Abstract
Extracellular vesicles (EVs) are nanoscale bilayer phospholipid membrane vesicles released by cells. Contained large molecules such as nucleic acid, protein, and lipid, EVs are an integral part of cell communication. The contents of EVs vary based on the cell source and play an important role in both pathological and physiological conditions. EVs can be used as drugs or targets in disease treatment, and changes in the contents of EVs can indicate the progression of diseases. In recent years, with the continuous exploration of the structure, characteristics, and functions of EVs, the potential of engineered EVs for drug delivery and therapy being constantly explored. This review provides a brief overview of the structure, characteristics and functions of EVs, summarizes the advanced application of EVs and outlook on the prospect of it. It is our hope that this review will increase understanding of the current development of medical applications of EVs and help us overcome future challenges.
Introduction
Extracellular vesicles (EVs) were originally described as a general term for all extracellular particles of unknown origin.Citation1 Although evidence of their existence has been available for more than 80 years, it is only in recent decades that research into their production, function, and potential application has begun to emerge. Early studies on EVs received little attention from scientists, and EVs were generally regarded as a waste product of cell metabolism.Citation2 In 1987, Rose M. Johnstone defined a material produced by the release pathway of polyvesicles after fusion with the cell membrane as exosomes. At the time, exosomes were believed to be just a type of “garbage can”.Citation3,Citation4 Later, a breakthrough was made in the study of the outer membrane of cells. Scientists found that the function of exosomes was not limited to the transport of metabolic waste. For example, exosomes have enzyme activities that promote blood clotting and antigen presentation, and there is interaction between bacteria-derived exosomes and human intestinal cells.Citation5,Citation6 Among them, exosomes, play the most important role in anti-tumor immunity. Dendritic cell-derived exosomes (Dex) can carry cancer cell antigens and be utilized for tumor treatment, which has been verified by clinical experiments.Citation7 Since then, the field of EVs has gradually gained the attention in the fields of cell biology and biotechnology, especially has experienced rapid development in the past 20 years.Citation8 In 2018, the International Extracellular Vesicle Association (ISEV) published the Minimal Information for Studies of Extracellular Vesicles (MISEV 2018) to better isolate and classify different types of EVs. It provides a reference and research paradigm for EVs research.Citation9
Figure 2 Advanced application of EVs. A. Direct therapeutic B. Indirect therapeutic C. Block EVs associated with diseases D. Block the uptake of EVs associated with diseases E. Physical elimination of EVs associated with diseases F. Engineered EVs: drug delivery G. Engineered EVs: Internal modification H. Engineered EVs: parent cell surface modification I. Engineered EVs: EVs surface modification.
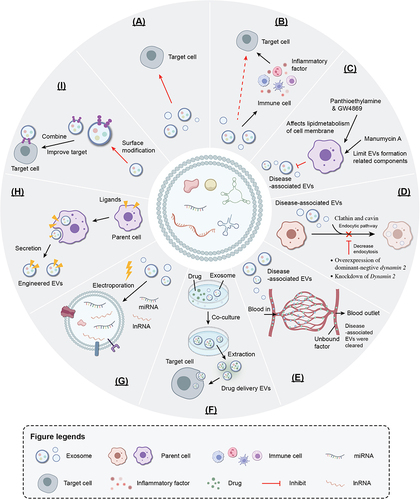
In recent years, there has been an increasing clarity in understanding the relationship between the components of EVs from different cell sources and their biological functions. Meanwhile, advancements in click chemistry and biological orthogonal technology have led to a surge in application studies that focus on EV components and biological characteristics. The applications can be roughly divided into the following three classes based on their basis: 1. Application based on EVs’ biological function (pure application, treatment or blocking). 2. Applications based on EVs’ biological properties (membrane structure, engineered exosomes). 3. Applications based on the relationship between EVs and diseases (diagnostic application).
In this review, the characteristics and functions of EVs are briefly described. And the main cutting-edge applications of EVs in disease therapy, engineering, and diagnosis in recent years are reviewed.
Characteristics of Extracellular Vesicles
Structure
EVs are nanoscale bilayer phospholipid membrane vesicles covered by membranes. There are lipid rafts on the vesicles that restrict the membrane fluidity. The vesicles contain large molecules such as nucleic acid, protein, and lipid.Citation10,Citation11 EVs are released by most cells type and can be isolated from various body fluids, including blood, cerebrospinal fluid, tears.Citation12,Citation13
EVs are diverse and can be divided into three subtypes based on their cell origin, biogenesis, size and function to receptors: Exosomes (50–150nm EVs from the endocytic pathway); Microvesicles (MVs) (200–800 nm EVs from plasma membrane budding); Apoptotic bodies (diameter > 1μm EVs from migratory and apoptotic cells).Citation14–16 Most current research has focused on the first two types of EVs.
EVs contain proteins that are related to membrane transport, such as attached proteins, Rab GTPases, flotillins, and multivesicular body (MVB)-production proteins. Exosomes also contain various nucleic acids, including microRNAs (miRNAs) and long non-coding RNAs (lncRNAs), which perform as regulators of gene expression and could be used as biomarkers.Citation17 Lipid components in exosomes contain sphingomyelin (SM), phosphatidylserine (PS), phosphatidylinositol (PI), phosphatidylacid (PA), ceramides, and cholesterin.Citation18 The bilayer phospholipid membrane of EVs contain phosphatidylcholine and sphingolipin in the outer layer and PS and phosphatidylethanolamine (PE) in the inner layer. The lipid in the outer layer helps protect EVs from hydrolysis by enzymes in different body fluids and under different potential of hydrogen (PH) values.Citation19
Recent studies have shown that components of exosomes are heterogeneous and depended on the source cells that secrete them.Citation11,Citation20 For example, a proteomic analysis showed that exosomes secreted by breast cancer epithelial and mesenchymal cells contain completely different proteins and nucleic acids.Citation21 The cholesterin and phospholipids in exosomes secreted by cancer cells and non-cancer cells are also significantly different.Citation22
Biological Genesis Mechanism
EVs have the following mechanism: the formation of exosomes begins with endocytosis on the cell membrane surface, and early endosomes are formed through inward budding. As these early endosomes mature into late endosomes, they then form multiple intraluminal vesicles (ILVs). During this process, RNA, DNA, and lipids are actively and selectively incorporated into the ILVs.Citation23 The ILVs then sprout inside the cell and form the MVB. Most MVBs fuses with the lysosome, resulting in degradation of their contents. Under the regulation of Rab27a and Rab27b enzymes, a few MVBs that contain CD63, lysosomal-associated membrane protein 1 (LAMP1), and LAMP2 are exocytosed through fusion with the cell membrane or degradation pathways, and their contents are wrapped in vesicles and released outside the cell to form exosomes.Citation24,Citation25
The biological genesis mechanism of ILVs and MVB are driven by the endosomal sorting complex required for transport (ESCRT). This is a multiprotein complex consisting of thirty proteins.Citation26 The primary function of ESCRT is to classify specific cargo into ILVs, which serve as precursors to exosomes.Citation27 The process of MVBs fusing with the cell membrane and releasing exosomes into body fluids is mainly dependent on the Rab family and the soluble N-ethylmaleimide-sensitive factor attachment protein receptor (SNARE) family. The SNARE and Rabs control the germination, transport, and localization of intracellular vesicles on the plasma membrane.Citation24,Citation28–30 MVs are formed through outward budding of the plasma membrane and finally breaking off under the action of ARF6, RhoA, and Rab22A.Citation31,Citation32
Physiological Function
EVs are an integral part of cell communication. Cells release EVs into the extracellular space to participate in cell biological functions, and they hold an important status in pathophysiological conditions.Citation33 EVs interact with receptor cells through three primary mechanisms of entry into cells: 1. Binding to surface receptors triggers a signaling cascade that complements classical paracrine signaling of secreted soluble factors;Citation34 2. Endocytosis, phagocytosis or macropinocytosis;Citation35,Citation36 3. Fusion with cells to deliver substances directly to the cytoplasmic membrane and cytoplasmic matrix, delivering large amounts of functional biomolecules to neighboring cells.Citation37
Under physiological conditions, EVs play a crucial role in transmitting intercellular signals that regulate the function of other cells and maintain physiological homeostasis, such as angiogenesis, cell migration, and immune regulation.Citation38 For instance, exosomes can influence angiogenesis by regulating the expression of miR-424, miR-31-5p, and miR-21-3p.Citation39–41 Additionally, exosomes secreted by cells can induce the migration of parent cells. Developments in imaging and labeling techniques have made it possible to capture of exosome secretion and internal trafficking events in both parent and recipient cells. A study have demonstrated the role of EVs in cell migration through the use of a novel bicolor reporter. The results showed that exosomes are secreted at the front end of migrating cells, and the cells move along the exosome trail with intense pathfinding behavior.Citation42
Exosomes are also involved in immunomodulation, with their regulatory mechanisms primarily involving direct action on target cells to initiate downstream signals, as well as miRNA-mediated regulation.Citation43 There are Fas Ligand (FasL) and Tumor Necrosis Factor-Related Apoptosis-Inducing Ligand (TRAIL) expressed by placental tissue and exosomes, respectively. These signaling molecules trigger T cell apoptosis through contact with target cells, thus maintaining normal pregnancy.Citation44 Another study found that the main component of placental-exosome miRNA, C19MCmiRNA, can inhibit the proliferation of viruses and induce self-phagocytosis, endowing the fetus with antiviral protection.Citation45 Dex can migrate to tumors or present antigens to T cells, inducing an immune response.Citation46 For example, Dex can improve the tumor microenvironment (TME) of hepatocellular carcinoma (HCC), leading to the increase of immune-stimulating cytokines and the infiltration of CD8 cytotoxic T cell, and the decrease of immunosuppressive cytokines and regulatory T-cell (Tregs).Citation47 As more studies are conducted, more functions of EVs are being discovered, suggesting that they may play a more crucial role in normal physiological activities than previously thought.
Pathological Effect
In the pathological process, EVs can enhance tumor cell invasion, cardiovascular disease, and pathogen infections.Citation48–52 Exosomes derived from tumor cells act as signal sensors or messengers, regulating communication between tissues and cells. This regulation contributes to the migration and growth of tumor cells, modification of the microenvironment, metastasis, and invasion.Citation53–55 Cancer cells may secrete different types of exosomes, depending on their genetic background and the stage of cancer. Primary tumors may contain heterogeneous populations of cancer cells with different invasive abilities, and communication between these populations can occur through the potential pathway of EVs. This creates ideal conditions for cancer cell proliferation and invasion, and enhances the invasion of low-grade cancer cell populations through the influence of high-grade cancer cells, promoting the invasion and metastasis of the tumor.Citation54,Citation56 The cancer pre-metastatic niche is an important link in cancer metastasis. Studies have shown that in colorectal cancer, cancer cells transfer metastasis-promoting miRNA (miR-25-3p) to endothelial cells through exosomes, targeting KLF2 and KLF4 to promote endothelial angiogenesis and increase vascular permeability.Citation49 The creation of TME around cancer cells is closely related to the EVs produced by cancer cells. These EVs can recruit fibroblasts through the release of signals, promoting the transformation of mesenchymal stem cells into myofibroblasts and the formation of endothelial cells into blood vessels. All these behaviors promote the creation of TME.Citation57,Citation58 EVs are essential to the immunological escape mechanism of tumor cells. The interaction of PD-L1 (CD274)/PD-1 (PDCD1) can inhibit the T cell response in TME, and peripheral blood T cells can be stimulated to produce IL-10 to mediate immunosuppression. PD-L1 is a critical checkpoint molecule that suppresses the immune response and accelerates tumor growth by allowing tumor cells to evade detection by the immune system.Citation59 In the TME, cancer cells release exosomes that can increase the expression of PD-L1 around the cell population, leading to T cell apoptosis and inhibition of the immune response.Citation60 The TME also contains cancer-associated fibroblasts (CAFs), which play a key role in tumors growth. MicroRNA-92 in the exosomes of breast cancer CAFs bind to target gene LATS2 in cancer cells, increasing PD-L1 expression and T cell apoptosis.Citation61 Moreover, human lung tumor cells produce epithelial cell adhesion molecule+ (EpCAM+) exosomes that can regulate the phenotype of immune cells in the TME, leading to the preference of M2 phenotype in TME-infiltrating macrophages, which is associated with tumor progression and survival.Citation62 T-cell immunity is reduced in patients with chronic lymphocytic leukemia (CLL). Leukemia cells can also promote disease development through paracrine exosome transport, with recent studies showing that CLL-induced myeloid-derived suppressor cells (MDSCs) secrete miR-155 exosomes that bind to T-cells and inhibit Treg activity, allowing the leukemia to escape immune system attack and spread.Citation63 In airway inflammation, EVs secreted by the airway are involved in the pathogenesis of allergic airway inflammation under allergen exposure, and EVs in bronchoalveolar lavage fluid of asthmatic patients induce significantly higher levels of IL-8 in bronchial epithelial cells.Citation64 In the pathological condition of atherosclerosis, smooth muscle cells of the cardiovascular tissue release EVs containing calcification-promoting cargo, which become risk factors for plaque formation when released into the extracellular matrix.Citation50,Citation52 Macrophages stimulated by lipopolysaccharide during septicemia release more EVs containing proinflammatory cytokines, and this increase in cytokines in EVs is associated with the progression of myocardial dysfunction.Citation65 Exosomes are also involved in the transmission of some viruses. After the induction of Epstein-Barr virus, exosomes contain the viral BGLF2 protein and infect the uninfected receptor cells. In this process, exosomes can avoid the body’s immune surveillance and attack.Citation48 Related studies between exosomes and viruses have found that exosomes carrying RNA virus components can promote viral infection, and viruses may transfer proteins and miRNAs through exosomes. Some RNA viruses, such as human immunodeficiency virus (HIV) and human T-lymphotropic virus (HTLV), can rely on the ESCRT pathway that generates exosomes and use the double membrane of exosomes as acquired cysts to neutralize host antibodies and escape host immunity.Citation66 In terms of neurodegeneration, exosomes containing substances such as interferon and tumor necrosis factor (TNF) can destroy the blood-brain barrier and release their contents, leading to a decrease of the organization and tightness between the blood-brain barrier and brain endothelial cells. Exosomes also cause the migration of white blood cells, leading to detachment of the myelin sheath and multiple sclerosis.Citation67
Many research studies have been published on the role of EVs in cancer, trauma, autoimmune diseases, infectious diseases, and cardiovascular disease . In conclusion, it has become a new therapeutic strategy that promoting beneficial EVs and inhibiting the release of pathogenic EVs to mitigate the negative effects of disease. Above all, the structure, features, and functions of EVs are summarized in this review ().
