Abstract
Ultrasound is an important local stimulus for triggering drug release at the target tissue. Ultrasound-responsive drug delivery systems (URDDS) have become an important research focus in targeted therapy. URDDS include many different formulations, such as microbubbles, nanobubbles, nanodroplets, liposomes, emulsions, and micelles. Drugs that can be loaded into URDDS include small molecules, biomacromolecules, and inorganic substances. Fields of clinical application include anticancer therapy, treatment of ischemic myocardium, induction of an immune response, cartilage tissue engineering, transdermal drug delivery, treatment of Huntington’s disease, thrombolysis, and disruption of the blood–brain barrier. This review focuses on recent advances in URDDS, and discusses their formulations, clinical application, and problems, as well as a perspective on their potential use in the future.
Introduction
Creating a drug delivery system that responds to a stimulus exerted by an external force or produced by the target tissue itself is of great research interest. Many physical and chemical stimuli in the microenvironment, such as heat, magnets, enzymes, and pH, can be used as triggers.Citation1
Ultrasound consists of pressure waves at frequencies of 20 kHz or greater. Like optical and audio waves, ultrasonic waves can be focused, reflected, and refracted through a medium. As a mature medical technology, ultrasound imaging can be used repeatedly without concern about residual radiation.Citation2 Therefore, ultrasound imaging is acceptable to most patients and the equipment involved is generally less expensive than that of other imaging technologies.
However, blood is a poor scatterer of ultrasound waves at clinical diagnostic transmitting frequencies, which lie between 1 MHz and 40 MHz. To enhance contrast in ultrasound imaging, so-called ultrasound contrast agents, consisting of gas encapsulated in biodegradable shells, have been developed. With their gas-nuclear structure, ultrasound contrast agents can oscillate in ultrasonic pressure with volume expansion and contraction. This phenomenon is known as acoustic cavitation.Citation3
Cavitation at a higher ultrasonic pressure will result in more violent oscillation of ultrasound contrast agents, leading eventually to their destruction. When drugs are loaded into ultrasound contrast agents, acoustic cavitation can be used as a trigger to release drugs at desired sites.Citation4–Citation7
In recent times, drug-loaded ultrasound contrast agent systems, also termed ultrasound-responsive drug delivery systems (URDDS), have become an increasing focus of research. URDDS include microbubbles,Citation8–Citation10 nanobubbles,Citation11,Citation12 nanodroplets,Citation13 liposomes,Citation14,Citation15 emulsion,Citation16 and micelles.Citation17–Citation19 A combination of two or more formulations can be used as URDDS, such as liposomal bubblesCitation20,Citation21 and microemulsions.Citation22 The drugs loaded can include inorganic substances, eg, titanium dioxide,Citation23 small molecules (curcumin,Citation24 doxorubicin,Citation25 cisplatin,Citation5 epirubicin hydrochloride,Citation26 10-hydroxycamptothecinCitation27), proteins,Citation28,Citation29 small interfering RNA,Citation8,Citation21 DNA,Citation30 and antisense oligodeoxynucleotides.Citation31,Citation32 Their therapeutic applications include anticancer therapy,Citation23,Citation33 treatment of ischemic myocardium,Citation29 induction of an immune response,Citation34 cartilage tissue engineering,Citation35 transdermal drug delivery,Citation36,Citation37 treatment of Huntington’s diseaseCitation38 and thrombolysis,Citation39,Citation40 and disruption of the blood–brain barrier.Citation41,Citation42 This review focuses on recent advances in URDDS, and discusses their formulations, clinical applications, and problems, with a perspective on their potential use in the future.
Multiple ultrasound-responsive drug delivery systems
Microbubbles
Ultrasound exposure alone had been proven to enhance cell membrane permeability,Citation43 and addition of microbubbles has a significant potentiating effect.Citation31 If cells are located in close proximity to gas-filled microbubbles which serve as cavitation nuclei, permeability of the cell membrane will be increased.Citation6,Citation44 In the last decade, microbubble agents have been investigated as carriers for systemic drug administration. Plasmid DNA and other therapeutic materials may adhere to the microbubbles or be packed into the microbubbles, which is beneficial for targeted drug delivery. The possible mechanism of gene transfection or targeted drug delivery is related to sonoporation. High-speed microstreams or microjets cause shear stress on the cell membrane, and transient nonlethal holes appear in the membrane. The pressure gradient and shear stress enhance the permeability of the cell membrane, enabling transport of plasmid DNA or therapeutics into the cell.Citation30
As shown in , microbubbles are one type of widely used ultrasound contrast agent, and are microsized (1–10 μm) gas bubbles with a shell composed of phospholipids, polymers, or proteins (). First-generation microbubbles are air-filled microspheres, with mean diameters in the range of 1–8 μm, that are capable of passing through pulmonary capillaries. However, these air-filled microbubbles disappear from the bloodstream rapidly because of low resistance to arterial pressure gradients and the high solubility of air in blood.Citation45 Therefore, second-generation contrast agents have been developed using microbubbles filled with high molecular weight hydrophobic gases, including perfluorocarbons or sulfur hexafluoride (). Surfactants, albumin, and phospholipids are used to form the bubble shell in order to improve stability in the bloodstream. Sonazoid® (GE Healthcare, Chalfont, UK), a phosphatidylserine microbubble agent filled with perfluorobutane, is a contrast agent specifically targeted to tumor tissue in the liver.Citation46,Citation47 Sonazoid is taken up by Kupffer cells (macrophages) in the liver, enhancing contrast in the liver parenchyma within about 10 minutes of injection, whereas the contrast effects in tumors that lack Kupffer cells are not enhanced.Citation47 Sonazoid has been commercially available in Japan since 2007.
Table 1 Main commercially available ultrasound contrast agents
Figure 1 Schematic illustration of various ultrasound-responsive drug delivery systems. (A) Microbubbles, (B) nanobubbles and nanodroplets, (C) polymeric micelles, and (D) microemulsions.
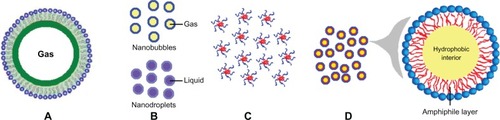
Microbubbles are a suitable ultrasound contrast agent because they can interact with the ultrasound wave.Citation48 Since the 1990s, a number of research groups have designed drug-loaded microbubbles. As shown in , their advantages include visualization of drug-loaded microbubbles with low acoustic pressures, ie, image-guided drug delivery; protection of biopharmaceuticals, such as proteins and nucleic acids, against being degraded on administration; targeted drug delivery to specific tissue (ie, tissue that is exposed to ultrasound) and thus reducing side effects; and enhanced uptake by cells via the cavitation effect upon exposure to ultrasound.
Methods used to prepare drug-loaded microbubbles include association of drugs with the shell,Citation25 covalent linkage with the building blocks, and drug encapsulation in an oil reservoir within the core of the microbubble.Citation24 Another preparation method involves packing the drug into nanoparticles, which are subsequently attached to the surface of the microbubble.Citation49
Nanobubbles and nanodroplets
A major disadvantage of microbubbles as drug delivery systems is their relatively large size (1–10 μm), which is a problem for microbubbles needing to penetrate through the epithelial cells of the vasculature to the target tissue. On intravenous injection, microbubbles are infused into the circulation and eventually become trapped in the lungs where gas exchange occurs. Therefore, drug-loaded microbubbles are mainly restricted to cardiovascular targets and tumor endothelium.
