and bi-directional devices. Front Med Technol. 2022;4:1–17. doi:10.3389/fmedt.2022.924501
- Manini G, Deldime M, Benali S, Raquez JM, Goole J. Long-acting implantable dosage forms containing paliperidone palmitate obtained by 3D printing. Int J Pharm. 2021;603:120702. doi:10.1016/j.ijpharm.2021.120702
- Wong PT, Wang SH, Ciotti S, et al. Formulation and characterization of nanoemulsion intranasal adjuvants: effects of surfactant composition on mucoadhesion and immunogenicity. Mol Pharm. 2015;11(2):531–544. doi:10.1021/mp4005029.Formulation
- Gonçalves VSS, Matias AA, Poejo J, Serra AT, Duarte CMM. Application of RPMI 2650 as a cell model to evaluate solid formulations for intranasal delivery of drugs. Int J Pharm. 2016;515(1–2):1–10. doi:10.1016/j.ijpharm.2016.09.086
- Reichl S, Becker K. Cultivation of RPMI 2650 cells as an in-vitro model for human transmucosal nasal drug absorption studies: optimization of selected culture conditions. J Pharm Pharmacol. 2012;64(11):1621–1630. doi:10.1111/j.2042-7158.2012.01540.x
- Wengst A, Reichl S. {RPMI} 2650 epithelial model and three-dimensional reconstructed human nasal mucosa as in vitro models for nasal permeation studies. Eur J Pharm Biopharm. 2010;74(2):290–297. doi:10.1016/j.ejpb.2009.08.008
- Bai S, Yang T, Abbruscato TJ, Ahsan F. Evaluation of human nasal RPMI 2650 cells grown at an air–liquid interface as a model for nasal drug transport studies. J Pharm Sci. 2008;97(3):1165–1178. doi:10.1002/jps.21031
- Gavini E, Rassu G, Ferraro L, et al. Influence of polymeric microcarriers on the in vivo intranasal uptake of an anti-migraine drug for brain targeting. Eur J Pharm Biopharm. 2013;83(2):174–183. doi:10.1016/j.ejpb.2012.10.010
- Williams G, Suman JD. In vitro anatomical models for nasal drug delivery. Pharmaceutics. 2022;14(7):1353. doi:10.3390/pharmaceutics14071353
- Charlton ST, Davis SS, Illum L. Evaluation of bioadhesive polymers as delivery systems for nose to brain delivery: in vitro characterisation studies. J Control Release. 2007;118:225–234. doi:10.1016/j.jconrel.2006.12.014
- Scherließ R. Nasal formulations for drug administration and characterization of nasal preparations in drug delivery. Ther Deliv. 2020;11(3):183–191. doi:10.4155/tde-2019-0086
- Mistry A, Stolnik S, Illum L. Nanoparticles for direct nose-to-brain delivery of drugs. Int J Pharm. 2009;379(1):146–157. doi:10.1016/j.ijpharm.2009.06.019
- Shadab MD, Khan RA, Mustafa G, et al. Bromocriptine loaded chitosan nanoparticles intended for direct nose to brain delivery: pharmacodynamic, Pharmacokinetic and Scintigraphy study in mice model. Eur J Pharm Sci. 2013;48(3):393–405. doi:10.1016/j.ejps.2012.12.007
- Rassu G, Soddu E, Cossu M, Gavini E, Giunchedi P, Dalpiaz A. Particulate formulations based on chitosan for nose-to-brain delivery of drugs. A review. J Drug Deliv Sci Technol. 2016;32:77–87. doi:10.1016/j.jddst.2015.05.002
- Rassu G, Ferraro L, Pavan B, Giunchedi P, Gavini E, Dalpiaz A. The role of combined penetration enhancers in nasal microspheres on in vivo drug bioavailability. Pharmaceutics. 2018;10(4):8–10. doi:10.3390/pharmaceutics10040206
- Raj R, Wairkar S, Sridhar V, Gaud R. Pramipexole dihydrochloride loaded chitosan nanoparticles for nose to brain delivery: development, characterization and in vivo anti-Parkinson activity. Int J Biol Macromol. 2018;109:27–35. doi:10.1016/j.ijbiomac.2017.12.056
- von Halling Laier C, Sonne Alstrøm T, Travers Bargholz M, et al. Evaluation of the effects of spray drying parameters for producing cubosome powder precursors. Eur J Pharm Biopharm. 2019;135:44–48. doi:10.1016/j.ejpb.2018.12.008
- Li L, Sun S, Parumasivam T, et al. L-Leucine as an excipient against moisture on in vitro aerosolization performances of highly hygroscopic spray-dried powders. Eur J Pharm Biopharm. 2016;102:132–141. doi:10.1016/j.ejpb.2016.02.010
