Abstract
The real problem in pharmaceutical preparation is drugs’ poor aqueous solubility, low permeability through biological membranes, and short biological t1/2. Conventional drug delivery systems are not able to overcome these problems. However, cyclodextrins (CDs) and their derivatives can solve these challenges. This article aims to summarize and review the history, properties, and different applications of cyclodextrins, especially the ability of inclusion complex formation. It also refers to the effects of cyclodextrin on drug solubility, bioavailability, and stability. Moreover, it focuses on preparing and applying gold nanoparticles (AuNPs) as novel drug delivery systems. It also studies the uses and effects of cyclodextrins in this field as novel drug carriers and targeting devices. The system formulated from AuNPs linked with CD molecules combines the advantages of both CD and AuNPs. Cyclodextrins benefit in increasing aqueous drug solubility, loading capacity, stability, and size control of gold NPs. Also, AuNPs are applied as diagnostic and therapeutic agents because of their unique chemical properties. Plus, AuNPs possess several advantages such as ease of detection, targeted and selective drug delivery, greater surface area, high loading efficiency, and higher stability than microparticles. In the present article, we tried to present the potential pharmaceutical applications of CD-derived AuNPs in biomedical applications including antibacterial, anticancer, gene-drug delivery, and various targeted drug delivery applications. Also, the article highlighted the role of CDs in the preparation and improvement of catalytic enzymes, the formation of self-assembling molecular print boards, the fabrication of supramolecular functionalized electrodes, and biosensors formation.
Introduction
Cyclodextrin (CD) and cyclic oligosaccharides, which combine glucose units (six units or more), are linked to each other by α-1,4-glycosidic linkage forming a hollow truncated cone shape structure. It was isolated and first described by Villiers from a culture medium of Bacillus Amylobacter, which was grown in starch.Citation1,Citation2 There are three famous kinds of cyclodextrins named alpha-cyclodextrin (α-CD) containing six glucose molecules, beta-cyclodextrin (β-CD) of seven glucose molecules, and gamma-cyclodextrin (γ-CD) composed of eight glucose units (-).Citation3,Citation4 These three major CDs are non-hygroscopic and crystalline materials.Citation5 The important characteristics of the mentioned cyclodextrins are stated in . In addition, illustrate the chemical structures of different CDs. Based on their architecture, toroidal or cone-shaped, there are two open-sided (one narrow side and the other is wider). The hydroxyl groups are in the periphery and classified into two categories, primary and secondary hydroxyl groups. The secondary OH groups are located on the wider edges of cyclodextrin molecules. The primary (-OH) groups are located on the narrow side of the CD torus. The melting points of α-, β-, and γ-CD are between 240°C and 265°C.Citation6,Citation7
Table 1 Distinctive Characteristic Features of α-, β-, and γ-CDs.
Figure 1 Schematic representation of α-, β-, and γ-Cyclodextrin, and the chemical derivatization of β-CD molecule.
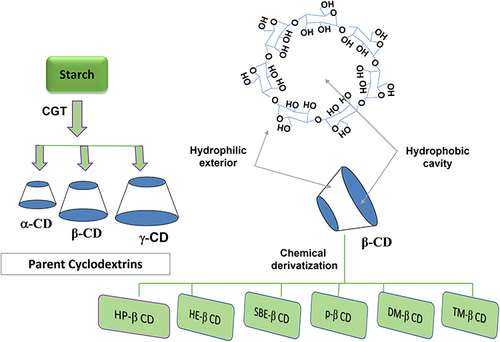
The aqueous solubility of β-CD is lower than that of the linear dextrin, which may be attributed to the strong internal hydrogen binding of the cyclodextrin molecules in its crystal state.Citation10 Chemical modifications were applied to produce highly water-soluble amorphous β-CD derivatives, including hydroxypropyl-β-CD, sufobutylether-β-CD, and epichlorohydrin-β-CD polymer (more than 500 mg/mL) ().Citation11,Citation12 Especially, the water-soluble polymerized β-CDs of higher molecular weight offer the advantages of complexation without toxic effects and amorphous state.Citation13,Citation14
Table 2 Characteristics of Different β-CD Derivatives
Herein, we report on a new review, summarizing the recent advance in constructing CD-based gold nanoparticles (CD-AuNPs) functional systems. The present review article discusses design methodologies, physicochemical properties, and unique advantages of different CD-based nanoparticles in detail. Also, the applications of the modified systems, including drug delivery, enzymatic catalysis, biomedical diagnosis, and sensing were highlighted in this article.
Ability of Cyclodextrin to Form Inclusion Complexes
Cyclodextrins have relatively hydrophobic inner cavities and a hydrophilic outer surface covered with hydroxyl groups.Citation8,Citation15 Therefore, CDs can provide a favorable environment for the inclusion of complex formation with hydrophobic organic bioactive compounds in different drug:CD ratios (1:1, 1:2, or 2:1).Citation16,Citation17
The phase solubility diagram will be useful for studying the inclusion complexation, which investigates the impact of CD (as a solubilizing agent) on the included drugs.Citation18,Citation19 The investigated drugs’ solubility behavior is classified, including category (A) and category (B) curves. The category (A) curves represent the soluble inclusion complexes formation, while the category (B)-type curves indicate the construction of poorly soluble inclusion complexes ().Citation18 Also, the A-category curves are subclassified into different subtypes, including AL (linearly, the guest solubility increases with the increase in CD concentration), AP in which a positive deviation isotherm was obtained, and AN (showing a negative deviation isotherm) subtypes.
Figure 2 Phase solubility diagram of β-CD. According to Higuchi and Connors, the types of phase-solubility diagrams of cyclodextrin presenting the solubility behavior of the included drugs upon increasing the CD concentration are two types (A and B) curves. Type (A) phase diagram is classified into three subtypes; AL: linear diagram; AP: positive deviation from linearity; AN: negative deviation from linearity. Also, type (B) is classified into two subtypes; B S: indicating the complex of limited solubility; and B I: showing the insoluble complex.
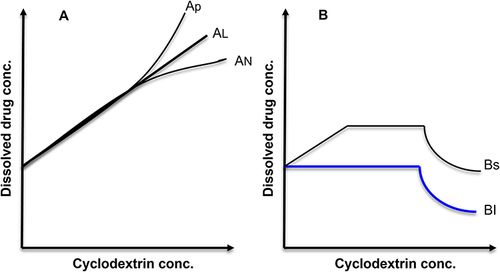
Similarly, B types are subclassified into B1 and Bs subtypes, indicating the formation of insoluble inclusion complexes and partially soluble complexes, respectively.Citation20 The most famous, frequent, and simple drug: CD ratio is 1:1. However, experimentally, there are different other uncommon drugs: CD ratios such as 1:2, 2:1, 1:3, or even more complicated associations were reported.Citation21,Citation22 The stoichiometric ratio (n) can be determined by isothermal titration calorimetry (ITC), which gives other parameters in addition to the complex ratio named the enthalpy, the entropy, and the binding constant, indicating the complexing affinity of the selected drug to the kind of cyclodextrin cavity. For example, adamantane molecules showed a (1:1) stoichiometric ratio with β-CD due to the perfect ball-like structure, size, and geometry of adamantane, which is suitable to form a perfect inclusion complex with β-CD. While as, in the case of cholesterol, as an example of larger molecules, the ITC results, which were reported previously indicated that the n value was (1:2) cholesterol:β-CD. An equilibrium is established between the associated and the dissociated species upon dissolving or dissociating these complexes.Citation23 The obtained equilibrium of each complex is expressed by the stability constant (Ka).Citation20,Citation24 For the 1:1 type of inclusion complexes, the equilibrium association or binding constant can be determined using the following equation.Citation25
Ka = slope/S0 (1-slope) (1)
Where S0 is the intrinsic solubility of the drug. Also, the slope in the equation is the slope of the curve linear portion of solubility phase diagrams, which will be constructed by plotting the drug solubility against cyclodextrin concentration.
The formation of soluble inclusion complexes (solubilization properties) comes from the replacement of water molecules that exist in the hydrophobic cavities of cyclodextrin molecules by the hydrophobic guest molecules via hydrophobic–hydrophobic interaction.Citation26,Citation27 Interestingly, the inclusion ability of CD molecules has been utilized as a base for their different pharmaceutical applications, including the solubility enhancement of hydrophobic drugs,Citation28,Citation29 increasing their dissolution rate and bioavailability,Citation30,Citation31 improving the guest stability and biological half-lives,Citation32,Citation33 and even for several food industry applications.Citation34
Moreover, the inclusion complexation ability of CDs was utilized as a base for the construction of more sophisticated supramolecular systems, including polymers,Citation35,Citation36 hydrogels,Citation37,Citation38 and nanoparticles.Citation39,Citation40 Examples of previously reported inclusion complexes with CDs and their applications are displayed in .
Table 3 Inclusion Complexes with Cyclodextrin and Their Applications
It is worth mentioning that CD complexation usually involves a host: guest of 1:1 ratio; concurrently higher-order complicated complexations (2:1, 1:2, 2:2, etc) could occur. The complexation process takes place as the CD cavity is slightly non-polar in aqueous solutions; therefore, it could be entrapped via the non-covalent interaction by water molecules of high enthalpy that are ready to be replaced with other less polar “guest molecules” forming CD complexes.Citation48 Noteworthy, several analytical techniques are applicable for characterizing the modified drug/CD complexes in the solid state, including thermo-analytical techniques (Differential scanning calorimetry (DSC), Thermogravimetric analysis (TGA), Hot-stage microscopy (HSM)), X-ray diffraction (Single crystal X-ray diffraction (SCXRD), powder X-ray diffraction (PXRD)), Spectroscopic techniques (Fourier-transform infra-red (FT-IR) spectroscopy, Attenuated total reflectance (ATR)-FTIR spectroscopy, and Raman spectroscopy), and Scanning electron microscopy (SEM).Citation49
Factors Affecting Inclusion Complex Formation
Type and Cavity Size of Cyclodextrin
It was reported that the kind of CD molecules (native CDs or derivatives) affected both the formation and the effectiveness of guest/CD complexes. In the case of Ibuproxam, the increase in the drug dissolution rate has been obtained upon using either β- or γ-CD molecules, but α-CD molecules were less suitable for stable inclusion complexes. The dissolution performance of the formed inclusion complexes appeared to be related to both the steric factors of the host molecule and the preparation method of the prepared solid systems. It was reported that the cavity size of α-CD was less suitable for accommodating Ibuproxam as a guest molecule, whereas a true inclusion complex of such a drug in either β-CD or γ-CD cavities. The solubilizing efficiency of the drug enclosed within all investigated CDs was 8, 16, and 13 regarding α-CD, β-CD, and γ-CD, respectively. Also, the stability constant of the formed complex was 65, 17,500, and 150, respectively.Citation50
Also, better dissolution performance has been observed from the inclusion complexation of Ketoprofen with methyl-beta-cyclodextrin (M-βCDs) compared to β-CDs.Citation51 Moreover, the polymerization of cyclodextrin improves the inclusion ability and accompanying characteristics of parent CDs since they were converted to the amorphous form.Citation21 Additionally, the formation of CD nano-sponges (CD-NS) was also accompanied by very high inclusion ability and encapsulation capacity.Citation52,Citation53 Recently, the solubility and solution rate of docetaxel was affected by the CD type utilized in the inclusion complexation.Citation54
Drug Ionization State/Medium pH
The structure of both cyclodextrins (neutral as native CD or charged one as SBE-CD) and the guest molecules (positively or negatively charged) play an important role in the degree of inclusion complexation. Generally, the presence of charge on the cyclodextrin structure provides an additional site of interaction compared to neutral cyclodextrins. This may be attributed to the fact that the strength of inclusion complexation increased with the existence of opposite charge between the guest and the host due to the increase in electrostatic or ionic interaction.Citation55 In comparison with HP-β-CD, SBE-B-CD of negative charges increase the solubility and inclusion ability of positively charged drugs such as DY-9760e, a novel cytoprotective agent.Citation56 On the other hand, the ionization of the drug is an important issue in the complexation ability of native CDs (neutral). It was reported that the unionizable drugs are easier to form inclusion complexation with CDs than the ionizable ones. The value of K1:1 of non-ionized sulindac was 340 M−1 at pH 2, and it was 139 M−1 at pH 6.Citation57
The complexation ability of CD molecules increases when the guest molecules carry opposite charges.Citation56 For example, the cationic (2- hydroxyl-3-[tri-methyl-ammonia] propyl)-β-CD acted as a very suitable solubilizer for many acidic drugs.Citation58 Also, the complexation of β-CD with a unionized form of sulindac was easier than in the case of an ionized one.Citation57 Regarding Piroxicam, the binding or stability constant values for the drug/CD complexes were affected by the change of pH values since it decreased by the increase in pH since it was 87 M−1 and 29 M−1 for pH 4.5 and 6, respectively. As a result, more effective complexation was obtained at an acidic pH.Citation59 Similarly, pH affects the thermodynamic stability of thymol and carvacrol inclusion complexes with β-CD in an aqueous medium.Citation60
Preparation Methods
Different techniques and methods were utilized for the preparation of inclusion complexes, including kneading (slurry),Citation48,Citation61,Citation62 solid dispersion,Citation47,Citation63 grinding,Citation64 supercritical CO2,Citation65 microwave irradiation,Citation66,Citation67 sealed heating,Citation68 freeze-drying,Citation69,Citation70 spray drying,Citation71,Citation72 dry mixing,Citation19 damp (wet) mixing, extrusion, solvent evaporation, or co-precipitation.Citation48 and summarize the common methods of CD complexation.
Table 4 Preparation Methods of CD Complexes
Noteworthy, the degree of complexation depends on the preparation method.Citation23,Citation113 Both spray-drying and freeze-drying techniques were reported as the most effective drug/CD inclusion complexation methods. The enhanced dissolution rate of spray-dried products might be attributed to the decreased particle size, the high-energetic amorphous state, and the inclusion complex formation.Citation114 Also, as a powder production method commonly used in pharmaceutical fields, spray-drying possesses the advantages of being rapid, low-cost, and easily scalable. In addition, the spray-dried microcapsules were of spherical and uniform particle size. The solubility of the guest molecules upon using the spray-dried technique may be attributed to the encapsulation of the guest into the cylindrical cavity of cyclodextrin, and thus the thermal properties of the host and guest molecules were changed.Citation115
Gold Nanoparticles (AuNPs)
AuNPs are the most stable, fascinating, and attractive metal nanoparticles for nanotechnological research because of their stability, uniformity, biocompatibility, etc,Citation17,Citation116,Citation117 and also for their optical, electronic, magnetic, and supramolecular properties.Citation118,Citation119 Additionally, AuNPs can conjugate many biological substances and form active complexes to provide targeted drug delivery.Citation120 Also, due to their very small size (nanoscales), AuNPs have a larger surface area. Consequently, the large surface area allowed better contact of AuNPs containing the bioactive molecules with the biological membranes and improved their bioavailability.Citation17,Citation20,Citation121,Citation122 Regarding their application in medicine, AuNPs are utilized for the early detection and diagnosis of different diseases, as well as in their treatment.Citation123,Citation124
Stabilized noble metal NPs have been produced using one of the two main techniques, named bottom-up and top-down techniques (). In the case of “top-down method” AuNPs can be produced from their constituent metals by the aid of microscopic machine and converted into nanoscale dimensions. Whereas in the bottom-up method, AuNPs can be produced from the gold atom solution using different methods as we will discuss below. There are three main methods for AuNPs synthesis including chemical, physical, and biological methods.Citation125 In the chemical methods, the synthesis procedure involves the decomposition or precipitation of gold via the reduction of tetrachloro-auric acid (HAuCl4) as a gold precursor with the aid of a suitable reducing agent such as phosphorus,Citation126 citric acid,Citation127 sodium borohydride (NaBH4), or trisodium citrate.