EVs as Drug or Target in Therapeutic Application
As previously stated, researchers are investigating the role of EVs in pathophysiological conditions to enhance the positive stimulatory effect of EVs for a direct therapeutic effect. Current research focuses on mesenchymal stem cells, which are considered the “bank” of regenerative medicine due to their ability to evade the immune system, self-regenerate, and differentiate into various cell types with greater specificity than differentiated cells.Citation68–70 However, safety concerns have been raised regarding stem cell therapy, including ethical issues and the risk of teratoma formation with embryonic stem cell therapy, genomic instability and tumorigenesis with pluripotent stem cell therapy, and promoting tumor growth, metastasis, and differentiation into non-target tissues with mesenchymal stem cell therapy.Citation71–75 Encouragingly, recent evidence suggests that stem cells may exhibit beneficial effects through the EVs they release, playing a role similar to stem cells in tissue regeneration and damage repair.Citation69,Citation76 Furthermore, the secretions of stem cell-drived EVs are more controllable compared to stem cells, thus avoiding the risk of tumorigenesis.Citation77
Direct/Indirect Therapeutic
Current studies have shown that MSCs-drived EVs can promote tissue regeneration and regulate the immune response, which is expected to be an important part of cell-free therapy.Citation78–80 Nerve injury repair is always a difficult problem in tissue regeneration. Studies of nerve injury repair have shown that R28 retinal cells can endocytose MSC-EVs via the heparin sulfate proteoglycans (HSPG) receptor-mediated pathway on the cell surface. By competitively blocking the HSPG binding site on EVs, endocytosis of MSC-EVs in R28 retinal cells occurs by disrupting cell membrane cholesterol or dose-dependently blocking heparin, reducing neuroinflammation, and promoting retinal functional recovery.Citation81 Another study found that embryonic stem cells (ESCs)-drived EVs induced a significant increase in regulatory Tregs after stroke, possibly due to the enrichment of ESC-sEVTGF-β, Smad2, and Smad4 proteins in stem cell-EVs. These proteins can be delivered to activate the TGF-β/Smad pathway in CD4 T cells, inducing Treg amplification, regulating neuroinflammation, and promoting nerve recovery.Citation80 In studies of intervertebral disc degeneration, MSCs-derived exosomes inhibit reactive oxygen species (ROS) production and NLRP3 inflammasome activation while reducing nucleus pulposus cell apoptosis. Moreover, MSC-EVs can inhibit the expression of catabolic enzymes (MMP3, MMP13) and enhance anabolism (Col2a1, SOX). These findings suggest that MSC-EVs can alleviate the destruction of extracellular matrix in the nucleus pulposus and contribute to the alleviation of intervertebral disc degeneration.Citation82 The therapeutic effect of exosomes can also be achieved through the regulation of macrophages. In a study with osteoarthritis (OA), intraarticular injection of bone marrow stem cells (BMSCs)-derived exosomes can significantly downregulated inflammatory cytokines IL-1β, TNF-a, and IL-6 while promoting the expression of the anti-inflammatory cytokines IL-10 and TGF-1. By the way, BMSC-derived exosomes also reduced synovitis cell infiltration, decreased damage to articular cartilage, and delayed the progression of OA.Citation83 Exosomes can promote the transformation of macrophages from M1 macrophages to M2 macrophages in the synovium of joint and inhibit chondrocyte hypertrophy and degeneration caused by M1 macrophages.Citation84 Parturition is an important process of human reproduction and development. During parturition, human umbilical cord mesenchymal stem cell (UMSC)-derived exosomes can release HIPK3. As miR-421/FOXO3a is a direct target of circular IPK3, UMSC- derived exosomes can regulate miR-421 and increase the expression of FOXO3a, thereby inhibiting phosphorylation and release of IL-1β and IL-18, and protecting the process of human parturition.Citation85 In addition to the parturition process, heart health is also critical. In a mice model of myocardial infarction, EVs derived from cardiosphere-derived cells (CDCs) were enriched with miR-146a, which can improve cardiac function by enhancing cardiomyocyte viability and preventing oxidative stress.Citation86
Block EVs Associated with Diseases
Although there may be therapeutic effects of EVs, it is important to note that EVs are also involved in disease progression. Therefore, current research is focusing on how to identify and block major EV subgroups that are associated with specific diseases, without affecting those EVs that perform important physiological functions. In tumor therapy, current research is investigating how to regulate the release of cancer cell-derived exosomes, block the uptake of specific exosomes by recipient cells, and remove exosomes from body fluids.Citation87 Researchers have found that in cancer cells overexpressed with the Rab27a gene, the enrichment of immunoactivating molecules, such as Hsp70 or Hsp90, in exosomes can induce a stronger anti-tumor immune response and inhibit tumor invasion.Citation88 In addition to gene regulation, other pathways have also been explored for exosome regulation. For example, calcitonin inhibits EV release and causes the accumulation of docetaxel and methotrexate within tumor cells, resulting in a significant reduction in tumor cell proliferation.Citation89,Citation90 Ras enzyme, ESCRT, and hnRNP H1 all play a key role in exosome release. Manumycin A can inhibit these substances, thereby inhibiting exosomes secretion.Citation91–93
Compounds associated with lipid metabolism include pantethine and GW4869. Pantethine affects the membrane lipid bilayer recombination process in MV formation by reducing lipid metabolism and total cholesterol levels, which inhibits the release of MV from the membrane.Citation94,Citation95 For example, in the brain syndrome model of Plasmodium bergi Anka-infected mice, the disulfur group in the molecular structure of pantethine inhibits EV release from infected cells, thus preventing the occurrence of cerebral malaria.Citation96 GW4869 is a dihydroimidazolamide compound that affects lipid metabolism, and can be used as a non-competitive inhibitor of membrane lipid neutral sphingomyelinase (nSMase) to indirectly inhibit exosome release. In melanoma studies, B16BL6 melanoma cells treated with GW4869 showed significant growth inhibition compared to the control group, which may be related to the inhibition of GW4869 on exosome secretion, while also inhibiting the autosecretory regulatory proliferation of mouse B16BL6 cells.Citation97 Moreover, the drug has been shown to reverse ischemic preconditioning (IPC)-mediated heart damage, improve postoperative cognitive dysfunction caused by miR-182-5p in exosomes, and reduce the survival rate of pancreatic cancer (PC) cells.Citation87,Citation98–102
Block the Uptake of EVs Associated with Diseases
Inhibiting exosome uptake is a potential method of regulating exosome activity. Cells take up exosomes through pathways such as macrocytosis and phagocytosis.Citation103–105 Current studies have shown that treating exosomes with heparin and protease K can significantly reduce their uptake and internalization by cancer cells. This indicates that heparan sulfate (HS) proteoglycans and exosome surface proteins play a role in exosome uptake.Citation106–108 Furthermore, exosome uptake is related to clathrin and cavin endocytosis pathways, and knocking down dynamin2 or overexpressing a dominant-negative form of dynamin2 can inhibit exosome uptake.Citation104
Physical Elimination of EVs Associated with Diseases
Another successful strategy for treating cancer is the physical elimination of exosomes that are secreted by cancer cells. As previously mentioned, cellular communication is mainly through the inclusion of exosomes and uptake by endocytosis, which is particularly significant in tumor cell communication.Citation109,Citation110 Therefore, the use of a hemofiltration system that target cancer cell exosomes by specifically targeting the human epidermal growth factor receptor 2 (HER2) on their surface could play an important role in blocking communication between cancer cells and inhibiting cancer cell invasion.Citation111,Citation112
Even though the above studies have proven that the regulation of detrimental EVs can be used reliably as therapeutic methods, further studies on their effects on EV release in healthy cells are still needed (). Precise delivery of EV inhibitors to cancer cells is required to minimize the impact on normal tissue cells. Ultimately, the suitability of exosomes as therapeutic agents must be determined by the benefit-to-risk ratio.
Table 1 Examples of Exosomes for Therapeutic Applications
EVs as Disease Marker in Diagnostic Application
Solid biopsy is considered the gold standard for pathological diagnosis. In clinical practice, it is necessary to perform solid biopsy to determine the histology and staging of tumor, as these pathological results serve as the basis for cancer diagnosis and treatment.Citation113 Early detection of tumor markers is beneficial for early cancer diagnosis and plays an important role in the early detection and treatment, precision medicine, efficacy monitoring, and prognosis prediction. However, solid biopsies are invasive and pose a risk of bleeding and infection. Additionally, the small tissue samples obtained from solid biopsies have limited ability to effectively monitor dynamic tumor progression due to the heterogeneity of tumors both spatially and temporally. Morever, invasive detection methods may also increase the risk of metastasis and negatively impact survival and prognosis.Citation114,Citation115 To address the limitations of solid biopsies, liquid biopsies are being promoted as a complement to solid biopsies. These minimally invasive liquid biopsies allow for real-time cancer diagnosis and monitoring, leading to early detection and reduced cancer mortality.Citation11 Exosomes, which are widely distributed and highly stable, are attractive as liquid biopsies that can reflect the overall status of tumors in real time.Citation11,Citation116 Thus, the identification of highly effective liquid biopsy biomarkers holds promise for the non-invasive diagnosis of cancer. Currently, the diagnosis of central nervous system diseases, cancer, and cardiovascular diseases is a critical area of research and application for EVs.Citation117,Citation118
Protein
Specific proteins can be found in exosomes, and these specific proteins can be used as a basis for fluid diagnosis. Studies have shown that these proteins are valuable in detecting neuron-associated mutations in serum exosomes. For instance, research on exosomes in the plasma of patients with Parkinson’s disease (PD) has shown that the concentrations of astrocytes and oligodendrocyte derived-exosomes, as well as apolipoprotein A1 in the serum of patients with early PD, are correlated with disease progression and consistent with the severity of the disease.Citation119,Citation120 In addition, plasma exosome detection also plays a role in the identification of other diseases. HSP90 levels in SOD1G93A astrocytes and peripheral blood mononuclear cells were found to be lower in amyotrophic lateral sclerosis (ALS) mice than in other atrophic diseases, suggesting that ALS patients can be distinguished from spinal and bulbar muscular atrophy (SBMA) by measuring HSP90 in plasma EVs.Citation121 These exosomes also contain specific proteins that can be used in the diagnosis of tumors. For instance, exosomes contain lectin galactoside-binding protein (LGALS3BP), which contributes to the proliferation and migration of endometrial cancer (EC) cells by activating the PI3K/AKT/VEGFA signaling pathway in vitro and in vivo. Therefore, elevated exosome levels, including LGALS3BP, provide a new perspective for the diagnosis of EC.Citation122
Different types of RNA play an important role in the development of diseases. When these RNAs are contained in exosomes and secreted into body fluids, they can serve as diagnostic tools for disease detection.
