Abstract
Over the past two decades, many types of natural and synthetic polymer-based micro- and nanocarriers, with exciting properties and applications, have been developed for application in various types of tissue regeneration, including bone, cartilage, nerve, blood vessels, and skin. The development of suitable polymers scaffold designs to aid the repair of specific cell types have created diverse and important potentials in tissue restoration. Fibrinogen (Fbg)- and fibrin (Fbn)-based micro- and nanostructures can provide suitable natural matrix environments. Since these primary materials are abundantly available in blood as the main coagulation proteins, they can easily interact with damaged tissues and cells through native biochemical interactions. Fbg- and Fbn-based micro and nanostructures can also be consecutively furnished/or encapsulated and specifically delivered, with multiple growth factors, proteins, and stem cells, in structures designed to aid in specific phases of the tissue regeneration process. The present review has been carried out to demonstrate the progress made with micro and nanoscaffold applications and features a number of applications of Fbg- and Fbn-based carriers in the field of biomaterials, including the delivery of drugs, active biomolecules, cells, and genes, that have been effectively used in tissue engineering and regenerative medicine.
Introduction
Tissue engineering is a multidisciplinary area that encompasses biology, chemical engineering, material science, and chemistry. Existing techniques have the potential to create specific tissues and organs in vitro and in vivo. For fabrication of biomimetic scaffolds, a range of synthetic and protein-based polymer scaffolds, such as collagen, gelatin, elastin, proteoglycan, hyaluronan, laminin, silk fibroin, soybean, fibrinogen (Fbg), and fibrin (Fbn) have been widely used. Among these polymers, Fbg- and Fbn-based scaffolds have been widely used to construct functional substitutes (tissues and organs), which may provide a permanent solution to damaged tissue and organs without need for additional therapies, due to their nonimmunogenicity (autograft) and their ability to mimic the organization of native tissue structures. Fbg is a 340 kDa blood protein involved in blood coagulation and platelet aggregation (thrombogenesis). When Fbg and thrombin solutions are mixed together in the presence of Ca2+ forms Fbn through polymerization, it can be digested by the proteolytic enzyme (plasmin) through fibrinolysis.Citation1 Similar to collagen, Fbg and Fbn scaffolds can attain a high cell seeding efficiency and uniform cell distribution and can then proliferate, migrate, and differentiate into specific tissues/organs by secreting extracellular matrix (ECM) (which is involved in the generation of tissue).Citation2 Fbg has major healing advantages, as it provides a favorable surface for cellular attachment and proliferation – a three-dimensional (3-D) fibrous structural support and nanotextured surfaces, which consist of a fibrous network for cell signaling, and cell-matrix and cell-cell interactions.Citation3 Especially in tissue engineering, Fbg- and Fbn-based scaffolds induce ECM production that renders support to connective tissues, such as cartilage, ligament, bones, tendons, nerves, blood vessels, and skin.Citation4–Citation8
Fbg and Fbn can be easily formulated into diverse structural scaffolds because of their micro- and nanocharacteristic; micro/nanoporous scaffolds, such as microspheres,Citation9 microfibers,Citation10 microtubes,Citation11 nanoparticles,Citation12 nanofibers,Citation13 and hydrogels,Citation14 have been formulated for diverse tissue engineering applications. In a vast range of severe pathological conditions, such as chronic wounds, diabetic ulcer, and ischemic heart disease, the rate of reepithelialization and neovascularization can predict the therapeutic approach towards perfusion and healing of damaged tissue.Citation15–Citation17 Since Fbg and Fbn scaffolds are considered to be natural provisional ECM, they also play a significant role in the early stages of hemostasis and wound repair, in addition to blood clotting. Furthermore, Fbg contains two Arginine-Glycine-Aspartate (RGD) integrin-binding sites, which usually bind endothelial and fibroblast cells.Citation18 Fbg also has a high affinity towards biomolecules, such as vascular endothelial growth factor (VEGF), fibroblast growth factor (FGF), and many other tissue-inductive cytokines.Citation5,Citation19