The chemical procedure is the most famous and widely utilized technique because of its reagents’ availability. This kind of the selected reducing agent affects the particle size of the produced colloids, which ranges from 1 to 100 nm.Citation128 For example, sodium citrate was utilized to produce AuNPs with a size range of 12–64 nm, depending upon the citrate/HAuCl4 ratio.Citation127,Citation129 Also, the smaller particles (3–6 nm) are often prepared by using NaBH4 or a mixture of tannic acid and trisodium citrate.Citation128,Citation130 The chemical method includes four main categories: Turkevich, Brust, Seeded growth, and Miscellaneous methods. The first two categories produce spherical AuNPs, while the other two categories generate various shaped (anisotropic) AuNPs (ie, rods, cubes, tubes, etc). Specifically, the Turkevich method includes the direct reduction of Au3+ ions into Au0 atoms using a reducing agent followed by stabilization using a stabilizing (capping) agent to generate a size range of 10–20 nm. Brust method includes the incorporation of the Au3+ ions into an organic solvent using a phase transfer agent (ie, Tetraoctylammonium Bromide (TOAB)) before the strong reduction using NaBH4 (NaBH4) to generate nanospheres of 1.5–5.2 nm. Seeded growth (anisotropic growth of AuNPs) is the most widely used technique that includes the strong reduction of the Au salt (ie, by using NaBH4) to form seed particles, which are then added to other metal salt solution (seed solution) in presence of a weak reducing agent (ie, ascorbic acid) + structure directing agent to generate various shaped AuNPs. Miscellaneous methods include ligands digestion using thiols, amines, silanes, phosphines, etc, or by ultrasonic waves, microwaves, laser ablation, solvothermal method, electrochemical, and photochemical reduction to generate mono-disperse AuNPs from poly-disperse AuNPs.Citation125,Citation131
Alternatively, different physical methods are utilized for the synthesis of anisotropic AuNPs.Citation132 One of these methods is the formation of gas-phase gold via thermal evaporation of gold under high vacuum. Then, the gas was allowed to be deposited on the surface of a single crystal substrate.Citation133 Another physical technique was utilized to produce gas-phase Au clusters via magnetron sputtering of a high-purity gold target with argon ions. To obtain uniformly dispersed AuNPs, the sputtered gold atoms were allowed to be deposited on the surface of a moving powder support material such as Aluminium Oxide (γ-Al2O3). Separation of the loaded gold was achieved by using 5 mL of aqua regia (3:1 mixture of hydrochloric acid and nitric acid) that dissolved Au from the samples. Then, γ-Al2O3 was separated and washed 3 times with deionized water.Citation133,Citation134 Also, an ordered arrangement of AuNPs on an inclusion compound of α-CD–dodecanethiol produced by magnetron sputtering. Here, a 2-D hexagonal lattice was formed from -SH groups of the guest molecule dodecanethiol linked with α-CD interact with and stabilize AuNPs, which consequently arranged them in an ordered way.Citation118 Additionally, femtosecond laser ablation, an environmentally friendly alternative technique for producing monodispersed AuNPs, has also been proven since it can be applied at ambient conditions without any possible chemical contamination.Citation135 The advantages of physical techniques over other preparation methods include that the process is friendly to the environment since the excess gold can be recovered from the chamber. Moreover, no reducing agents have been utilized for the production. Hence, there is no solvent or precursor contamination.
Since physicochemical methods require and/or produce toxic byproducts of AuNPs, which are not suitable for biological applications, the recent trend is to modify AuNPs by using eco-friendly biological reagents for several bioapplications.Citation136,Citation137 Green synthesis (Biosynthesis) of NPs is taking place using three main bio-strategies, including the use of biomolecules, microorganisms (bacteria and yeast), algae, fungi, and plant constituentsCitation138 that are known to interact with the inorganic metals and could be used in bioleaching of minerals.Citation131 displays certain studies on the common methods followed for the preparation of AuNPs.
Table 5 Different Preparation Methods Were Utilized for AuNPs Synthesis
Noteworthy, the properties (chemical, physical, or biological) of AuNPs are completely different compared with the corresponding gold ions. For example, we know that the color of bulk gold ions (AuCl4) is yellow. This color was changed from yellow to red upon the addition of a reducing agent such as sodium citrate with reflux, indicating the conversion of gold ions to NPs. Also, the color will be changed to dark grey-blue upon the addition of an excess reducing agent indicating the formation of aggregate or suspension of different sizes, dimensions, and plasmon resonance characteristics.Citation162 Besides, other properties can be affected by size and shape, including electromagnetic, optical, and catalytic properties.Citation163 Accordingly, the scientists were motivated to do their best to create a novel synthesis route that allows better size and shape control.
The surface plasmon resonance (SPR), the electron oscillation caused by electromagnetic radiation of the gold metal influences the photochemical characteristics of AuNPs, including the light scattering/absorption, photochemical conversion, and the enhancements of the electric field. The SPR depends basically on the AuNPs’ geometries. The spherical NPs have one SPR value (520 nm). Also, it was reported that upon changing the diameter of NPs from 10 to 100 nm, it was found that the SPR value was shifted by 50 nm.Citation164 Also, SPR values were influenced markedly by changing the shape of the synthesized NPs, since the gold nanorods have two SPR values, depending on the electron oscillation along with two different directions. It was 520 nm in the transverse SPR since the oscillation occurs along the short axis. While the value ranged from (600–1100) nm, in longitudinal SPR (ie the oscillation of electrons takes place along the long axis).Citation165
Impact of β-CD on AuNPs Properties and Applications
Several AuNPs, capped with thiolated CDs, were reported by different groups.Citation166,Citation167 Most of these reports discussed the construction and characterization of CD-AuNPs. However, the safety and efficacy of NPs have limited entrapment efficiency (with classical water emulsion polymerization procedures) which, consequently, requires the excessive administration of polymeric material.Citation168
Herein, our review article discusses the incorporation of β-CD in AuNPs and highlights its applications in different pharmaceutical and biomedical fields. Generally, several applications of CDs (as illustrated in ) have been found attractive in the formulation of AuNPs, including the spontaneous formation, stabilization, and size control, the increase in loading capacity and bioavailability of the preparation, the improvement of catalytic enzymes, and the construction of functionalized electrodes, etc.Citation15,Citation169
Stabilization and Size Controlling Role of CDs
It was reported that CD molecules have an important role in the control of nanoparticle size. For example, the size of CD-derived poly(isobutyl cyanoacrylate) nanospheres depends on CD type since the obtained NPs were 87 nm and 103 nm in the cases of HP-β-CD or HP-γ-CD, respectively. Furthermore, the concentration of the utilized CD can affect the size of the modified NPs. Upon increasing the concentration of HP-β-CD from 0 to 12.5 mg/mL in the polymerization medium, the size of the modified NPs decreased from 300 to 50 nm. Also, it was noted that the values of zeta potential (Z-potential) of the modified particles decreased from -40 mV to 0 mV.Citation170 Noteworthy, the reduction of the value of the Z-potential is detrimental for the suspension since at low values of Z-potential the suspension becomes unstable, and the flocculation occurs.
Regarding the stabilization of the modified NPs, it was reported that CD molecules act as a steric stabilizer during the polymerization process of poly(isobutyl cyanoacrylate) in an aqueous medium.Citation169
Cyclodextrin molecules bind directly to the surface of AuNPs via SH groups, and the AuNPs support the monolayer assembly of CDs.Citation171 The modified AuNPs can be affected by the type and concentration of the employed CD. The smallest particles were obtained using gamma-CD (γ-SH-CD), followed by both β-SH-CD and α-SH-CD. Also, the increase in the CD/AuCl4 ratio was accompanied by a decrease in NPs particle size. CD-modified AuNPs were constructed directly by adding either Per-6-thio-β-CD,Citation166 or Mono-6-lipoyl-amido-2,3,6-O-per methyl-β-CDCitation172 to AuNPs solutions. The role of CDs in these modified systems was to control the aggregation of particles via the inclusion complexes formation.Citation173 Similarly, the polymerized CD was utilized to conjugate AuNPs and reported by another research group.Citation174,Citation175 Besides, native cyclodextrins have been used and described in the preparation and characterization of AuNPs to investigate their impact on the size distribution of AuNPs. It was noted that the presence of CDs significantly reduced the size of AuNPs. The size of AUNPs was reduced from 12–15 to 4–6 nm by increasing the CD concentration. In addition, the kind of CD molecule affected the size of NPs since it was observed that the size value was 5.4 nm, 4.8, and 4.3 upon using β-CD, α-CD, and γ-CD, respectively.Citation120 Some common characteristic effects on the modified AuNPs by their coupling CDs are listed in .
Table 6 Effect of CD on AuNPs Characteristics
Enhancing the Loading Capacity and Bioavailability
Cyclodextrins can include and solubilize hydrophobic molecules. The conjugation of AuNPs with CD molecules helps increase the hydrophobic cavities available for loading the bioactive agents. Therefore, the increase in CD number conjugating AuNPs increased the loading capacity.Citation170,Citation178 Adeli and his coworkers reported the surface conjugation of gold NPs carriers with cyclodextrins/PEG poly-rotaxane, as a hybrid nano-system for improving solubility, bioavailability, and decreasing non-specific uptake of anticancer drugs (cisplatin and doxorubicin).Citation176 The modified CD nano-system allowed the controlled and targeted release of anticancer drugs, the enhanced permeability and the retention effect of both drugs, which were conjugated to AuNPs-hybrid nano-systems, which were endocytosed by cancer cells and, consequently, allowing the internally controlled drug release.Citation176
In addition, the attachment of polyrotaxanes on the surface of AuNPs increased the internalization capacity of the modified system and anticancer drugs into the cells.Citation176,Citation179,Citation180 The modified new system composed of AuNPs core and polyrotaxane shell has dual advantages regarding the delivery of anticancer drugs. The nano-system can cross the cell membranes rapidly and decrease the anticenter’s adverse effects. Also, the system comprising AuNPs can kill cancer cells via their photothermal properties.
Similarly, the inclusion ability of CD-coated AuNPs was utilized to construct a cytotoxic nano-drug system.Citation178 The idea was achieved via the inclusion complexation between β-CD and adamantane, end-capping oxo-platin (a prodrug of cisplatin). The results showed that the modified system still has anticancer efficiency with higher stability compared with free drugs.
Sierpe and his coworkers reported a ternary nano-system formulated by mixing AuNPs solution with the solution of a β-CD-loaded psychoactive drug (phenylethylamine). Full characterization of the constructed nanosystem was carried out, and the results showed that the modified system would allow the release of the loaded drug after photothermal laser irradiation.Citation181
Park and his coworkers reported a new nano-assembly that was constructed from the coating of AuNPs with CDs (AuNP/CD). The modified system was utilized to load or encapsulate an anticancer drug called β-Lapachone.Citation182 Besides, the incorporation of CDs in the gold nano-system was utilized as host cavities for the inclusion of targeting moieties called anti-epidermal growth factor (Anti-EGFR) receptor antibodies. There are two kinds of cancer cell lines, MCF-7 and A549. The results showed that the intercellular uptake and the extent of cellular inhibition of AuNPs-loaded drug were higher in the case of the system containing the targeting ligand than that does not contain the targeting ligand.Citation182
Gimenez and his co-workers report the modification of AuNPs by SH-β-CD. The modified nano-system was utilized to incorporate violacein as an anticancer agent via inclusion complexation with CD cavities to improve its solubility and bioavailability. The results illustrated that the loaded drug exhibited higher cytotoxic potential (based on MTT assay) on human leukemia cells (HL60) and lung fibroblast cells (V79) in comparison with the free drug.Citation183 lists the role of CD complexation in enhancing the characteristics of the loaded bioactive molecules.
Table 7 Role of CD Complexation in Enhancing the Characteristics of the Loaded Bioactive Molecules
Role of CD-AuNPs in Drug Targeting/Delivery
The presence of hydrophobic cavities in the CD structure makes it possible to carry certain hydrophobic materials or bioactive agents.Citation184 CDs and their derivatives, formed via structural modification of either primary or secondary face of parent CDs with aliphatic hydrocarbon chains, have been considered as potential excipients for different applications, including the production of nanospheres or nano-capsules of high loading capacity providing efficient targeting delivery.Citation185,Citation186 β-CD derived nanoparticles were reported for loading and release of Tamoxifen.Citation184 The results indicated that the drug was either included in CD cavities or entangled in the aliphatic chains, allowing the release of the drug in a controlled-release manner.Citation184 However, the application of β-CD as a drug carrier is restricted by its low aqueous solubility, which could be enhanced by structural modification of the parent CD and the design of new formulations such as formulations of β-CD-AuNPs.
AuNPs exhibit applications as transfection vectors,Citation187 siRNA and DNA-binding agents,Citation167,Citation188 protein inhibitors,Citation189 and spectroscopic markers.Citation190,Citation191 A monolayer of thiolated CD derivatives, with different spacer lengths between the thiol termination and CD cavities, were adsorbed on gold films and described by many researchers.Citation192,Citation193 The thickness and packing densities of the molecular monolayer as well as the orientation of the CD cavities and the outermost layers of these systems were controlled by changing the number of thiol groups and the length of the alkyl chain. The substitution of all secondary hydroxyl groups in the β-CD rings by S-H groups afforded the synthesis of highly water-soluble AuNPs with the outermost layer formed by β-CD cavities. Using a spacer group between the S-H terminations in the preparation of NPs is novel. It could also increase the number of adsorbed β-CD molecules and improve particle size control. One of these modified AuNPs is capped with β-CD-alkyl thiol derivatives, mono [6-deoxy-6-[(mercapto-hexamethylene) thiol]]–β-CD.
The inclusion complexation of certain hydrophobic drugs such as violacein, an antitumor drug, in β-CD-S(CH2)6-S-AuNPs afforded violacein transfer to an aqueous medium.Citation183 Cell viability measurements indicated that the system violacein-modified NPs maintained the violacein cytotoxicity on myeloid leukemia cells (HL60) and reduced the activity towards the normal cells (V79), ie, it became less cytotoxic on normal V79 cells compared to other violacein/β-CD complexes (). Hence, this novel modified-NPs system enhanced the selectivity and targeting ability toward tumor cells.Citation183
Figure 6 Formation of the ternary system which is composed of the inclusion complexation of guest drug (eg, anticancer drug) in the β-CD cavity and then conjugation with gold nanoparticles (β-CD-S(CH2)6-S-AuNPs) for drug delivery.
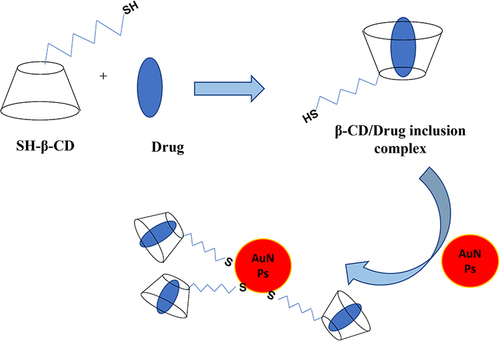
The conjugation of AuNPs by CD molecules occurs during the reduction process, using Na-borohydride in dimethylsulphoxide solvent. The obtained AuNPs-CD gold can be utilized as multisite hosts for binding other guests in the solution such as 1-adamantane and ferrocene methanol.Citation173
Generally, a great interest has been directed toward developing and modifying nanomaterials as novel drug delivery systems.Citation194 The inorganic AuNPs were considered promising drug delivery and targeting carriers, especially for cancer treatment and phototherapy.Citation119,Citation195 However, the application of such unmodified NPs for drug delivery is limited by the quick release of the loaded drugs from the surface of AuNPs, as well as their considerable cytotoxicity.Citation196 To overcome this limitation, the chemical modification of such NPs to relatively sustain the drug release is required. The modification of AuNPs with CD molecules is a famous example and had great interest from researchers in the last decade.Citation197 The modified complex system has the advantages of both CD (inclusion ability of different hydrophobic drugs, improving solubility and bioavailability and stability of the included drugs)Citation198 together with the advantages of AuNPs (increasing the drug selectivity and targeting to a specific site of action via the addition of targeting molecules)Citation196,Citation199 making the combined new system as an attractive and promising approach for drug delivery.Citation171 Curcumin (CUR), a drug utilized for the prevention and treatment of osteoporosis, was loaded on AuNP-CDs by Heo et al.Citation200 It was noted that the modified NPs were spherical, ranging from 20 nm to 40 nm. Also, the obtained Au-CD loaded-CUR showed a better effect at lower concentrations compared with either the plain drug or the unmodified AuNPs.
Similarly, curcumin as an anticancer was loaded on the CD-NPs system via inclusion complexation and reported by Möller et al.Citation201 The results indicated that the modified system loaded with the drug exhibited a higher cytotoxic effect compared with the free drug. The complete inhibition of cancer cells was achieved within a few hours of incubation.