MiRNA
The miRNAs contained in exosomes can serve as biomarkers for diagnosis and prognosis, providing unique insights and a more dynamic perspective on the progression and therapeutic response of various diseases. For example, in the prediction of heart failure, significant changes in the exosome contents of miR-425, miR-744, and miR-92b-5p were detected earlier than conventional biomarkers, suggesting that monitoring exosomal contents may enable earlier detection of heart failure. Moreover, the expression level of these miRNAs increases with the severity of the disease.Citation123–125 Currently, several studies have found associations between exosomal miRNAs and cardiovascular diseases. For instance, exosome-miR-21-3p, exosome-miR-132, and exosome-miRNA-200 are associated with cardiac hypertrophy, while exosome-miR-106A and miR-24 are associated with aortic aneurysm. In acute myocardial infarction (AMI) patients, the plasma levels of miR-122-5p, miR-17-5p, miR-126-5p, and miR-145-3p were significantly increased.Citation126–134 These findings suggest that the discovery of miRNA in exosomes has the potential to contribute to the early diagnosis of cardiovascular diseases. In the diagnosis of cancer, exosomal miRNAs have been found to be valuable biomarkers for early detection. Low alpha-fetoprotein HCC enters the extracellular environment through the release of miR-21-5p by exosomes to participate in the tumorgenesis process, making the exosome miR-21-5p a biomarker for early diagnosis.Citation135 Exosomes containing miR-145, miR-155, and miR-382 were overexpressed in breast cancer cells, while the expression of miR-148a was down-regulated.Citation136,Citation137 These specific miRNAs have the potential to enable the early diagnosis of breast cancer. In the diagnostic application of metabolic diseases, acromegaly is an endocrine and metabolic disease caused by growth hormone secreting pituitary adenoma (GHPA). Studies have found that miR-21-5p contained in exosomes secreted by GHPA activates osteoblasts through the GH/IGF1 pathway and leads to osteogenesis, suggesting that exosome-derived miR-21-5p may be a candidate biomarker for acromegaly.Citation138,Citation139
CircRNA
CircRNA, a recently discovered non-coding RNA, has gained attention for its potential as a reliable diagnostic marker. CircRNA is highly stable and specific, and is not easily affected by the external environment, particularly when present in exosomes.Citation140 In a study comparing the circRNA profiles of breast cancer patients and benign patients, significant differences in expression patterns were found between the cancer patients and the control group. Nine specific circRNAs (circ_0002190, circ_0007177, circ_0000642, circ_0001439, circ_0001417, circ_0005552, circ_0001073, circ_0000267, and circ_0006404) were identified as potential circRNA markers for breast cancer.Citation141 In a study of plasma exosomes in patients with HCC, circ_0051443 was found to mediate the upregulation of Bri1-associated kinase 1 (BAK1) by competing with miR-331-3p, promoting apoptosis, and preventing the inhibition of HCC proliferation. The level of circ_0051443 in plasma exosomes of HCC patients was significantly lower than that in healthy individuals.Citation142,Citation143 Similarly, in the early diagnosis of gastric cancer, exosome circNRIP1 has been found to promote the proliferation, migration, and invasion abilities of gastric cancer cells in vivo through the AKT1/mTOR pathway and can be detected early by liquid detection.Citation144 Furthermore, highly expressed circGAPVD1 in plasma-derived exosomes of colorectal cancer (CRC) patients may become a novel diagnostic biomarker for CRC.Citation145 These findings suggest that circRNA in exosomes holds great potential as a diagnostic marker for various diseases, and further research is needed to explore its clinical applications.
lncRNA
Lately, lncRNAs have gained recognition for their potential as effective molecular markers for early cancer detection and as effective therapeutic targets for early cancer treatment. By separating exosomes from patients with early gastric cancer and healthy individuals, researchers have screened characteristic exosome lncRNAs as potential biomarkers for early gastric cancer through performing exosome lncRNA sequencing and diagnostic ability analysis.Citation146 Exosome lncRNAs also play a role in the diagnosis of diabetic retinopathy (DR). According to research, exosome lncRNAs DLX6-AS1 and PRINS have a high diagnostic value in evaluating DR in the general population. The expression of exosome lncRNA PRINS is also involved in the prediction and diagnosis of female DR as a unique biomarker.Citation147 In an atrial fibrillation (AF) diagnosis study, the authors analyzed the serum exosome lncRNAs of AF patients and the normal population and discovered that lncRNA LOC107986997 in serum exosomes was closely correlated with AF expression, potentially making it a sensitive and specific diagnostic biomarker for AF.Citation148 Furthermore, lncRNA-Linc00662, lncRNA-CHASERR, and lncRNA-PCA3 were significantly elevated in the extracellular vesicles isolated from the urine of prostate cancer (PC) patients.Citation149 Plasma and urinary EVs as a potential sources of RNA biomarkers for prostate cancer in liquid biopsies.
tRNA
Exosomes tRNA is a regulatory factor involved in cell proliferation and differentiation that is specifically expressed in various diseases, including breast cancer, liver cancer, and the thymus, making tRNA a potentially effective biomarker. RNA sequencing technology has been used to analyze differences in the levels of tRNA-derived small RNAs (tRFs) in exosomes. The levels of four tRNAs (tRNA-ValTAC-3, tRNA-GlyTCC-5, tRNA-ValAAC-5, and tRNA-GluCTC-5) were significantly increased in the plasma exosomes of patients with liver cancer.Citation150 However, the levels of tRF-Leu-TAA-005, tRF-Asn-GTT-010, tRF-Ala-AGC-036, tRF-Lys-CTT-049 and tRF-Trp-CCA-057 were significantly downregulated in patients with non-small cell lung cancer (NSCLC).Citation151 Moreover, the levels of tRF0-Ile-AAT-01 and tiRNA0-Lys-CTT-01 contained in urinary exosomes of lupus nephritis (LN) patients were upregulated, which could be used to distinguish systemic lupus erythematosus (SLE) patients with or without LN. The levels of tRF3-Ile-AAT-1 and tiRNA5-Lys-CTT-1 derived from urinary exosomes were higher in SLE patients with mild and moderate-to-severe activity.Citation152 These studies highlight the potential of tRFs in plasma exosomes as a novel molecular marker for tumor and LN diagnosis.Citation150,Citation152
Exosomes, which are associated with cancer invasion and malignancy, are commonly used in liquid biopsies as highly potential markers for cancer diagnosis. However, exosome analysis requires complicated pretreatment and is challenging to perform quickly. If a method with high sensitivity and simple detection of exosomes in body fluids can be developed, it could be an effective disease detection method. A newly developed nanomembrane system, iTEARS, can rapidly obtain high yield and purity exosomes from tears, allowing clinicians to diagnose diseases and disorders more quickly and effectively.Citation153 The new system, iTEARS, separates exosomes in just five minutes by using an oscillating pressure flow to filter a small amounts of tear across a nanoporous membrane to reduce clogging. Proteins from exosomes are labeled with fluorescent probes, which are then transferred to other instruments for further analysis. Based on the proteomic analysis of extracted proteins, researchers were able to distinguish between healthy people and patients with dry eye disease. Similarly, they analyzed miRNAs in tear exosomes in patients with and without DR. The analysis results showed that miR-145-5p, miR-214-3p, miR-218-5p, and miR-9-5p were disordered in the development of DR, which verified that iTEARS can also help track the progress of the disease. Similarly, other researchers have developed a multifunctional system, MORPH (mechanical metamaterial operating at a critical point for hyper-responsive analysis), that leverages the advanced behavior of mechanical metamaterials for nanoscale molecular analysis of EVs in the ascites of cancer patients. This system uses a mechanical metamaterial based on a double-response hydrogel as a shape-shifting chiral interferometer. The researchers used amplitude and kinetic analyses of MORPH to perform molecular analyses of entire exosomes, characterizing the biomarker composition of these nanoscale vesicles. This system enables sensitive quantification and differentiation of vesicle mixtures with different biomarker distributions.Citation154
In conclusion, exosomes and their components are promising biological markers for the diagnosis of a variety of diseases, opening up a new avenue for early screening, diagnosis, and treatment of diseases, particularly in cases where tissue samples are not available (). However, the road to biomarker validation has not been smooth, and there is a need for improved liquid biopsy tools for cancer detection and monitoring. In the process of conducting research on exosomes, a series of steps such as extraction, purification, identification, staining, and sequencing are generally required. The amount of exosomes extracted and the purity level are critical to the success of the experiment. More evidence is required to support the transition from the research phase to clinical application. Nonetheless, the potential of exosomes as biomarkers should not be ignored.
Table 2 Examples of Exosomal Contents for Clinical Diagnostic Applications
Engineered EVs in Targeted Therapy and Drug Delivery
Exosomes have garnered widespread attention due to their exceptional biocompatibility, safety, stability, and ability to cross the blood-brain barrier, particularly as drug carriers.Citation155–157 Moreover, exosomes can be modified to reach the target cell effectively, encapsulating the therapeutic components within the exosome and facilitating their uptake by the cell to play a therapeutic regulatory role. However, it is essential to ensure that the methods used to modify exosomes do not significantly alter their structure and function.
As described above, EVs are cell-derived lipid membrane structures that transport various active biomolecules between cells, thereby altering the physiology of recipient cells.Citation158 The uptake of EVs by receptor cells is partially selective, and the specific proteins, lipids, glycans, and overall negative charge can affect their targeting to specific organs and ensure minimal non-specific interactions. This becomes the basis for targeted drug delivery.Citation23,Citation25,Citation159–162 The superior tissue-targeting ability of exosomes has been identified in studies of parent and target cells. For example, during T-cell immunosynapse, microRNA-loaded exosomes are unidirectionally transferred from T cells to antigen presenting cells.Citation163 Another important reason for using EVs as drug delivery vehicles is their low response to the immune system during delivery, as demonstrated by the low toxicity and low immune response observed in vivo trials of EV therapeutics.