To overcome this limitation, nanobubblesCitation11,Citation20 and nanodropletsCitation13 with sizes smaller than 1 μm have been developed (). Nanobubbles are generally prepared by sonicating in the presence of a fluorinated gas, such as perfluorocarbons or sulfur hexafluoride. This method has been used successfully in the delivery of plasmid DNA, small interfering RNA, and coumarin.Citation11 With their low boiling points, fluorocarbon gases can be evaporated easily on exposure to the thermal effects of ultrasound. For example, a liquid fluorocarbon can be emulsified in water at a nanoscale particle size and vaporized into gas bubbles.Citation50,Citation51 Via the enhanced permeability and retention effect, these nanoscale droplets can penetrate into tumor tissue after intravenous administration.Citation52 When ultrasound is focused on the tumor tissue, a liquid to gas phase transition occurs because of the thermal effect, and ultrasonic microbubbles are formed in situ.Citation53
Polymeric micelles
As shown in , polymeric micelles are formed spontaneously in aqueous solutions containing amphiphilic block copolymers and have a core-shell architecture. Self-assembly occurs when the copolymer concentration reaches a threshold value, known as the critical micelle concentration. The size of polymeric micelles varies from 10 nm to 100 nm. In previously reported research, release of doxorubicin from Pluronic micelles was influenced by high-frequency ultrasound.Citation19 Onset of transient cavitation and release of doxorubicin from micelles were observed at much higher power densities than at low-frequency ultrasound (20–100 kHz). Even a short exposure to high-frequency ultrasound of 15–20 seconds can significantly enhance the intracellular uptake of Pluronic micelles.
Other research has demonstrated that continuous wave and pulsed ultrasound enhances uptake of doxorubicin from phosphate-buffered solution and from Pluronic micelles.Citation17 The main factor influencing drug uptake is density of the ultrasound, with drug uptake being enhanced with increasing power. These observations imply two independent mechanisms controlling acoustic activation of drug uptake from Pluronic micelles. The first is acoustically triggered drug release from micelles, that results in a higher concentration of free drug in the incubation medium. The second mechanism is based on the perturbation of cell membranes, resulting in increased uptake of the drug encapsulated within the micelles.
Other researchers have investigated the chemical conjugation of doxorubicin with polymeric micelles,Citation54 which were found to enhance the antitumor activity of doxorubicin and to circumvent multidrug resistance in A549 cells (). With their low critical concentration, high loading efficiency, diameter in the nano-range, good penetration ability, and controlled-release behavior, polymeric micelles might be developed as a new type of URDDS for cancer therapy.
Figure 3 Fluorescence microscopy of A549 cells containing doxorubicin polymeric micelles, (A) A549 cells incubated with free doxorubicin and (B) A549 cells incubated with polymeric micelles conjugated with doxorubicin.
Note: Doxorubicin concentration = 10 μg/mL. Copyright © 2011. Elsevier. Reproduced with permission from Zhao Y, Sun C, Lu C, et al. Characterization and anti-tumor activity of chemical conjugation of doxorubicin in polymeric micelles (DOX-P) in vitro. Cancer Lett. 2011;311:187–194.Citation54
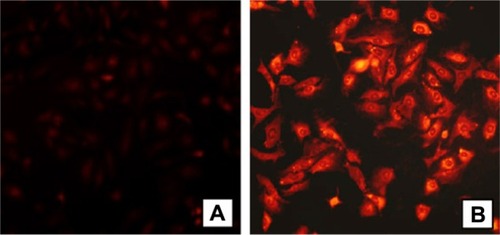
Microemulsions
A microemulsion is a system of water, oil, and an amphiphile, and exists a single optically isotropic and thermodynamically stable liquid solution (). Surfactant molecules that stabilize microemulsions are often the same as those that form micelles. Accumulation of microemulsions in tumor tissue has been confirmed by ultrasound imaging.
Rapoport et al have reported that a paclitaxel-loaded microemulsion converted into microbubbles locally in tumor tissue under the action of tumor-directed therapeutic ultrasound.Citation22 Systemic injection of a drug-loaded microemulsion combined with therapeutic ultrasound achieved dramatic regression of ovarian, breast, and orthotopic pancreatic tumors in animal experiments, indicating efficient ultrasound-triggered drug release from nanodroplets accumulated in the tumor. No curative effect of the nanodroplet-ultrasound combination was observed without the drug, indicating that the therapeutic effect was attributable to the ultrasound-enhanced chemotherapeutic action of the tumor-targeted drug, rather than the mechanical or thermal action of ultrasound itself.
Curcumin, an important natural antioxidant and anticancer compound, can be stably entrapped into microemulsion droplets of average size 20–35 nm.Citation24 To release curcumin, low frequency (40 kHz) ultrasound at an intensity of 3.8 W/cm2 or 9.8 W/cm2 was applied to the microemulsion using a probe sonicator. On insonation, much of the curcumin was released from the microemulsion. The initial release rate (assayed by rate of change in absorption) was as high as 0.11 μg (1.87%) per second in phosphate-buffered saline solution at neutral pH, but decreased at acidic pH. Although the cargo in a microemulsion is partitioned rather than encapsulated (for example, in liposomes), microemulsions might be feasible for some types of ultrasonic-responsive drug delivery.
Clinical application of URDDS
Transdermal drug delivery
Use of ultrasound to deliver drugs more efficiently through the skin is referred to as sonophoresis.Citation55 In earlier studies, the ultrasound used most often was high-frequency sonophoresis (frequencies ≥ 0.7 MHz). Typical enhancement of skin penetration using high-frequency sonophoresis was about 1–10-fold.Citation56 Because the cavitation effect correlated negatively with the frequency of the ultrasound,Citation57 Mitragotri et al hypothesized that low-frequency sonophoresis (20–100 kHz) should be more effective in enhancing skin permeability.Citation56 It was shown that low-frequency sonophoresis at 20 kHz was three orders of magnitude more efficient than high-frequency sonophoresis at 1 MHz.
Parameters influencing sonophoresis include the ultrasound duty cycle (ratio of the time that ultrasound is on), distance between the ultrasonic horn and the skin, treatment duration, and composition of the ultrasound coupling medium (ie, an aqueous solution or a gel-like formulation). Commonly used ultrasound duty cycles are 10% (eg, 0.1 second on and 0.9 second off),Citation58,Citation59 50% (eg, 5 seconds on and 5 seconds off),Citation36,Citation60,Citation61 or continuous application.Citation62,Citation63 The horn-to-skin distances used range from the ultrasound horn being in direct contact with the skin (zero distance) to 4.0 cm.Citation62 The most common distance with low-frequency sonophoresis ranges from 0.3 cm to 1.0 cm,Citation63–Citation65 and is much smaller than for high-frequency sonophoresis. Treatment times can vary from a few secondsCitation66,Citation67 to a few minutes,Citation36,Citation60 to hours to days.Citation58,Citation68 The viscosity, surface tension, density, acoustic impedance, and other bulk and interfacial properties of the coupling medium play an important role in enhancement of skin permeability.
Disruption of blood–brain barrier
The blood–brain barrier is the brain’s first line of defense against harmful substances in the blood stream, and is composed of endothelial cells supported by neurovascular cells. The capillary network in the brain is dense (forming an area of about 20 m2 per 1300 g of human brain).Citation69 Transport across the blood–brain barrier involves movement across the luminal and abluminal membranes of the capillary endothelium. The adjacent endothelial cells are cemented together by tight junctions, preventing involvement of the paracellular pathway.