Baicalin (BC), as an anticancer cancer drug, has low cancer cellular uptake.Citation200 Therefore, AuNP@CDs were utilized as a drug carrier for (BC). The results illustrate that the modified AuNP-CD-BC solved the targeting problem since they inhibited cellular growth and induced apoptosis of all targeted cells upon using 100 mM drug concentration.Citation202
Similar findings were obtained and reported previously on the high activity and selectivity of the modified paclitaxel (PTX)-loaded AuNP@CDsCitation203 and β-lapachone AuNP@CDsCitation182 for the treatment of cancer. Shi and his coworkers studied the impact of CD-coated AuNPs as a delivery carrier for a prodrug of cisplatin (Adamantane–Oxoplatin conjugate) to produce a cytotoxic nanodrug in vivo.Citation178 The inclusion complexation between CDs and adamantane moieties was utilized for the construction of a nanodrug with a stoichiometric ratio of 1:1. The results indicate CD decorating AuNPs play an important role in the increase of the photothermal potential of AuNPs as anti-tumor.
Silva and co-workers utilized the inclusion complexation of β-CD, coating AuNPs, for loading and controlled release of methotrexate (MTX) as an anticancer drug.Citation204 CDs enhanced the solubility and decreased the side effects of the drug. The drug was allowed to release from the modified system via laser irradiation of the cells, causing laser light absorption by AuNPs. Then, the absorbed light was transformed into heat dissipated into the environment. Consequently, this allowed the controlled release of the included drug from CD cavities. Afterward, the released MTX gives its cytotoxic effect. MTT assay was utilized for the investigation of HeLa tumor cell viability. The results showed that the irradiated cells with the modified ternary system caused huge cell growth inhibition. lists the applications of CD-NPs for drug delivery and targeting.
Table 8 Different Reported Drug Targeting/Delivery Applications of CD-AuNPs Complexes
β-CD-Modified AuNP Aggregates
One of the properties of AuNPs is their ability to form nano-aggregates of specific surface plasmon resonance (SPR).Citation207 In fact, the random unintended aggregation of AuNPs is undesirable in general. However, the intended and planned aggregate formation was the subject of plenty of research articles in the last decade. In our review, we focused on some of these pharmaceutical and biomedical applications. AuNPs aggregates were formulated by different approaches including metal–ligand interaction,Citation208 ion–pair interaction,Citation209 hydrogen bonding,Citation210 and hydrophobic interaction via host-guest inclusion complexationCitation166 etc. There are several research articles discussing the formulation and applications of AuNP nano-aggregates, but only a few articles focus on aggregation reversibility and the factors that may affect such property, including pH change,Citation211,Citation212 temperature change,Citation213 and biomolecular recognition.Citation214 For AuNPs aggregation, it is important to conjugate the nanoparticles with various materials, including proteins,Citation215 DNA,Citation216,Citation217 synthetic polymers,Citation213 fullerenes,Citation217 and cyclodextrins (CDs)Citation218.
Cyclodextrins are preferable over other materials because of their low toxicity, and high solubility. We study the complexation between β-CD, conjugating AuNPs, and diazo (guest).Citation219 Diazo molecules have a double azobenzene structure, acting as a crosslinking agent to bind the individual nanoparticles together (). It was observed that the color of the gold colloid was changed upon aggregation into purple, accompanying the shift in the absorption spectrum. Also, the average number of AuNPs, in the constructed nanoaggregate depended on the amount of both CD and diazo. The dissociation of the formulated gold aggregates was realized via the addition of α-CD, which acts as a competitive host to β-CD.
Figure 7 The representation of the aggregation and the competitive dissociation of smart AuNPs-β-CD via the addition of either guest molecules (ie, PEG-Ad or diazo) causing aggregation, or α-CD as compotator host molecules.
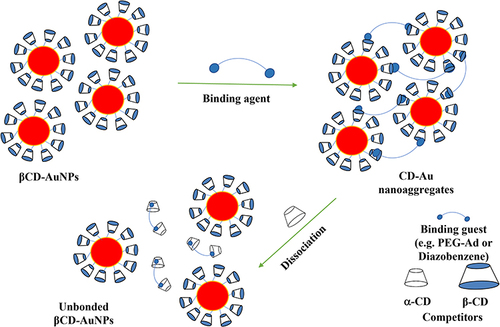
Hence, α-CD formed new inclusion complexes with the utilized guest molecules by capturing them from the cavities of β-CDs. Interestingly, the recovery and self-assembling of AuNPs-β-CD were achieved upon the addition of the guest molecules again. Therefore, the modified system is smart and reversible, which is controlled by the addition of either β-CDs or guest molecules.Citation219
Also, the aggregate of AuNPs which were conjugated with thiolated CDs (SH-CD) was performed in an aqueous solution via the inclusion complexation between 1,10-phenanthroline (guest molecules, utilized as molecular retractor which binds the nanoparticles together) and BCD (host molecules). The modified system was characterized by different analysis techniques including fluorescence, FT-IR, and UV spectroscopy. The results showed that the aggregation process depends on the concentration of both guest and CD molecules.Citation220
Role of Cyclodextrin in Gene-Drug Delivery (DNA Concentration)
Cyclodextrins are helpful in gene delivery applications because of their binding affinity to nucleic acids and their ability to reduce the cytotoxicity of other gene carriers.Citation221,Citation222 CD-containing polymers were demonstrated by Davis for DNA aggregation. The modified system is a highly biocompatible matrix for recombinant adenovirus-mediated gene delivery to local wound sites.Citation223 Another research article showed the utilization of CDs in the improvement of adenoviral-mediated gene transfer into the jejunum of rats.Citation224 CDs can bind various molecules via the formation of inclusion complexes.Citation225 Therefore, CDs have been used to construct supramolecular aggregates acting as receptors in biological technology. In addition, cyclodextrins are considered effective absorption and gene-delivery enhancers. An earlier study showed the utilization of CDs in improving adenoviral-mediated gene transfer into the jejunum of rats.Citation224 The obtained improvement was attributed to CD molecules in the gold NPs, which enhanced the viral binding and subsequent internalization into the host cells. Moreover, the derivatization of CD at the 6-position was reported for the improvement of plasmid DNA (32P-labeled pDNA) complexation capacity and consequent cellular uptake efficiency as in the case of COS-7 cell transfection.Citation226
It was reported that adamantane (Ad) forms perfect inclusion complexes with β-CD. This kind of complexation has been utilized in the construction of self-assembling nanoparticles.Citation227 As a nonviral vector, the CD-based polycations have been synthesized to deliver DNA.Citation223,Citation228 These polycationic polymers can be self-assembled with DNA molecules via electrostatic interactions to form nano-assembly which are called “polyplexes.” The modified NPs exhibited higher cellular internalization of the gene compared with unmodified DNA.Citation229,Citation230 The modified polyplexes, based on CD, were specifically immobilized on Ad-functionalized surfaces via inclusion complexation. Compared to PEI-modified NPs, CD-PEI NPs showed noticeably greater adsorption on Ad surfaces. Additionally, compared to single CD molecules, the combined CD-PEI NPs have a greater binding affinity Ad surface due to multivalent interactions. On the other hand, the immobilized NPs, illustrated in , were examined by AFM and fluorometric spectroscopy.Citation231
Figure 8 Schematic representation of CD-AuNPs immobilization on Ad substrate via inclusion complexation for pDNA concentration according to the following steps. (1) Ad-modified self-assembled monolayer (SAM) gold substrate; (2) Immobilization of PEI-pDNA polyplex NPs on the modified SAM; (3) pDNA using Heparane; (4) Immobilization of β-CD-PEI-pDNA polyplex NPs on the modified SAM; (5) pDNA using Heparane.
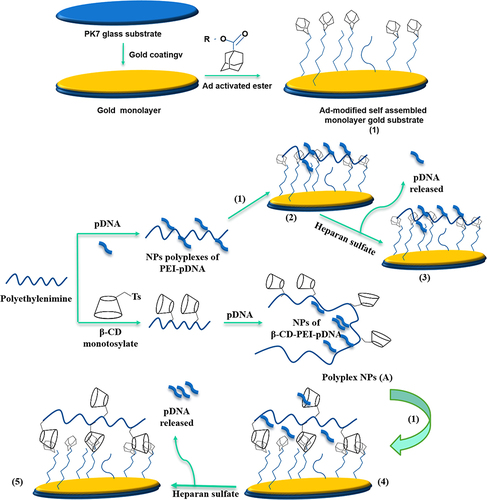
Oligo (ethylenediamine)-β-CD-modified AuNP (OED-CD-NP) was constructed as a non-viral vector for DNA.Citation232 The aggregation behaviors of these constructed systems with DNA were characterized by several microscopic and spectroscopic techniques. The obtained results indicated a direct relationship between the morphological feature of the modified aggregate and the initial concentration of both OEA-CD-NPs and DNA. The transfection efficiency of the modified system was evaluated by MTT assay using MCF-7 cells. The obtained results indicated the successful delivery of noroviral vectors into the cancer cells. In contrast, the system free of CD molecules had a weak bond with the DNA receptor, indicating the importance of CD in the modified system.Citation232
Several AuNPs-capped CDs have been reported by different groups for application in gene delivery.Citation17,Citation233,Citation234 These studies focus on the construction and characterization of CD-AuNPs. The utilization of cyclodextrin-containing cross-linked matrixes for DNA aggregation has been established and reported by Davis and coworkers.Citation223 The modified cyclodextrin-based system was regarded as a highly biocompatible matrix for gene delivery. displays the effect of CD on gene delivery using AuNPs.
Table 9 Effect of CD on Gene Delivery Using AuNPs
Role of CDs in Preparation and Improvement of Catalytic Enzymes
Cyclodextrin-gold NPs were utilized to enhance the loading efficiency, control the release, and targeting of enzymes. Accordingly, different studies presented that CD-AuNPs are considered attractive and promising building blocks for effective artificial enzyme model engineering.Citation236
For example, an artificial enzyme model was developed via the complexation of metal catalytic centers with β-CD modified AuNPs. Firstly, AuNPs were coated with SH-CD molecules. Secondly, the constructed CD-AuNPs were utilized as a backbone to install metal catalytic centers via self-assembling or inclusion complexation between adamantane-modified copper-triethylenetetramine complex and β-CD cavities, which act as hosts for adamantane guest molecules.Citation236 The results showed that in the prepared system, enzyme has catalytic behaviors, which were considered as an esterase mimic enzyme. Besides, the prepared enzyme exhibited excellent hydrolysis ability for catalyzing the breakdown of an active ester 4,4‘dinitro-diphenyl carbonate.
Similarly, the host-guest supramolecular interactions were utilized by other researchers to formulate enzyme nano-catalysts.Citation237,Citation238 The immobilization of enzymes (proteins) on the CD-containing AuNPs system was achieved via the inclusion complexation of one or more of the protein bulky moieties and the hydrophobic cavities of CD. Alternatively, the loading of the enzyme can be done by linking a hydrophobic moiety, such as adamantane molecules to the enzyme. Hence, the loading can be carried out via the inclusion of complexation between Ad molecules and CDs.
Additionally, the Ad/CD inclusion complexation was utilized for the formulation of nanodevices acting as biosensors. The modified system was conjugated with superoxide dismutase and catalase enzymes. The conjugation or loading of these enzymes was carried out by decorating these enzymes with adamantane moieties, which will be included in the CD cavities. The results of the analysis indicated the successful construction and development of the biosensor, based on Ad-modified bi-enzymatic nanodevice.Citation237,Citation239 The same method was utilized for the formulation of uni- and bi-enzymatic nanocatalysts reported by another research group.Citation81,Citation238 Ad-COOH was utilized for making hydrophobic capping of catalase enzymes to be immobilized on β-CD-AuNPs via supramolecular associations, and then the enzymatic nano-catalyst was further constructed via co-immobilization of β-CD-modified-dismutase (copper/zinc superoxide dismutase enzyme).Citation81 The results showed that the modified system still retained about 35% and 73% of initial specific activity for dismutase and catalase, respectively. It was also observed that the thermal stability was improved by the process of co-immobilization. Additionally, the bi-enzymatic immobilization with catalase on metal nanoparticles improved the resistance efficacy of superoxide dismutase by 90-fold to the inactivation by H2O2 (at a concentration of 100 mM).Citation81
Recently, thiolated β-CD-AuNPs supramolecular associations (water soluble) have been utilized to immobilize native or Ad-modified enzyme structures through host-guest interactions. For example, the enzyme L-phenylalanine dehydrogenase (PhDH) modified by Ad-carboxylic acid (Ad-PhDH) was immobilized on β-CD-AuNPs via supramolecular interactions. The results revealed that the formulated enzyme retained its catalytic activity and affinity to the substrate.Citation239 Noteworthy, AuNPs, and CD derivatives have catalytic capacity, which mainly comes from guest molecules.Citation171 lists the selected studies on some CD-AuNPs complexes used for the preparation and improvement of catalytic enzymes.
Table 10 Selected Studies on Some CD-AuNPs Complexes Used for the Preparation and Improvement of Catalytic Enzymes
Role CD-AuNPs in the Formation of Self-Assembling Molecular Print Boards
Owing to their high binding strength and reversibility,Citation111,Citation243 the inclusion complexation has been utilized for the construction of receptor-functionalized molecules and nanoparticles called molecular print boards. The concept of “molecular print boards” was introduced by the Reinhoudt group,Citation244 which are β-CD self-assembled mono- and multilayers on silicon oxide or gold substrates that possess supramolecular host properties.Citation245,Citation246
The inclusion complexation between adamantane (Ad) and cyclodextrin cavities was utilized for the construction of physical nano assemblies. Huskens and his co-workers reported that Ad-end capping polypropylene imine dendrimer Ad-PPI), ranging from 4 to 64 Ad moieties, act as guest molecules, which will be included in the cavities of CD-decorating AuNPs (host molecules)Citation247 to form self-assembled monolayer molecular print board, as illustrated in (). Upon using multiple inclusion complexes between CD and Ad (Ad-CD) stable assemblies can be obtained of high strength. The modified structural surfaces can be utilized for different nanotechnological and electronic applications. Also, the modified systems can be utilized as molecular print boards on which the molecules can be firmly placed.Citation247
Figure 9 Chemical structures of adamantly functionalized PPI dendrimers (Ad-PPI) and their formation of inclusion complexes with cyclodextrins to construct water-soluble assemblies (A); the formation of a monolayer of these modified assemblies on a gold substrate via adsorption (CD-AuNPs monolayer) (B); and formation of multilayer CD-assemblies on gold (C). Reproduced with permission from Copyright 2002 Wiley VCH GmbH.Citation247
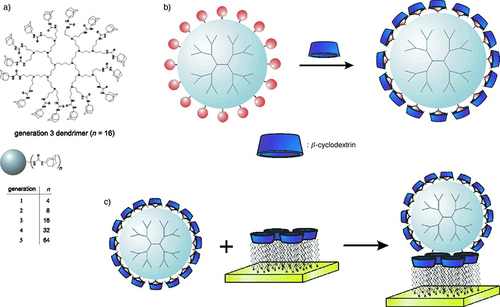
Similarly, a novel multi-layered, self-assembled organic-metal NP was constructed via layer-by-layer assembly. The modified nano-assembly was characterized by UV and SPR spectroscopy as well as atomic force microscopy. The results indicated a direct relationship between the number of bilayers and the extent of absorption. Various structures of multiple supramolecular interaction assemblies might be obtained via such protocols. This provides a general pattern for nanofabrication via integrating several components from inorganic, organic, metallic, and bio-molecular reagents while maintaining the specificity of supramolecular interfacing.Citation248 Previously, this group of researchers also studied the irreversible precipitation of dendrimer/CD-AuNPs aggregates induced by the complexation of a CD-modified AuNPs solution with adamantyl dendrimers.Citation249
Other research groupsCitation250,Citation251 demonstrated nanostructures of supramolecular layer-by-layer assembly of 3D multicomponent nanoparticles. Generally, there are two classes of nanofabrication methods: “top-down” and “bottom-up.” There are different\common techniques utilized in the case of the “top-down” class method, including directed self-assembly, supramolecular assembly, and probe lithography techniques. On the other hand, soft lithography, lithography, and evaporation techniques are common examples of the ‘bottom-up’ class (). Layer-by-layer assembly and nanoimprint lithography (NIL) were used as tools of patterning that fabricated patterned β-CD-SAMs complexes and retained nanoparticles on the substrate. In addition, a combination of metallic and inorganic NPs with organic molecules built-up multi-layered and multicomponent NP arrays ie, CD-conjugated gold NPs (CD-Au, d ~3 nm), CD-coated silica NPs (CD-SiO2, d ~350 nm), ferrocenyl-coated silica NPs (Fc-SiO2, d ~60 nm), and adamantyl-end-capping poly(propylene imine) dendrimers (G1-PPI-(Ad)4) (generation 1). The gathering of guest- and host-functionalized NPs appeared in a layer–by–layer alternating assembly ().