Current 3-D scaffolds may incorporate different structural features, such as micro/nanoporous matrices or composites, micro/nanofibers with porous characteristics, micro/nanospheres or particles, and micro/nanofiber composites within porous scaffold. Micro/nanospheres or particles within a porous scaffold (shown in ) have attracted special interest for potential tissue engineering and regenerative applications. In many cases, growth factors/cells/deoxyribonucleic acid (DNA) can be integrated into Fbg and Fbn scaffolds by bulk encapsulation, specific/nonspecific physical adsorption, surface fictionalization, or by the use of protein or cell adhesive binders, as illustrated in . Micro/nanocarriers or composites encapsulated with growth factors and/or drugs can be delivered to a targeted site to induce/mimic ECM productions, where tissues need to be regenerated. For specific tissue engineering applications, cell proliferation and differentiation towards specific phenotype is very favorable. To attain this successfully, in situ and in vivo sustained delivery with various drug and growth factors within Fbg and Fbn micro/nano scaffolds have been studied.Citation20,Citation21 Further, these scaffolds loaded with growth factors, such as VEGF,Citation22 epidermal growth factor (EGF),Citation23 nerve growth factor (NGF),Citation24 and bone morphogenic protein (BMP)Citation25 were shown to stimulate blood vessel, skin, nerve and bone growth, respectively. Such scaffolds have also been extensively studied with various tissue inductive growth factors, such as transforming growth factor (TGF),Citation26 FGF,Citation27 fibronectin (FN),Citation28 keratinocytes,Citation29 etc, for the growth of various tissues. Furthermore, the controlled release of these growth factors from Fbg and Fbn scaffolds provides a number of vital advantages, as growth factors transported into the target environment may locally govern multiple processes of the cell, such as attachment, proliferation, differentiation, and morphogenesis.
Figure 1 Polymer composite scaffolds prepared with incorporation of micro/nanospheres or micro/nanofibers.
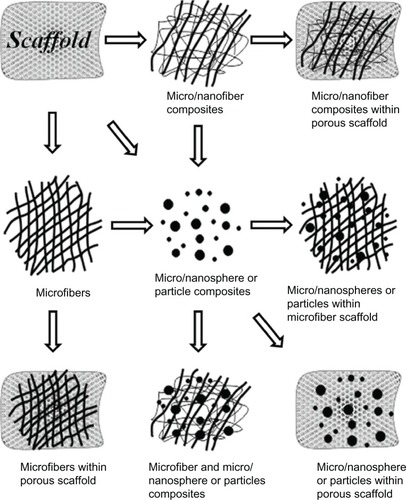
Figure 2 Incorporation of cells/DNA/growth factors into the scaffold matrix.
Abbreviation: DNA, deoxyribonucleic acid.
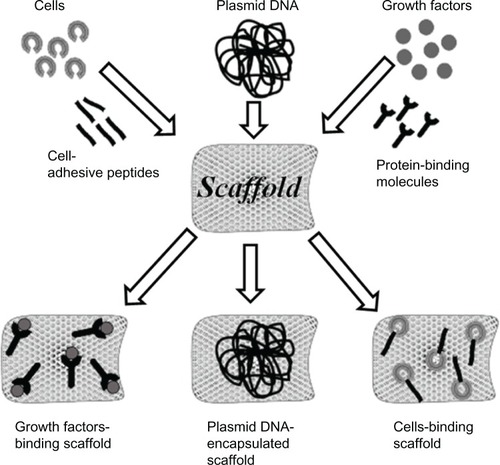
The development of techniques for the regeneration of different tissues (skin, nerve, vascular, and cartilage) is not only based on the delivery of corresponding growth factors, but is also dependent upon the polymer carrier and types of cell interactions. For example, alginate,Citation30 dextran,Citation31 hyaluronan,Citation32 heparin,Citation32 polyvinyl alcohol,Citation33 and poly lactic-co-glycolic acid (PLGA)Citation34 scaffolds have all been used for vascular repair/regeneration, wound healing, and other tissue engineering applications. However, these polymeric materials have their own disadvantages, such as rapid degradation, denaturation of growth factors due to irreversible complexation, lack of cell proliferation, and production of acidic environments in the ischemic wound area, which could reduce protein moiety functions, as well as cause potential immune responses and acute inflammation.Citation35
The material used for fabricating an ideal scaffold must meet a number of basic criteria, such as providing an appropriate environment for enabling cellular function, cell migration, proliferation, and differentiation, and also must allow for tissue regeneration/development. Most importantly, the scaffold should not evoke any immune reactions, while providing a feasible model for cellular interaction/proliferation. Moreover, scaffolds should mimic both the fibrallar form and complex biochemical role of native ECM.Citation3 In this review, we highlight the methods for fabricating Fbg- and Fbn-based micro/nanocarriers, including the use of hydrogel and Fbg/Fbn blended natural and synthetic matrices that are used for biomedical applications like drug release, wound dressing, and in tissue engineering and cancer therapy.
