Abstract
Purpose
To investigate the anchoring of plasmid DNA/anti-DNA antibody/cationic lipid tri-complex (DACmicelles) onto bisphosphonate-modified 316 L coronary stents for cardiovascular site-specific genedelivery.
Methods
Stents were first modified with polyallylamine bisphosphonate (PAA-BP), thereby enabling theretention of a PAA-BP molecular monolayer that permits the anchoring (via vector-binding molecules)of DAC micelles. DAC micelles were then chemically linked onto the PAA-BP-modified stents by usingN-succinimidyl-3-(2-pyridyldithiol)-propionate (SPDP) as a crosslinker. Rhodamine-labeled DNA wasused to assess the anchoring of DAC micelles, and radioactive-labeled antibody was used to evaluatebinding capacity and stability. DAC micelles (encoding green fluorescent protein) were tethered ontothe PAA-BP-modified stents, which were assessed in cell culture. The presence of a PAA-BP molecularmonolayer on the steel surface was confirmed by X-ray photoelectron spectroscopy and atomic forcemicroscope analysis.
Results
The anchoring of DAC micelles was generally uniform and devoid of large-scale patches of defects.Isotopic quantification confirmed that the amount of antibody chemically linked on the stents was17-fold higher than that of the physical adsorbed control stents and its retention time was alsosignificantly longer. In cell culture, numerous green fluorescent protein-positive cells were foundon the PAA-BP modified stents, which demonstrated high localization and efficiency of genedelivery.
Conclusion
The DAC micelle-immobilized PAA-BP-modified stents were successful as a gene delivery system.Gene delivery using DAC micelle-tethered stent-based PAA-BP functionalization should be suitable fora wide array of single or multiple therapeutic gene strategies, and could be used on cardiovascularmetallic implants for achieving efficient gene therapy.
Introduction
Cardiovascular gene delivery has been previously investigated for mitigation of in-stentrestenosis.Citation1–Citation4 The use of the stent itself as a platform for gene delivery isattractive because it is site specific, potentially helping to avoid distal spread of therapeuticDNA and viral vectors. For example, a wide range of therapeutic DNA and viruses were delivered fromthe stent surface to reduce the extent of restenosis.Citation5–Citation7 The above studies have all usedpolymer-coated stents to elute the genes. This polymer component, however, has been shown to causelocal inflammatory responses and late thrombosis.Citation8,Citation9 The use of a technology that does notneed a polymer layer may simplify the complex device coating formulation. For example, Fishbein etal has investigated alternatives to polymer coatings.Citation10,Citation11 They reported that pretreatment ofmetal alloy surfaces with an aqueous bisphosphonate solution, polyallylamine bisphosphonate(PAA-BP), enables adenoviral expressing inducible nitric oxide synthase vectors binding to baremetal, which showed effective gene vector delivery and resulted in significant inhibition ofrestenosis in vivo. Biocompatibility evaluation in rats also showed that there was no differencebetween PAA-BP-modified stents and control bare metal stents in the inflammatory response.
Since the late 1970s, it has been demonstrated that phosphonic acid, RPO3H2(R is an organic component), readily reacts with a wide range of metal salts and oxides, leading toa rich variety of one- to three-dimensional metal organic frameworks, also called metalphosphonates. The cohesion of these networks results from the formation of metal phosphonateionocovalent bonds, which usually provide highly stable architectures.Citation12,Citation13 Moreover,phosphonic acids show a high compatibility with other organic functional groups and both their esterand acid forms can generally be used for surface modifications. In the study by Levy et al, PAA-BPthat can both interact with metal oxide surfaces and provide reactive sites for chemical conjugationwas synthesized by a direct Michael addition of PAA to the activated double bond ofvinylidenebisphosphonic acid. The metallic surface could be modified through aminobisphosphonatesexposure, thereby attaching to the metallic surface a derivatizable polybisphosphonate molecule thatcould, in turn, be covalently conjugated with vector-binding agents.