- Lechanteur A, Evrard B. Influence of composition and spray-drying process parameters on carrier-free DPI properties and behaviors in the lung: a review. Pharmaceutics. 2020;12:1. doi:10.3390/pharmaceutics12010055
- Yue-Xing C, Fei-Fei Y, Han W, et al. The effect of L-leucine on the stabilization and inhalability of spray-dried solid lipid nanoparticles for pulmonary drug delivery. J Drug Deliv Sci Technol. 2018;46(151):474–481. doi:10.1016/j.jddst.2018.06.011
- Cui Y, Zhang X, Wang W, et al. Moisture-resistant co-spray-dried netilmicin with l-leucine as dry powder inhalation for the treatment of respiratory infections. Pharmaceutics. 2018;10(4). doi:10.3390/pharmaceutics10040252
- Chan JGY, Tyne AS, Pang A, et al. A rifapentine-containing inhaled triple antibiotic formulation for rapid treatment of tubercular infection. Pharm Res. 2014;31(5):1239–1253. doi:10.1007/s11095-013-1245-7
- Chang YX, Yang JJ, Le PR, Chang Q, Liao YH. Anti-hygroscopic effect of leucine on spray-dried herbal extract powders. Powder Technol. 2014;266:388–395. doi:10.1016/j.powtec.2014.06.058
- Aquino RP, Prota L, Auriemma G, et al. Dry powder inhalers of gentamicin and leucine: formulation parameters, aerosol performance and in vitro toxicity on CuFi1 cells. Int J Pharm. 2012;426(1–2):100–107. doi:10.1016/j.ijpharm.2012.01.026
- Raula J, Thielmann F, Kansikas J, et al. Investigations on the humidity-induced transformations of salbutamol sulphate particles coated with L-leucine. Pharm Res. 2008;25(10):2250–2261. doi:10.1007/s11095-008-9613-4
- Boraey MA, Hoe S, Sharif H, Miller DP, Lechuga-Ballesteros D, Vehring R. Improvement of the dispersibility of spray-dried budesonide powders using leucine in an ethanol-water cosolvent system. Powder Technol. 2013;236:171–178. doi:10.1016/j.powtec.2012.02.047
- Wang X, Wan W, Lu J, Quan G, Pan X, Liu P. Effects of L-leucine on the properties of spray-dried swellable microparticles with wrinkled surfaces for inhalation therapy of pulmonary fibrosis. Int J Pharm. 2021;610:121223. doi:10.1016/j.ijpharm.2021.121223
- Yarragudi SB, Kumar H, Jain R, Tawhai M, Rizwan S. Olfactory targeting of microparticles through inhalation and bi-directional airflow: effect of particle size and nasal anatomy. J Aerosol Med Pulm Drug Deliv. 2020;33(5):258–270. doi:10.1089/jamp.2019.1549
- Schroeter JD, Tewksbury EW, Wong BA, Kimbell JS. Experimental measurements and computational predictions of regional particle deposition in a sectional nasal model. J Aerosol Med Pulm Drug Deliv. 2015;28(1):20–29. doi:10.1089/jamp.2013.1084
- Shi H, Kleinstreuer C, Zhang Z. Modeling of inertial particle transport and deposition in human nasal cavities with wall roughness. J Aerosol Sci. 2007;38(4):398–419. doi:10.1016/j.jaerosci.2007.02.002
- EMA. Invega, INN-paliperidone - scientific discussion; 2017.
- Barriga HMG, Ces O, Law RV, Seddon JM, Brooks NJ. Engineering swollen cubosomes using cholesterol and anionic lipids. Langmuir. 2019;35(50):16521–16527. doi:10.1021/acs.langmuir.9b02336
- Zabara A, Chong JTY, Martiel I, et al. Design of ultra-swollen lipidic mesophases for the crystallization of membrane proteins with large extracellular domains. Nat Commun. 2018;9:1. doi:10.1038/s41467-018-02996-5
- Sou T, McIntosh MP, Kaminskas LM, Prankerd RJ, Morton DAV. Designing a multicomponent spray-dried formulation platform for pulmonary delivery of biomacromolecules: the effect of polymers on the formation of an amorphous matrix for glassy state stabilization of biomacromolecules. Dry Technol. 2013;31(13–14):1451–1458. doi:10.1080/07373937.2013.788019
- Gates-Rector S, Blanton T. The powder diffraction file: a quality materials characterization database. Powder Diffr. 2019;34(4):352–360. doi:10.1017/S0885715619000812
- Nasr M, Ghorab MK, Abdelazem A. In vitro and in vivo evaluation of cubosomes containing 5-fluorouracil for liver targeting. Acta Pharm Sin B. 2015;5(1):79–88. doi:10.1016/j.apsb.2014.12.001