Figure 10 Schematic representation of the multicomponent nanostructures construction using (A) CD-Au and Fc-SiO; (B) The nano-assembly from small NPs to large NPs; and (C) nano-assembly from large NPs to small NPs. The artwork was reproduced from MDPI according to permission via license: CC BY 4.0.Citation251
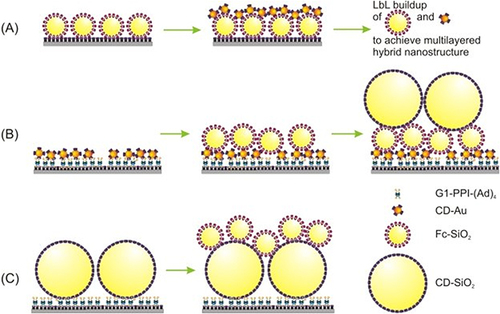
Furthermore, the ordering effects of the steps of nanoparticle assembly including, small-to-large and large-to-small nanoparticle assembling were compared. Interestingly, supramolecular LbL assembly provided control nanostructures over their height in the nanometre range, whereas NIL provided confinement nanostructure in the x and y directions. Full integration of these methods, therefore, lead to the fabrication of arbitrary shape from 3D nanostructures on substrates.Citation251
Also, multiple inclusion of the guests (adamantane end capping polyisobutene-alt-maleic acid polymer) into the cavities of β-CD self-assembled monolayers were constructed and reported by Crespo-Biel and co-workers.Citation252 The results indicated that the adsorption was irreversible, strong, and specific. Also, the polymer adsorption led to very thin polymer films on the surface as evidenced by both SPR spectroscopy and AFM. Moreover, the results indicated that there is no significant effect of the selected hydrophobic moieties (nature and number) decorating the polymer as well as the polymer concentration on the adsorption capacity.
Role of CD-AuNPs in the Fabrication of Supramolecular Functionalized Electrodes and Biosensors
Another novel application of β-CD-capped gold NPs is the development of a supramolecular-functionalized redox electrode acting by the physical assembly between β-CD coating AuNPs (β-CD-AuNPs) and ferrocene moieties coating indium tin oxide (Fc-ITO)Citation253 Cyclic voltammetry and AFM evaluated the immobilization of β-CD-AuNPs on the Fc-ITO vehicle. In addition, cyclic voltammetry confirmed the supramolecular characteristics of the immobilization process. CD-modified electrodes exhibited enhanced electrocatalytic and electroanalytic effects compared to the unmodified (CD-free) Fc-ITO-electrode toward the ascorbic acid.Citation253
CDs possess a special structure that enables them to generate polymers of various structures that perform different functions via several reactions,Citation199 as well as in synergy with inorganic nanoparticles.Citation254 Furthermore, entrapping of drugs via complexation with CDs improves their stability and bioavailability.Citation198
Interestingly, immersing the modified electrode in a saturated solution of adamantane carboxylic acid (Ad-COOH) leads to the disruption of the modified system. This may be because the adamantane moieties have a higher affinity to CD cavities and can form highly stable complexes with β-CD compared to Fc, causing the release of β-CD-AuNPs from the electrode surface. The obtained findings confirm that the immobilization mechanism depends on the previously described inclusion complexation hypothesis.Citation253
Bisphenol A (BPA) is an organic material used to manufacture polycarbonate plastics and epoxy resins, which are subsequently utilized for coating the internal surfaces of cans. Bisphenol A can disrupt the human health endocrine system. Therefore, it is important to detect its amount in the environment. A new molecular imprinted system was constructed from acryloyl-β-CD, acrylamide (AAm), and N, N-methylene bis-acrylamide (MBAA). Then, it was loaded with bisphenol, as illustrated in. The modified system was utilized as a sensor for the detection of bisphenol.Citation155 In this concern, bisphenol can be detected potentiometrically by monitoring the change in the color of the hydrogel system from blue to red. The modified system can detect bisphenol at a concentration of 0.5 mM.Citation155
An ultrasensitive detection of bisphenol A was also described and previously published.Citation255 The detection was carried out by utilizing an electrochemical biosensor, constructed from graphene oxide-AuNPs decorated with β-CD molecules. Then, the system was heated and electrodeposited to obtain a glassy carbon electrode. The modified biosensor was characterized by different analyses, including X-ray (XRD), UV, and SEM. The results exhibited a good detection limit of bisphenol (3 *10−9 M) with high stability.,Citation255
Similarly, an electrochemical biosensor was reported by Wu et al,Citation256 the modified system was constructed from the deposition of β-CD-AuNPs on stainless steel electrodes. The constructed system was utilized for the detection of low-density lipoprotein (LDL). In the modified system, β-CD was utilized as a binding receptor for LDL via different binding mechanisms including van der Waals forces, hydrophobic interactions, and hydrogen bonding. The results indicated that the modified β-CD-AuNPs biosensor exhibited a high sensitivity towards LDL.
Moreover, thiolated β-CD-AuNPs with reduced graphene oxide were modified and utilized for both acetaminophen and ofloxacin electrochemical sensing. The results showed that the detection limits for acetaminophen and ofloxacin were 3*10−8 M and 8*10−9 M, respectively. In addition to its wide detection range, the sensor showed high stability, and reproducibility.Citation198
Hydrogen peroxide (H2O2) biosensors were synthesized by incorporating AuNPs into the cyclodextrin/chitosan hydrogels. Noteworthy, H2O2 shares in mitochondrial electron transfer reactions. Also, this kind of oxygen species is considered the most stable one which can penetrate the cell membrane and damage the cell proteins. Hence, the detection of H2O2 level changes is very important. The results showed that the presence of AuNPs in such modified hydrogels improved the conductivity. Consequently, we improve the biosensor sensitivity with a low detection limit for H2O2 and its cell biocompatibility.Citation201
Moreover, a chemo-visual biosensor based on AuNPs-polymerized CD was constructed and reported by Lee et al for the detection of both cysteine and sodium diethyldithiocarbamate (SDDC).Citation202 The CD polymer acts as a stabilizer and reducing agent to form small AuNPs of about 15 nm. The results illustrated that the color of AuNPs was changed from red to blue upon attaching sodium diethyldithiocarbamate (SDDC) and cysteine as sulfated compounds. It was realized that the role of CD, as an amphiphilic structure, was the agglomeration of AuNPs and the entrapment of the sulfated compounds. The modified sensor detection limits were 0.05 and 0.07 µM for SDDC and cysteine, respectively. lists some selected studies on the fabrication and applications of supramolecular functionalized electrodes based on CD-AuNPs complexation.
Table 11 Selected Studies on the Fabrication of Supramolecular Functionalized Electrodes Using CD-AuNPs Complexation
Cyclodextrin as a Phase Transfer Agent
Ferrocene is an excellent guest molecule to be included in CD cavities via hydrophobic–hydrophobic interaction. The inclusion of complexation between ferrocene derivatives and CD molecules conjugating AuNPs has received much attention in recent years. The binding interactions of AuNPs were utilized to transfer the hydrophilic CD-modified NPs (hydrophilic) into less polar solution phases. Therefore, CD molecules play an important role in the phase transition, which acts as a phase transfer agent. From these ferrocene derivatives, both dodecyl ferrocene and hexadecyl ferrocene act as phase transfer agents, facilitating the solubility of AuNPs in an organic solvent, such as chloroform.Citation118 However, other ferrocene derivatives have a limited ability to act as phase transfer agents such as heptyl-ferrocene and propyl-ferrocene derivatives. This may be due to these derivatives having very limited amphiphilic properties. Therefore, the existence of CD molecules in the nano assemblies of gold could include the amphiphilic ferrocene moieties, which lead to phase transition via solubilization of the aqueous NP in chloroform.Citation118,Citation120 Also, it was proved that AuNP@CDs had the ability to reversible-phase transfer between the aqueous phase and the organic phase by UV and Vis light irradiation.Citation171,Citation265
Inclusion of Fullerenes
Fullerenes, special water-insoluble π-electronic systems, comprise lots of unsaturated carbon atoms linked to each other by single or double bonds to form hollow tubes or ball-like sphere structures. Fullerenes, also called Buckminster fullerenes” show various interesting magnetic, superconductive, electrical, and biochemical properties.Citation266,Citation267
Because fullerenes C60 (cage-like structure composed of 60 carbon atoms) is extremely water-insoluble, the water-soluble gold NPs conjugated with thiolated γ-CD hosts were utilized to solubilize such kind of fullerene via inclusion complexation between fullerenes and the cavities of CDs to form a large soluble nanoaggregates as illustrated in .
Figure 11 Schematic illustration of Fullerene tube or cylindrical structure (A), ball-like structure (B), and the formation of water-soluble nanoaggregates via the inclusion complexation between Fullerene and γ-CD-decorating AuNPs (C).
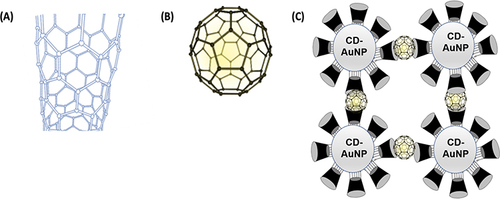
Additionally, because the method of fullerenes preparation is based on the thermal reactions of the appropriate carbon sources under various conditions,Citation268 the produced fullerenes are always obtained as a mixture of C60, C70, and higher homologs. Consequently, the separation of fullerenes from each other will be considered a challenge and a point of research for chemists. Water-soluble gold NPs modified with thio [2-(benzylamine) ethyl-amino]-β-CD are constructed and successfully used as a selective recycling extractor for the C60 fullerene analog. The mechanism of separation is based on the fact that β-CD can only form an inclusion complex with C60 (at a ratio of 2:1 CD:fullerene) but cannot form such inclusion complexes with either C70 or the higher fullerene molecules.Citation269 Thus, C60 can be easily separated from the mixture of C60 and others via inclusion complexation with CD-NPs to form large nano assemblies. Then, the included or captured C60 can be released via the addition of an inclusion compotator as adamantane molecules, as illustrated inCitation270 The reversibility of the modified smart system can be utilized also for the fabrication and designing of other functional supramolecular hybrid materials.Citation270 Similarly, a water-soluble nanoaggregate was constructed via the inclusion complexation between fullerene and α-CD.Citation271
Cyclodextrins can include linear polymers such as PEG and PPG to form CD-capped poly-rotaxanes, with specific lengths depending on the utilized polymers’ molecular weight.Citation272 There are various poly-pseudo-rotaxanes formulated from threading either native β-CD or L-tryptophan-modified β-CD onto the amino-terminated PPG chains of different Mwt or lengths.Citation273 Afterward, further assembling of the modified polysiloxanes with AuNPs leads to the construction of different supramolecular networks. The modified hydrophilic aggregates or networks were characterized using different analyses including UV spectroscopy, FT-IR, X-ray diffraction 1H NMR, TEM, and fluorescence spectroscopy.
The mechanism of polyurethane adsorption on the surfaces of AuNPs was carried out via the electrostatic interaction between the amino groups end capping polyrotaxanes and AuNPs. The results indicated that the sedimentation rate and the modified gold aggregates’ size mainly depended on the PPG chain lengths.Citation273
Interestingly, the modified Au-aggregates, involving many L-tryptophan moieties, have both water solubility. In addition, the modified system has the ability for capturing or loading of fullerene (C60 analog) in water solution (ie, improve the aqueous solubility of fullerenes). Moreover, the obtained nano assemblies loaded with fullerene exhibited not only water solubility but also a high ability for DNA cleavage under light irradiation, which can be a promising system having potential applications in material and biological science.Citation273
Conclusion
Due to its wide range of applications, nanotechnology is a promising and growing medical research area. AuNPs possess targeted drug delivery with low toxicity and ease of detection. Higher stability and drug loading compared with microparticles and liposomes. Cyclodextrins and their derivatives gain a great interest from researchers in the last decade due to their role in enhancing drug solubility and stability via the inclusion of complexation with hydrophobic moieties. In our article, we tried to highlight the chemistry and application of different cyclodextrins, especially the ability of inclusion complexes formation. Also, it focuses deeply on cyclodextrin’s role in improving drug loading capacity, stability, and size control of gold NPs. Moreover, in our review, we presented the reported roles of CDs in the design and applications of CD-conjugating gold nanoparticles (CD-AuNPs) in different biomedical fields, including drug delivery, antimicrobial, anticancer, and gene delivery and various targeted drug and gene delivery, preparation and improvement of catalytic enzymes, formation of self-assembling molecular print boards, and the fabrication of supramolecular functionalized electrodes and biosensors formation. Also, this review focused on applying nano-aggregates to separate fullerenes in an aqueous medium. The present review realized that the nano-systems composed of AuNPs-CD are very promising and open the door for further pharmaceutical and biomedical applications.
Disclosure
The authors declare that there are no conflicts of interest.
Acknowledgments
The authors extend their appreciation to the Deputyship for Research & Innovation, Ministry of Education, Saudi Arabia, for funding this research work through project number (QU-IF-1-2-1). The authors also thank Qassim University for its technical support.
References
- Villiers A. Sur la fermentation de la fécule par l’action du ferment butyrique. Compt Rend Acad Sci. 1891;112:536–538.
- D’Aria F, Pagano B, Giancola C. Thermodynamic properties of hydroxypropyl-β-cyclodextrin/guest interaction: a survey of recent studies. J Therm Anal Calorim. 2022;147(8):4889–4897. doi:10.1007/s10973-021-10958-1
- Saenger W. Cyclodextrin inclusion compounds in research and industry. Angew Chemie Int Ed English. 1980;19(5):344–362.
- Tian B, Xiao D, Hei T, Ping R, Hua S, Liu J. The application and prospects of cyclodextrin inclusion complexes and polymers in the food industry: a review. Polym Int. 2020;69(7):597–603. doi:10.1002/pi.5992
- Endo T, Nagase H, Ueda H, Shigihara A, Kobayashi S, Nagai T. Isolation, purification, and characterization of Cyclomaltooctadecaose (v-Cyclodextrin), Cyclomaltononadecaose (ξ-Cyclodextrin), Cyclomaltoeicosaose (o-Cyclodextrin) and Cyclomaltoheneicosaose (π-Cyclodextrin). Chem Pharm Bull. 1998;46(11):1840–1843.
- Rowe RC, Sheskey P, Quinn M. Handbook of Pharmaceutical Excipients. Libros Digitales-Pharmaceutical Press; 2009.
- Morin-Crini N, Fourmentin S, Fenyvesi E, et al. 130 Years of Cyclodextrin Discovery for Health, Food, Agriculture, and the Industry: A Review. Vol. 19. Springer International Publishing; 2021. doi:10.1007/s10311-020-01156-w
- Liu Z, Ye L, Xi J, Wang J. Cyclodextrin polymers: structure, synthesis, and use as drug carriers. Prog Polym Sci. 2021;118:101408. doi:10.1016/j.progpolymsci.2021.101408
- Del Valle EMM. Cyclodextrins and their uses: a review. Process Biochem. 2004;39(9):1033–1046. doi:10.1016/S0032-9592(03)00258-9
- Loftsson T, Duchene D. Cyclodextrins and their pharmaceutical applications. Int J Pharm. 2007;329(1–2):1–11. doi:10.1016/j.ijpharm.2006.07.009
- Qi Z, Sikorski CT. Controlled delivery using cyclodextrin technology. Pharm Tech Eur. 1999;13(11):17–20.
- Almawash S, El Hamd MA, Osman SK. Polymerized β-Cyclodextrin-based injectable hydrogel for sustained release of 5-Fluorouracil/Methotrexate mixture in breast cancer management: in vitro and In vivo analytical validations. Pharmaceutics. 2022;14(4):4. doi:10.3390/pharmaceutics14040817
- Gidwani B, Vyas A. A Comprehensive review on cyclodextrin-based carriers for delivery of chemotherapeutic cytotoxic anticancer drugs. Biomed Res Int. 2015;2015:675. doi:10.1155/2015/198268
- Kim Y. Inclusion complexation of ziprasidone mesylate with β-cyclodextrin sulfobutyl ether. J Pharm Sci. 1998;87(12):1560–1567. doi:10.1021/js980109t
- Pandey A. Cyclodextrin-based nanoparticles for pharmaceutical applications: a review. Environ Chem Lett. 2021;19(6):4297–4310. doi:10.1007/s10311-021-01275-y
- Szejtli J. Introduction and general overview of cyclodextrin chemistry. Chem Rev. 1998;98(5):1743–1753. doi:10.1002/chin.199839312
- Abdulaziz F, Salah D. Gold nanoparticles incorporated with cyclodextrins and its applications. J Biomater Nanobiotechnol. 2021;12(04):79–97. doi:10.4236/jbnb.2021.124007
- Higuchi T, Connor KA. A phase solubility technique. Adv Anal Chem Instrum. 1965;4:117–211.