Gene delivery vectors such as naked plasmids, viral vectors, and nonviral nanodelivery have beensuccessfully used in experimental gene therapy.Citation14–Citation16 However, there are severalrecurring issues that have led to reconsideration of their use in human clinical trials. Theseinclude inefficient in vivo gene transfer, high cytotoxicity, strong inflammatory reactions, andpotential risk of viral DNA integration to host genome. Previous studies by the authors’group have reported a novel plasmid DNA vector composed of plasmid DNA/anti-DNA antibody/cationiclipid tri-complex (DAC micelles), which exhibits high transfection efficiency, high nuclear entry,and low toxicity both in vitro and in vivo.Citation17,Citation18 This complex formed stablenanoscaled micelles with a mean particle diameter around 360 nm and a zeta potential of −15mV by self-assembling process. The authors’ studies also showed that DAC micelles arestructurally stable under cell culture conditions; furthermore, the entire complex can remain intactthroughout cellular processing including nuclear entry.
The authors’ group has focused on covalent linking of DAC micelles on the collagen-coatedstents in order to deliver plasmid DNA by a vector tethering mechanism.Citation19,Citation20 This approachhas been shown to be feasible, with high levels of regional expression in vitro and in vivo. In thecurrent study, the intention was to explore new applications of DAC micelles in gene delivery andfurther investigate the binding stability and the controlled delivery behavior of DAC micelles boundon a PAA-BP-modified stent surface. First, the stent surface was modified with PAA-BP, therebyenabling the retention of a PAA-BP molecular monolayer that permits the attachment (viavector-binding molecules) of DAC gene vectors. After modification, the PAA-BP-modified stent surfacewas activated by N-succinimidyl-3-(2-pyridyldithiol)-propionate (SPDP). These thiol-reactive siteswere then further reacted in each instance to attach DAC micelles for vector tethering. The novelplasmid DNA delivery system based on PAA-BP-modified stents was characterized by assessments of genetransfection efficiency.
Materials and methods
Materials
316 L stainless steel foils were obtained from Goodfellow Corporation (Coraopolis, PA, USA).Mustang 316 L stainless steel coronary stents (2.5 × 13 mm) were a gift from MicroPortMedical Co, Ltd (Shanghai, People’s Republic of China). 316 L stainless steel foils wereused for X-ray photoelectron spectroscopy (XPS) and atomic force microscopy (AFM) of the modifiedsteel surface, while 316 L stainless steel stents were applied to in vitro gene expression. PAA-BPwas a gift that Ivan S Alferiev synthesized. The plasmid encoding a green fluorescent protein (GFP)with enhanced fluorescence (pEGFP-N3) was purchased from Clontech Laboratories(Mountain View, CA, USA). Monoclonal mouse anti-bovine DNA antibody (immunoglobulin M) recognizingdouble-strand and single-strand DNA (1 mg/mL) was obtained from US Biological (Swampscott, MA, USA)and nonspecific mouse immunoglobulin M antibody was from Rockland Immunochemicals Inc(Gilbertsville, PA, USA). Rat arterial smooth muscle cell (A10) was purchased from American TissueType Collection (Manassas, VA, USA). Lipofectamine™ 2000 was from Life Technologies(Carls-bad, CA, USA). SPDP was from Thermo Fisher Scientific (Rockford, IL, USA). Sodium iodide(Na125I) was from GE Healthcare (Little Chalfont, UK). GFP expression was assessed usingfluorescent microscopy with a filter calibrated to detect fluorescein isothiocyanate (Eclipse E800;Nikon Corporation, Tokyo, Japan).