Engineered EVs: Drug Delivery
Exosomes can carry various therapeutic drugs, such as small molecule chemicals, proteins, and nucleic acids. By encapsulating small molecule chemical drugs in exosomes, the limitations of low solubility, high toxicity, and poor specificity can be avoided. This approach enables the drugs to easily enter the tumor and have a therapeutic effect. For instance, erastin is added to exosomes derived from human lung fibroblasts-1, which were labeled with folate. These exosomes are transported to folate receptor-rich MDA-MB-231 cells in triple-negative breast cancer for targeted antitumor effects, mitigating the nephrotoxic effects of erastin.Citation164 Macrophage-derived exosomes loaded with the chemotherapeutic drug doxorubicin have also been used to treat triple-negative breast cancer. This loading approach can avoid the disadvantages of doxorubicin’s high clearance rate and high toxicity in vivo, providing a new way for hydrophobic chemotherapy drug delivery that targets tumor cells more accurately.Citation165 In the study about ischemic stroke treatment, the researchers prepared an engineered exosome loaded with brain-derived neurotrophic factor (BDNF) from human neural stem cells (HNSCs). The results showed that BDNF-hNSC-Exo engineered exosomes led to a reduction in infarct volume and improvement in neurological function.Citation166 In addition, a study found that foreign exosomes can effectively deliver the NF-kB inhibitor (SR-IκBα) to the fetus in a mouse model, slowing fetal immune cell migration and delaying preterm birth. This method of loading drugs into exosomes can achieve sustained efficacy and prolong pregnancy, significantly improving fetal viability.Citation167
Engineered EVs: Internal Modification
For naturally isolated exosomes, EVs can be further engineered to enhance their stability and biological activity, as well as their binding ability to target cells. At present, there are two methods of modifying exosomes: internal and surface modification. In internal modification studies, many researchers have explored the active binding mechanism of exosomes after separation and purification. Active binding involves temporarily disrupting the cell membrane to allow drug entry, followed by recovery of the exosome membrane after drug diffusion.Citation168–172 Transfection is the most widely used method for introducing RNA into exosomes. This is achieved by incubating exosomes with drugs and surfactants that form membrane pores, enabling transfection of RNA. Transfection has a good loading efficiency and molecular stability. For example, transfection techniques have been used to introduce head and neck squamous cell carcinoma-related regulatory miRNAs into EVs, leading to observed inhibition or promotion of tumor growth after targeted binding of the engineered exosomes to tumor cells.Citation173 However, transfection changes the genetic material contained in EVs, which may impair the ability of EVs to deliver stably expressed siRNA and even raise potential biosafety problems.Citation174,Citation175 Electroporation, on the other hand, uses electric fields to create temporary hydrophilic pores in the phospholipid membrane of exosomes and load hydrophilic biomaterials. This technology is widely applicable and has been used to successfully deliver Adriamycin (for increased targeting) and doxorubicin (an antitumor agent) into MSC-EXO to TUBO breast cancer cell lines, reducing tumor growth rates, while free Adriamycin and non-targeted doxorubicin exosomes had no effect.Citation176 In the study of liver cancer cells, the migration and proliferation of cancer cells were significantly inhibited after the miR-26a-loaded exosomes enter cancer cells through the endocytic pathway.Citation177 However, electroporation may lead to morphological changes and aggregation in exosomes, which is not conducive to the storage and stability of EVs in vivo.Citation178–180
Engineered EVs: Parent Cell Surface Modification
The biological distribution of exosomes, their ability to target specific cells, and their therapeutic potential are critical considerations. Therefore, modifying the surface of exosome can achieve the desired properties and improve their ability to target cells. This can be accomplished through the genetic engineering of the parental cells. Parent cells are genetically modified by inserting the required ligands, which then secrete exosomes with the inserted properties on their surfaces. In the field of immunotherapy, cytokine release syndrome induced by chimeric antigen receptor (CAR) T cell therapy is is a significant concern.Citation181,Citation182 In preclinical models of cytokine release syndrome, the non-expression of the PD-1 protein in CAR-containing exosomes prevents the influence of PD-L1 on the tumor cell membrane surface. These exosomes from CAR T cells were found to express high levels of cytotoxic molecules without causing cytokine release syndrome, suggesting that they may be useful in future therapies targeting tumors.Citation181
Engineered EVs: EVs Surface Modification
Similarly, in order to achieve more specific delivery to target cells, isolated exosomes can be also directly modified to achieve more selective targeting of target tissues or cells by mixing specific ligands on their surfaces.Citation183 For instance, EVs secreted by adipose-derived mesenchymal stem cells can rebind with fluorinated peptide dendritic polymer (FPG3) to form exo@FPG3. Results showed that surface modification of the EVS with FPG3 improved the cellular uptake and biological activity of the extracellular vesicles. Exo@FPG3 significantly enhanced the in vitro angiogenesis and migration of human umbilical vein endothelial cells (HUVECs).Citation184 In other studies, a HER2-targeting peptide was fused to the N-terminus of Lamp2 on the exosome surface, along with the green fluorescent protein (GFP). This engineered exosome delivers 5-FU and miR-21i to HCT-116 with greater accuracy, enabling treatment of colorectal cancer while also tracking drug uptake and metabolism.Citation185–187 Synthetic multivalent antibodies retargeted exosomes/SMART-Exos have also been designed. By adding antibodies to the T cell membrane surface protein CD3 and EGFR antibodies to the breast cancer cell membrane proteins to exosomes, SMART-Exos were able to specifically enhance the immune response of T cells to breast cancer cells and inhibit tumor growth.Citation188 External regulation can also enhance the targeting effect. A study showed that after intravenous injection of IOPN-containing MSC-EXO, an external magnetic field controlled the biological distribution of iron oxide nanoparticles (IOPN) in the body, allowing the nanoparticles to navigate to the target area and increasing the concentration at the location site by 5.1 times.Citation189 A Phase 1 trial of Exo-ASO-STAT6 is underway, bringing us closer to practical clinical trials. Exo-ASO-STAT6 is a novel engineered exosome drug candidate loaded with antisense oligonucleotides (ASO) for the STAT6 (signal transducer and activator of transcription 6) transcription factor. It can selectively target tumor-associated macrophages and precisely interfere with STAT6 signaling, reprogramming TAMs into a pro-inflammatory M1 phenotype for anti-tumor immunity.Citation190 To increase the accumulation of drugs in the lesion site of the target disease and avoid rapid elimination of EVs, EVs were coated with polyethylene glycol, expressed with CD47, or combined with albumin binding domains (ABDs). These engineering modifications prolonged the presence of EVs in vivo and improved drug distribution.Citation191–197
In general, engineered exosomes are designed to retain their inherent characteristics, such as strong stability, minimal immune response, robust targeting ability, and excellent barrier penetration ability. By loading additional designed molecules, engineered exosomes can become more ideal vectors for genetic material and drug delivery (). However, as research on engineered exosomes continues to evolve, some scholars have expressed concerns. Compared to the manufacturing processes for protein and synthetic drug delivery systems, small changes in the production of EVs can have significant effects on product quality and activity. Due to their small size and complex composition and structure, detecting and evaluating changes in EV product quality is more challenging. Furthermore, processes for EV manufacturing are considered less stable than those for cell and antibody production.Citation198
Table 3 Engineered Exosome-Based Drug Delivery Systems
To improve the controllability of exosome production, comprehensive characterization, product analysis, and quality control of EVs are needed.Citation27,Citation199 Comprehensive characterization of EVs includes characterization of exosome proteomes, which can be used to identify amplified signaling pathways indicating cancer, as well as observation of tumor stromal responses and responses to cancer therapies.Citation200 To characterize EVs, NP-TRFIA techniques use transmembrane proteins and biotinylated antibodies to capture EVs directly from urine and cellular supernatants for detection and characterization. This technique has several advantages, including a simple separation procedure, high signal-to-noise ratio, identification and evaluation of tumor-related proteins on the surface of EVs, and the potential for comprehensive and rapid characterization of exosomes.Citation201 Evaluating the efficacy and safety of EVs requires determining their characteristics and purity, and measures must be taken to ensure that the final product meets previously identified key quality attributes. This poses significant analytical challenges, such as measurements of size and concentration, exclusion of contaminants, identification of functional markers, and evaluation of drug loads to assess therapeutic activity of each vesicle. Among the separation analysis methods, the size exclusion chromatography (SEC) technique may be the best method to isolate exosomes from proteins. SEC has the characteristics of high purity and high efficiency, and can improve plasma protein contamination to achieve the formation and function of complete exosomes from plasma.Citation202,Citation203 It can also be used in combination with other techniques to increase exosome purity.Citation204 Dual mode chromatography (DMC) has been successfully used to reduce contamination of lipoprotein particles (LPP) in plasma exotics.Citation205 However, SEC techniques cannot distinguish between exosomes and MVs of the same size, and cannot avoid biological target degeneration.Citation206 Therefore, SEC combined with ultrafiltration may be the best separation method.Citation207–209 Although SEC is currently an excellent candidate for exosome isolation, more direct comparative studies with other separation methods are needed as evidence.Citation209 In addition to improving exosome separation technique, a new exosome production technique called the exosome analog technique has been developed.Citation210,Citation211 This exosome-mimetics (EMs) technology mainly produces vesicles that retain the main characteristics of exosomes and eliminates the chemical process required to attach the targeted ligand to the surface of vesicles. It has the characteristics of low production time and high yield, and it is also a potential research direction to promote exosomes for practical clinical applications in the future.Citation212–214
Despite these potentially worrisome factors, most animal studies conducted so far have shown positive results for EVs, making them a promising component for future cell-free therapies. However, before these therapies can be widely used, individual EV agents must be assessed for factors such as immunogenicity, biocompatibility, liposomal carrier, and biological process. To improve the experimental design, the research should be based on more complex in vitro model, such as three-dimensional cell tissue model. Researchers should also more adequately evaluate exosome organ distribution and content expression in animal models, and conduct studies on repeated administration under various regimens for further clinical examination.Citation215,Citation216 A clinically oriented approach to using EVs involves transferring patient cells into culture and isolating vesicles for re-administration to the patient. However, there are still issues that need to be addressed, especially for acute diseases, such as infections or cardiovascular events.Citation217 The use of autologous EVs is feasible in cases where genetic compatibility needs to be maintained or where autogenous EVs are readily available.Citation218,Citation219 Nonetheless, most current applications prefer non-autologous EVs, mainly due to their well-established safety profiles and rigorous validation. For example, current MSC-EVs for regenerative medicine and EVs from dendritic cells for vaccine delivery are undergoing validation, and these vesicles have shown favorable safety profiles in several Phase I clinical trials.Citation220,Citation221
Conclusions and Prospects
Because of its diverse and pleiotropic effects on physiology and pathology, EVs have been increasingly studied as intermediates of intercellular communication. To date, EVs have been successfully used in various preclinical studies for disease treatment, engineering, and disease diagnosis (). However, it is critical to review and summarize the these results and ongoing challenges.Citation222,Citation223 Although current studies showing impressive results, the complex process of EV sample analysis still needs to be standardized for robust and repeatable results across laboratories. Further work is needed to be done before these studies have practical clinical applications, including controlling costs of EVs, improving isolation methods, comprehensively characterizing EVs, and exploring the underlying pathophysiological mechanisms to avoid any uncontrolled harm to patients. Despite the promising results in clinical trials of EVs, which show good efficacy and safety, caution is still required due to the complexity of EVs. The prospects for the clinical application of exosomes are broad, and we believe that the current research and applications are just the tip of the iceberg. With continued research, the challenges described in this paper will be addressed, eventually leading to the practical application of EVs in the treatment and diagnosis of diseases, bringing tangible benefits to patients.
Author Contributions
All authors made a significant contribution to the work reported, whether that is in the conception, study design, execution, acquisition of data, analysis and interpretation, or in all these areas; All authors took part in drafting, revising or critically reviewing the article; All authors gave final approval of the version to be published; All authors have agreed on the journal to which the article has been submitted; and all authors agree to be accountable for all aspects of the work.
Disclosure
The authors report no conflicts of interest in this work.
Acknowledgments
This study was supported by following: National Natural Science Foundation of China (81871782, 31900968), Applied Basic Research Multi-input Foundation of Tianjin (21JCYBJC01760), Applied Basic Research Foundation of Tianjin (22JCQNJC00360, 22JCQNJC00230).
References
- Buzas EI. The roles of extracellular vesicles in the immune system. Nat Rev Immunol. 2022;22:1–15. doi:10.1038/s41577-022-00763-8
- Cocucci E, Racchetti G, Meldolesi J. Shedding microvesicles: artefacts no more. Trends Cell Biol. 2009;19(2):43–51. doi:10.1016/j.tcb.2008.11.003
- Johnstone RM, Adam M, Hammond JR, Orr L, Turbide C. Vesicle formation during reticulocyte maturation. Association of plasma membrane activities with released vesicles (exosomes). J Biol Chem. 1987;262(19):9412–9420. doi:10.1016/S0021-9258(18)48095-7
- Johnstone RM. Revisiting the road to the discovery of exosomes. Blood Cells Mol Dis. 2005;34(3):214–219. doi:10.1016/j.bcmd.2005.03.002
- Kay HM, Birss AJ, Smalley JW. Glycylprolyl dipeptidase activity of Bacteroides gingivalis W50 and the avirulent variant W50/BEI. FEMS Microbiol Lett. 1989;48(1):93–96. doi:10.1016/0378-1097(89)90153-5
- Obermayer G, Afonyushkin T, Göderle L, et al. Natural IgM antibodies inhibit microvesicle-driven coagulation and thrombosis. Blood. 2021;137(10):1406–1415. doi:10.1182/blood.2020007155
- Lindenbergh MFS, Stoorvogel W. Antigen presentation by extracellular vesicles from professional antigen-presenting cells. Annu Rev Immunol. 2018;36:435–459. doi:10.1146/annurev-immunol-041015-055700
- Couch Y, Buzàs EI, Di Vizio D, et al. A brief history of nearly EV-erything - the rise and rise of extracellular vesicles. J Extracell Vesicles. 2021;10(14):e12144. doi:10.1002/jev2.12144
- Théry C, Witwer KW, Aikawa E, et al. Minimal information for studies of extracellular vesicles 2018 (MISEV2018): a position statement of the International Society for Extracellular Vesicles and update of the MISEV2014 guidelines. J Extracell Vesicles. 2018;7(1):1535750. doi:10.1080/20013078.2018.1535750
- O’Brien K, Breyne K, Ughetto S, Laurent LC, Breakefield XO. RNA delivery by extracellular vesicles in mammalian cells and its applications. Nat Rev Mol Cell Biol. 2020;21(10):585–606. doi:10.1038/s41580-020-0251-y
- Kalluri R, LeBleu VS. The biology, function, and biomedical applications of exosomes. Science. 2020;367(6478). doi:10.1126/science.aau6977
- Liao Z, Liu H, Ma L, et al. Engineering extracellular vesicles restore the impaired cellular uptake and attenuate intervertebral disc degeneration. ACS Nano. 2021;15(9):14709–14724. doi:10.1021/acsnano.1c04514
- Yuana Y, Sturk A, Nieuwland R. Extracellular vesicles in physiological and pathological conditions. Blood Rev. 2013;27(1):31–39. doi:10.1016/j.blre.2012.12.002
- Lai RC, Tan SS, Yeo RW, et al. MSC secretes at least 3 EV types each with a unique permutation of membrane lipid, protein and RNA. J Extracell Vesicles. 2016;5:29828. doi:10.3402/jev.v5.29828
- Valcz G, Buzás EI, Kittel Á, et al. En bloc release of MVB-like small extracellular vesicle clusters by colorectal carcinoma cells. J Extracell Vesicles. 2019;8(1):1596668. doi:10.1080/20013078.2019.1596668
- van Niel G, Carter DRF, Clayton A, Lambert DW, Raposo G, Vader P. Challenges and directions in studying cell-cell communication by extracellular vesicles. Nat Rev Mol Cell Biol. 2022;23(5):369–382. doi:10.1038/s41580-022-00460-3
- Oliveira GP, Zigon E, Rogers G, et al. Detection of extracellular vesicle RNA using molecular beacons. iScience. 2020;23(1):100782. doi:10.1016/j.isci.2019.100782
- Skotland T, Hessvik NP, Sandvig K, Llorente A. Exosomal lipid composition and the role of ether lipids and phosphoinositides in exosome biology. J Lipid Res. 2019;60(1):9–18. doi:10.1194/jlr.R084343
- Laulagnier K, Motta C, Hamdi S, et al. Mast cell- and dendritic cell-derived exosomes display a specific lipid composition and an unusual membrane organization. Biochem J. 2004;380(Pt 1):161–171. doi:10.1042/bj20031594
- Veerman RE, Teeuwen L, Czarnewski P, et al. Molecular evaluation of five different isolation methods for extracellular vesicles reveals different clinical applicability and subcellular origin. J Extracell Vesicles. 2021;10(9):e12128. doi:10.1002/jev2.12128
- Wen SW, Lima LG, Lobb RJ, et al. Breast cancer-derived exosomes reflect the cell-of-origin phenotype. Proteomics. 2019;19(8):e1800180. doi:10.1002/pmic.201800180
- Ferguson SW, Nguyen J. Exosomes as therapeutics: the implications of molecular composition and exosomal heterogeneity. J Control Release. 2016;228:179–190. doi:10.1016/j.jconrel.2016.02.037
- van Niel G, D’Angelo G, Raposo G. Shedding light on the cell biology of extracellular vesicles. Nat Rev Mol Cell Biol. 2018;19(4):213–228. doi:10.1038/nrm.2017.125
- Ostrowski M, Carmo NB, Krumeich S, et al. Rab27a and Rab27b control different steps of the exosome secretion pathway. Nat Cell Biol. 2010;12(1):19–30; sup pp 1–13. doi:10.1038/ncb2000
- Mathieu M, Martin-Jaular L, Lavieu G, Théry C. Specificities of secretion and uptake of exosomes and other extracellular vesicles for cell-to-cell communication. Nat Cell Biol. 2019;21(1):9–17. doi:10.1038/s41556-018-0250-9
- Larios J, Mercier V, Roux A, Gruenberg J. ALIX- and ESCRT-III-dependent sorting of tetraspanins to exosomes. J Cell Biol. 2020;219(3):154.