- Paczkowska M, Szymanowska-Powałowska D, Mizera M, et al. Cyclodextrins as multifunctional excipients: influence of inclusion into β-cyclodextrin on physicochemical and biological properties of tebipenem pivoxil. PLoS One. 2019;14(1):1–22. doi:10.1371/journal.pone.0210694
- Challa R, Ahuja A, Ali J, Khar RK. Cyclodextrins in drug delivery: an updated review. Aaps Pharmscitech. 2005;6(2):E329–E357.
- Osman SK, Soliman GM, Abd El Rasoul S. Physically cross-linked hydrogels of beta-cyclodextrin polymer and poly(ethylene glycol)-cholesterol as delivery systems for macromolecules and small drug molecules. Curr Drug Deliv. 2015;12:415–424.
- Rajbanshi B, Saha S, Das K, et al. Study to probe subsistence of host-guest inclusion complexes of α and β-cyclodextrins with biologically potent drugs for safety regulatory dischargement. Sci Rep. 2018;8(1):1–20. doi:10.1038/s41598-018-31373-x
- Osman SK, Brandl FP, Zayed GM, Teßmar JK, Göpferich AM. Cyclodextrin based hydrogels: inclusion complex formation and micellization of adamantane and cholesterol grafted polymers. Polymer (Guildf). 2011;52(21):4806–4812. doi:10.1016/j.polymer.2011.07.059
- Mohammad A, Singh S, Swain S. Cyclodextrins: concept to applications, regulatory issues and challenges. Nanomedicine Res J. 2020;5(3):202–214. doi:10.22034/NMRJ.2020.03.001
- Saokham P, Muankaew C, Jansook P, Loftsson T. Solubility of cyclodextrins and drug/cyclodextrin complexes. Molecules. 2018;23(5):1–15. doi:10.3390/molecules23051161
- Tomasik P, Schilling CH. Complexes of starch with organic guests. Adv Carbohydr Chem Biochem. 1998;53:263–343. doi:10.1016/s0065-2318(08)60047-5
- Marques CS, Carvalho SG, Bertoli LD, et al. β-Cyclodextrin inclusion complexes with essential oils: obtention, characterization, antimicrobial activity and potential application for food preservative sachets. Food Res Int. 2019;119:(January):499–509. doi:10.1016/j.foodres.2019.01.016
- Dora CP, Trotta F, Kushwah V, et al. Potential of erlotinib cyclodextrin nanosponge complex to enhance solubility, dissolution rate, in vitro cytotoxicity and oral bioavailability. Carbohydr Polym. 2016;137:339–349. doi:10.1016/j.carbpol.2015.10.080
- Bilensoy E, Çırpanlı Y, Şen M, Doğan AL, Çalış S. Thermosensitive mucoadhesive gel formulation loaded with 5-Fu: cyclodextrin complex for HPV-induced cervical cancer. J Incl Phenom Macrocycl Chem. 2007;57(1–4):363–370. doi:10.1007/s10847-006-9259-y
- Loftsson T, Jarho P, Másson M, Järvinen T. Cyclodextrins in drug delivery. Expert Opin Drug Deliv. 2005;2(2):335–351. doi:10.1517/17425247.2.1.335
- Gadade DD, Pekamwar SS. Cyclodextrin based nanoparticles for drug delivery and theranostics. Adv Pharm Bull. 2020;10(2):166–183. doi:10.34172/apb.2020.022
- Davis ME, Brewster ME. Cyclodextrin-based pharmaceutics: past, present and future. Nat Rev Drug Discov. 2004;3(12):1023–1035. doi:10.1038/nrd1576
- Crini G, Fourmentin S, Fenyvesi É, Torri G, Fourmentin M, Morin-Crini N. Cyclodextrins, from molecules to applications. Environ Chem Lett. 2018;16(4):1361–1375. doi:10.1007/s10311-018-0763-2
- Liu Y, Chen Y, Gao X, Fu J, Hu L. Application of cyclodextrin in food industry. Crit Rev Food Sci Nutr. 2020;62(10):2627–2640. doi:10.1080/10408398.2020.1856035
- Matencio A, Hoti G, Monfared YK, et al. Cyclodextrin monomers and polymers for drug activity enhancement. Polymers (Basel). 2021;13(11):1–18. doi:10.3390/polym13111684
- Petitjean M, García-Zubiri IX, Isasi JR. History of cyclodextrin-based polymers in food and pharmacy: a review. Environ Chem Lett. 2021;19(4):3465–3476. doi:10.1007/s10311-021-01244-5
- Yuan Z, Liu H, Wu H, et al. Cyclodextrin Hydrogels: rapid Removal of Aromatic Micropollutants and Adsorption Mechanisms. J Chem Eng Data. 2020;65(2):678–689. doi:10.1021/acs.jced.9b00913
- Malik NS, Ahmad M, Alqahtani MS, et al. β-cyclodextrin chitosan-based hydrogels with tunable pH-responsive properties for controlled release of Acyclovir: design, characterization, safety, and pharmacokinetic evaluation. Drug Deliv. 2021;28(1):1093–1108. doi:10.1080/10717544.2021.1921074
- Hong W, Guo F, Yu N, et al. A novel folic acid receptor-targeted drug delivery system based on curcumin-loaded β-cyclodextrin nanoparticles for cancer treatment. Drug Des Devel Ther. 2021;15:(May):2843–2855. doi:10.2147/DDDT.S320119
- Xu W, Li X, Wang L, et al. Design of cyclodextrin-based functional systems for biomedical applications. Front Chem. 2021;9:(February):1–13. doi:10.3389/fchem.2021.635507
- Balaji A, Pandey VP, Srinath MS, Manavalan R. Synthesis and characterization studies of cisplatin/hydroxypropyl-β-cyclodextrin complex. Pharmacologyonline. 2009;1:1135–1143.
- Zhang L, Man S, Qiu H, et al. Curcumin-cyclodextrin complexes enhanced the anti-cancer effects of curcumin. Environ Toxicol Pharmacol. 2016;48:31–38. doi:10.1016/j.etap.2016.09.021
- Nanda A, Sahoo RN, Pramanik A, et al. Drug-in-mucoadhesive type film for ocular anti-inflammatory potential of amlodipine: effect of sulphobutyl-ether-beta-cyclodextrin on permeation and molecular docking characterization. Colloids Surfaces B Biointerfaces. 2018;172:(August):555–564. doi:10.1016/j.colsurfb.2018.09.011
- Vieira da Silva SA, Clemente A, Rocha J, et al. Anti-inflammatory effect of limonin from cyclodextrin (un)processed Orange juices in in vivo acute inflammation and chronic rheumatoid arthritis models. J Funct Foods. 2018;49(August):146–153. doi:10.1016/j.jff.2018.08.024
- Ling W, Xuehua J, Weijuan X, Chenrui L. Complexation of tanshinone IIA with 2-hydroxypropyl-β-cyclodextrin: effect on aqueous solubility, dissolution rate, and intestinal absorption behavior in rats. Int J Pharm. 2007;341(1–2):58–67. doi:10.1016/j.ijpharm.2007.03.046
- Wu Y, Xiao Y, Yue Y, Zhong K, Zhao Y, Gao H. A deep insight into mechanism for inclusion of 2R,3R-dihydromyricetin with cyclodextrins and the effect of complexation on antioxidant and lipid-lowering activities. Food Hydrocoll. 2020;103:105718. doi:10.1016/j.foodhyd.2020.105718
- Lahiani-Skiba M, Bounoure F, Fessi H, Skiba M. Effect of cyclodextrins on lonidamine release and in-vitro cytotoxicity. J Incl Phenom Macrocycl Chem. 2011;69(3–4):481–485. doi:10.1007/s10847-010-9872-7
- Jacob S, Nair AB. Cyclodextrin complexes: perspective from drug delivery and formulation. Drug Dev Res. 2018;79(5):201–217. doi:10.1002/ddr.21452
- Mura P. Analytical techniques for characterization of cyclodextrin complexes in the solid state: a review. J Pharm Biomed Anal. 2015;113:226–238. doi:10.1016/j.jpba.2015.01.058
- Mura P, Adragna E, Rabasco AM, et al. Effects of the host cavity size and the preparation method on the physicochemical properties of ibuproxam-cyclodextrin systems. Drug Dev Ind Pharm. 1999;25(3):279–287.
- Mura P, Faucci MT, Parrini PL, Furlanetto S, Pinzauti S. Influence of the preparation method on the physicochemical properties of ketoprofen–cyclodextrin binary systems. Int J Pharm. 1999;179(1):117–128.
- Allahyari S, Trotta F, Valizadeh H, Jelvehgari M, Zakeri-Milani P. Cyclodextrin based nanosponges as promising carriers for active agents. Expert Opin Drug Deliv. 2019;16(5):467–479. doi:10.1080/17425247.2019.1591365
- Tannous M, Caldera F, Hoti G, Dianzani U, Cavalli R, Trotta F. Drug-encapsulated cyclodextrin nanosponges. In: supramolecules in Drug Discovery and Drug Delivery: methods and Protocols. Methods in Molecular Biology. 2021;2207. doi:10.1007/978-1-0716-0920-0
- Sadaquat H, Akhtar M. Comparative effects of β-cyclodextrin, HP-β-cyclodextrin and SBE7-β-cyclodextrin on the solubility and dissolution of docetaxel via inclusion complexation. J Incl Phenom Macrocycl Chem. 2020;96(3–4):333–351. doi:10.1007/s10847-020-00977-0
- Zia V, Rajewski RA, Stella VJ. Effect of cyclodextrin charge on complexation of neutral and charged substrates: comparison of (SBE)7m-β-CD to HP-β-CD. Pharm Res. 2001;18(5):667–673. :
- Nagase Y, Hirata M, Wada K, et al. Improvement of some pharmaceutical properties of DY-9760e by sulfobutyl ether b-cyclodextrin. Int J Pharm. 2001;229:163–172. doi:10.1007/s10847-010-9870-9
- Tros de Ilarduya MC, Martín C, Goñi MM, Martínez-Ohárriz MC. Solubilization and interaction of sulindac with β-cyclodextrin in the solid state and in aqueous solution. Drug Dev Ind Pharm. 1998;24(3):301–306. doi:10.3109/03639049809085624
- Loftsson T, Brewster ME. Pharmaceutical applications of cyclodextrins. 1. Drug solubilization and stabilization. J Pharm Sci. 1996;85(10):1017–1025. doi:10.1021/js950534b
- Dalmora MEA. Inclusion complex of piroxicam with β-cyclodextrin and incorporation in hexadecyltrimethylammonium bromide based microemulsion. Int J Pharm. 1999;184(2):184.
- Cedillo-Flores OE, Rodríguez-Laguna N, Hipólito-Nájera AR, Nivón-Ramírez D, Gómez-Balderas R, Moya-Hernández R. Effect of the pH on the thermodynamic stability of inclusion complexes of thymol and carvacrol in β-cyclodextrin in water. Food Hydrocoll. 2022;124(March):2021. doi:10.1016/j.foodhyd.2021.107307
- Yavuz B, Bilensoy E, Vural I, Şumnu M. Alternative oral exemestane formulation: improved dissolution and permeation. Int J Pharm. 2010;398(1–2):137–145. doi:10.1016/j.ijpharm.2010.07.046
- Wadhwa G, Kumar S, Chhabra L, Mahant S, Rao R. Essential oil–cyclodextrin complexes: an updated review. J Incl Phenom Macrocycl Chem. 2017;89(1–2):39–58. doi:10.1007/s10847-017-0744-2
- Jiang L, Yang J, Wang Q, Ren L, Zhou J. Physicochemical properties of catechin/β-cyclodextrin inclusion complex obtained via co-precipitation. CYTA - J Food. 2019;17(1):544–551. doi:10.1080/19476337.2019.1612948
- Jug M, Mura PA. Grinding as solvent-free green chemistry approach for cyclodextrin inclusion complex preparation in the solid state. Pharmaceutics. 2018;10:4. doi:10.3390/pharmaceutics10040189
- Banchero M. Supercritical carbon dioxide as a green alternative to achieve drug complexation with cyclodextrins. Pharmaceuticals. 2021;14:6. doi:10.3390/ph14060562
- Kaur K, Jindal R, Jindal D. Synthesis, characterization and studies on host-guest interactions of inclusion complexes of metformin hydrochloride with β–cyclodextrin. J Mol Liq. 2019;282:162–168. doi:10.1016/j.molliq.2019.02.127
- Khushbu JR. Cyclodextrin mediated controlled release of edaravone from pH-responsive sodium alginate and chitosan based nanocomposites. Int J Biol Macromol. 2022;202:(January):11–25. doi:10.1016/j.ijbiomac.2022.01.001
- Al-Marzouqi AH, Elwy HM, Shehadi I, Adem A. Physicochemical properties of antifungal drug-cyclodextrin complexes prepared by supercritical carbon dioxide and by conventional techniques. J Pharm Biomed Anal. 2009;49(2):227–233. doi:10.1016/j.jpba.2008.10.032
- Moyano JR, Arias MJ, Gines JM, Perez JI, Rabasco AM. Dissolution Behavior of Oxazepam in Presence of Cyclodextrins: evaluation of Oxazepam-Dimeb Binary Systemxs. Drug Dev Ind Pharm. 1997;23(4):379–385.
- Li B, Li N, Wang S, Gao J, Fang S. Pharmacokinetics of injectable beta -Cyclodextrin-Oridonin inclusion complex, a novel formulation of oridonin in Wistar rats. Natl J Physiol Pharm Pharmacol. 2012;2(1):52–57.
- Castillo JA, Palomo-Canales J, Garcia JJ, Lastres JL, Bolas F, Torrado JJ. Preparation and characterization of albendazole β-cyclodextrin complexes. Drug Dev Ind Pharm. 1999;25(12):1241–1248.
- Cid-Samamed A, Rakmai J, Mejuto JC, Simal-Gandara J, Astray G. Cyclodextrins inclusion complex: preparation methods, analytical techniques and food industry applications. Food Chem. 2022;384:132467. doi:10.1016/j.foodchem.2022.132467
- Parlati S, Gobetto R, Barolo C, et al. Preparation and application of a β-cyclodextrin-disperse/reactive dye complex. J Incl Phenom Macrocycl Chem. 2007;57(1–4):463–470. doi:10.1007/s10847-006-9235-6
- Pereva S, Sarafska T, Bogdanova S, Spassov T. Efficiency of “cyclodextrin-ibuprofen” inclusion complex formation. J Drug Deliv Sci Technol. 2016;35:34–39. doi:10.1016/j.jddst.2016.04.006
- Ghorab MK, Adeyeye MC. Enhancement of ibuprofen dissolution via wet granulation with β-cyclodextrin. Pharm Dev Technol. 2001;6(3):305–314. doi:10.1081/PDT-100002611
- Reddy MN, Rehana T, Ramakrishna S, Chowdary KPR, Diwan PV. β-cyclodextrin complexes of celecoxib: molecular-modeling, characterization, and dissolution studies. AAPS J. 2004;6(1):1–9. doi:10.1208/ps060107
- Patel HM, Suhagia BN, Shah SA, Rathod IS, Parmar VK. Preparation and characterization of etoricoxib-β-cyclodextrin complexes prepared by the kneading method. Acta Pharm. 2007;57(3):351–359. doi:10.2478/v10007-007-0028-2
- Swami G, Koshy MK, Pandey M, Saraf SA. Preparation and characterization of domperidone- β-cyclodextrin complexes prepared by kneading method. Int J Adv Pharm Sci. 2010;1(1):68–74. doi:10.5138/ijaps.2010.0976.1055.01008
- Songkro S, Hayook N, Jaisawang J, Maneenuan D, Chuchome T, Kaewnopparat N. Investigation of inclusion complexes of citronella oil, citronellal and citronellol with b-cyclodextrin for mosquito repellent. J Incl Phenom Macrocycl Chem. 2012;72(3–4):339–355. doi:10.1007/s10847-011-9985-7
- Pralhad T, Rajendrakumar K. Study of freeze-dried quercetin-cyclodextrin binary systems by DSC, FT-IR, X-ray diffraction and SEM analysis. J Pharm Biomed Anal. 2004;34(2):333–339. doi:10.1016/S0731-7085(03)00529-6
- Villalonga R, Cao R, Fragoso A, Damiao AE, Ortiz PD, Caballero J. Supramolecular-mediated bienzymatic immobilization of catalase and superoxide dismutase on β-cyclodextrin-modified gold nanospheres. J Mol Catal B Enzym. 2005;35:79.