Metal surface modification with functional PAA-BPCitation11
To clean the stainless steel surface of any impurities, the 316 L stainless steel foils wererinsed twice in 1.5 N nitric acid (about 30 seconds each time), washed copiously with doubledistilled water, and incubated twice in isopropanol at 55°C with shaking (15 minutes eachtime). For stent cleaning/surface activation, the additional steps of chloroform incubation (15minutes, 55°C, shaking) and heating (in a glass vial) at 250°C for 2 hours weretaken. For PAA-BP modification, the cleaned metal samples were exposed to 3% (w/v) aqueousPAA-BP (pH 5.5) at 60°C for 4 hours. Upon completion of incubation with PAA-BP, each samplewas rinsed with an excess amount of double distilled water.
Surface characterization
The surface elemental composition was analyzed by XPS ESCALABMk II (VG Scienta, Uppsala, Sweden)equipped with an aluminum Kα X-ray source. Surface profiles were visualized by using aDimension™ 3100 AFM (Veeco Instruments, Santa Barbara, CA, USA). Imaging was performed inthe intermittent noncontact (tapping) mode by using oscillating linear silicon tips with a resonancefrequency range of 300–350 Hz. Each data scan was collected over a 25 μm2area at a scanning frequency of 0.50 Hz.
Protocol for preparing DAC micelle-tethered PAA-BP-modified stents
The PAA-BP-modified stents were reacted with SPDP (20 mg/mL) at room temperature for 2 hoursfollowed with dithiothreitol to introduce sulfhydryl groups on the stents. Separately, the mousemonoclonal anti-DNA antibody was reacted with an excess of SPDP to introduce dithiol groups on theantibody molecules. The dithiol-activated anti-DNA antibody was then chemically linked to the stentsthrough the thiol exchange reaction. The antibody-bound stent was then incubated in apEGFP-N3 DNA solution (20 μg pure plasmid DNA in 200 μLDulbecco’s modified Eagle’s medium [DMEM]) at 37°C for 1hour followed by an extensive rinse with copious phosphate buffered saline (PBS) solution. Thestents were further reacted with Lipofectamine 2000 reagent (5 μL/200 μL) andincubated at room temperature for 30 minutes before incubation with cells. A nonspecific antibodywas attached to the modified stents in the same manner as the contrast. In some cases, physicalabsorption of the antibody onto PAA-BP-modified stents was performed and used as the control.
Rhodamine-labeled DNA was used to assess the anchoring of DAC micelles on PAA-BP-modified stentsunder a fluorescence microscope (Nikon Eclipse E800).
Binding capacity and stability of 125I-labeled anti-DNA antibody onPAA-BP-modified stents
Anti-DNA antibody was iodinated by a modified chloramine-T procedure.Citation21 A 250 μg aliquot of anti-DNA antibody (2.2 mg/mL) was mixedwith 3.5 μL Na125I (37 MBq) and 60 μL chloramine-T (5 mg/mL, pH 7.5) atroom temperature and incubated for 3 minutes. The reaction was stopped by adding 80 μL of asodium metabisulfite solution (10 mg/mL, pH7.5). 125I-labeled antibody was purified bygel filtration on a Sephadex® G-50 column (Sigma-Aldrich, St Louis, MO, USA) toremove unreacted iodine. The 125I-labeled antibody was activated with SPDP and chemicallylinked on the stents as described above (n = 5). A control group was made by directlysoaking the PAA-BP-modified stents in the 125I-labeled antibody solution (n = 5).Both types of antibody-loaded stents were subjected to the same PBS rinse procedure. To remove theunbound antibody, the eluting solution was monitored using a gamma counter until the level ofradioactivity was close to the background. The binding capacity of the antibody on the stents wasdetermined by measuring the radioactivity remaining on the stents. To evaluate the binding stabilityof the 125I-labeled antibody, stents were incubated in PBS at 37°C with shaking(140 rpm). At predetermined time intervals, the incubation solution was replaced with the samevolume of fresh PBS and the radioactivity remaining on the stents was measured with a gammacounter.