- Wei D, Zhan W, Gao Y, et al. RAB31 marks and controls an ESCRT-independent exosome pathway. Cell Res. 2021;31(2):157–177. doi:10.1038/s41422-020-00409-1
- Yang L, Peng X, Li Y, et al. Long non-coding RNA HOTAIR promotes exosome secretion by regulating RAB35 and SNAP23 in hepatocellular carcinoma. Mol Cancer. 2019;18(1):78. doi:10.1186/s12943-019-0990-6
- Yu Z, Shi M, Stewart T, et al. Reduced oligodendrocyte exosome secretion in multiple system atrophy involves FasL dysfunction. Brain. 2020;143(6):1780–1797. doi:10.1093/brain/awaa110
- Agliardi C, Meloni M, Guerini FR, et al. Oligomeric α-Syn and SNARE complex proteins in peripheral extracellular vesicles of neural origin are biomarkers for Parkinson’s disease. Neurobiol Dis. 2021;148:105185. doi:10.1016/j.nbd.2020.105185
- Clancy JW, Zhang Y, Sheehan C, D’Souza-Schorey C. An ARF6-Exportin-5 axis delivers pre-miRNA cargo to tumour microvesicles. Nat Cell Biol. 2019;21(7):856–866. doi:10.1038/s41556-019-0345-y
- Wang T, Gilkes DM, Takano N, et al. Hypoxia-inducible factors and RAB22A mediate formation of microvesicles that stimulate breast cancer invasion and metastasis. Proc Natl Acad Sci U S A. 2014;111(31):E3234–42. doi:10.1073/pnas.1410041111
- Ashrafizaveh S, Ashrafizadeh M, Zarrabi A, et al. Long non-coding RNAs in the doxorubicin resistance of cancer cells. Cancer Lett. 2021;508:104–114. doi:10.1016/j.canlet.2021.03.018
- Yáñez-Mó M, Siljander PR, Andreu Z, et al. Biological properties of extracellular vesicles and their physiological functions. J Extracell Vesicles. 2015;4:27066. doi:10.3402/jev.v4.27066
- Mulcahy LA, Pink RC, Carter DR. Routes and mechanisms of extracellular vesicle uptake. J Extracell Vesicles. 2014;3:24641. doi:10.3402/jev.v3.24641
- Costa Verdera H, Gitz-Francois JJ, Schiffelers RM, Vader P. Cellular uptake of extracellular vesicles is mediated by clathrin-independent endocytosis and macropinocytosis. J Control Release. 2017;266:100–108. doi:10.1016/j.jconrel.2017.09.019
- Pitt JM, Kroemer G, Zitvogel L. Extracellular vesicles: masters of intercellular communication and potential clinical interventions. J Clin Invest. 2016;126(4):1139–1143. doi:10.1172/jci87316
- Herrmann IK, Wood MJA, Fuhrmann G. Extracellular vesicles as a next-generation drug delivery platform. Nat Nanotechnol. 2021;16(7):748–759. doi:10.1038/s41565-021-00931-2
- Najafi F, Kelaye SK, Kazemi B, et al. The role of miRNA-424 and miR-631 in various cancers: focusing on drug resistance and sensitivity. Pathol Res Pract. 2022;239:154130. doi:10.1016/j.prp.2022.154130
- Hu Y, Rao SS, Wang ZX, et al. Exosomes from human umbilical cord blood accelerate cutaneous wound healing through miR-21-3p-mediated promotion of angiogenesis and fibroblast function. Theranostics. 2018;8(1):169–184. doi:10.7150/thno.21234
- Yan C, Chen J, Wang C, et al. Milk exosomes-mediated miR-31-5p delivery accelerates diabetic wound healing through promoting angiogenesis. Drug Deliv. 2022;29(1):214–228. doi:10.1080/10717544.2021.2023699
- Sung BH, von Lersner A, Guerrero J, et al. A live cell reporter of exosome secretion and uptake reveals pathfinding behavior of migrating cells. Nat Commun. 2020;11(1):2092. doi:10.1038/s41467-020-15747-2
- Zhou X, Xie F, Wang L, et al. The function and clinical application of extracellular vesicles in innate immune regulation. Cell Mol Immunol. 2020;17(4):323–334. doi:10.1038/s41423-020-0391-1
- Stenqvist AC, Nagaeva O, Baranov V, Mincheva-Nilsson L. Exosomes secreted by human placenta carry functional Fas ligand and TRAIL molecules and convey apoptosis in activated immune cells, suggesting exosome-mediated immune privilege of the fetus. J Immunol. 2013;191(11):5515–5523. doi:10.4049/jimmunol.1301885
- Dumont TMF, Mouillet JF, Bayer A, et al. The expression level of C19MC miRNAs in early pregnancy and in response to viral infection. Placenta. 2017;53:23–29. doi:10.1016/j.placenta.2017.03.011
- Tkach M, Kowal J, Zucchetti AE, et al. Qualitative differences in T-cell activation by dendritic cell-derived extracellular vesicle subtypes. EMBO j. 2017;36(20):3012–3028. doi:10.15252/embj.201696003
- Lu Z, Zuo B, Jing R, et al. Dendritic cell-derived exosomes elicit tumor regression in autochthonous hepatocellular carcinoma mouse models. J Hepatol. 2017;67(4):739–748. doi:10.1016/j.jhep.2017.05.019
- Sato Y, Yaguchi M, Okuno Y, et al. Epstein-Barr virus tegument protein BGLF2 in exosomes released from virus-producing cells facilitates de novo infection. Cell Commun Signal. 2022;20(1):95. doi:10.1186/s12964-022-00902-7
- Zeng Z, Li Y, Pan Y, et al. Cancer-derived exosomal miR-25-3p promotes pre-metastatic niche formation by inducing vascular permeability and angiogenesis. Nat Commun. 2018;9(1):5395. doi:10.1038/s41467-018-07810-w
- Mas-Bargues C, Borrás C, Alique M. The contribution of extracellular vesicles from senescent endothelial and vascular smooth muscle cells to vascular calcification. Front Cardiovasc Med. 2022;9:854726. doi:10.3389/fcvm.2022.854726
- Liu X, Wang C, Meng H, Liao S, Zhang J, Guan Y, Tian H and Peng J. (2022). Research Progress on Exosomes in Osteonecrosis of the Femoral Head. Orthopaedic Surgery, 14(9), 1951–1957. 10.1111/os.13393
- Buendía P, Montes de Oca A, Madueño JA, et al. Endothelial microparticles mediate inflammation-induced vascular calcification. FASEB j. 2015;29(1):173–181. doi:10.1096/fj.14-249706
- Deb A, Gupta S, Mazumder PB. Exosomes: a new horizon in modern medicine. Life Sci. 2021;264:118623. doi:10.1016/j.lfs.2020.118623
- Latifkar A, Hur YH, Sanchez JC, Cerione RA, Antonyak MA. New insights into extracellular vesicle biogenesis and function. J Cell Sci. 2019;132(13). doi:10.1242/jcs.222406
- Knox MC, Ni J, Bece A, et al. A clinician’s guide to cancer-derived exosomes: immune interactions and therapeutic implications. Front Immunol. 2020;11:1612. doi:10.3389/fimmu.2020.01612
- Kok VC, Yu CC. Cancer-derived exosomes: their role in cancer biology and biomarker development. Int J Nanomedicine. 2020;15:8019–8036. doi:10.2147/ijn.S272378
- Song YH, Warncke C, Choi SJ, et al. Breast cancer-derived extracellular vesicles stimulate myofibroblast differentiation and pro-angiogenic behavior of adipose stem cells. Matrix Biol. 2017;60-61:190–205. doi:10.1016/j.matbio.2016.11.008
- Feng Q, Zhang C, Lum D, et al. A class of extracellular vesicles from breast cancer cells activates VEGF receptors and tumour angiogenesis. Nat Commun. 2017;8:14450. doi:10.1038/ncomms14450
- Chen S, Crabill GA, Pritchard TS, et al. Mechanisms regulating PD-L1 expression on tumor and immune cells. J Immunother Cancer. 2019;7(1):305. doi:10.1186/s40425-019-0770-2
- Yin Z, Yu M, Ma T, et al. Mechanisms underlying low-clinical responses to PD-1/PD-L1 blocking antibodies in immunotherapy of cancer: a key role of exosomal PD-L1. J Immunother Cancer. 2021;9(1):e001698. doi:10.1136/jitc-2020-001698
- Dou D, Ren X, Han M, et al. Cancer-associated fibroblasts-derived exosomes suppress immune cell function in breast cancer via the miR-92/PD-L1 pathway. Front Immunol. 2020;11:2026. doi:10.3389/fimmu.2020.02026
- Pritchard A, Tousif S, Wang Y, et al. Lung tumor cell-derived exosomes promote M2 macrophage polarization. Cells. 2020;9(5):1303. doi:10.3390/cells9051303
- Yeh YY, Ozer HG, Lehman AM, et al. Characterization of CLL exosomes reveals a distinct microRNA signature and enhanced secretion by activation of BCR signaling. Blood. 2015;125(21):3297–3305. doi:10.1182/blood-2014-12-618470
- Qazi KR, Torregrosa Paredes P, Dahlberg B, Grunewald J, Eklund A, Gabrielsson S. Proinflammatory exosomes in bronchoalveolar lavage fluid of patients with sarcoidosis. Thorax. 2010;65(11):1016–1024. doi:10.1136/thx.2009.132027
- Bai X, Li J, Li L, et al. Extracellular vesicles from adipose tissue-derived stem cells affect notch-miR148a-3p axis to regulate polarization of macrophages and alleviate sepsis in mice. Front Immunol. 2020;11:1391. doi:10.3389/fimmu.2020.01391
- Crenshaw BJ, Gu L, Sims B, Matthews QL. Exosome biogenesis and biological function in response to viral infections. Open Virol J. 2018;12:134–148. doi:10.2174/1874357901812010134
- Ebrahimkhani S, Beadnall HN, Wang C, et al. Serum exosome microRNAs predict multiple sclerosis disease activity after fingolimod treatment. Mol Neurobiol. 2020;57(2):1245–1258. doi:10.1007/s12035-019-01792-6
- Tian Y, Wang T, Bu H, Shao G, Zhang W and Zhang L. (2022). Role of Exosomal miR ‐223 in Chronic Skeletal Muscle Inflammation. Orthopaedic Surgery, 14(4), 644–651. 10.1111/os.13232
- Rani S, Ryan AE, Griffin MD, Ritter T. Mesenchymal stem cell-derived extracellular vesicles: toward cell-free therapeutic applications. Mol Ther. 2015;23(5):812–823. doi:10.1038/mt.2015.44
- Crapnell K, Blaesius R, Hastings A, Lennon DP, Caplan AI, Bruder SP. Growth, differentiation capacity, and function of mesenchymal stem cells expanded in serum-free medium developed via combinatorial screening. Exp Cell Res. 2013;319(10):1409–1418. doi:10.1016/j.yexcr.2013.04.004
- Volarevic V, Markovic BS, Gazdic M, et al. Ethical and safety issues of stem cell-based therapy. Int J Med Sci. 2018;15(1):36–45. doi:10.7150/ijms.21666
- Murry CE, Keller G. Differentiation of embryonic stem cells to clinically relevant populations: lessons from embryonic development. Cell. 2008;132(4):661–680. doi:10.1016/j.cell.2008.02.008
- De Trizio E, Brennan CS. The business of human embryonic stem cell research and an international analysis of relevant laws. J Biolaw Bus. 2004;7(4):14–22.