Targeted ultrasound-induced disruption of the blood–brain barrier may offer a solution to the problem of delivery of small-molecule and large-molecule drugs to the brain. Ultrasound-based techniques could be used in clinical experiments for most disorders of the central nervous system. Thresholds for disruption of the blood–brain barrier have been estimated to be 0.69, 0.47, and 0.36 MPafor 0.1, 1 and 10 msec bursts, respectively.Citation41
For example, focused ultrasound significantly enhanced penetration of 1,3-bis(2-chloroethyl)-1-nitrosourea (BCNU) through the blood–brain barrier in normal brains (by 340%) and tumor-implanted brains (by 202%) without causing hemorrhage.Citation42 Treatment of tumor-implanted rats with focused ultrasound alone had no beneficial effect on tumor progression or on animal survival up to 60 days, and administration of BCNU only transiently controlled tumor progression. However, compared with untreated controls, animal survival was improved by treatment with BCNU only (increase in median survival time [ISTmedian], 15.7%, P = 0.023). Treatment with focused ultrasound before administration of BCNU demonstrated controlled tumor progression (0.05 ± 0.1 cm3 versus 0.28 ± 0.1 cm3, day 31) and improved animal survival when compared with untreated controls (ISTmedian, 85.9%, P = 0.0015).
In a study of Rhesus macaques, McDannold et al evaluated whether an intravenously circulating microbubble agent combined with ultrasound could be applied safely, reliably, and in a controlled manner to temporarily permeabilize the blood–brain barrier.Citation70 All animals in this study recovered from each ultrasound session without behavioral deficits, visual deficits, or loss of visual acuity. Disruption of the blood–brain barrier using this clinical strategy was reliably and repeatedly produced without evidence of histological or functional damage in this clinically relevant animal model.
Therapy for thrombotic disease
Advances in ultrasound-guided delivery of therapy for thrombotic disease have been encouraging. A novel nanosized delivery system for tissue-type plasminogen activator (t-PA) was designed that suppressed the thrombolytic activity of t-PA, which recovered only when exposed to ultrasound.Citation71 t-PA-cationized gelatin and PEGylated gelatin was used to form nanosized complexes by simple mixing with PEG chains. The t-PA activity in the PEGylated complexes was suppressed significantly to 45% that of nonmodified t-PA. However, when exposed to ultrasound in vitro, the activity of t-PA recovered fully. The half-life of the complexed t-PA in the circulation was prolonged by approximately threefold. Further, in a rabbit model of thrombosis, intravenous administration of PEGylated complexes followed by exposure to ultrasound resulted in complete recanalization, which was in marked contrast with administration of the complex alone. It was concluded that the PEG-modified complex is a promising delivery system for t-PA which can enhance biological activity at the target site when local ultrasound irradiation is used.
In another study, urokinase-type plasminogen activator was encapsulated in hollow nanogels in the size range of 200–300 nm, and found to have a longer circulation time than that of the naked urokinase in vivo.Citation39 Protein release could be triggered more rapidly under diagnostic ultrasound conditions at 2 MHz, and significantly enhanced thrombolysis of clots. These results are promising in terms of increasing the specificity and positive effects of thrombolytic agents, such as recombinant tissue plasminogen activator, in the treatment of ischemic vascular disease.
Most ischemic heart disease results from thrombotic disease. Zhao et al investigated the cardioprotective effect of acidic fibroblast growth factor combined with heparin-modified microbubbles (aFGF-HMB) using an ultrasound technique ().Citation29 Echocardiography of the heart parenchyma was enhanced after injection of aFGF-HMB. The aFGF-HMB suspension demonstrated a good cardioprotective effect in ultrasonic contrast imaging of the heart. M-mode echocardiography showed that a combination of aFGF-HMB and ultrasound markedly stimulated neogenesis in myocardial vessels, resulting in significant improvement of both regional and global contractile function in the myocardium ().
Figure 4 Improving intravenous delivery of acidic fibroblast growth factor to the ischemic myocardium by heparin-modified microbubbles (aFGF-HMB) combined with the UTMD technique. (A) Illustration of the method of administration. (B) M-mode echocardiography for the group that received aFGF-HMB combined with US (ultrasound).Citation29
Note: Copyright © 2012. Informa Healthcare. Reproduced with permission from Zhao Y, Lu C, Li X, et al. Improving the cardio protective effect of aFGF in ischemic myocardium with ultrasound-mediated cavitation of heparin modified microbubbles: preliminary experiment. J Drug Target. 2012;20:623–631.Citation29
Abbreviations: IVST, intraventricular septum thickness; LVDd, left ventricular diastolic dimension; LVPW, left ventricular posterior wall; UTMD, ultrasound-targeted microbubble destruction.
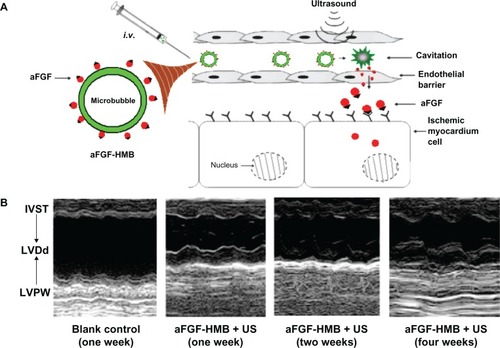
Drug delivery to tumor tissue
URDDS have been used to induce apoptosis in tumor-targeted therapy.Citation15,Citation22,Citation26 There are two ultrasound-induced effects in this respect, ie, thermal and nonthermal (such as radiation pressure and cavitation). Relatively low temperatures (about 43°C) for 30–60 minutes can be used for hyperthermiaCitation72 to sensitize tumors to chemotherapy and radiotherapy. At higher temperatures (>60°C), ultrasound may be used for thermal ablation of tumors in many organs, including the prostate,Citation73,Citation74 liver,Citation75,Citation76 breast,Citation77 bone,Citation78 pancreas,Citation43 and uterus,Citation79 or for thermal coagulation of blood vessels.Citation80
Suzuki et al reported that a combination of nanobubbles and ultrasound could permeabilize cancer cells and potentiate the cytotoxicity of cisplatin and 5-fuorouracilCitation12 in 293T human kidney, MCF7 human breast adenocarcinoma, EMT6 murine mammary carcinoma, and colon 26 murine rectum carcinoma cell lines. Under optimal conditions, nanobubbles (containing albumin or lipid, 10%, v/v) combined with ultrasound (frequency 945 kHz, duty cycle ratio 20%–80%, pressure 0.96 mPa) produced significant cytotoxicity that was not seen with either ultrasound or the drugs used alone. Cytotoxicity could be further enhanced by increasing the duty cycle ratio up to 80%. Rapid collapse of the nanobubbles when combined with ultrasound, led the authors to hypothesize that subnanobubbles, ie, cavitation bubbles, were produced by collapse of the nanobubbles and the shock waves generated by bubble cavitation, leading to transient membrane permeability, followed by entry of plasmid DNA and/or drugs.