Herein we present a detailed discussion of the theory behind Fbg and Fbn use and of their use as micro/nanoscaffolds, integrated with biomolecules and cells, in tissue engineering applications; their applications for specific tissues, such as vascular maladies, bone and cartilage, skin constructs, and nerve conduits, will also be discussed in detail.
Preparation of Fbg- and Fbn-based carriers and their biomedical applications
Different fabrication methods have been used to prepare a diversity of Fbg and Fbn scaffolds, such as microspheres, microbeads, microfibers, nanoparticles, nanofibers, and hydrogels. These scaffolds have been designed and optimized with desired properties, such as size, shape, and porosity with interconnected structures that are suitable for culturing preferred types of cells. Most of the scaffolds used in tissue engineering applications have either a uniform size or shape, and random pore structures with interconnected networks. These pore structures can aid cell attachments, cell–matrix interactions, and nutrient/waste metabolite transport. For most biological applications, such pore structures/surfaces are required for the sustained release of loaded drug/biomolecules, to maintain bioavailability or specific tissue regeneration. In many cases, Fbg and Fbn scaffolds have an amorphous nature, which can improve the drug-loading efficacy and mechanical performances of the scaffolds. The following sections describe the various types of micro- and nanocarrier preparations and their applications.
Fbg and Fbn microspheres/beads and nanoparticles as scaffolds
Fbg- and Fbn-based microspheres/beads and nanoparticles are widely used in a diverse range of pharmaceutical and biomedical applications, such as drug delivery, protein/peptide delivery, chemotherapy, and wound dressings and hemostatic bandages. Miyazaki et al were the first to report the fabrication of Fbg microspheres using a water and oil (W/O) emulsification method.Citation36 The prepared microspheres were then applied as a novel drug carrier for anticancer drug delivery. A few years later, Fbn microbeads were prepared by mixing both Fbg and coagulation factors (thrombin and calcium ions); additionally, glutaraldehyde was added to this system to minimize the coagulation of Fbn beads. The beads were then successfully used for various macromolecular delivery systems (insulin, lysozyme, ovalbumin, and bovine serum albumin).Citation37 In another study, Fbg-coated albumin microcapsules were administered via intravenous administration, to reduce bleeding in animals and to reduce bleeding time in surgical wounds in muscles and abdominal skin.Citation38 Generally, microspheres contain pore structures with interconnected networks, which assist in cell infiltration and in cell–matrix interaction. Recently, our group prepared Fbg microspheres (100–150 μm) with pore structures using a W/O emulsification solvent extraction method, for use in various biomedical applications. These are shown in . Using this method, the spheres size and uniformity can be controlled with the Fbg solution concentrations (wt%), flow rate, and the stirrer rotating speed.Citation9 Using the same method, Fbg nanospheres were also developed by further optimization of the above parameters, as shown in . However, since the data is not yet published, the preparation method is not described here. lists methods for fabricating Fbg and Fbn micro- and nanoscale scaffolds, which have been used for various pharmaceutical applications.
Table 1 Microcarrier fabrication methods using Fbg and Fbn and their biomedical applications
Figure 3 Microscopic images of Fbg microspheres.
Notes: (A) Light microscope image shows the microsphere sphericity and approximately uniform sizes, between 100–150 μm; (B) SEM image further confirmed sphericity; (C) Magnified image of (B), reveals the porous structures. Copyright © 2011, Springer Science+Business Media. Reproduced with permission from Rajangam T, Paik HJ, An SSA. Development of fibrinogen microspheres as a biodegradable carrier for tissue engineering. BioChip J. 2011;5(2):175–183.Citation9
Abbreviations: Fbg, fibrinogen; SEM, scanning electron microscope.
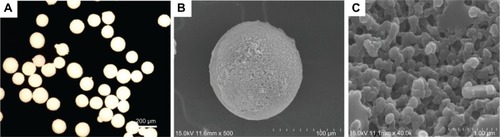
Fbg and Fbn micro/nanofiber as scaffolds
In tissue engineering, 3-D fibrous scaffolds with a large surface area can generally support high cell density and long-term cultures, and provide a better link between single cells and organs than do conventional 2-D cultures. There is a list of methods available for the fabrication of polymer micro and nanofibrous scaffolds with 3-D structures: electrospinning, self-assembly, phase separation, 3-D printing, and fiber bonding. Each method has its own advantages and disadvantages, although the choice of method is also based on the polymer characteristics.