- Breitbach M, Bostani T, Roell W, et al. Potential risks of bone marrow cell transplantation into infarcted hearts. Blood. 2007;110(4):1362–1369. doi:10.1182/blood-2006-12-063412
- Yoshihara M, Hayashizaki Y, Murakawa Y. Genomic instability of iPSCs: challenges towards their clinical applications. Stem Cell Rev Rep. 2017;13(1):7–16. doi:10.1007/s12015-016-9680-6
- Tsiapalis D, O’Driscoll L. Mesenchymal stem cell derived extracellular vesicles for tissue engineering and regenerative medicine applications. Cells. 2020;9(4):991. doi:10.3390/cells9040991
- van Balkom BWM, Gremmels H, Giebel B, Lim SK. Proteomic signature of mesenchymal stromal cell-derived small extracellular vesicles. Proteomics. 2019;19(1–2):e1800163. doi:10.1002/pmic.201800163
- Zhao M, Liu S, Wang C, et al. Mesenchymal stem cell-derived extracellular vesicles attenuate mitochondrial damage and inflammation by stabilizing mitochondrial DNA. ACS Nano. 2021;15(1):1519–1538. doi:10.1021/acsnano.0c08947
- Harrell CR, Jovicic N, Djonov V, Arsenijevic N, Volarevic V. Mesenchymal stem cell-derived exosomes and other extracellular vesicles as new remedies in the therapy of inflammatory diseases. Cells. 2019;8(12):1605. doi:10.3390/cells8121605
- Xia Y, Hu G, Chen Y, et al. Embryonic stem cell derived small extracellular vesicles modulate regulatory T cells to protect against ischemic stroke. ACS Nano. 2021;15(4):7370–7385. doi:10.1021/acsnano.1c00672
- Mathew B, Ravindran S, Liu X, et al. Mesenchymal stem cell-derived extracellular vesicles and retinal ischemia-reperfusion. Biomaterials. 2019;197:146–160. doi:10.1016/j.biomaterials.2019.01.016
- Xia C, Zeng Z, Fang B, et al. Mesenchymal stem cell-derived exosomes ameliorate intervertebral disc degeneration via anti-oxidant and anti-inflammatory effects. Free Radic Biol Med. 2019;143:1–15. doi:10.1016/j.freeradbiomed.2019.07.026
- He L, He T, Xing J, et al. Bone marrow mesenchymal stem cell-derived exosomes protect cartilage damage and relieve knee osteoarthritis pain in a rat model of osteoarthritis. Stem Cell Res Ther. 2020;11(1):276. doi:10.1186/s13287-020-01781-w
- Wang R, Xu B. TGF-β1-modified MSC-derived exosomal miR-135b attenuates cartilage injury via promoting M2 synovial macrophage polarization by targeting MAPK6. Cell Tissue Res. 2021;384(1):113–127. doi:10.1007/s00441-020-03319-1
- Yan B, Zhang Y, Liang C, et al. Stem cell-derived exosomes prevent pyroptosis and repair ischemic muscle injury through a novel exosome/circHIPK3/ FOXO3a pathway. Theranostics. 2020;10(15):6728–6742. doi:10.7150/thno.42259
- Ibrahim AG, Cheng K, Marbán E. Exosomes as critical agents of cardiac regeneration triggered by cell therapy. Stem Cell Rep. 2014;2(5):606–619. doi:10.1016/j.stemcr.2014.04.006
- Lan B, Zeng S, Grützmann R, Pilarsky C. The role of exosomes in pancreatic cancer. Int J Mol Sci. 2019;20(18):4332. doi:10.3390/ijms20184332
- Li W, Mu D, Tian F, et al. Exosomes derived from Rab27a‑overexpressing tumor cells elicit efficient induction of antitumor immunity. Mol Med Rep. 2013;8(6):1876–1882. doi:10.3892/mmr.2013.1738
- Jorfi S, Ansa-Addo EA, Kholia S, et al. Inhibition of microvesiculation sensitizes prostate cancer cells to chemotherapy and reduces docetaxel dose required to limit tumor growth in vivo. Sci Rep. 2015;5:13006. doi:10.1038/srep13006
- Siklos M, BenAissa M, Thatcher GR. Cysteine proteases as therapeutic targets: does selectivity matter? A systematic review of calpain and cathepsin inhibitors. Acta Pharm Sin B. 2015;5(6):506–519. doi:10.1016/j.apsb.2015.08.001
- Datta A, Kim H, Lal M, et al. Manumycin A suppresses exosome biogenesis and secretion via targeted inhibition of Ras/Raf/ERK1/2 signaling and hnRNP H1 in castration-resistant prostate cancer cells. Cancer Lett. 2017;408:73–81. doi:10.1016/j.canlet.2017.08.020
- Shilo A, Ben Hur V, Denichenko P, et al. Splicing factor hnRNP A2 activates the Ras-MAPK-ERK pathway by controlling A-Raf splicing in hepatocellular carcinoma development. Rna. 2014;20(4):505–515. doi:10.1261/rna.042259.113
- Tamai K, Tanaka N, Nakano T, et al. Exosome secretion of dendritic cells is regulated by Hrs, an ESCRT-0 protein. Biochem Biophys Res Commun. 2010;399(3):384–390. doi:10.1016/j.bbrc.2010.07.083
- Baranger K, van Gijsel-Bonnello M, Stephan D, et al. Long-term pantethine treatment counteracts pathologic gene dysregulation and decreases alzheimer’s disease pathogenesis in a transgenic mouse model. Neurotherapeutics. 2019;16(4):1237–1254. doi:10.1007/s13311-019-00754-z
- Ranganathan S, Jackson RL, Harmony JA. Effect of pantethine on the biosynthesis of cholesterol in human skin fibroblasts. Atherosclerosis. 1982;44(3):261–273. doi:10.1016/0021-9150(82)90002-8
- Penet MF, Abou-Hamdan M, Coltel N, et al. Protection against cerebral malaria by the low-molecular-weight thiol pantethine. Proc Natl Acad Sci U S A. 2008;105(4):1321–1326. doi:10.1073/pnas.0706867105
- Matsumoto A, Takahashi Y, Nishikawa M, et al. Accelerated growth of B16BL6 tumor in mice through efficient uptake of their own exosomes by B16BL6 cells. Cancer Sci. 2017;108(9):1803–1810. doi:10.1111/cas.13310
- Barger JF, Rahman MA, Jackson D, Acunzo M, Nana-Sinkam SP. Extracellular miRNAs as biomarkers in cancer. Food Chem Toxicol. 2016;98(Pt A):66–72. doi:10.1016/j.fct.2016.06.010
- Bastos N, Ruivo CF, da Silva S, Melo SA. Exosomes in cancer: use them or target them? Semin Cell Dev Biol. 2018;78:13–21. doi:10.1016/j.semcdb.2017.08.009
- Luo Z, Hu X, Wu C, et al. Plasma exosomes generated by ischaemic preconditioning are cardioprotective in a rat heart failure model. Br J Anaesth. 2022;130:29–38. doi:10.1016/j.bja.2022.08.040
- Wei FS, Rao MW, Huang YL, Chen SB, Wu YQ, Yang L. miR-182-5p delivered by plasma exosomes promotes sevoflurane-induced neuroinflammation and cognitive dysfunction in aged rats with postoperative cognitive dysfunction by targeting brain-derived neurotrophic factor and activating NF-κB pathway. Neurotox Res. 2022;40:1902–1912. doi:10.1007/s12640-022-00597-1
- Wang L, Yang L, Zhuang T, Shi X. Tumor-derived exosomal miR-29b reduces angiogenesis in pancreatic cancer by silencing ROBO1 and SRGAP2. J Immunol Res. 2022;2022:4769385. doi:10.1155/2022/4769385
- Fitzner D, Schnaars M, van Rossum D, et al. Selective transfer of exosomes from oligodendrocytes to microglia by macropinocytosis. J Cell Sci. 2011;124(Pt 3):447–458. doi:10.1242/jcs.074088
- Feng D, Zhao WL, Ye YY, et al. Cellular internalization of exosomes occurs through phagocytosis. Traffic. 2010;11(5):675–687. doi:10.1111/j.1600-0854.2010.01041.x
- Tian T, Zhu YL, Zhou YY, et al. Exosome uptake through clathrin-mediated endocytosis and macropinocytosis and mediating miR-21 delivery. J Biol Chem. 2014;289(32):22258–22267. doi:10.1074/jbc.M114.588046
- Escrevente C, Keller S, Altevogt P, Costa J. Interaction and uptake of exosomes by ovarian cancer cells. BMC Cancer. 2011;11:108. doi:10.1186/1471-2407-11-108
- Lima LG, Chammas R, Monteiro RQ, Moreira ME, Barcinski MA. Tumor-derived microvesicles modulate the establishment of metastatic melanoma in a phosphatidylserine-dependent manner. Cancer Lett. 2009;283(2):168–175. doi:10.1016/j.canlet.2009.03.041
- Christianson HC, Svensson KJ, van Kuppevelt TH, Li JP, Belting M. Cancer cell exosomes depend on cell-surface heparan sulfate proteoglycans for their internalization and functional activity. Proc Natl Acad Sci U S A. 2013;110(43):17380–17385. doi:10.1073/pnas.1304266110
- Xu R, Rai A, Chen M, Suwakulsiri W, Greening DW, Simpson RJ. Extracellular vesicles in cancer - implications for future improvements in cancer care. Nat Rev Clin Oncol. 2018;15(10):617–638. doi:10.1038/s41571-018-0036-9
- Naito Y, Yoshioka Y, Yamamoto Y, Ochiya T. How cancer cells dictate their microenvironment: present roles of extracellular vesicles. Cell Mol Life Sci. 2017;74(4):697–713. doi:10.1007/s00018-016-2346-3
- Marleau AM, Chen CS, Joyce JA, Tullis RH. Exosome removal as a therapeutic adjuvant in cancer. J Transl Med. 2012;10:134. doi:10.1186/1479-5876-10-134
- Ciravolo V, Huber V, Ghedini GC, et al. Potential role of HER2-overexpressing exosomes in countering trastuzumab-based therapy. J Cell Physiol. 2012;227(2):658–667. doi:10.1002/jcp.22773
- Li S, Yi M, Dong B, Tan X, Luo S, Wu K. The role of exosomes in liquid biopsy for cancer diagnosis and prognosis prediction. Int J Cancer. 2021;148(11):2640–2651. doi:10.1002/ijc.33386
- Sala M, Ros M, Saltel F. A complex and evolutive character: two face aspects of ECM in tumor progression. Front Oncol. 2020;10:1620. doi:10.3389/fonc.2020.01620
- Li W, Liu JB, Hou LK, et al. Liquid biopsy in lung cancer: significance in diagnostics, prediction, and treatment monitoring. Mol Cancer. 2022;21(1):25. doi:10.1186/s12943-022-01505-z
- Zhou B, Xu K, Zheng X, et al. Application of exosomes as liquid biopsy in clinical diagnosis. Signal Transduct Target Ther. 2020;5(1):144. doi:10.1038/s41392-020-00258-9
- Zhang Y, Hu YW, Zheng L, Wang Q. Characteristics and roles of exosomes in cardiovascular disease. DNA Cell Biol. 2017;36(3):202–211. doi:10.1089/dna.2016.3496
- Fitts CA, Ji N, Li Y, Tan C. Exploiting exosomes in cancer liquid biopsies and drug delivery. Adv Healthc Mater. 2019;8(6):e1801268. doi:10.1002/adhm.201801268
- Ohmichi T, Mitsuhashi M, Tatebe H, Kasai T, Ali El-Agnaf OM, Tokuda T. Quantification of brain-derived extracellular vesicles in plasma as a biomarker to diagnose Parkinson’s and related diseases. Parkinsonism Relat Disord. 2019;61:82–87. doi:10.1016/j.parkreldis.2018.11.021