- Li N, Wang N, Wu T, et al. Preparation of curcumin-hydroxypropyl-β-cyclodextrin inclusion complex by cosolvency-lyophilization procedure to enhance oral bioavailability of the drug. Drug Dev Ind Pharm. 2018;44(12):1966–1974. doi:10.1080/03639045.2018.1505904
- Elgindy N, Elkhodairy K, Molokhia A, Elzoghby A. Lyophilization monophase solution technique for improvement of the physicochemical properties of an anticancer drug, flutamide. Eur J Pharm Biopharm. 2010;74(2):397–405. doi:10.1016/j.ejpb.2009.11.011
- Eid EEM, Abdul AB, Suliman FEO, Sukari MA, Rasedee A, Fatah SS. Characterization of the inclusion complex of zerumbone with hydroxypropyl-β-cyclodextrin. Carbohydr Polym. 2011;83(4):1707–1714. doi:10.1016/j.carbpol.2010.10.033
- Ozdemir N, Pola CC, Teixeira BN, Hill LE, Bayrak A, Gomes CL. Preparation of black pepper oleoresin inclusion complexes based on beta-cyclodextrin for antioxidant and antimicrobial delivery applications using kneading and freeze drying methods: a comparative study. Lwt-Food Sci Technol. 2018;91:439–445. doi:10.1016/j.lwt.2018.01.046
- Skalko-basnet N, Pavelic Z, Becirevic-lacan M. Liposomes containing drug and cyclodextrin prepared by the one-step spray-drying method. Drug Dev Ind Pharm. 2000;26(12):1279–1284.
- Cabral-Marques H, Almeida R. Optimisation of spray-drying process variables for dry powder inhalation (DPI) formulations of corticosteroid/cyclodextrin inclusion complexes. Eur J Pharm Biopharm. 2009;73(1):121–129. doi:10.1016/j.ejpb.2009.05.002
- Borghetti GS, Lula IS, Sinisterra RD, Bassani VL. Quercetin/β-Cyclodextrin solid complexes prepared in aqueous solution followed by spray-drying or by physical mixture. AAPS PharmSciTech. 2009;10(1):235–242. doi:10.1208/s12249-009-9196-3
- Miletic T, Kyriakos K, Graovac A, Ibric S. Spray-dried voriconazole-cyclodextrin complexes: solubility, dissolution rate and chemical stability. Carbohydr Polym. 2013;98(1):122–131. doi:10.1016/j.carbpol.2013.05.084
- Watson MA, Lea JM, Bett-Garber KL. Spray drying of pomegranate juice using maltodextrin/cyclodextrin blends as the wall material. Food Sci Nutr. 2017;5(3):820–826. doi:10.1002/fsn3.467
- Ramos AI, Braga TM, Silva P, et al. Chloramphenicol·cyclodextrin inclusion compounds: co-dissolution and mechanochemical preparations and antibacterial action. CrystEngComm. 2013;15(15):2822–2834. doi:10.1039/c3ce26414a
- Mura P, Faucci MT, Maestrelli F, Furlanetto S, Pinzauti S. Characterization of physicochemical properties of naproxen systems with amorphous β-cyclodextrin-epichlorohydrin polymers. J Pharm Biomed Anal. 2002;29(6):1015–1024. doi:10.1016/S0731-7085(02)00142-5
- Tan Q, He D, Wu M, et al. Characterization, activity, and computer modeling of a molecular inclusion complex containing rifaldazine. Int J Nanomedicine. 2013;8:477–484. doi:10.2147/IJN.S38937
- He D, Deng P, Yang L, et al. Molecular encapsulation of rifampicin as an inclusion complex of hydroxypropyl-β-cyclodextrin: design; characterization and in vitro dissolution. Colloids Surfaces B Biointerfaces. 2013;103:580–585. doi:10.1016/j.colsurfb.2012.10.062
- Cugovčan M, Jablan J, Lovrić J, Cinčić D, Galić N, Jug M. Biopharmaceutical characterization of praziquantel cocrystals and cyclodextrin complexes prepared by grinding. J Pharm Biomed Anal. 2017;137:42–53. doi:10.1016/j.jpba.2017.01.025
- Ali HRH, Saleem IY, Tawfeek HM. Insight into inclusion complexation of indomethacin nicotinamide cocrystals. J Incl Phenom Macrocycl Chem. 2016;84(3–4):179–188. doi:10.1007/s10847-016-0594-3
- Jug M, Mennini N, Kövér KE, Mura P. Comparative analysis of binary and ternary cyclodextrin complexes with econazole nitrate in solution and in solid state. J Pharm Biomed Anal. 2014;91:81–91. doi:10.1016/j.jpba.2013.12.029
- Majewska K, Skwierawska A, Kamińska B, Prześniak-Welenc M. Improvement of opipramol base solubility by complexation with β-cyclodextrin. Supramol Chem. 2018;30(1):20–31. doi:10.1080/10610278.2017.1350677
- Malaquias LFB, Sá-Barreto LCL, Freire DO, et al. Taste masking and rheology improvement of drug complexed with beta-cyclodextrin and hydroxypropyl-β-cyclodextrin by hot-melt extrusion. Carbohydr Polym. 2018;185:19–26. doi:10.1016/j.carbpol.2018.01.011
- Conceição J, Farto-Vaamonde X, Goyanes A, et al. Hydroxypropyl-β-cyclodextrin-based fast dissolving carbamazepine printlets prepared by semisolid extrusion 3D printing. Carbohydr Polym. 2019;221:55–62. doi:10.1016/j.carbpol.2019.05.084
- Granados PA, Pinho LAG, Sa-Barreto LL, Gratieri T, Gelfuso GM, Cunha-Filho M. Application of hot-melt extrusion in the complexation of naringenin with cyclodextrin using hydrophilic polymers. Adv Powder Technol. 2022;33(1):103380. doi:10.1016/j.apt.2021.11.032
- Sauceau M, Rodier E, Fages J. Preparation of inclusion complex of piroxicam with cyclodextrin by using supercritical carbon dioxide. J Supercrit Fluids. 2008;47(2):326–332. doi:10.1016/j.supflu.2008.07.006
- Banchero M, Ronchetti S, Manna L. Characterization of ketoprofen/methyl-β-cyclodextrin complexes prepared using supercritical carbon dioxide. J Chem. 2013;2013:45. doi:10.1155/2013/583952
- Hussein K, Türk M, Wahl MA. Comparative evaluation of Ibuprofen/β-cyclodextrin complexes obtained by supercritical carbon dioxide and other conventional methods. Pharm Res. 2007;24(3):585–592. doi:10.1007/s11095-006-9177-0
- Al-Marzouqi AH, Jobe B, Dowaidar A, Maestrelli F, Mura P. Evaluation of supercritical fluid technology as preparative technique of benzocaine-cyclodextrin complexes-Comparison with conventional methods. J Pharm Biomed Anal. 2007;43(2):566–574. doi:10.1016/j.jpba.2006.08.019
- Al-Marzouqi A, Jobe B, Corti G, Cirri M, Mura P. Physicochemical characterization of drug-cyclodextrin complexes prepared by supercritical carbon dioxide and by conventional techniques. J Incl Phenom Macrocycl Chem. 2007;57(1–4):223–231. doi:10.1007/s10847-006-9192-0
- Al-Marzouqi AH, Solieman A, Shehadi I, Adem A. Influence of the preparation method on the physicochemical properties of econazole-β-cyclodextrin complexes. J Incl Phenom Macrocycl Chem. 2008;60(1–2):85–93. doi:10.1007/s10847-007-9356-6
- Rudrangi SRS, Trivedi V, Mitchell JC, Wicks SR, Alexander BD. Preparation of olanzapine and methyl-β-cyclodextrin complexes using a single-step, organic solvent-free supercritical fluid process: an approach to enhance the solubility and dissolution properties. Int J Pharm. 2015;494(1):408–416. doi:10.1016/j.ijpharm.2015.08.062
- Mohammed AM, Faisal W, Saleh KI, Osman SK. Aqueous solubility and dissolution rate improvement of etodolac via inclusion complexation technique. Int J Pharmacol Pharm Res Hum Journals. 2016;6(3):304–318.
- Adeoye O, Costa C, Casimiro T, Aguiar-Ricardo A, Cabral-Marques H. Preparation of ibuprofen/hydroxypropyl-Γ-cyclodextrin inclusion complexes using supercritical CO2-assisted spray drying. J Supercrit Fluids. 2018;133:479–485. doi:10.1016/j.supflu.2017.11.009
- Ling XY, Malaquin L, Reinhoudt DN, Wolf H, Huskens J. An in situ study of the adsorption behavior of functionalized particles on self-assembled monolayers via different chemical interactions. Langmuir. 2007;23(20):9990–9999. doi:10.1021/la701671s
- Maestrelli F, González-Rodríguez ML, Rabasco AM, Mura P. Preparation and characterisation of liposomes encapsulating ketoprofen-cyclodextrin complexes for transdermal drug delivery. Int J Pharm. 2005;298(1):55–67. doi:10.1016/j.ijpharm.2005.03.033
- Veiga F, Fernandes C, Maincent P. Influence of the preparation method on the physicochemical properties of tolbutamide/cyclodextrin binary systems. Drug Dev Ind Pharm. 2001;27(6):523–532. doi:10.1081/DDC-100105177
- Shan-Yang L, Yuh-Horng K. Solid particulates of drug-β-cyclodextrin inclusion complexes directly prepared by a spray-drying technique. Int J Pharm. 1989;56(3):249–259. doi:10.1016/0378-5173(89)90022-7
- Yang X, Shen J, Liu J, et al. Spray-drying of hydroxypropyl β-cyclodextrin microcapsules for co-encapsulation of resveratrol and piperine with enhanced solubility. Crystals. 2022;12:5. doi:10.3390/cryst12050596
- Gao X, Chen G, Ning L. Plasmonic characteristics of nanorod-based metallic nanostructures. Opt Laser Technol. 2013;48:394–400. doi:10.1016/j.optlastec.2012.10.036
- Wanunu M, Popovitz-Biro R, Cohen H, Vaskevich A, Rubinstein I. Coordination-based gold nanoparticle layers. J Am Chem Soc. 2005;127(25):9207–9215. doi:10.1021/ja050016v
- Barrientos L, Yutronic N, Del monte F, Gutiérrez MC, Jara P. Ordered arrangement of gold nanoparticles on an α-cyclodextrin-dodecanethiol inclusion compound produced by magnetron sputtering. New J Chem. 2007;31(8):1400–1402. doi:10.1039/b706346f
- Dreaden EC, Alkilany AM, Huang X, Murphy CJ, El-Sayed MA. The golden age: gold nanoparticles for biomedicine. Chem Soc Rev. 2012;41(7):2740–2779. doi:10.1039/c1cs15237h
- Liu Y, Male KB, Bouvrette P, Luong JHT. Control of the size and distribution of gold nanoparticles by unmodified cyclodextrins. Chem Mater. 2003;15(22):4172–4180. doi:10.1021/cm0342041
- Nie S, Xing Y, Kim GJ, Simons JW. Nanotechnology applications in cancer. Annu Rev Biomed Eng. 2007;9:257–288. doi:10.1146/annurev.bioeng.9.060906.152025
- Wang MD, Shin DM, Simons JW, Nie S. Nanotechnology for targeted cancer therapy. Expert Rev Anticancer Ther. 2007;7(6):833–837. doi:10.1586/14737140.7.6.833
- You -C-C, Miranda OR, Gider B, et al. Detection and identification of proteins using nanoparticle-fluorescent polymer “chemical nose” sensors. Nat Nanotechnol. 2007;2(5):318–323. doi:10.1038/nnano.2007.99
- Bhattacharya R, Patra CR, Earl A, et al. Attaching folic acid on gold nanoparticles using noncovalent interaction via different polyethylene glycol backbones and targeting of cancer cells. Nanomed Nanotechnol Biol Med. 2007;3(3):224–238. doi:10.1016/j.nano.2007.07.001
- Li J, Lou Z. Synthesis and applications of gold nanoparticles. Pharmacologyonline. 2021;3:1870–1874. doi:10.47583/ijpsrr.2022.v77i01.003
- Faraday M. The Bakerian Lecture: experimental relations of gold (and other metals) to light. Philos Trans R Soc London. 1857;147:145–181. doi:10.1098/rstl.1857.0011
- Turkevich J, Stevenson PC, Hillier J. A study of the nucleation and growth processes in the synthesis of colloidal gold. Discuss Faraday Soc. 1951;11(c):55–75. doi:10.1039/DF9511100055
- Hayat MA. Colloidal Gold: Principles, Methods, and Applications. Elsevier; 2012.
- Frens G. Controlled nucleation for the regulation of the particle size in monodisperse gold suspensions. Nat Phys Sci. 1973;241(105):20–22.
- Zhao L, Jiang D, Cai Y, Ji X, Xie R, Yang W. Tuning the size of gold nanoparticles in the citrate reduction by chloride ions. Nanoscale. 2012;4(16):5071–5076. doi:10.1039/c2nr30957b
- Shah M, Badwaik V, Kherde Y, et al. Gold nanoparticles : various methods of synthesis and antibacterial applications. Front Biosci. 2014;19:1320–1344.
- Esumi K, Matsuhisa K, Torigoe K. Preparation of rodlike gold particles by UV irradiation using cationic micelles as a template. Langmuir. 1995;11:3285–3287.
- Valden M, Lai X, Goodman DW. Onset of catalytic activity of gold clusters on titania with the appearance of nonmetallic properties. Science. 1998;281(5383):1647–1650.
- Veith GM, Lupini AR, Pennycook SJ, Ownby GW, Dudney NJ. Nanoparticles of gold on γ-Al2O3 produced by dc magnetron sputtering. J Catal. 2005;231(1):151–158. doi:10.1016/j.jcat.2004.12.008
- Kabashin AV, Meunier M, Kingston C, Luong JHT. Fabrication and characterization of gold nanoparticles by femtosecond laser ablation in an aqueous solution of cyclodextrins. J Phys Chem B. 2003;107(19):4527–4531. doi:10.1021/jp034345q
- Usman AI, Abdul Aziz A, Abu Noqta O. Application of green synthesis of gold nanoparticles: a review. J Teknol. 2017;79(5):1–5. doi:10.11113/jt.v81.11409
- Santhosh PB, Julia Genova HC. Green synthesis of gold nanoparticles: an eco-friendly approach. Chemistry. 2015;4:345–369. doi:10.3390/chemistry4020026
- Teimuri-Mofrad R, Hadi R, Tahmasebi B, Farhoudian S, Mehravar M, Nasiri R. Green synthesis of gold nanoparticles using plant extract: mini-review. Nanochemistry Res. 2017;2(1):8–19. doi:10.22036/ncr.2017.01.002
- Kumar S, Gandhi KS, Kumar R. Modeling of formation of gold nanoparticles by citrate method. Ind Eng Chem Res. 2007;46(10):3128–3136. doi:10.1021/ie060672j
- Sivaraman SK, Kumar S, Santhanam V. Monodisperse sub-10 nm gold nanoparticles by reversing the order of addition in Turkevich method – the role of chloroauric acid. J Colloid Interface Sci. 2011;361(2):543–547. doi:10.1016/j.jcis.2011.06.015
- Ojea-Jiménez I, Romero FM, Bastús NG, Puntes V. Small gold nanoparticles synthesized with sodium citrate and heavy water: insights into the reaction mechanism. J Phys Chem C. 2010;114(4):1800–1804. doi:10.1021/jp9091305
- Merza KS, Al-Attabi HD, Abbas ZM, Yusr HA. Comparative study on methods for preparation of gold nanoparticles. Green Sustain Chem. 2012;2(1):26–28. doi:10.4236/gsc.2012.21005
- Waters CA, Mills AJ, Johnson KA, Schiffrin DJ. Purification of dodecanethiol derivatised gold nanoparticles. Chem Commun. 2003;3(4):540–541. doi:10.1039/b211874b
- Luty-błocho M, Fitzner K, Hessel V, Löb P, Maskos M, Metzke D. Synthesis of gold nanoparticles in an interdigital micromixer using ascorbic acid and sodium borohydride as reducers. Chem Eng. 2011;171:279–290. doi:10.1016/j.cej.2011.03.104
- Xu Z-C, Shen C-M, Xiao C-W, et al. Wet chemical synthesis of gold nanoparticles using silver seeds: a shape control from nanorods to hollow spherical nanoparticles. Nanotechnology. 2007;18(11):115608. doi:10.1088/0957-4484/18/11/115608
- Shao L, Susha AS, Cheung LS, Sau TK, Rogach AL, Wang J. Plasmonic properties of single multispiked gold nanostars: correlating modeling with experiments. Langmuir. 2012;28(24):8979–8984. doi:10.1021/la2048097
- Sau TK, Rogach AL, Döblinger M, Feldmann J. One-step high-yield aqueous synthesis of size-tunable multispiked gold nanoparticles. Small. 2011;7(15):2188–2194. doi:10.1002/smll.201100365
- Prasad BLV, Stoeva SI, Sorensen CM, Klabunde KJ. Digestive ripening of thiolated gold nanoparticles: the effect of alkyl chain length. Langmuir. 2002;18:7515–7520.