Influencing factors and problems
Ultrasound wave and drug delivery
The characteristics of the ultrasound wave, ie, number of cycles per ultrasound pulse, peak negative pressure, and frequency, play an important role in ultrasonic drug delivery. In most reports of URDDS, the frequency of ultrasound waves is set at 1 MHz because this frequency allows microbubbles in the 1–3 μm size range to respond to ultrasound. An ultrasound contrast agent shows inertial cavitation at higher acoustic pressure (above approximately 500 kPa),Citation81 that may result in formation of shockwaves and microjets,Citation82 generate a temporary pore in the cell membrane, and facilitate drug delivery to the cytoplasm of the cell.Citation83,Citation84 If lower acoustic pressures are used (mechanical index < 0.05–0.1), the bubbles oscillate linearly and the reflected frequency is the same as that of the frequency transmitted (). If bubbles are located near the cell membrane, gentle oscillation may cause the cell membrane to become unstable, thereby enhancing endocytosis.Citation81,Citation85 Increased acoustic pressure (0.1 < mechanical index < 0.3) will cause nonlinear expansion and compression of the microbubbles, causing the bubbles to become more resistant to compression than to expansion. This is known as noninertial cavitation (), which results in emission of nonlinear harmonic signals.Citation86 Harmonic imaging with microbubbles can be used to enhance the bubble-to-tissue backscatter signal ratio.Citation2 Higher acoustic pressure (mechanical index > 0.3–0.6) will cause expansion, compression, and disruption of the microbubbles (). This inertial cavitation caused microstreams or microjets to form near bubbles used as flashreplenishment in a diagnostic reperfusion study ().Citation87 These microstreams can reach a peak velocity of 700 m/sec, causing formation of transient pores which increase the permeability of the cell membrane.Citation88
Figure 5 Scheme showing microbubble behavior in acoustic fields.
Notes: At very low intensity, ultrasound induces linear oscillation of the microbubble. At low intensity, ultrasound induces oscillation of the microbubble with a gradual increase in microbubble diameter until it reaches a resonant diameter, at which point stable oscillation occurs. At high intensity, ultrasound causes a rapid increase in the diameter of the microbubble for a few cycles, which induces bubble disruption.
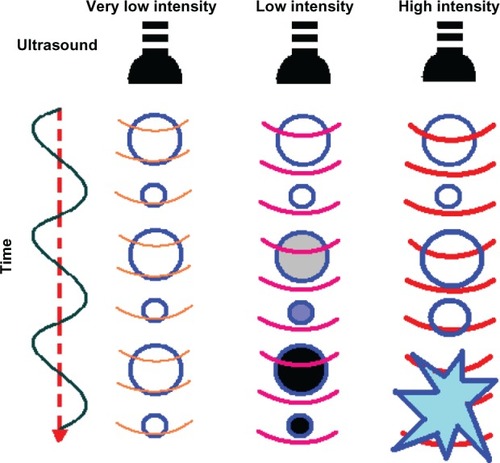
Figure 6 Scheme showing the pore formation in the cell membrane by oscillating or disrupting microbubble. (A) Noninertial cavitation of the microbubble, (B) inertial cavitation causing rupture of the cell membrane, and (C) transmembrane flux of fluid and loaded drugs under sonoporation effect.
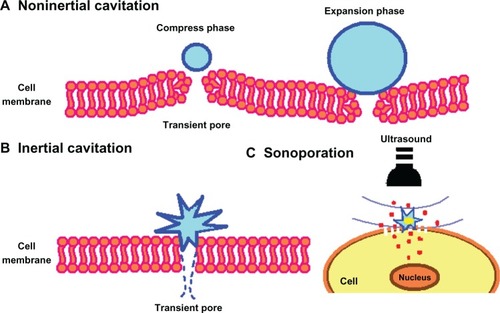
Another important parameter is the number of acoustic cycles (ie, acoustic oscillations per ultrasound pulse). Bubbles will oscillate at lower pressures when 100 cycles are used. However, when more cycles are used in combination with higher pressures, the bubbles are destroyed immediately.Citation89
Characteristics of URDDS in drug delivery
The efficiency of URDDS in targeted therapy can be affected by many factors, including the parameters used in ultrasound exposure, the characteristics of the ultrasound contrast agent, the concentration and molecular weight of the therapeutics loaded into the system, and the physiological characteristics of the disease being treated. In this section, we summarize the characteristics most likely to affect the efficiency of targeted URDDS.
Exposure parameters
To maximize drug release from URDDS, the parameters used for ultrasound exposure must be carefully chosen. Most of the parameters used in ultrasound have been investigated in this regard, with some promising results.
Yeh and Su reported the effects of acoustic parameters on destruction of the ultrasound contrast agent.Citation90 In their experiments, they introduced three insonation parameters, ie, acoustic pressure (0–1 mPa), pulse frequency (1, 2.25, 5, and 7.5 MHz) and pulse length (1–10 cycles). The percentage of the ultrasound contrast agent that survived decreased with decreasing pulse frequency and with increasing transmission acoustic pressure and pulse length. Further, the extent of destruction of the ultrasound contrast agent was not related to the mechanical index.
Xu et al developed a novel strategy to enhance the effect of cavitation.Citation91 In their study, targeting of tumor tissue was initiated by a short, high-intensity sequence of pulses and sustained by lower intensity pulses.
Zhu et al used different pulse periods (0.5 msec, 1 msec, 3.3 ms, 10 msec, 15 msec, 33 msec, and 0.1 and 1 second) to study peak cavitation activity reached by high-intensity focused ultrasound exposure,Citation92 using pulsed high-intensity focused ultrasound (1.05 MHz) with an intensity 2400 W/cm2 and a 1:1 duty cycle (with on phase equal to off phase). The irradiation time was 2 minutes. The cavitation activity for pulsed high-intensity focused ultrasound peaked at a pulse period of 10 msec, and was significantly greater for pulse durations from 2 msec to 20 msec than for other pulse durations.
To ensure a cavitation effect at the target region, Chen et al used high-speed photography to observe formation of cavitation bubble clouds generated by a high-intensity focused ultrasound transducer in water.Citation93 Their results suggest that it might be possible to achieve stable cavitation at the target region in a controllable manner by adjusting the duration of high-intensity focused ultrasound exposure and other physical parameters.
Previous research has shown that the strength of inertial cavitation depends on the initial concentration of the ultrasound contrast agent used.Citation94 Tu et al reported that the strength of inertial cavitation increased significantly with increasing acoustic pressure, concentration of the ultrasound contrast agent, and pulse length, and with decreasing pulse-repetition frequency.Citation95
Characteristics of ultrasound contrast agents and therapeutic loads
Being the main components of URDDS, ultrasound contrast agents and therapeutic loads have an important role to play in future therapeutic application of such systems. With regard to ultrasound contrast agents, important factors affecting the efficiency of drug delivery include concentration, gas encapsulated, components used in the shell, surface modification, and size distribution. Chen et al compared the thresholds for ultrasonic destruction of three specific contrast agents, ie, Sonazoid, Optison® (GE Healthcare, Chalfont, UK), and biSpheres® (Point Biomedical Corporation, San Carlos, CA, USA).Citation96 Albumin-shelled Optison and surfactant-shelled Sonazoid had low fragmentation thresholds (mean 0.13 and 0.15 MPa at 1.1 MHz, and mean 0.48 and 0.58 MPa at 3.5 MHz, respectively), while polymer-shelled biSpheres had a significantly higher threshold (mean 0.19 and 0.23 MPa) for thin-shelled and thick-shell biSpheres (1.1 MHz, 0.73, and 0.96 MPa of thin-shell and thick-shell biSpheres at 3.5 MHz, respectively). At comparable initial concentrations, surfactant-shelled Sonazoid produced a much higher inertial cavitation effect than biSpheres or Optison after destruction of the shell. Thick-shelled biSpheres had the highest fragmentation threshold. The propensity of the bubbles to undergo inertial cavitation depended on the properties of the gas and shell used in the ultrasound contrast agents. According to another report, Optison had a larger effect in terms of increasing the permeability of the blood–brain barrier than Definity® (Lantheus Medical Imaging, North Billerica, MA, USA) when the same pressure amplitude was used.Citation97
The concentration, molecular weight, and surface charge on the loaded therapeutic agent are also critical determinants of successful drug delivery. For instance, therapeutics with low molecular weight can be easily encapsulated into microbubbles, enabling efficient delivery or transfection. When using URDDS for gene delivery, Duvshani-Eshet and Machluf reported that increasing the DNA concentration contributed to increased total gene expression, but did not necessarily improve transfection efficiency.Citation98
Other characteristics of URDDS in drug delivery
Undoubtedly, there are further characteristics that may facilitate the use of URDDS. For example, Miller et al found that the ultrasound-induced cavitation effect was influenced by the amount of gas dissolved in the medium.Citation99 Further, Brotchie et al identified a strong correlation between the radius of the bubbles used and the concentration of gas dissolved in the cavitation medium.Citation100 They also observed an auxiliary function of the electrolyte concentration in the medium.