- Kitamura Y, Kojima M, Kurosawa T, et al. Proteomic profiling of exosomal proteins for blood-based biomarkers in parkinson’s disease. Neuroscience. 2018;392:121–128. doi:10.1016/j.neuroscience.2018.09.017
- Pasetto L, Callegaro S, Corbelli A, et al. Decoding distinctive features of plasma extracellular vesicles in amyotrophic lateral sclerosis. Mol Neurodegener. 2021;16(1):52. doi:10.1186/s13024-021-00470-3
- Song Y, Wang M, Tong H, et al. Plasma exosomes from endometrial cancer patients contain LGALS3BP to promote endometrial cancer progression. Oncogene. 2021;40(3):633–646. doi:10.1038/s41388-020-01555-x
- Wang L, Liu J, Xu B, Liu YL, Liu Z. Reduced exosome miR-425 and miR-744 in the plasma represents the progression of fibrosis and heart failure. Kaohsiung J Med Sci. 2018;34(11):626–633. doi:10.1016/j.kjms.2018.05.008
- Wang L, Lv Y, Li G, Xiao J. MicroRNAs in heart and circulation during physical exercise. J Sport Health Sci. 2018;7(4):433–441. doi:10.1016/j.jshs.2018.09.008
- Wu T, Chen Y, Du Y, et al. Circulating exosomal miR-92b-5p is a promising diagnostic biomarker of heart failure with reduced ejection fraction patients hospitalized for acute heart failure. J Thorac Dis. 2018;10(11):6211–6220. doi:10.21037/jtd.2018.10.52
- Xu JY, Chen GH, Yang YJ. Exosomes: a rising star in falling hearts. Front Physiol. 2017;8:494. doi:10.3389/fphys.2017.00494
- Kuwabara Y, Ono K, Horie T, et al. Increased microRNA-1 and microRNA-133a levels in serum of patients with cardiovascular disease indicate myocardial damage. Circ Cardiovasc Genet. 2011;4(4):446–454. doi:10.1161/circgenetics.110.958975
- Maegdefessel L, Azuma J, Tsao PS. MicroRNA-29b regulation of abdominal aortic aneurysm development. Trends Cardiovasc Med. 2014;24(1):1–6. doi:10.1016/j.tcm.2013.05.002
- Bang C, Batkai S, Dangwal S, et al. Cardiac fibroblast-derived microRNA passenger strand-enriched exosomes mediate cardiomyocyte hypertrophy. J Clin Invest. 2014;124(5):2136–2146. doi:10.1172/jci70577
- Fang X, Stroud MJ, Ouyang K, et al. Adipocyte-specific loss of PPARγ attenuates cardiac hypertrophy. JCI Insight. 2016;1(16):e89908. doi:10.1172/jci.insight.89908
- Han ZL, Wang HQ, Zhang TS, He YX, Zhou H. Up-regulation of exosomal miR-106a may play a significant role in abdominal aortic aneurysm by inducing vascular smooth muscle cell apoptosis and targeting TIMP-2, an inhibitor of metallopeptidases that suppresses extracellular matrix degradation. Eur Rev Med Pharmacol Sci. 2020;24(15):8087–8095. doi:10.26355/eurrev_202008_22493
- Maegdefessel L, Spin JM, Raaz U, et al. miR-24 limits aortic vascular inflammation and murine abdominal aneurysm development. Nat Commun. 2014;5:5214. doi:10.1038/ncomms6214
- Ling H, Guo Z, Du S, et al. Serum exosomal miR-122-5p is a new biomarker for both acute coronary syndrome and underlying coronary artery stenosis. Biomarkers. 2020;25(7):539–547. doi:10.1080/1354750x.2020.1803963
- Xue S, Liu D, Zhu W, et al. Circulating MiR-17-5p, MiR-126-5p and MiR-145-3p are novel biomarkers for diagnosis of acute myocardial infarction. Front Physiol. 2019;10:123. doi:10.3389/fphys.2019.00123
- Ghosh S, Bhowmik S, Majumdar S, et al. The exosome encapsulated microRNAs as circulating diagnostic marker for hepatocellular carcinoma with low alpha-fetoprotein. Int J Cancer. 2020;147(10):2934–2947. doi:10.1002/ijc.33111
- Gonzalez-Villasana V, Rashed MH, Gonzalez-Cantú Y, et al. Presence of circulating miR-145, miR-155, and miR-382 in exosomes isolated from serum of breast cancer patients and healthy donors. Dis Markers. 2019;2019:6852917. doi:10.1155/2019/6852917
- Li D, Wang J, Ma LJ, et al. Identification of serum exosomal miR-148a as a novel prognostic biomarker for breast cancer. Eur Rev Med Pharmacol Sci. 2020;24(13):7303–7309. doi:10.26355/eurrev_202007_21889
- Zhao P, Cheng J, Li B, et al. Up-regulation of the expressions of MiR-149-5p and MiR-99a-3p in exosome inhibits the progress of pituitary adenomas. Cell Biol Toxicol. 2021;37(4):633–651. doi:10.1007/s10565-020-09570-0
- Xiong Y, Tang Y, Fan F, et al. Exosomal hsa-miR-21-5p derived from growth hormone-secreting pituitary adenoma promotes abnormal bone formation in acromegaly. Transl Res. 2020;215:1–16. doi:10.1016/j.trsl.2019.07.013
- Yu T, Wang Y, Fan Y, et al. CircRNAs in cancer metabolism: a review. J Hematol Oncol. 2019;12(1):90. doi:10.1186/s13045-019-0776-8
- Lin L, Cai GX, Zhai XM, et al. Plasma-derived extracellular vesicles circular RNAs serve as biomarkers for breast cancer diagnosis. Front Oncol. 2021;11:752651. doi:10.3389/fonc.2021.752651
- Wang M, Wang Y, Ye F, et al. Exosome encapsulated ncRNAs in the development of HCC: potential circulatory biomarkers and clinical therapeutic targets. Am J Cancer Res. 2021;11(8):3794–3812.
- Chen W, Quan Y, Fan S, et al. Exosome-transmitted circular RNA hsa_circ_0051443 suppresses hepatocellular carcinoma progression. Cancer Lett. 2020;475:119–128. doi:10.1016/j.canlet.2020.01.022
- Zhang X, Wang S, Wang H, et al. Circular RNA circNRIP1 acts as a microRNA-149-5p sponge to promote gastric cancer progression via the AKT1/mTOR pathway. Mol Cancer. 2019;18(1):20. doi:10.1186/s12943-018-0935-5
- Li T, Zhou T, Wu J, et al. Plasma exosome-derived circGAPVD1 as a potential diagnostic marker for colorectal cancer. Transl Oncol. 2023;31:101652. doi:10.1016/j.tranon.2023.101652
- Lin LY, Yang L, Zeng Q, et al. Tumor-originated exosomal lncUEGC1 as a circulating biomarker for early-stage gastric cancer. Mol Cancer. 2018;17(1):84. doi:10.1186/s12943-018-0834-9
- Ye Q, Li L, Shao Z, et al. Association between lncRNAs in plasma exosomes and diabetic retinopathy. Front Endocrinol (Lausanne). 2022;13:987488. doi:10.3389/fendo.2022.987488
- Kang JY, Mun D, Kim H, Yun N, Joung B. Serum exosomal long noncoding RNAs as a diagnostic biomarker for atrial fibrillation. Heart Rhythm. 2022;19(9):1450–1458. doi:10.1016/j.hrthm.2022.05.033
- Bajo-Santos C, Brokāne A, Zayakin P, et al. Plasma and urinary extracellular vesicles as a source of RNA biomarkers for prostate cancer in liquid biopsies. Front Mol Biosci. 2023;10:980433. doi:10.3389/fmolb.2023.980433
- Zhu L, Li J, Gong Y, et al. Exosomal tRNA-derived small RNA as a promising biomarker for cancer diagnosis. Mol Cancer. 2019;18(1):74. doi:10.1186/s12943-019-1000-8
- Zheng B, Song X, Wang L, et al. Plasma exosomal tRNA-derived fragments as diagnostic biomarkers in non-small cell lung cancer. Front Oncol. 2022;12:1037523. doi:10.3389/fonc.2022.1037523
- Chen S, Zhang X, Meng K, et al. Urinary exosome tsRNAs as novel markers for diagnosis and prediction of lupus nephritis. Front Immunol. 2023;14:1077645. doi:10.3389/fimmu.2023.1077645
- Hu L, Zhang T, Ma H, et al. Discovering the secret of diseases by incorporated tear exosomes analysis via rapid-isolation system: iTEARS. ACS Nano. 2022;16:11720–11732. doi:10.1021/acsnano.2c02531
- Zhao H, Pan S, Natalia A, et al. A hydrogel-based mechanical metamaterial for the interferometric profiling of extracellular vesicles in patient samples. Nat Biomed Eng. 2022;7:135–148. doi:10.1038/s41551-022-00954-7
- Alvarez-Erviti L, Seow Y, Yin H, Betts C, Lakhal S, Wood MJ. Delivery of siRNA to the mouse brain by systemic injection of targeted exosomes. Nat Biotechnol. 2011;29(4):341–345. doi:10.1038/nbt.1807
- Cooper JM, Wiklander PB, Nordin JZ, et al. Systemic exosomal siRNA delivery reduced alpha-synuclein aggregates in brains of transgenic mice. Mov Disord. 2014;29(12):1476–1485. doi:10.1002/mds.25978
- Liu Y, Li D, Liu Z, et al. Targeted exosome-mediated delivery of opioid receptor Mu siRNA for the treatment of morphine relapse. Sci Rep. 2015;5:17543. doi:10.1038/srep17543
- Möller A, Lobb RJ. The evolving translational potential of small extracellular vesicles in cancer. Nat Rev Cancer. 2020;20(12):697–709. doi:10.1038/s41568-020-00299-w
- Srinivasan S, Yeri A, Cheah PS, et al. Small RNA sequencing across diverse biofluids identifies optimal methods for exRNA isolation. Cell. 2019;177(2):446–462.e16. doi:10.1016/j.cell.2019.03.024
- György B, Hung ME, Breakefield XO, Leonard JN. Therapeutic applications of extracellular vesicles: clinical promise and open questions. Annu Rev Pharmacol Toxicol. 2015;55:439–464. doi:10.1146/annurev-pharmtox-010814-124630
- Murphy DE, de Jong OG, Brouwer M, et al. Extracellular vesicle-based therapeutics: natural versus engineered targeting and trafficking. Exp Mol Med. 2019;51(3):1–12. doi:10.1038/s12276-019-0223-5
- Hoppstädter J, Dembek A, Linnenberger R, et al. Toll-like receptor 2 release by macrophages: an anti-inflammatory program induced by glucocorticoids and lipopolysaccharide. Front Immunol. 2019;10:1634. doi:10.3389/fimmu.2019.01634
- Mittelbrunn M, Gutiérrez-Vázquez C, Villarroya-Beltri C, et al. Unidirectional transfer of microRNA-loaded exosomes from T cells to antigen-presenting cells. Nat Commun. 2011;2:282. doi:10.1038/ncomms1285
- Yu M, Gai C, Li Z, et al. Targeted exosome-encapsulated erastin induced ferroptosis in triple negative breast cancer cells. Cancer Sci. 2019;110(10):3173–3182. doi:10.1111/cas.14181
- Li S, Wu Y, Ding F, et al. Engineering macrophage-derived exosomes for targeted chemotherapy of triple-negative breast cancer. Nanoscale. 2020;12(19):10854–10862. doi:10.1039/d0nr00523a
- Zhu ZH, Jia F, Ahmed W, et al. Neural stem cell-derived exosome as a nano-sized carrier for BDNF delivery to a rat model of ischemic stroke. Neural Regen Res. 2023;18(2):404–409. doi:10.4103/1673-5374.346466
- Sheller-Miller S, Radnaa E, Yoo JK, et al. Exosomal delivery of NF-κB inhibitor delays LPS-induced preterm birth and modulates fetal immune cell profile in mouse models. Sci Adv. 2021;7(4):54.