- John MG, Tibbetts KM. One-step femtosecond laser ablation synthesis of sub-3 nm gold nanoparticles stabilized by silica. Appl Surf Sci. 2019;475:1048–1057. doi:10.1016/j.apsusc.2019.01.042
- Gingery D, Bühlmann P. Formation of gold nanoparticles on multiwalled carbon nanotubes by thermal evaporation. Carbon N Y. 2008;46(14):1966–1972. doi:10.1016/j.carbon.2008.08.007
- Gaspar D, Pimentel AC, Mateus T, et al. Influence of the layer thickness in plasmonic gold nanoparticles produced by thermal evaporation. Sci Rep. 2013;3:1469. doi:10.1038/srep01469
- Aswathy B, Avadhani GS, Suji S, Sony G. Synthesis of β-cyclodextrin functionalized gold nanoparticles for the selective detection of Pb2+ ions from aqueous solution. Front Mater Sci. 2012;6(2):168–175. doi:10.1007/s11706-012-0165-5
- George JM, Mathew B. Cyclodextrin-mediated gold nanoparticles as multisensing probe for the selective detection of hydroxychloroquine drug. Korean J Chem Eng. 2021;38(3):624–634. doi:10.1007/s11814-020-0719-7
- Philip D. Honey mediated green synthesis of gold nanoparticles. Spectrochim Acta. 2009;73(4):650–653. doi:10.1016/j.saa.2009.03.007
- Cherian T, Maity D, Kumar R, et al. Green chemistry based gold nanoparticles synthesis using the marine bacterium Lysinibacillus odysseyi PBCW2 and their multitudinous activities. Nanomaterials. 2022;12(17):ye6. doi:10.3390/nano12172940
- Zhang X, Qu Y, Shen W, et al. Biogenic synthesis of gold nanoparticles by yeast Magnusiomyces ingens LH-F1 for catalytic reduction of nitrophenols. Colloids Surfaces a Physicochem Eng Asp. 2016;497:280–285. doi:10.1016/j.colsurfa.2016.02.033
- Molnár Z, Bódai V, Szakacs G, et al. Green synthesis of gold nanoparticles by thermophilic filamentous fungi. Sci Rep. 2018;8(1):1–12. doi:10.1038/s41598-018-22112-3
- Ramakrishna M, Rajesh Babu D, Gengan RM, Chandra S, Nageswara Rao G. Green synthesis of gold nanoparticles using marine algae and evaluation of their catalytic activity. J Nanostructure Chem. 2016;6(1):1–13. doi:10.1007/s40097-015-0173-y
- Colin JA, Pech-Pech IE, Oviedo M, Águila SA, Romo-Herrera JM, Contreras OE. Gold nanoparticles synthesis assisted by marine algae extract: biomolecules shells from a green chemistry approach. Chem Phys Lett. 2018;708:(August):210–215. doi:10.1016/j.cplett.2018.08.022
- Sadeghi B, Mohammadzadeh M, Babakhani B. Green synthesis of gold nanoparticles using Stevia rebaudiana leaf extracts: characterization and their stability. J Photochem Photobiol B Biol. 2015;148:101–106. doi:10.1016/j.jphotobiol.2015.03.025
- Awad MA, Eisa NE, Virk P, et al. Green synthesis of gold nanoparticles: preparation, characterization, cytotoxicity, and anti-bacterial activities. Mater Lett. 2019;256:126608. doi:10.1016/j.matlet.2019.126608
- Bhattacharya R, Mukherjee P. Biological properties of “naked” metal nanoparticles. Adv Drug Deliv Rev. 2008;60(11):1289–1306. doi:10.1016/j.addr.2008.03.013
- Burda C, Chen X, Narayanan R, El-Sayed MA. Chemistry and properties of nanocrystals of different shapes. Chem Rev. 2005;105(4):1025–1102. doi:10.1021/cr030063a
- Mulvaney P. Surface plasmon spectroscopy of nanosized metal particles. Langmuir. 1996;12(3):788–800. doi:10.1021/la9502711
- Link S, El-Sayed MA. Spectral Properties and Relaxation Dynamics of Surface Plasmon Electronic Oscillations in Gold and Silver Nanodots and Nanorods. J Phys Chem B. 1999;103(40):8410–8426. doi:10.1021/jp9917648
- Liu J, Mendoza S, Roma E, Lynn MJ, Xu R, Kaifer AE. Cyclodextrin-modified gold nanospheres. Host-guest interactions at work to control colloidal properties. J Am Chem Soc. 1999;121(9):4304–4305.
- Wang Y, Li H, Jin Q, Ji J. Intracellular host-guest assembly of gold nanoparticles triggered by glutathione. Chem Commun. 2016;52(3):582–585. doi:10.1039/c5cc07195j
- Memişoǧlu E, Bochot A, Şen M, Duchêne D, Hincal AA. Non-surfactant nanospheres of progesterone inclusion complexes with amphiphilic β-cyclodextrins. Int J Pharm. 2003;251(1–2):143–153. doi:10.1016/S0378-5173(02)00593-8
- Da Silveira AM, Ponchel G, Puisieux F, Duchêne D. Combined poly(isobutylcyanoacrylate) and cyclodextrins nanoparticles for enhancing the encapsulation of lipophilic drugs. Pharm Res. 1998;15(7):1051–1055. doi:10.1023/A:1011982211632
- Duchêne D. Cyclodextrins in targeting Application to nanoparticles. Adv Drug Deliv Rev. 1999;36(1):29–40. doi:10.1016/S0169-409X(98)00053-2
- Wang Y, Han Y, Tan X, Dai Y, Xia F, Zhang X. Cyclodextrin capped gold nanoparticles (AuNP@CDs): from synthesis to applications. J Mater Chem B. 2021;9(11):2584–2593. doi:10.1039/d0tb02857f
- Carofiglio T, Fornasier R, Jicsinszky L, Tonellato U, Turco C. Synthesis, characterization and chemisorption on gold of a β-cyclodextrin–lipoic acid conjugate. Tetrahedron Lett. 2001;42(31):5241–5244. doi:10.1016/S0040-4039(01)01001-2
- Liu J, Ong W, Román E, Lynn MJ, Kaifer AE. Cyclodextrin-modified gold nanospheres. Langmuir. 2000;16(7):3000–3002. doi:10.1021/la991519f
- Manickam P, Vashist A, Madhu S, et al. Gold nanocubes embedded biocompatible hybrid hydrogels for electrochemical detection of H2O2. Bioelectrochemistry. 2020;131:107373. doi:10.1016/j.bioelechem.2019.107373
- Neri G, Cordaro A, Scala A, Cordaro M, Mazzaglia A, Piperno A. PEGylated bis-adamantane carboxamide as guest bridge for graphene poly-cyclodextrin gold nanoassemblies. J Mol Struct. 2021;1240:130519. doi:10.1016/j.molstruc.2021.130519
- Adeli M, Sarabi RS, Yadollahi Farsi R, Mahmoudi M, Kalantari M. Polyrotaxane/gold nanoparticle hybrid nanomaterials as anticancer drug delivery systems. J Mater Chem. 2011;21(46):18686–18695. doi:10.1039/c1jm12412a
- Andreani SA, Tachrim ZP, et al. The effect of α-cyclodextrin and β-cyclodextrin as stabilizing agents on the size of gold nanoparticles. AIP Conference Proceedings. Vol 2493. AIP Publishing LLC; 2022:060005.
- Shi Y, Goodisman J, Dabrowiak JC. Cyclodextrin capped gold nanoparticles as a delivery vehicle for a prodrug of cisplatin. Inorg Chem. 2013;52(16):9418–9426. doi:10.1021/ic400989v
- Yang C, Wang X, Li H, Tan E, Lim CT, Li J. Cationic polyrotaxanes as gene carriers: physicochemical properties and real-time observation of DNA complexation, and gene transfection in cancer cells. J Phys Chem B. 2009;113(22):7903–7911. doi:10.1021/jp901302f
- Zhang X, Zhu X, Ke F, et al. Preparation and self-assembly of amphiphilic triblock copolymers with polyrotaxane as a middle block and their application as carrier for the controlled release of Amphotericin B. Polymer (Guildf). 2009;50(18):4343–4351. doi:10.1016/j.polymer.2009.07.006
- Sierpe R, Lang E, Jara P, et al. Gold Nanoparticles Interacting with β-Cyclodextrin–Phenylethylamine Inclusion Complex: a Ternary System for Photothermal Drug Release. ACS Appl Mater Interfaces. 2015;7(28):15177–15181. doi:10.1021/acsami.5b00186
- Park C, Youn H, Kim H, et al. Cyclodextrin-covered gold nanoparticles for targeted delivery of an anti-cancer drug. J Mater Chem. 2009;19(16):2310–2315. doi:10.1039/b816209c
- Gimenez IF, Anazetti MC, Melo PS, et al. Cytotoxicity on V79 and HL60 Cell Lines by Thiolated-β-Cyclodextrin-Au/Violacein Nanoparticles. J Biomed Nanotechnol. 2005;1(3):352–358. doi:10.1166/jbn.2005.041
- Memişoğlu-Bilensoy E, Vural I, Bochot A, et al. Tamoxifen citrate loaded amphiphilic β-cyclodextrin nanoparticles: in vitro characterization and cytotoxicity. J Control Release. 2005;104(3):489–496. doi:10.1016/j.jconrel.2005.03.006
- Hincal AA, Memişoğlu-Bilensoy E, Bochot A, Duchene D. β-cyclodextrines amphiphiles: Évaluation de nouveaux excipients pour la préparation de nanoparticules destinées à l’administration par voie parentérale ou topique. Bull Tech Gattefossé. 2003;96(2):59–71.
- Zhou Y, Wang C, Wang F, Li C, Dong C, Shuang S. β-Cyclodextrin and its derivatives functionalized magnetic nanoparticles for targeting delivery of curcumin and cell imaging. Chinese J Chem. 2016;34(6):599–608. doi:10.1002/cjoc.201500756
- Sandhu KK, McIntosh CM, Simard JM, Smith SW, Rotello VM. Gold nanoparticle-mediated transfection of mammalian cells. Bioconjug Chem. 2002;13(1):3–6. doi:10.1021/bc015545c
- Wang G, Zhang J, Murray RW. DNA binding of an ethidium intercalator attached to a monolayer-protected gold cluster. Anal Chem. 2002;74(17):4320–4327. doi:10.1021/ac0257804
- Fischer NO, McIntosh CM, Simard JM, Rotello VM. Inhibition of chymotrypsin through surface binding using nanoparticle-based receptors. Proc Natl Acad Sci U S A. 2002;99(8):5018–5023. doi:10.1073/pnas.082644099
- Park S, Taton TA, Mirkin CA. Array-based electrical detection of DNA with nanoparticle probes. Science. 2002;295(5559):1503–1506.
- Bohl Kullberg E, Bergstrand N, Carlsson J, et al. Development of EGF-conjugated liposomes for targeted delivery of boronated DNA-binding agents. Bioconjug Chem. 2002;13(4):737–743. doi:10.1021/bc0100713
- Liu J, Alvarez J, Ong W, Román E, Kaifer AE. Phase transfer of hydrophilic, cyclodextrin-modified gold nanoparticles to chloroform solutions. J Am Chem Soc. 2001;123(45):11148–11154. doi:10.1021/ja003957a
- Weisser M, Nelles G, Wenz G, Mittler-Neher S. Guest-host interactions with immobilized cyclodextrins. Sensors Actuators, B Chem. 1997;38-39(1–3):58–67. doi:10.1016/S0925-4005(97)80172-4
- Sztandera K, Gorzkiewicz M, Klajnert-Maculewicz B. Gold nanoparticles in cancer treatment. Mol Pharm. 2019;16(1):1–23. doi:10.1021/acs.molpharmaceut.8b00810
- Yao C, Zhang L, Wang J, et al. Gold nanoparticle mediated phototherapy for cancer. J Nanomater. 2016:5497136. doi:10.1155/2016/5497136
- Alkilany AM, Murphy CJ. Toxicity and cellular uptake of gold nanoparticles: what we have learned so far? J Nanoparticle Res. 2010;12(7):2313–2333. doi:10.1007/s11051-010-9911-8
- Hădărugă NG, Bandur GN, David I, Hădărugă DI. A review on thermal analyses of cyclodextrins and cyclodextrin complexes. Environ Chem Lett. 2019;17(1):349–373. doi:10.1007/s10311-018-0806-8
- Fenyvesi É, Puskás I, Szente L. Applications of steroid drugs entrapped in cyclodextrins. Environ Chem Lett. 2019;17(1):375–391. doi:10.1007/s10311-018-0807-7
- Tian B, Liu J. The classification and application of cyclodextrin polymers: a review. New J Chem. 2020;44(22):9137–9148. doi:10.1039/c9nj05844c
- Heo DN, Ko W-K, Moon H-J, et al. Inhibition of osteoclast differentiation by gold nanoparticles functionalized with cyclodextrin curcumin complexes. ACS Nano. 2014;8(12):12049–12062.
- Möller K, Macaulay B, Bein T. Curcumin encapsulated in crosslinked cyclodextrin nanoparticles enables immediate inhibition of cell growth and efficient killing of cancer cells. Nanomaterials. 2021;11(2):1–21. doi:10.3390/nano11020489
- Lee D, Ko WK, Hwang DS, et al. Use of baicalin-conjugated gold nanoparticles for apoptotic induction of breast cancer cells. Nanoscale Res Lett. 2016;11(1):381. doi:10.1186/s11671-016-1586-3
- Chen Y, Li N, Yang Y, Liu Y. A dual targeting cyclodextrin/gold nanoparticle conjugate as a scaffold for solubilization and delivery of paclitaxel. RSC Adv. 2015;5(12):8938–8941. doi:10.1039/c4ra13135e
- Silva N, Riveros A, Yutronic N, et al. Photothermally controlled methotrexate release system using β-cyclodextrin and gold nanoparticles. Nanomaterials. 2018;8(12):1–15. doi:10.3390/nano8120985
- Aykaç A, Martos-Maldonado MC, Casas-Solvas JM, et al. Β-Cyclodextrin-bearing gold glyconanoparticles for the development of site specific drug delivery systems. Langmuir. 2014;30(1):234–242. doi:10.1021/la403454p
- Hoshikawa A, Nagira M, Tane M, Fukushige K, Tagami T, Ozeki T. Preparation of curcumin-containing α-, β-, and γ-cyclodextrin/ polyethyleneglycol-conjugated gold multifunctional nanoparticles and their in vitro cytotoxic effects on A549 cells. Biol Pharm Bull. 2018;41(6):908–914. doi:10.1248/bpb.b18-00010
- Hu M, Chen J, Li ZY, et al. Gold nanostructures: engineering their plasmonic properties for biomedical applications. Chem Soc Rev. 2006;35(11):1084–1094. doi:10.1039/b517615h
- Norsten TB, Frankamp BL, Rotello VM. Metal directed assembly of terpyridine-functionalized gold nanoparticles. Nano Lett. 2002;2(12):1345–1348. doi:10.1021/nl020217m
- Kolny J, Kornowski A, Weller H. Self-organization of cadmium sulfide and gold nanoparticles by electrostatic interaction. Nano Lett. 2002;2(4):361–364. doi:10.1021/nl0156843
- Boal AK, Rotello VM. Intra-and Inter monolayer hydrogen bonding in amide-functionalized alkanethiol self-assembled monolayers on gold nanoparticles. Langmuir. 2000;16(24):9527–9532.