Although ultrasound contrast agents can be used directly as carriers for therapeutic agents, their gas-nuclear structure limits the space available for loading drugs, especially macromolecules. Nanoscale particles have advantages for drug targeted delivery. Compared with ultrasound contrast agents, nanoscale particles provide both an abundant surface area for modification of particles and an internal volume capable of encapsulating drugs. As mentioned earlier, liposomes, microemulsions, nanodroplets, and polymeric micelles can be used in URDDS to facilitate drug delivery. For example, Chappell et al have reported that nanoparticles loaded with fibroblast growth factor-2 via ultrasonic destruction of microbubbles represent an effective and minimally invasive strategy for targeted stimulation of therapeutic arteriogenesis.Citation101 Further, Marin et al have used Pluronic micelles combined with pulsed ultrasound to enhance uptake of doxorubicin.Citation17
Problems
One important shortcoming of ultrasound is that it is strongly attenuated by bone. Large surface area phase arrays and information derived from modern imaging methods may correct the distortion of ultrasound waves produced by the skull to expose the brain tissue by the focal, trans-skull ultrasound. Recently developed clinical ultrasound systems focused using magnetic resonance imaging may make it possible to deliver therapeutics through the intact skull to targeted regions in the brain, and encouraging results in this regard have been seen in animal experiments.Citation102
Meanwhile, the molecular mechanism underlying the DNA damage induced by URDDS remains subtle and elusive, and needs further study. Furusawa et alCitation103 found that Akt, a substrate of ataxia telangiectasia-mutated and DNA-dependent protein kinase (ATM/DNA-PK), was phosphorylated to the active form in U937 and Molt-4 cell lines without p53 when exposed to ultrasound. Furusawa et alCitation104 observed induction of apoptosis in cancer cells, which strongly supports involvement of a purely mechanical mechanism. These researchers were the first to demonstrate that exposure to ultrasound at even a moderate level of intensity has genotoxic potential because of its ability to damage DNA in cancer cell lines.
Perspectives
URDDS are now a hot topic in drug delivery system research, and the promising results reported so far have encouraged us to shift our attention from fundamental research to potential clinical application of these novel systems, with a focus on the following possibilities.
Theranostics
Theranostics is a promising technique combining therapy and diagnosis via medical imaging, such as in ultrasound-guided magnetic resonance imaging.Citation105 An ultrasound contrast agent can be loaded with FeO2Citation106 or other magnetic nanoparticles to provide contrast in magnetic resonance imaging.Citation107 A breakthrough would be if a drug-loaded ultrasound contrast agent could be developed which visualizes pathophysiological tissues simultaneously. For example, human vascular endothelial growth factor receptor 2-targeted lipopeptide can be incorporated into the microbubble shell to create a molecularly targeted clinical ultrasound contrast agent.Citation108 If chemotherapeutics can be loaded into this ultrasound contrast agent, it will achieve the dual function of theranostics, ie, monitoring of tumor angiogenesis and delivering targeted therapy.
Permeabilization of cell membranes for drug transport
URDDS can offer a nonchemical, nonviral, and noninvasive method for drug transport to target cells. Nearly every stage of transient cavitation, including bubble expansion, collapse, and subsequent shock waves may contribute to membrane permeabilization.Citation109 In addition, pores on cell membranes may be lasting for seconds to minutes,Citation110,Citation111 and even 24 hoursCitation112 after exposure to ultrasound. This is beneficial for drugs when they are penetrating cells. Research in which TO-PRO-3-loaded thermosensitive liposomes and microbubbles released TO-PRO-3 in response to heating with high intensity focused ultrasoundCitation113 has validated this concept. Further, pore formation in the cell membrane promoted uptake of TO-PRO-3 by the target cancer cells. It could be even more advantageous to sonoporate the target tissue followed by administration of drug-loaded ultrasound contrast agents.
Targeted therapy for vascular disease
Because ultrasound contrast agents can be used to image the blood pool, URDDS have been investigated as targeted therapy to the vasculature, including thrombolysis,Citation114 lithotripsy,Citation115 chemotherapy,Citation116 anti-inflammatory treatment,Citation117 stem cell transplantationCitation118,Citation119 and gene therapy for coronary heart disease. In recent years, some researchers have started investigating URDDS as therapy targeted to the brain.Citation120
Transdermal drug delivery
Three aspects of bubble-stratum corneum interaction have been considered, including shock wave emission, microjet penetration into the stratum corneum, and the impact of the microjet on the stratum corneum.Citation121 The most common types of drugs delivered through the skin with high-frequency sonophoresis have been anti-inflammatory medications for joint and muscle pain, with an increasing shift in interest recently from topical steroids to nonsteroidal anti-inflammatory drugs, including diclofenac,Citation122 ibuprofen,Citation123 ketoprofen,Citation124 ketorolac,Citation125 and piroxicam.Citation126 This may reflect the fact that oral NSAIDs generally cause gastrointestinal side effects, including nausea, heartburn, gastrointestinal ulcers, and nonspecific colitis.Citation127 Therefore, the combination of topical NSAID therapy and ultrasound is promising, particularly using high-frequency sonophoresis.
Acknowledgments
This research was supported by grants from the National Natural Science Fund (81272160, 81071164, 81271584, 81000526), Zhejiang Provincial Natural Science Foundation (LY12H30003, LY12H31003, LQ12H30001, LY12H03001, LY12H18001, Y2110587, Y2090834), Beijing Natural Science Foundation (7112100), Scientific Research Joint Funds of Health Ministry and Zhejiang Province (WKJ2011-2-010), Wenzhou Bureau of Science and Technology (Y20090016, H20110008, Y20110034, S20100049, H20100017, Y20100029), Zhejiang Provincial Foundation for Health Department (2011ZDA017, 2012KYB126, 2012KYA134), Zhejiang Provincial Foundation for Education Department (Y201016664), Zhejiang Provincial Project of Key Scientific Group (2010R50042, 2012R10042-06), and the Major Scientific Project of Guangdong Province (2012A080201010).
Disclosure
The authors report no conflicts of interest in this work.