- Mangal S, Meiser F, Tan G, et al. Relationship between surface concentration of l-leucine and bulk powder properties in spray dried formulations. Eur J Pharm Biopharm. 2015;94:160–169. doi:10.1016/j.ejpb.2015.04.035
- Foo MY, Cheng Y-S-S, Su W-C-C, Donovan MD. The influence of spray properties on intranasal deposition. J Aerosol Med. 2007;20(4):495–508. doi:10.1089/jam.2007.0638
- Moraga-Espinoza D, Warnken Z, Moore A, Williams RO, Smyth HDCC. A modified USP induction port to characterize nasal spray plume geometry and predict turbinate deposition under flow. Int J Pharm. 2018;548(1):305–313. doi:10.1016/j.ijpharm.2018.06.058
- Pu Y, Goodey AP, Fang X, Jacob K. A comparison of the deposition patterns of different nasal spray formulations using a nasal cast. Aerosol Sci Technol. 2014;48(9):930–938. doi:10.1080/02786826.2014.931566
- Van Strien J, Petersen P, Lappas P, et al. Spray characteristics from nasal spray atomization. J Aerosol Sci. 2022;165. doi:10.1016/j.jaerosci.2022.106009
- Inthavong K, Tian ZF, Tu JY, Yang W, Xue C. Optimising nasal spray parameters for efficient drug delivery using computational fluid dynamics. Comput Biol Med. 2008;38(6):713–726. doi:10.1016/j.compbiomed.2008.03.008
- Kaliner M, Shelhamer JH, Borson B, et al. Human respiratory mucus. J Allergy Clin Immunol. 1984;73(3):318–323. doi:10.1016/0091-6749(84)90403-2
- Nikogeorgos N, Efler P, Kayitmazer AB, Lee S. “Bio-glues” to enhance slipperiness of mucins: improved lubricity and wear resistance of porcine gastric mucin (PGM) layers assisted by mucoadhesion with chitosan. Soft Matter. 2015;11(3):489–498. doi:10.1039/c4sm02021a
- Yakubov GE, Papagiannopoulos A, Rat E, Waigh TA. Charge and interfacial behavior of short side-chain heavily glycosylated porcine stomach mucin. Biomacromolecules. 2007;8(12):3791–3799. doi:10.1021/bm700721c
- Bansil R, Turner BS. The biology of mucus: composition, synthesis and organization. Adv Drug Deliv Rev. 2018;124:3–15. doi:10.1016/j.addr.2017.09.023
- Sogias IA, Williams AC, Khutoryanskiy VV. Why is chitosan mucoadhesive? Biomacromolecules. 2008;9(7):1837–1842. doi:10.1021/bm800276d
- Mazzarino L, Travelet C, Ortega-Murillo S, et al. Elaboration of chitosan-coated nanoparticles loaded with curcumin for mucoadhesive applications. J Colloid Interface Sci. 2012;370(1):58–66. doi:10.1016/j.jcis.2011.12.063
- Pardeshi CV, Belgamwar VS. Direct nose to brain drug delivery via integrated nerve pathways bypassing the blood–brain barrier: an excellent platform for brain targeting. Expert Opin Drug Deliv. 2013;10(7):957–972. doi:10.1517/17425247.2013.790887
- Djupesland PG. Nasal drug delivery devices: characteristics and performance in a clinical perspective—a review. Drug Deliv Transl Res. 2013;3(1):42–62. doi:10.1007/s13346-012-0108-9
- Nakamura H, Watano S. Direct permeation of nanoparticles across cell membrane: a review. KONA Powder Part J. 2018;2018(35):49–65. doi:10.14356/kona.2018011
- Salatin S, Maleki Dizaj S, Yari Khosroushahi A. Effect of the surface modification, size, and shape on cellular uptake of nanoparticles. Cell Biol Int. 2015;39(8):881–890. doi:10.1002/cbin.10459
- Arvizo RR, Miranda OR, Thompson MA, et al. Effect of nanoparticle surface charge at the plasma membrane and beyond. Nano Lett. 2010;10(7):2543–2548. doi:10.1021/nl101140t
- Clementino AR, Pellegrini G, Banella S, et al. Structure and fate of nanoparticles designed for the nasal delivery of poorly soluble drugs. Mol Pharm. 2021;18(8):3132–3146. doi:10.1021/acs.molpharmaceut.1c00366
- Albarki MA, Donovan MD. Uptake of cationic PAMAM-PLGA nanoparticles by the nasal mucosa; 2022.
- Akilo OD, Choonara YE, Strydom AM, et al. AN in vitro evaluation of a carmustine-loaded Nano-co-Plex for potential magnetic-targeted intranasal delivery to the brain. Int J Pharm. 2016;500(1–2):196–209. doi:10.1016/j.ijpharm.2016.01.043
- Fröhlich E. The role of surface charge in cellular uptake and cytotoxicity of medical nanoparticles. Int J Nanomed. 2012;7:5577–5591. doi:10.2147/IJN.S36111