Cell transfection by DAC micelle-tethered stents in vitro
The stents were incubated in a 1 × 106 A10 cell (rat arterial smooth musclecells) suspension at 37°C for 1 hour before being placed into 35 mm cell culture plates. Asuspension of 1 × 105 A10 cells in DMEM was added. The cells were incubated inserum-free medium for 5 hours followed by the addition of fetal bovine serum to a finalconcentration of 5%. The culture medium was changed to growth medium (DMEM +10% fetal bovine serum + 1% penicillin/streptomycin) after 24 hours. ThepEGFP-N3 gene expression, as demonstrated by fluorescein isothiocyanate-positivecells, was observed on a Nikon Eclipse E800 fluorescent microscope (Nikon Eclipse E800) equippedwith SPOT version 3.02 software (SPOT Imaging Solutions, Sterling Heights, MI, USA) and photographswere taken after 3 days of cell culture.
Statistical analysis
Data for all experiments were expressed as the mean ± standard error of the mean. Thesignificance of differences was assessed using Student’s t-test or analysisof variance. P < 0.05 was considered statistically significant.
Results and discussion
Chemical reasoning of tethering DAC micelles onto the PAA-BP-modified metal surface
In this study, the 316 L stainless steel substrates – mainly composed of iron, chromium,and nickel – were modified through PAA-BP exposure, thereby attaching to the metallicsurface a derivatizable polybisphosphonate molecule that could, in turn, be covalently conjugatedwith DAC micelles for local gene delivery. A heterobifunctional crosslinker, SPDP, was used tochemically link the DAC micelles on the metal stent via a PAA-BP monolayer that provided thereactive amine groups. The basic chemistry for DAC micelles for binding on PAA-BP-modified stentsurface is: (1) to introduce sulfhydryl functional groups on the PAA-BP monolayer; (2) to derivatizethe anti-DNA antibody with pyridyl disulfides; (3) to couple the dithiol antibody to thesulfhydryl-activated PAA-BP monolayer; and (4) to form stable DAC micelles with incubation withplasmid DNA and cationic lipid. Thus, DAC micelles were covalently linked on the PAA-BP-modifiedstents.
Surface characterization of a functional PAA-BP-modified metal surface
XPS analysis was undertaken to verify the surface modification with PAA-BP. XPS confirmed thepresence of a PAA-BP molecular monolayer on the steel surface, demonstrating the emergence of acharacteristic phosphorus 2p signal in the XPS of the treated sample; a phosphorus signal is notpresent in the control 316 L steel ().Furthermore, the characteristic iron 2p peaks of the steel substrate were still present in the XPSfrom the PAA-BP-modified sample (),indicating that the thickness of the PAA-BP coordination layer is less than the effective XPSsampling depth (5 nm).
Figure 1 X-ray photoelectron spectroscopy to detect (A) phosphorus and (B) ironon nonmodified and polyallylamine bisphosphonate-modified steel surfaces demonstrates the appearanceof phosphorus after polyallylamine bisphosphonate treatment with persistent iron signals.
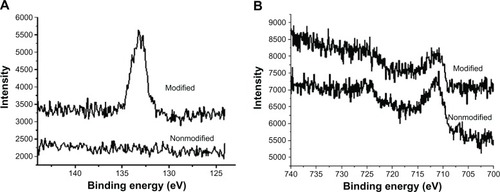
AFM was used to visualize surface topography after PAA-BP modification. AFM images of the surfaceof the bare and PAA-BP-modified metal surface are shown in . AFM images show that the PAA-BP-modified metal surface displayed substantiallyincreased roughness (root mean square: 25 ± 5 nm, measured on 2 μm × 2μm region), compared with that of bare surface (11 ± 3 nm). After PAA-BPmodification, the surface morphology changed tremendously. The increase of local surface roughnessfollowing PAA-BP modification illustrates that topographical as well as chemical changes to themetal surface can occur as a result of surface modification. It is likely that a thin, rough, butstable PAA-BP monolayer was formed on the metal surface.