- Si S, Mandal TK. pH-controlled reversible assembly of peptide-functionalized gold nanoparticles. Langmuir. 2007;23(1):190–195. doi:10.1021/la061505r
- Li D, He Q, Cui Y, Li J. Fabrication of pH-responsive nanocomposites of gold nanoparticles/poly(4- vinylpyridine). Chem Mater. 2007;19(3):412–417.
- Zhu M-Q, Wang L-Q, Exarhos GJ, Li ADQ. Thermosensitive gold nanoparticles. J Am Chem Soc. 2004;126(9):2656–2657. doi:10.1021/ja038544z
- Aslan K, Luhrs CC, Pérez-Luna VH. Controlled and reversible aggregation of biotinylated gold nanoparticles with streptavidin. J Phys Chem B. 2004;108(40):15631–15639. doi:10.1021/jp036089n
- Jin R, Wu G, Li Z, Mirkin CA, Schatz GC. What controls the melting properties of DNA-linked gold nanoparticle assemblies? J Am Chem Soc. 2003;125(6):1643–1654. doi:10.1021/ja021096v
- Sharma J, Chhabra R, Yan H, Liu Y. pH-driven conformational switch of “i-motif” DNA for the reversible assembly of gold nanoparticles. Chem Commun. 2007;2(5):477–479. doi:10.1039/b612707j
- Sudeep PK, Ipe BI, Thomas KG, et al. Fullerene-functionalized gold nanoparticles. A self-assembled Photoactive antenna-metal nanocore assembly. Nano Lett. 2002;2(1):29–35. doi:10.1021/nl010073w
- Banerjee IA, Yu L, Matsui H. Application of host-guest chemistry in nanotube-based device fabrication: photochemically controlled immobilization of azobenzene nanotubes on patterned α-CD monolayer/Au substrates via molecular recognition. J Am Chem Soc. 2003;125(32):9542–9543. doi:10.1021/ja0344011
- Liu Z, Jiang M. Reversible aggregation of gold nanoparticles driven by inclusion complexation. J Mater Chem. 2007;17(40):4249–4254. doi:10.1039/b707910a
- Liu Y, Song S-H. Cyclodextrin-modified gold nanoparticle aggregate formed by simple host-guest interactions with 1,10-phenanthroline. J Chem Res. 2004;3(2):152–153. doi:10.3184/030823404323000567
- Ye BF, Zhao YJ, Cheng Y, et al. Colorimetric photonic hydrogel aptasensor for the screening of heavy metal ions. Nanoscale. 2012;4(19):5998–6003. doi:10.1039/c2nr31601c
- Lyu D, Chen S, Guo W. Liposome Crosslinked Polyacrylamide/DNA Hydrogel: a Smart Controlled-Release System for Small Molecular Payloads. Small. 2018;14(15):1–8. doi:10.1002/smll.201704039
- Davis ME, Pun SH, Bellocq NC, et al. Self-assembling nucleic acid delivery vehicles via linear, water-soluble, cyclodextrin-containing polymers. Curr Med Chem. 2004;11(2):179–197. doi:10.2174/0929867043456179
- Costa D, Valente AJM, Miguel MG, Queiroz J. Plasmid DNA hydrogels for biomedical applications. Adv Colloid Interface Sci. 2014;205:257–264. doi:10.1016/j.cis.2013.08.002
- Liu Y, Wang H, Liang P, Zhang HY. Water-soluble supramolecular fullerene assembly mediated by metallobridged β-cyclodextrins. Angew Chemie Int Ed. 2004;43(20):2690–2694. doi:10.1002/anie.200352973
- Li F, Wang C, Guo W. Multifunctional poly-N-isopropylacrylamide/DNAzyme microgels as highly efficient and recyclable catalysts for biosensing. Adv Funct Mater. 2018;28(10):1–8. doi:10.1002/adfm.201705876
- Mahalingam V, Onclin S, Péter M, Ravoo BJ, Huskens J, Reinhoudt DN. Directed self-assembly of functionalized silica nanoparticles on molecular printboards through multivalent supramolecular interactions. Langmuir. 2004;20(26):11756–11762. doi:10.1021/la047982w
- Pun SH, Bellocq NC, Liu A, et al. Cyclodextrin-modified polyethylenimine polymers for gene delivery. Bioconjug Chem. 2004;15(4):831–840.
- Kihara F, Arima H, Tsutsumi T, Hirayama F, Uekama K. In vitro and in vivo gene transfer by an optimized α-cyclodextrin conjugate with polyamidoamine dendrimer. Bioconjug Chem. 2003;14(2):342–350. doi:10.1021/bc025613a
- Forrest ML, Gabrielson N, Pack DW. Cyclodextrin-Polyethylenimine conjugates for targeted in vitro gene delivery. Biotechnol Bioeng. 2004;89(4):416–423. doi:10.1002/bit.20356
- Park I-K, von Recum HA, Jiang S, Pun SH. Supramolecular assembly of cyclodextrin-based nanoparticles on solid surfaces for gene delivery. Langmuir. 2006;22(20):8478–8484. doi:10.1021/la061757s
- Wang H, Chen Y, Li XY, Liu Y. Synthesis of oligo(ethylenediamino)-β-cyclodextrin modified gold nanoparticle as a DNA concentrator. Mol Pharm. 2007;4(2):189–198. doi:10.1021/mp060045s
- Qiu J, Kong L, Cao X, Li A, Tan H, Shi X. Dendrimer-entrapped gold nanoparticles modified with β-cyclodextrin for enhanced gene delivery applications. RSC Adv. 2016;6(31):25633–25640. doi:10.1039/c6ra03839e
- Qiu J, Kong L, Cao X, et al. Enhanced delivery of therapeutic siRNA into glioblastoma cells using dendrimer-entrapped gold nanoparticles conjugated with β-cyclodextrin. Nanomaterials. 2018;8(131):1–11. doi:10.3390/nano8030131
- Zhao D, Chen Y, Liu Y. Construction and DNA condensation of cyclodextrin-coated gold nanoparticles with anthryl grafts. Chemistry. 2014;9:1895–1903. doi:10.1002/asia.201402078
- Li X, Qi Z, Liang K, et al. An artificial supramolecular nanozyme based on β-cyclodextrin-modified gold nanoparticles. Catal Letters. 2008;124(3–4):413–417. doi:10.1007/s10562-008-9494-5
- Cao R, Villalonga R, Fragoso A. Towards nanomedicine with a supramolecular approach: a review. IEEE Proc Nanobiotechnol. 2005;152(5):159–164. doi:10.1049/ip-nbt
- Villalonga R, Fragoso A, Cao R, Ortiz PD, Villalonga ML, Damiao AE. Supramolecular-mediated immobilization of trypsin on cyclodextrin-modified gold nanospheres. Supramol Chem. 2005;17(5):387–391. doi:10.1080/10610270500126743
- Villalonga R, Tachibana S, Cao R, Ortiz PD, Gomez L, Asano Y. Supramolecular-mediated immobilisation of L-phenylalanine dehydrogenase on β-cyclodextrin-modified gold nanospheres. J Exp Nanosci. 2006;1(2):249–260. doi:10.1080/17458080600684487
- Zhao Y, Huang Y, Zhu H, Zhu Q, Xia Y. Three-in-one: sensing, self-assembly, and cascade catalysis of cyclodextrin modified gold nanoparticles. J Am Chem Soc. 2016;138(51):16645–16654. doi:10.1021/jacs.6b07590
- Zhou DH, Liang CC, Nie J, Zhu XQ. Construction of a repairable fixed porous catalytic bed loaded with gold nanoparticles via multivalent host-guest interactions. ACS Sustain Chem Eng. 2017;5(9):7587–7593. doi:10.1021/acssuschemeng.7b00879
- An P, Xue X, Rao H, et al. Gold nanozyme as an excellent co-catalyst for enhancing the performance of a colorimetric and photothermal bioassay. Anal Chim Acta. 2020;1125:114–127. doi:10.1016/j.aca.2020.05.047
- Ling XY, Reinhoudt DN, Huskens J. Reversible attachment of nanostructures at molecular printboards through supramolecular glue. Chem Mater. 2008;20(11):3574–3578. doi:10.1021/cm703597w
- Ludden MJW, Reinhoudt DN, Huskens J. Molecular printboards: versatile platforms for the creation and positioning of supramolecular assemblies and materials. Chem Soc Rev. 2006;35(11):1122–1134. doi:10.1039/b600093m
- Beulen MWJ, Bügler J, Lammerink B, et al. Self-assembled monolayers of heptapodant-cyclodextrins on gold. Langmuir. 1998;14:6424–6429.
- Onclin S, Mulder A, Huskens J, Ravoo BJ, Reinhoudt DN. Molecular printboards: monolayers β-cyclodextrins on silicon oxide surfaces. Langmuir. 2004;20(13):5460–5466. doi:10.1021/la049561k
- Huskens J, Deij MA, Reinhoudt DN. Attachment of molecules at a molecular printboard by multiple host-guest interactions. Angew Chemie Int Ed. 2002;41(23):4467–4471. doi:10.1002/1521-3773(20021202)41:23<4467::AID-ANIE4467>3.0.CO;2-M
- Crespo-Biel O, Dordi B, Reinhoudt DN, Huskens J. Supramolecular layer-by-layer assembly: alternating adsorptions of guest- and host-functionalized molecules and particles using multivalent supramolecular interactions. J Am Chem Soc. 2005;127(20):7594–7600. doi:10.1021/ja051093t
- Crespo-Biel O, Jukovic A, Karlsson M, Reinhoudt DN, Huskens J. Multivalent aggregation of cyclodextrin gold nanoparticles and adamantyl-terminated guest molecules. Isr J Chem. 2005;45(3):353–362. doi:10.1560/af3p-k2a6-mdck-1678
- Maury P, Crespo-Biel O, Péter M, Reinhoudt DN, Huskens J. Integration of top-down and bottom-up nanofabrication schemes. Mater Res Soc Symp Proc. 2005;901:441–449. doi:10.1557/proc-0901-rb12-01
- Ling XY, Phang IY, Reinhoudt DN, Vancso GJ, Huskens J. Supramolecular layer-by-layer assembly of 3D multicomponent nanostructures via multivalent molecular recognition. Int J Mol Sci. 2008;9(4):486–497. doi:10.3390/ijms9040486
- Crespo-Biel O, Péter M, Bruinink CM, Ravoo BJ, Reinhoudt DN, Huskens J. Multivalent host-guest interactions between β-cyclodextrin self-assembled monolayers and poly(isobutene-alt-maleic acid)s modified with hydrophobic guest moieties. Chem - a Eur J. 2005;11(8):2426–2432. doi:10.1002/chem.200400393
- Zuo F, Luo C, Zheng Z, Ding X, Peng Y. Supramolecular assembly of β-cyclodextrin-capped gold nanoparticles on ferrocene-functionalized ITO surface for enhanced voltammetric analysis of ascorbic acid. Electroanalysis. 2008;20(8):894–899. doi:10.1002/elan.200704113
- Gómez-Graña S, Pérez-Juste J, Hervés P. Cyclodextrins and inorganic nanoparticles: another tale of synergy. Adv Colloid Interface Sci. 2021;288:102338. doi:10.1016/j.cis.2020.102338
- Yun Y. Electrochemical sensor for ultrasensitive determination of bisphenol a based on gold nanoparticles/β-cyclodextrin functionalized reduced graphene oxide nanocomposite. Int J Electrochem Sci. 2016;11(4):2778–2789. doi:10.20964/110402778
- Wu H, Fang F, Wang C, Hong X, Chen D, Huang X. Selective molecular recognition of low density lipoprotein based on β-cyclodextrin coated electrochemical biosensor. Biosensors. 2021;11(7):1–10. doi:10.3390/bios11070216
- Chen M, Diao G. Electrochemical study of mono-6-thio-β-cyclodextrin/ferrocene capped on gold nanoparticles: characterization and application to the design of glucose amperometric biosensor. Talanta. 2009;80(2):815–820. doi:10.1016/j.talanta.2009.07.068
- Díez P, Piuleac CG, Martínez-Ruiz P, et al. Supramolecular immobilization of glucose oxidase on gold coated with cyclodextrin-modified cysteamine core PAMAM G-4 dendron/Pt nanoparticles for mediatorless biosensor design. Anal Bioanal Chem. 2013;405(11):3773–3781. doi:10.1007/s00216-012-6491-8
- Zheng X, Li L, Cui K, et al. Ultrasensitive enzyme-free biosensor by coupling cyclodextrin functionalized Au nanoparticles and high-performance Au-paper electrode. ACS Appl Mater Interfaces. 2018;10(4):3333–3340. doi:10.1021/acsami.7b17037
- Zhang NMY, Qi M, Wang Z, et al. One-step synthesis of cyclodextrin-capped gold nanoparticles for ultra-sensitive and highly-integrated plasmonic biosensors. Sensors Actuators, B Chem. 2019;286:429–436. doi:10.1016/j.snb.2019.01.166
- Luo S, Wu Y, Mou Q, Li J, Luo X. A thio-β-cyclodextrin functionalized graphene/gold nanoparticle electrochemical sensor: a study of the size effect of the gold nanoparticles and the determination of tetrabromobisphenol A. RSC Adv. 2019;9(31):17897–17904. doi:10.1039/c9ra02614b
- Rajamanikandan R, Lakshmi AD, Ilanchelian M. Smart phone assisted, rapid, simplistic, straightforward and sensitive biosensing of cysteine over other essential amino acids by β-cyclodextrin functionalized gold nanoparticles as a colorimetric probe. New J Chem. 2020;44(28):12169–12177. doi:10.1039/d0nj02152k
- Kapan B, Kurbanoglu S, Esenturk EN, Soylemez S, Toppare L. Electrochemical catechol biosensor based on β-cyclodextrin capped gold nanoparticles and inhibition effect of ibuprofen. Process Biochem. 2021;108:(June):80–89. doi:10.1016/j.procbio.2021.06.004
- Fang A, Feng D, Luo X, Shi F. Gold nanoparticles prepared with cyclodextrin applied to rapid vertical flow technology for the detection of Brucellosis. Biosensors. 2022;12(7):75. doi:10.3390/bios12070531
- Peng L, You M, Wu C, et al. Reversible phase transfer of nanoparticles based on photoswitchable host-guest chemistry. ACS Nano. 2014;8(3):2555–2561. doi:10.1021/nn4061385
- Tokuyama H, Yamago S, Nakamura E, Shiraki T, Sugiura Y. Photoinduced biochemical activity of fullerene carboxylic acid. J Am Chem Soc. 1993;115(17):7918–7919. doi:10.1021/ja00070a064
- Liu Y, Zhao YL, Chen Y, Liang P, Li L. A water-soluble β-cyclodextrin derivative possessing a fullerene tether as an efficient photodriven DNA-cleavage reagent. Tetrahedron Lett. 2005;46(14):2507–2511. doi:10.1016/j.tetlet.2005.01.181
- Xie SY, Bin HR, Yu LJ, Ding J, Zheng LS. Microwave synthesis of fullerenes from chloroform. Appl Phys Lett. 1999;75(18):2764–2766. doi:10.1063/1.125142
- Murthy CN, Geckeler KE. The water-soluble β-cyclodextrin-[60]fullerene complex. Chem Commun. 2001;1(13):1194–1195. doi:10.1039/b102142g
- Liu Y, Yang YW, Chen Y. Thio[2-(benzoylamino)ethylamino]-β-CD fragment modified gold nanoparticles as recycling extractors for [60]fullerene. Chem Commun. 2005;2(33):4208–4210. doi:10.1039/b507650a
- Liu J, Alvarez J, Ong W, Kaifer AE. Network aggregates formed by C60 and gold nanoparticles capped with γ-cyclodextrin hosts. Nano Lett. 2001;1(2):57–60. doi:10.1021/nl0001813
- Liu Y, Yang YW, Chen Y, Zou HX. Polyrotaxane with cyclodextrins as stoppers and its assembly behavior. Macromolecules. 2005;38(13):5838–5840. doi:10.1021/ma047327v
- Liu Y, Wang H, Chen Y, Ke CF, Liu M. Supramolecular aggregates constructed from gold nanoparticles and L-Try-CD polypseudorotaxanes as captors for fullerenes. J Am Chem Soc. 2005;127(2):657–666. doi:10.1021/ja046294w