References
- TimkoBPDvirTKohaneDSRemotely triggerable drug delivery systemsAdv Mater201024925494320818618
- MulvaghSLDeMariaANFeinsteinSBContrast echocardiography: current and future applicationsJ Am Soc Echocardiogr20001333134210756254
- BouakazAVersluisMde JongNHigh-speed optical observations of contrast agent destructionUltrasound Med Biol20053139139915749563
- TorchilinVPTargeted pharmaceutical nanocarriers for cancer therapy and imagingAAPS J20079E128E14717614355
- SchroederAHonenRTurjemanKGabizonAKostJBarenholzYUltrasound triggered release of cisplatin from liposomes in murine tumorsJ Control Release2009137636819303426
- ZhaoYZLuoYKLuCTPhospholipids-based microbubbles sonoporation pore size and reseal of cell membrane cultured in vitroJ Drug Target200816182518172816
- RapoportNYChristensenDAFainHDBarrowsLGaoZUltrasound-triggered drug targeting of tumor in vitro and in vivoUltrasonics20044294395015047411
- OtaniKYamaharaKOhnishiSObataHKitamuraSNagayaNNonviral delivery of siRNA into mesenchymal stem cells by a combination of ultrasound and microbubblesJ Control Release200913314615318976686
- SuzukiROdaYUtoguchiNMaruyamaKProgress in the development of ultrasound-mediated gene delivery systems utilizing nano- and microbubblesJ Control Release2011149364120470839
- ZhaoYLuCFuHPhospholipid-based ultrasonic microbubbles for loading protein and ultrasound-triggered releaseDrug Dev Ind Pharm2009351121112719555252
- WangYLiXZhouYHuangPXuYPreparation of nanobubbles for ultrasound imaging and intracelluar drug deliveryInt J Pharm201038414815319781609
- SuzukiMKoshiyamaKShinoharaFNanobubbles enhanced drug susceptibility of cancer cells using ultrasoundInternational Congress Series20051284338339
- DuLJinYZhouWZhaoJUltrasound-triggered drug release and enhanced anticancer effect of doxorubicin-loaded poly (D,L-lactide-co-glycolide)-methoxy-poly(ethylene glycol) nanodropletsUltrasound Med Biol2011371252125821683513
- IbsenSBenchimolMSimbergDSchuttCSteinerJEsenerSA novel nested liposome drug delivery vehicle capable of ultrasound triggered release of its payloadJ Control Release201115535836621745505
- ZhaoYDaiDLuCUsing acoustic cavitation to enhance chemotherapy of DOX liposomes: experiment in vitro and in vivoDrug Dev Ind Pharm2012381090109822188116
- MedinaJSalvadoAdel PozoAUse of ultrasound to prepare lipid emulsions of lorazepam for intravenous injectionInt J Pharm20012161811274800
- MarinAMuniruzzamanMRapoportNAcoustic activation of drug delivery from polymeric micelles: effect of pulsed ultrasoundJ Control Release20017123924911295217
- RapoportNCombined cancer therapy by micellar-encapsulated drug and ultrasoundInt J Pharm200427715516215158978
- MarinASunHHusseiniGAPittWGChristensenaDARapoportNYDrug delivery in Pluronic micelles: effect of high-frequency ultrasound on drug release from micelles and intracellular uptakeJ Control Release200284394712399166
- SuzukiRNamaiEOdaYCancer gene therapy by IL-12 gene delivery using liposomal bubbles and tumoral ultrasound exposureJ Control Release201014224525019883708
- NegishiYEndoYFukuyamaTDelivery of siRNA into the cytoplasm by liposomal bubbles and ultrasoundJ Control Release200813212413018804499
- RapoportNYKennedyAMSheaJEScaifeCLNamK-HControlled and targeted tumor chemotherapy by ultrasound-activated nanoemulsions/microbubblesJ Control Release200913826827619477208
- HaradaYOgawaKIrieYUltrasound activation of TiO2 in melanoma tumorsJ Control Release201114919019520951750
- LeeM-HLinH-YChenH-CThomasJLUltrasound mediates the release of curcumin from microemulsionsLangmuir2008241707171318205414
- EisenbreyJRBursteinOMKambhampatiRForsbergFLiuJBWheatleyMADevelopment and optimization of a doxorubicin loaded poly(lactic acid) contrast agent for ultrasound directed drug deliveryJ Control Release2010143384420060024
- LuCZhaoYWuYExperiment on enhancing antitumor effect of intravenous epirubicin hydrochloride by acoustic cavitation in situ combined with phospholipid-based microbubblesCancer Chemother Pharmacol20116834334820978763
- LiPZhengYRanHUltrasound triggered drug release from 10-hydroxycamptothecin-loaded phospholipid microbubbles for targeted tumor therapy in miceJ Control Release201216234935422800580
- LuCZhaoYGaoHComparing encapsulation efficiency and ultrasound-triggered release for protein between phospholipid-based microbubbles and liposomesJ Microencapsul20102711512119538032
- ZhaoYLuCLiXImproving the cardio protective effect of aFGF in ischemic myocardium with ultrasound-mediated cavitation of heparin modified microbubbles: preliminary experimentJ Drug Target20122062363122758395
- YoonCSParkJHUltrasound-mediated gene deliveryExpert Opin Drug Deliv2010732133020166854
- HaagPFrauscherFGradlJMicrobubble-enhanced ultrasound to deliver an antisense oligodeoxynucleotide targeting the human androgen receptor into prostate tumoursJ Steroid Biochem Mol Biol200610210311317055720
- ZhaoYLuCFactors that affect the efficiency of antisense oligodeoxyribonucleotide transfection by insonated gas-filled lipid microbubblesJ Nanopart Res200810449454
- NomikouNMcHaleAPExploiting ultrasound-mediated effects in delivering targeted, site-specific cancer therapyCancer Lett201029613314320598800
- BioleyGLassusABussatPTerrettazJTranquartFCorthésyBGas-filled microbubble-mediated delivery of antigen and the induction of immune responsesBiomaterials2012335935594622652023
- LimaEGDurneyKMSirsiSRMicrobubbles as biocompatible porogens for hydrogel scaffoldsActa Biomater201284334434122868194
- SetoJEPolatBELopezRFVBlankschteinDLangerREffects of ultrasound and sodium lauryl sulfate on the transdermal delivery of hydrophilic permeants: comparative in vitro studies with full-thickness and split-thickness pig and human skinJ Control Release2010145263220346994
- PolatBEHartDLangerRBlankschteinDUltrasound-mediated transdermal drug delivery: mechanisms, scope, and emerging trendsJ Control Release201115233034821238514
- BurgessAHuangYQuerbesWSahDWHynynenKFocused ultrasound for targeted delivery of siRNA and efficient knockdown of Htt expressionJ Control Release201216312512922921802
- JinHTanHZhaoLUltrasound-triggered thrombolysis using urokinase-loaded nanogelsInt J Pharm201243438439022683455
- ZhouXBQinHLiJPlatelet-targeted microbubbles inhibit re-occlusion after thrombolysis with transcutaneous ultrasound and microbubblesUltrasonics20115127027420888024
- VykhodtsevaNMcDannoldNHynynenKProgress and problems in the application of focused ultrasound for blood–brain barrier disruptionUltrasonics20084827929618511095
- LiuH-LHuaM-YChenP-YBlood–brain barrier disruption with focused ultrasound enhances delivery of chemotherapeutic drugs for glioblastoma treatmentRadiology201025541542520413754
- RapoportNKennedyAMSheaJEScaifeCLNamK-HUltrasonic nanotherapy of pancreatic cancer: lessons from ultrasound imagingMol Pharm20097223119899813
- FerraraKPollardRBordenMUltrasound microbubble contrast agents: fundamentals and application to gene and drug deliveryAnnu Rev Biomed Eng2007941544717651012