Binding capacity and stability of 125I-labeled anti-DNA antibody onPAA-BP-modified stents
The anti-DNA antibody was successfully labeled with radioisotope 125I. Unreacted125I was completely separated from the 125I-labeled antibodies(125I-antibody) by gel filtration on a Sephadex G-50 column. The radiochemical purityreached a level of 98%, as determined by paper chromatography. The final concentration ofthe dithiol derivative 125I-antibody (after SPDP activation) was approximately 60μg/mL, as determined by Coomassie Brilliant Blue G-250. The results show that the amount of125I-antibody chemically linked on the PAA-BP-modified stents was 17-fold higher than onthe physically adsorbed control stents (;P < 0.01). The chemically linked 125I-antibody showed asustained release over a course of 21 days and approximately 50% of the total bound antibodywas retained on the stents at the end of 21 days. However, the physically adsorbed antibody on thecontrol stents was almost completely eluted out within 3 days ().
Figure 3 Binding capacity and stability of 125I-labeled anti-DNA antibody on thePAA-BP-modified stents. (A) The amount of antibodies chemically linked on stents was 19-fold higherthan on the physically adsorbed control stents (**P< 0.01). (B) The chemically linked 125I-labeled anti-DNA antibody showeda sustained release over the course of 21 days and 50% of the total bound antibodies wereretained on the stents by the end of 21 days. However, physically adsorbed antibody on the controlstents was almost completely eluted out within 3 days.
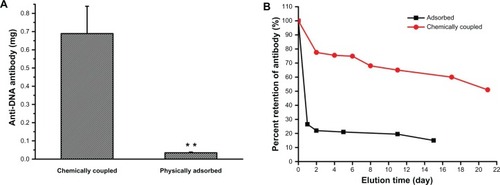
There was no significant difference in the binding capacity of 125I-antibodychemically linked on the PAA-BPP-modified stents (about 0.68 μg) and the collagen-coatedstents (about 0.76 μg) previously studied by the authors’ group, in which anti-DNAantibody was covalently immobilized on the collagen-coated stents in the same way as thePAA-BP-modified stents.Citation19 The antibody releasedfrom collagen-coated stents was relatively faster than the PAA-BP-modified stents, which shows thatonly 25% of the total bound antibody was retained on the collagen-coated stents at the endof 16 days. The results indicate that the method of PAA-BP-modified stents was superior to thecollagen-coated stents in the binding stability of the antibody.
Anchoring of rhodamine-labeled DAC micelles on PAA-BP-modified stents
DAC micelles were successfully immobilized onto PAA-BP-modified stents as demonstrated byimmunofluorescence studies using rhodamine-labeled DNA; control stents that did not containsulfhydryl-linked anti-DNA antibody demonstrated faint autofluorescence (). The result confirmed specific anchoring of micelles onSPDP-activated PAA-BP-modified stents. Further inspection of revealed that the anchoring of DAC micelles was generallyuniform and devoid of large-scale patches of defects. It was previously reported that plasmidDNA/polyethyleneimine polyplexes nonspecifically adsorbed on hydrophobic surfaces were highlyaggregated, resulting in reduced gene transfection efficiency.Citation22,Citation23 In the currentstudy, the chemically linked DAC micelles were less aggregated and better distributed on the surfaceof PAA-BP-modified stent, which would increase the chance of being transported within adhered cellsthrough substrate-mediated gene transfection.
Figure 4 Representative fluorescence microscopy images of immobilization of rhodamine-labeled DNA/anti-DNAantibody/cationic lipid micelles on polyallylamine bisphosphonate-modified stents. (A)Chemically linked on the stents; (B) physically adsorbed on the control stents(fluorescein isothiocyanate, ×100).