- Wang X, Zhang H, Yang H, et al. Cell-derived exosomes as promising carriers for drug delivery and targeted therapy. Curr Cancer Drug Targets. 2018;18(4):347–354. doi:10.2174/1568009617666170710120311
- Tan A, Rajadas J, Seifalian AM. Exosomes as nano-theranostic delivery platforms for gene therapy. Adv Drug Deliv Rev. 2013;65(3):357–367. doi:10.1016/j.addr.2012.06.014
- Yang Z, Shi J, Xie J, et al. Large-scale generation of functional mRNA-encapsulating exosomes via cellular nanoporation. Nat Biomed Eng. 2020;4(1):69–83. doi:10.1038/s41551-019-0485-1
- Gebeyehu A, Kommineni N, Meckes DG, Sachdeva MS. Role of exosomes for delivery of chemotherapeutic drugs. Crit Rev Ther Drug Carrier Syst. 2021;38(5):53–97. doi:10.1615/CritRevTherDrugCarrierSyst.2021036301
- Sun J, Shen H, Shao L, et al. HIF-1α overexpression in mesenchymal stem cell-derived exosomes mediates cardioprotection in myocardial infarction by enhanced angiogenesis. Stem Cell Res Ther. 2020;11(1):373. doi:10.1186/s13287-020-01881-7
- Zheng Y, Song A, Zhou Y, et al. Identification of extracellular vesicles-transported miRNAs in Erlotinib-resistant head and neck squamous cell carcinoma. J Cell Commun Signal. 2020;14(4):389–402. doi:10.1007/s12079-020-00546-7
- McCann J, Sosa-Miranda CD, Guo H, et al. Contaminating transfection complexes can masquerade as small extracellular vesicles and impair their delivery of RNA. J Extracell Vesicles. 2022;11(10):e12220. doi:10.1002/jev2.12220
- Lamichhane TN, Raiker RS, Jay SM. Exogenous DNA loading into extracellular vesicles via electroporation is size-dependent and enables limited gene delivery. Mol Pharm. 2015;12(10):3650–3657. doi:10.1021/acs.molpharmaceut.5b00364
- Gomari H, Forouzandeh Moghadam M, Soleimani M, Ghavami M, Khodashenas S. Targeted delivery of doxorubicin to HER2 positive tumor models. Int J Nanomedicine. 2019;14:5679–5690. doi:10.2147/ijn.S210731
- Liang G, Kan S, Zhu Y, Feng S, Feng W, Gao S. Engineered exosome-mediated delivery of functionally active miR-26a and its enhanced suppression effect in HepG2 cells. Int J Nanomedicine. 2018;13:585–599. doi:10.2147/ijn.S154458
- Jeyaram A, Lamichhane TN, Wang S, et al. Enhanced loading of functional miRNA cargo via pH gradient modification of extracellular vesicles. Mol Ther. 2020;28(3):975–985. doi:10.1016/j.ymthe.2019.12.007
- Familtseva A, Jeremic N, Tyagi SC. Exosomes: cell-created drug delivery systems. Mol Cell Biochem. 2019;459(1–2):1–6. doi:10.1007/s11010-019-03545-4
- Radnaa E, Richardson LS, Sheller-Miller S, et al. Extracellular vesicle mediated feto-maternal HMGB1 signaling induces preterm birth. Lab Chip. 2021;21(10):1956–1973. doi:10.1039/d0lc01323d
- Fu W, Lei C, Liu S, et al. CAR exosomes derived from effector CAR-T cells have potent antitumour effects and low toxicity. Nat Commun. 2019;10(1):4355. doi:10.1038/s41467-019-12321-3
- Xu Z, Zeng S, Gong Z, Yan Y. Exosome-based immunotherapy: a promising approach for cancer treatment. Mol Cancer. 2020;19(1):160. doi:10.1186/s12943-020-01278-3
- Armstrong JP, Holme MN, Stevens MM. Re-engineering extracellular vesicles as smart nanoscale therapeutics. ACS Nano. 2017;11(1):69–83. doi:10.1021/acsnano.6b07607
- Ma S, Song L, Bai Y, et al. Improved intracellular delivery of exosomes by surface modification with fluorinated peptide dendrimers for promoting angiogenesis and migration of HUVECs. RSC Adv. 2023;13(17):11269–11277. doi:10.1039/d3ra00300k
- Rana S, Yue S, Stadel D, Zöller M. Toward tailored exosomes: the exosomal tetraspanin web contributes to target cell selection. Int J Biochem Cell Biol. 2012;44(9):1574–1584. doi:10.1016/j.biocel.2012.06.018
- Mentkowski KI, Lang JK. Exosomes engineered to express a cardiomyocyte binding peptide demonstrate improved cardiac retention in vivo. Sci Rep. 2019;9(1):10041. doi:10.1038/s41598-019-46407-1
- Liang G, Zhu Y, Ali DJ, et al. Engineered exosomes for targeted co-delivery of miR-21 inhibitor and chemotherapeutics to reverse drug resistance in colon cancer. J Nanobiotechnology. 2020;18(1):10. doi:10.1186/s12951-019-0563-2
- Cheng Q, Shi X, Han M, Smbatyan G, Lenz HJ, Zhang Y. Reprogramming exosomes as nanoscale controllers of cellular immunity. J Am Chem Soc. 2018;140(48):16413–16417. doi:10.1021/jacs.8b10047
- Kim HY, Kim TJ, Kang L, et al. Mesenchymal stem cell-derived magnetic extracellular nanovesicles for targeting and treatment of ischemic stroke. Biomaterials. 2020;243:119942. doi:10.1016/j.biomaterials.2020.119942
- Kamerkar S, Leng C, Burenkova O, et al. Exosome-mediated genetic reprogramming of tumor-associated macrophages by exoASO-STAT6 leads to potent monotherapy antitumor activity. Sci Adv. 2022;8(7):eabj7002. doi:10.1126/sciadv.abj7002
- Kooijmans SAA, Fliervoet LAL, van der Meel R, et al. PEGylated and targeted extracellular vesicles display enhanced cell specificity and circulation time. J Control Release. 2016;224:77–85. doi:10.1016/j.jconrel.2016.01.009
- Kamerkar S, LeBleu VS, Sugimoto H, et al. Exosomes facilitate therapeutic targeting of oncogenic KRAS in pancreatic cancer. Nature. 2017;546(7659):498–503. doi:10.1038/nature22341
- Wei Z, Chen Z, Zhao Y, et al. Mononuclear phagocyte system blockade using extracellular vesicles modified with CD47 on membrane surface for myocardial infarction reperfusion injury treatment. Biomaterials. 2021;275:121000. doi:10.1016/j.biomaterials.2021.121000
- Belhadj Z, He B, Deng H, et al. A combined “eat me/don’t eat me” strategy based on extracellular vesicles for anticancer nanomedicine. J Extracell Vesicles. 2020;9(1):1806444. doi:10.1080/20013078.2020.1806444
- Gómez-Cid L, López-Donaire ML, Velasco D, et al. Cardiac extracellular matrix hydrogel enriched with polyethylene glycol presents improved gelation time and increased on-target site retention of extracellular vesicles. Int J Mol Sci. 2021;22(17):9226. doi:10.3390/ijms22179226
- Liang X, Niu Z, Galli V, et al. Extracellular vesicles engineered to bind albumin demonstrate extended circulation time and lymph node accumulation in mouse models. J Extracell Vesicles. 2022;11(7):e12248. doi:10.1002/jev2.12248
- Wiklander OP, Nordin JZ, O’Loughlin A, et al. Extracellular vesicle in vivo biodistribution is determined by cell source, route of administration and targeting. J Extracell Vesicles. 2015;4:26316. doi:10.3402/jev.v4.26316
- Iancu EM, Kandalaft LE. Challenges and advantages of cell therapy manufacturing under good manufacturing practices within the hospital setting. Curr Opin Biotechnol. 2020;65:233–241. doi:10.1016/j.copbio.2020.05.005
- Rohde E, Pachler K, Gimona M. Manufacturing and characterization of extracellular vesicles from umbilical cord-derived mesenchymal stromal cells for clinical testing. Cytotherapy. 2019;21(6):581–592. doi:10.1016/j.jcyt.2018.12.006
- LeBleu VS, Kalluri R. Exosomes as a multicomponent biomarker platform in cancer. Trends Cancer. 2020;6(9):767–774. doi:10.1016/j.trecan.2020.03.007
- Islam MK, Syed P, Lehtinen L, et al. A nanoparticle-based approach for the detection of extracellular vesicles. Sci Rep. 2019;9(1):10038. doi:10.1038/s41598-019-46395-2
- Baranyai T, Herczeg K, Onódi Z, et al. Isolation of exosomes from blood plasma: qualitative and quantitative comparison of ultracentrifugation and size exclusion chromatography methods. PLoS One. 2015;10(12):e0145686. doi:10.1371/journal.pone.0145686
- Stranska R, Gysbrechts L, Wouters J, et al. Comparison of membrane affinity-based method with size-exclusion chromatography for isolation of exosome-like vesicles from human plasma. J Transl Med. 2018;16(1):1. doi:10.1186/s12967-017-1374-6
- Kitka D, Mihály J, Fraikin JL, Beke-Somfai T, Varga Z. Detection and phenotyping of extracellular vesicles by size exclusion chromatography coupled with on-line fluorescence detection. Sci Rep. 2019;9(1):19868. doi:10.1038/s41598-019-56375-1
- Van Deun J, Jo A, Li H, et al. Integrated dual-mode chromatography to enrich extracellular vesicles from plasma. Adv Biosyst. 2020;4(12):e1900310. doi:10.1002/adbi.201900310
- Zhang X, Borg EGF, Liaci AM, Vos HR, Stoorvogel W. A novel three step protocol to isolate extracellular vesicles from plasma or cell culture medium with both high yield and purity. J Extracell Vesicles. 2020;9(1):1791450. doi:10.1080/20013078.2020.1791450
- Konoshenko MY, Lekchnov EA, Vlassov AV, Laktionov PP. Isolation of extracellular vesicles: general methodologies and latest trends. Biomed Res Int. 2018;2018:8545347. doi:10.1155/2018/8545347
- Bordas M, Genard G, Ohl S, et al. Optimized protocol for isolation of small extracellular vesicles from human and murine lymphoid tissues. Int J Mol Sci. 2020;21(15):5586. doi:10.3390/ijms21155586
- Sidhom K, Obi PO, Saleem A. A review of exosomal isolation methods: is size exclusion chromatography the best option? Int J Mol Sci. 2020;21(18):6466. doi:10.3390/ijms21186466
- Nordin JZ, Lee Y, Vader P, et al. Ultrafiltration with size-exclusion liquid chromatography for high yield isolation of extracellular vesicles preserving intact biophysical and functional properties. Nanomedicine. 2015;11(4):879–883. doi:10.1016/j.nano.2015.01.003
- Soltani F, Parhiz H, Mokhtarzadeh A, Ramezani M. Synthetic and biological vesicular nano-carriers designed for gene delivery. Curr Pharm Des. 2015;21(42):6214–6235. doi:10.2174/1381612821666151027153410
- Witwer KW, Wolfram J. Extracellular vesicles versus synthetic nanoparticles for drug delivery. Nat Rev Mater. 2021;6(2):103–106. doi:10.1038/s41578-020-00277-6
- Severic M, Ma G, Pereira SGT, Ruiz A, Cheung CCL, Al-Jamal WT. Genetically-engineered anti-PSMA exosome mimetics targeting advanced prostate cancer in vitro and in vivo. J Control Release. 2021;330:101–110. doi:10.1016/j.jconrel.2020.12.017
- Zhao Q, Hai B, Zhang X, Xu J, Koehler B, Liu F. Biomimetic nanovesicles made from iPS cell-derived mesenchymal stem cells for targeted therapy of triple-negative breast cancer. Nanomedicine. 2020;24:102146. doi:10.1016/j.nano.2019.102146
- Li YJ, Wu JY, Wang JM, Hu XB, Xiang DX. Emerging strategies for labeling and tracking of extracellular vesicles. J Control Release. 2020;328:141–159. doi:10.1016/j.jconrel.2020.08.056
- Thippabhotla S, Zhong C, He M. 3D cell culture stimulates the secretion of in vivo like extracellular vesicles. Sci Rep. 2019;9(1):13012. doi:10.1038/s41598-019-49671-3
- de Abreu RC, Fernandes H, da Costa Martins PA, Sahoo S, Emanueli C, Ferreira L. Native and bioengineered extracellular vesicles for cardiovascular therapeutics. Nat Rev Cardiol. 2020;17(11):685–697. doi:10.1038/s41569-020-0389-5
- Ikeda G, Santoso MR, Tada Y, et al. Mitochondria-rich extracellular vesicles from autologous stem cell-derived cardiomyocytes restore energetics of ischemic myocardium. J Am Coll Cardiol. 2021;77(8):1073–1088. doi:10.1016/j.jacc.2020.12.060
- Villa A, Garofalo M, Crescenti D, et al. Transplantation of autologous extracellular vesicles for cancer-specific targeting. Theranostics. 2021;11(5):2034–2047. doi:10.7150/thno.51344
- Lener T, Gimona M, Aigner L, et al. Applying extracellular vesicles based therapeutics in clinical trials - an ISEV position paper. J Extracell Vesicles. 2015;4:30087. doi:10.3402/jev.v4.30087
- Escudier B, Dorval T, Chaput N, et al. Vaccination of metastatic melanoma patients with autologous dendritic cell (DC) derived-exosomes: results of the first phase I clinical trial. J Transl Med. 2005;3(1):10. doi:10.1186/1479-5876-3-10
- Kumar DN, Chaudhuri A, Aqil F, et al. Exosomes as emerging drug delivery and diagnostic modality for breast cancer: recent advances in isolation and application. Cancers. 2022;14(6):1435. doi:10.3390/cancers14061435
- Huda MN, Nafiujjaman M, Deaguero IG, et al. Potential use of exosomes as diagnostic biomarkers and in targeted drug delivery: progress in clinical and preclinical applications. ACS Biomater Sci Eng. 2021;7(6):2106–2149. doi:10.1021/acsbiomaterials.1c00217