- KabalnovAKleinDPeluraTSchuttEWeersJDissolution of multicomponent microbubbles in the bloodstream: 1. TheoryUltrasound Med Biol1998247397499695277
- KorenagaKKorenagaMFurukawaMYamasakiTSakaidaIUsefulness of Sonazoid contrast-enhanced ultrasonography for hepatocellular carcinoma: comparison with pathological diagnosis and superparamagnetic iron oxide magnetic resonance imagesJ Gastroenterol20094473374119387532
- WatanabeRMatsumuraMMunemasaTFujimakiMSuematsuMMechanism of hepatic parenchyma-specific contrast of microbubble-based contrast agent for ultrasonography: microscopic studies in rat liverInvest Radiol20074264365117700280
- QinSCaskeyCFFerraraKWUltrasound contrast microbubbles in imaging and therapy: physical principles and engineeringPhys Med Biol200954R27R5719229096
- AzminMMohamediGEdirisingheMStrideEPDissolution of coated microbubbles: the effect of nanoparticles and surfactant concentrationMater Sci Eng C Mater Biol Appl20123226542658
- RapoportNNamKHGuptaRUltrasound-mediated tumor imaging and nanotherapy using drug loaded, block copolymer stabilized perfluorocarbon nanoemulsionsJ Control Release201115341521277919
- SheeranPSLuoisSDaytonPAMatsunagaTOFormulation and acoustic studies of a new phase-shift agent for diagnostic and therapeutic ultrasoundLangmuir201127104121042021744860
- FangJNakamuraHMaedaHThe EPR effect: unique features of tumor blood vessels for drug delivery, factors involved, and limitations and augmentation of the effectAdv Drug Deliv Rev20116313615120441782
- SheeranPSWongVPLuoisSDecafluorobutane as a phase-change contrast agent for low-energy extravascular ultrasonic imagingUltrasound Med Biol2011371518153021775049
- ZhaoYSunCLuCCharacterization and anti-tumor activity of chemical conjugation of doxorubicin in polymeric micelles (DOX-P) in vitroCancer Lett201131118719421872982
- MitragotriSSonophoresis: a 50-year journeyDrug Discov Today2004973573615450237
- MitragotriSBlankschteinDLangerRTransdermal drug delivery using low frequency sonophoresisPharm Res1996134114208692734
- GaertnerWFrequency dependence of ultrasonic cavitationJ Acoust Soc Am195426977980
- KushnerJKimDSoPBlankschteinDLangerRDual-channel two-photon microscopy study of transdermal transport in skin treated with low-frequency ultrasound and a chemical enhancerJ Invest Dermatol20071272832284617554365
- Alvarez-RománRMerinoGKaliaYNaikAGuyRSkin permeability enhancement by low frequency sonophoresis: lipid extraction and transport pathwaysJ Pharm Sci2003921138114612761803
- PolatBFigueroaPBlankschteinDLangerRTransport pathways and enhancement mechanisms within localized and non-localized transport regions in skin treated with low-frequency sonophoresis and sodium lauryl sulfateJ Pharm Sci201110051252920740667
- WollochLKostJThe importance of microjet vs shock wave formation in sonophoresisJ Control Release201014820421120655341
- LeeSChoiKMenonGPenetration pathways induced by low-frequency sonophoresis with physical and chemical enhancers: iron oxide nanoparticles versus lanthanum nitratesJ Invest Dermatol20101301063107219940858
- UedaHMutohMSekiTKobayashiDMorimotoYAcoustic cavitation as an enhancing mechanism of low-frequency sonophoresis for transdermal drug deliveryBiol Pharm Bull20093291692019420764
- LavonIGrossmanNKostJKimmelEEndenGBubble growth within the skin by rectified diffusion might play a significant role in sonophoresisJ Control Release200711724625517197050
- MorimotoYMutohMUedaHElucidation of the transport pathway in hairless rat skin enhanced by low frequency sonophoresis based on the solute-water transport relationship and confocal microscopyJ Control Release200510358759715820406
- SpieringsEBrevardJKatzNTwo-minute skin anesthesia through ultrasound pretreatment and iontophoretic delivery of a topical anesthetic: a feasibility studyPain Med20089555918254767
- BrownMMartinGJonesSAkomeahFDermal and transdermal drug delivery systems: current and future prospectsDrug Deliv20061317518716556569
- TangHBlankschteinDLangerRPrediction of steady-state skin permeabilities of polar and nonpolar permeants across excised pig skin based on measurements of transient diffusion: characterization of hydration effects on the skin porous pathwayJ Pharm Sci2002911891190712115816
- PardridgeWMAdvances in cell biology of blood–brain barrier transportSemin Cell Biol199124194261813031
- McDannoldNArvanitisCDVykhodtsevaNLivingstoneMSTemporary disruption of the blood–brain barrier by use of ultrasound and microbubbles: safety and efficacy evaluation in rhesus macaquesCancer Res2012723652366322552291
- UesugiYKawataHJoJ-iSaitoYTabataYAn ultrasound-responsive nano delivery system of tissue-type plasminogen activator for thrombolytic therapyJ Control Release201014726927720696194
- DiederichCJHynynenKUltrasound technology for hyperthermiaUltrasound Med Biol19992587188710461714
- ChapelonJYRibaultMVernierFSouchonRGeletATreatment of localised prostate cancer with transrectal high intensity focused ultrasoundEur J Ultrasound19999313810099164
- GeletAChapelonJYPoissonnierLLocal recurrence of prostate cancer after external beam radiotherapy: early experience of salvage therapy using high-intensity focused ultrasonographyUrology20046362562915072864
- KohrmannKUMichelMSGaaJMarlinghausEAlkenPHigh intensity focused ultrasound as noninvasive therapy for multifocal renal cell carcinoma: case study and review of the literatureJ Urol20021672397240311992045
- WuFWangZBChenWZExtracorporeal high intensity focused ultrasound ablation in the treatment of patients with large hepatocellular carcinomaAnn Surg Oncol2004111061106915545506
- WuFWangZBCaoYDA randomised clinical trial of high-intensity focused ultrasound ablation for the treatment of patients with localised breast cancerBr J Cancer2003892227223314676799
- ChenWWangZWuFHigh intensity focused ultrasound in the treatment of primary malignant bone tumorZhonghua Zhong Liu Za Zhi200224612615 Chinese12667339
- ChanAHFujimotoVYMooreDEMartinRWVaezySAn image-guided high intensity focused ultrasound device for uterine fibroids treatmentMed Phys2002292611262012462728
- VaezySNobleMLKeshavarziALiver hemostasis with high-intensity ultrasound: repair and healingJ Ultrasound Med Biol200423217225
- AfadziMDaviesCDHansenYHEffect of ultrasound parameters on the release of liposomal calceinUltrasound Med Biol20123847648622264409
- OhlCDAroraMIkinkRSonoporation from jetting cavitation bubblesBiophys J2006914285429516950843
- LentackerIWangNVandenbrouckeREDemeesterJSmedtSCDSandersNNUltrasound exposure of lipoplex loaded microbubbles facilitates direct cytoplasmic entry of the lipoplexesMol Pharm2009645746719718799
- GeersBLentackerIAlonsoAElucidating the mechanisms behind sonoporation with adeno-associated virus-loaded microbubblesMol Pharm201182244225122014166
- MeijeringBDJuffermansLJvan WamelAUltrasound and microbubble-targeted delivery of macromolecules is regulated by induction of endocytosis and pore formationCirc Res200910467968719168443