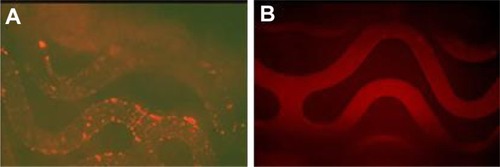
In vitro gene transfection mediated by DAC micelle-tethered stents
The PAA-BP-modified stents deposited with DAC micelles demonstrated efficient GFP genetransfection in A10 cells. The stents retrieved from cell culture after 72 hours of incubationcontained numerous GFP-transfected cells that infiltrated the PAA-BP coating on the stents,indicating a highly localized and efficient gene delivery pattern (). However, on the control stents that had been immobilized withnonspecific antibody and incubated with the same amount pEGFP-N3, only a fewtransfected GFP-positive cells were found (). Moreover, the stents showed no detrimental effects on cell growth.
Figure 5 In vitro gene transfection mediated by pEGFP-N3/anti-DNA antibody/cationic lipidmicelles-tethered stents in A10 cells. (A) Positive transfection usingpEGFP-N3/anti-DNA antibody/cationic lipid micelles modified stent (fluoresceinisothiocyanate, ×100); (B) negative control stents with a nonspecific anti-DNAantibody modification (fluorescein isothiocyanate, ×100).
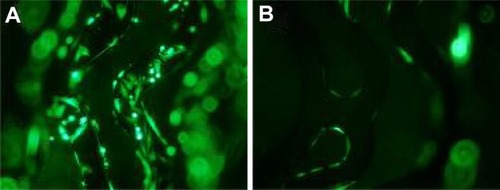
The authors’ group has focused on covalent linking of DAC micelles on the collagen-coatedstents in order to deliver the plasmid DNA by a vector tethering mechanism.Citation19,Citation20 This approachhas been shown to be feasible, with high levels of regional expression and no detectable spreadingof the vector beyond the implanted artery. However, polymer-coated gene delivery stents isproblematic because of harmful properties of the polymer coatings.Citation8,Citation9 Therefore, in thecurrent study, DAC micelles were directly immobilized onto the surface of PAA-BP-modified stentswithout the use of a polymer coating. In vitro transfection data showed that DAC micelles linked onPAA-BP-modified stents by chemical and immunoreaction ensured highly localized and efficient genetransfection. This is simply because surface-immobilized DAC micelles have more chance of being inclose proximity or in contact with cells, which can lead to high and long-lasting gene expression byimmediate internalization and sustained release effects.Citation24,Citation25 In addition, the formation ofnanoscale micelles can be crucial for cellular internalization of DNA and subsequent traffickingwithin cells.Citation22,Citation26
Conclusion
Through surface modification with PAA-BP and subsequent anchoring of DAC micelles, functionalizedstents with PAA-BP and DNA were created for cardiovascular gene delivery. The DACmicelle-immobilized PAA-BP-modified stents were successful as a gene delivery system, giving rise toefficient and localized gene delivery to arterial smooth muscle cells. Gene delivery using DACmicelle-tethered stent-based PAA-BP functionalization should be suitable for a wide array of singleor multiple therapeutic gene strategies, and could be used on cardiovascular metallic implants forachieving efficient gene therapy.
The financial support of the projects was from the NSFC of China (50830106, 50903093, and81271706).
Disclosure
The authors report no conflicts of interest in this work.