- UngerECHershEVannanMMatsunagaTOMcCreeryTLocal drug and gene delivery through microbubblesProg Cardiovasc Dis200144455411533926
- KalantariniaKBelcikJTPatrieJTWeiKReal-time measurement of renal blood flow in healthy subjects using contrast-enhanced ultrasoundAm J Physiol Renal Physiol2009297F1129F113419625375
- TaniyamaYTachibanaKHiraokaKLocal delivery of plasmid DNA into rat carotid artery using ultrasoundCirculation20021051233123911889019
- MannarisCAverkiouMAInvestigation of microbubble response to long pulses used in ultrasound-enhanced drug deliveryUltrasound Med Biol20123868169122341047
- YehCKSuSYEffects of acoustic insonation parameters on ultrasound contrast agent destructionUltrasound Med Biol2008341281129118343019
- XuZFowlkesJBCainCAA new strategy to enhance cavitational tissue erosion using a high-intensity, initiating sequenceIEEE Trans Ultrason Ferroelectr Freq Control2006531412142416921893
- ZhuCHeSShanMChenJStudy of a peak in cavitation activity from HIFU exposures using TA fluorescenceUltrasonics200644Suppl 1e349e35116945397
- ChenHLiXWanMThe inception of cavitation bubble clouds induced by high-intensity focused ultrasoundUltrasonics200644Suppl 1e427e42916782158
- ChenWSBraymanAAMatulaTJCrumLAInertial cavitation dose and hemolysis produced in vitro with or without OptisonUltrasound Med Biol20032972573712754072
- TuJMatulaTJBraymanAACrumLAInertial cavitation dose produced in ex vivo rabbit ear arteries with Optison by 1-MHz pulsed ultrasoundUltrasound Med Biol20063228128816464673
- ChenWSMatulaTJBraymanAACrumLAA comparison of the fragmentation thresholds and inertial cavitation doses of different ultrasound contrast agentsJ Acoust Soc Am200311364365112558300
- McDannoldNVykhodtsevaNHynynenKUse of ultrasound pulses combined with definity for targeted blood–brain barrier disruption: a feasibility studyUltrasound Med Biol20073358459017337109
- Duvshani-EshetMMachlufMTherapeutic ultrasound optimization for gene delivery: a key factor achieving nuclear DNA localizationJ Control Release200510851352816243409
- MillerMWEverbachECMillerWMBattagliaLFBiological and environmental factors affecting ultrasound-induced hemolysis in vitro: 2. Medium dissolved gas (pO2) contentUltrasound Med Biol2003299310212604120
- BrotchieAStathamTZhouMDharmarathneLGrieserFAshokkumarMAcoustic bubble sizes, coalescence, and sonochemical activity in aqueous electrolyte solutions saturated with different gasesLangmuir201026126901269520593787
- ChappellJCSongJBurkeCWKlibanovALPriceRJTargeted delivery of nanoparticles bearing fibroblast growth factor-2 by ultrasonic microbubble destruction for therapeutic arteriogenesisSmall200841769177718720443
- HynynenKClementGMcDannoldNVykhodtsevaNThe feasibility of noninvasive image-guided treatments of brain disodereds by focused ultrasoundJ Acoust Soc Am20041152446
- FurusawaYFujiwaraYHassanMAInhibition of DNA-dependent protein kinase promotes ultrasound-induced cell death including apoptosis in human leukemia cellsCancer Lett201232210711222366497
- FurusawaYFujiwaraYCampbellPDNA double-strand breaks induced by cavitational mechanical effects of ultrasound in cancer cell linesPLoS One20127e2901222235259
- LammersTAimeSHenninkWEStormGKiesslingFTheranostic nanomedicineAcc Chem Res2011441029103821545096
- LiuZLammersTEhlingJIron oxide nanoparticle-containing microbubble composites as contrast agents for MR and ultrasound dual-modality imagingBiomaterials2011326155616321632103
- LimYTChoMYKangJHPerfluorodecalin/[InGaP/ZnS quantum dots] nanoemulsions as 19F MR/optical imaging nanoprobes for the labeling of phagocytic and nonphagocytic immune cellsBiomaterials2010314964497120346494
- PochonSTardyIBussatPBR55: a lipopeptide-based VEGFR2-targeted ultrasound contrast agent for molecular imaging of angiogenesisInvest Radiol201045899520027118
- SundaramJMelleinBRMitragotriSAn experimental and theoretical analysis of ultrasound-induced permeabilization of cell membranesBiophys J2003843087310112719239
- SchlicherRKHutchesonJDRadhakrishnaHApkarianRPPrausnitzMRChanges in cell morphology due to plasma membrane wounding by acoustic cavitationUltrasound Med Biol20103667769220350691
- van WamelAKooimanKEmmerMten CateFJVersluisMde JongNUltrasound microbubble induced endothelial cell permeabilityJ Control Release2006116e100e10217718938
- YudinaALepetit-CoiffeMMoonenCTEvaluation of the temporal window for drug delivery following ultrasound-mediated membrane permeability enhancementMol Imaging Biol20111323924920521134
- YudinaAde SmetMLepetit-CoifféMUltrasound-mediated intracellular drug delivery using microbubbles and temperature-sensitive liposomesJ Control Release201115544244821689699
- MaxwellADCainCADuryeaAPYuanLGurmHSXuZNoninvasive thrombolysis using pulsed ultrasound cavitation therapy – histotripsyUltrasound Med Biol2009351982199419854563
- YoshizawaSIkedaTItoAOtaRTakagiSMatsumotoYHigh intensity focused ultrasound lithotripsy with cavitating microbubblesMed Biol Eng Comput20094785186019360448
- GourevichDGeroldBArdittiFUltrasound activated nano-encapsulated targeted drug delivery and tumour cell porationAdv Exp Med Biol201273313514422101719
- AskariATUnzekSPopovicZBEffect of stromal-cell-derived factor 1 on stem-cell homing and tissue regeneration in ischaemic cardiomyopathyLancet200336269770312957092
- GhanemASteingenCBrenigFFocused ultrasound-induced stimulation of microbubbles augments site-targeted engraftment of mesenchymal stem cells after acute myocardial infarctionJ Mol Cell Cardiol20094741141819540842
- ZhongSShuSWangZEnhanced homing of mesenchymal stem cells to the ischemic myocardium by ultrasound-targeted microbubble destructionUltrasonics20125228128621937069
- PintonGAubryJFFinkMTanterMNumerical prediction of frequency dependent 3D maps of mechanical index thresholds in ultrasonic brain therapyMed Phys20123945546722225316
- TezelAMitragotriSInteractions of inertial cavitation bubbles with stratum corneum lipid bilayers during low-frequency sonophoresisBiophys J2003853502351214645045
- SharmaDHaneshMYahyaAMohamedMPhonophoresis with diclofenac versus ketoprofen for knee joint injuriesMiddle East J Int Med20092912
- MeshaliMAbdel-AleemHSakrFNazzalSEl-MalahYEffect of gel composition and phonophoresis on the transdermal delivery of ibuprofen: in vitro and in vivo evaluationPharm Dev Technol2011169310120100032
- KayaKDelialougluSBabadagMCombined physiotherapy in patients with arthrogenous pain of temporomandibular jointJ Phys Med Rehab Sci201013614
- YangJHKimTYLeeJHYoonSWYangKHShinSCAntihyperalgesic and anti-inflammatory effects of ketorolac tromethamine gel using pulsed ultrasound in inflamed ratsArch Pharm Res20083151151718449510
- MeshaliMAbdel-AleemHSakrFNazzalSEl-MalahYIn vitro phonophoresis: effect of ultrasound intensity and mode at high frequency on NSAIDs transport across cellulose and rabbit skin membranesPharmazie200863495318271303
- RainsfordKDProfile and mechanisms of gastrointestinal and other side effects of nonsteroidal anti-inflammatory drugs (NSAIDs)Am J Med19991072735