References
- SharifFDalyKCrowleyJO’BrienTCurrent status of catheter- and stent-based gene therapyCardiovasc Res200464220821615485679
- GaffneyMMHynesSOBarryFO’BrienTCardiovascular gene therapy: current status and therapeutic potentialBr J Pharmacol2007152217518817558439
- FishbeinIStachelekSJConnollyJMWilenskyRLAlferievILevyRJSite specific gene delivery in the cardiovascularsystemJ ControlRelease20051091–3374816298010
- RobertsonKEMcDonaldRAOldroydKGNicklinSABakerAHPrevention of coronary in-stent restenosis and vein graft failure:does vascular gene therapy have a role?PharmacolTher20121361233422796519
- SharifFHynesSOCooneyRGene-eluting stents: adenovirus-mediated delivery of eNOS to the bloodvessel wall accelerates re-endothelialization and inhibits restenosisMolTher200816101674168018714308
- CheHLBaeIHLimKSSuppression of post-angioplasty rest-enosis with an Akt1siRNA-embedded coronary stent in a rabbitmodelBiomaterials201233338548855622940215
- EgashiraKNakanoKOhtaniKLocal delivery of anti-monocyte chemoattractant protein-1 bygene-eluting stents attenuates in-stent stenosis in rabbits andmonkeysArterioscler Thromb VascBiol200727122563256817885211
- CarterAJAggarwalMKopiaGALong-term effects of polymer-based, slow-release, sirolimus-elutingstents in a porcine coronary modelCardiovascRes200463461762415306217
- TesfamariamBLocal vascular toxicokinetics of stent-based drugdeliveryToxicolLett200716829310217169513
- FishbeinIAlferievIBakayMLocal delivery of gene vectors from bare-metal stents by use of abiodegradable synthetic complex inhibits in-stent restenosis in rat carotidarteriesCirculation2008117162096210318413497
- FishbeinIAlferievISNyanguileOBisphosphonate-mediated gene vector delivery from the metal surfacesof stentsProc Natl Acad Sci U SA2006103115916416371477
- QueffelecCPetitMJanvierPKnightDABujoliBSurface modification using phosphonic acids andestersChemRev201211273777380722530923
- Van AlstenJGSelf-assembled monolayers on engineering metals: structure,derivatization, andutilityLangmuir1999152276057614
- GiaccaMZacchignaSVirus-mediated gene delivery for human genetherapyJ ControlRelease2012161237738822516095
- ElsabahyMNazaraliAFoldvariMNon-viral nucleic acid delivery: key challenges and futuredirectionsCurr DrugDeliv20118323524421291381
- ZhuLMahatoRILipid and polymeric carrier-mediated nucleic aciddeliveryExpert Opin DrugDeliv20107101209122620836625
- BurtonDYSongCXFishbeinIThe incorporation of an ion channel gene mutation associated with thelong QT syndrome (Q9E-hMiRP1) in a plasmid vector for site-specific arrhythmia gene therapy: invitro and in vivo feasibility studiesHum GeneTher200314990792212828861
- SongCXZhangLHZhangCLevyRJStudy on a novel non-virus triplex gene vectors composed of plasmidDNAProg BiochemBiophys2007348830835
- JinXMeiLSongCXImmobilization of plasmid DNA on an anti-DNA antibody modifiedcoronary stent for intravascular site-specific gene therapyJ GeneMed200810442142918213735
- ZhangLHLuoTZhangCAnti-DNA antibody modified coronary stent for plasmid gene delivery:results obtained from a porcine coronary stent modelJ GeneMed2011131374521259407
- HussainAAAwadRCrooksPADittertLWChloramine-T in radio-labeling techniques. I. Kinetics and mechanismof the reaction between chloramine-T and amino acidsAnalBiochem199321424954998109739
- SeguraTSheaLDSurface-tethered DNA complexes for enhanced genedeliveryBioconjugateChem2002133621629
- KimTGLeeYParkTGControlled gene-eluting metal stent fabricated by bio-inspired surfacemodification with hyaluronic acid and deposition of DNA/PEI polyplexesInt JPharm20103841–218118819799974
- LuoDSaltzmanWMEnhancement of transfection by physical concentration of DNA at thecell surfaceNatBiotechnol200018889389510932162
- ShenHTanJSaltzmanWMSurface-mediated gene transfer from nano-composites of controlledtextureNatMater20043856957415258575
- JiangJHBengaliZHouchinTLSheaLDSurface adsorption of DNA to tissue engineering scaffolds forefficient gene deliveryJ Biomed MaterRes20067715058