To fabricate 3-D Fbg fibers, electrospinning methods have been preferred due to their practicality and flexibility. Wnek et al were the first to report the fabrication of Fbg nanofiber using an electrospinning method: First, an Fbg suspension was prepared with 1,1,1,3,3,3-hexafluoro-2-propanol (HFP) and minimal essential medium (9:1); the mixture was left overnight and then, the homogenous solution was electrospun with a high-voltage supply (22 kV).Citation13 The electrospun nanofiber showed an average size of 80–700 nm diameter, and the 3-D fiber was effectively used as a wound dressing and hemostatic bandage. McManus et al also prepared a Fbg nanofiber, using the same method and suspending agents, and in order to further evaluate the fiber surface porosity, nanofibers with two different Fbg concentrations were fabricated.Citation43 The nanofiber produced with the lower Fbg concentration (110 mg/mL) showed a higher average porosity value (59%) than did the high-concentration Fbg (130 mg/mL) nanofiber (54%), due to the greater degree of solvent evaporation. However, both spun fibrous mats could act as a biomimetic scaffold and were deemed to be especially appropriate for use as wound dressings. The same research group, in other studies, assessed the cellular interaction and scaffold remodeling with Fbg nanofibers, using neonatal rat cardiac fibroblast cells; in these, the postculture mat histology evidenced that the Fbg scaffold was easily remodeled by the deposition of native collagen.Citation44 Electrospinning-prepared Fbg nanofiber was also used to evaluate in vitro cell migration on the scaffold surface.Citation45 Carlisle et al analyzed the mechanical properties of a single electrospun Fbg nanofiber, using a combined atomic force/fluorescence microscopic technique, and the result indicated that Fbg nanofiber had a high extensibility.Citation46 High-extensibility fiber scaffolds have been found to be more widely applicable for in vitro and in vivo tissue engineering due to their flexibility. Electrospun Fbg nanofibers that interacted with human bladder smooth muscle cells showed rapid proliferation and demodulation as well as relatively slow rates of degradation.Citation47 In other studies, McManus et al reported the fabrication of Fbg-polydioxanone composite nanofibers with high mechanical strength for potential in situ urologic tissue development.Citation48 In electrospinning, the fiber size can be controlled by controlling the voltage and the distance between the needle and the collecting plate.
Usually, 3-D fiber scaffolds of the micron size range have greater mechanical strength, a property that could enhance cell adhesion, proliferation, and ease of handling during in vitro postmodification or implantation over the nanofiber scaffolds. On the other hand, they lack nanotextured topographical features, which are needed for the scaffold to mimic like native ECM.Citation49 Further, successes in directing cells towards certain cellular morphologies, functions, and differentiations have been studied with diverse types of nanofibers.Citation49,Citation50 Recent studies of tissue engineering have paid great attention to combining these two scaffold features towards the development of ideal scaffold fabrication. Recently, we developed nanofiber-aligned Fbg microfibers, which showed a higher rate of biomolecule encapsulation and proliferation than other highly porous and less pores Fbg microfibers.Citation51 The structurally different Fbg microfibers were prepared by a simple tubular gel-solvent extraction method without high-power voltage or high pressure (). Here, the Fbg suspension was prepared using aqueous phosphate-buffered saline. In our laboratory, we also prepared a highly porous Fbg sheet composed of micro- and nanostructures, using a freeze-drying method (). However, since the data has not yet been published, the preparation method has not been described.
Figure 5 SEM images of Fbg microfibers fabricated from three different concentrations of Fbg.
Notes: Decreasing fiber diameter was observed with decreasing Fbg concentration. Images (A–C) show 200 μm, 150 μm, and 75 μm fibers, respectively, which were made from 15, 10, and 5 wt% Fbg, respectively. Images (D–F) represent highly aligned nanostructures, highly interporous fibers with aggregated structures, and less porous fibers with unaligned structure, respectively, and are the same as the lower magnification images in (A–C), respectively (inlet images scale bar is 1 μm). Copyright © 2012, Dove Medical Press Ltd. Reproduced with permission from Rajangam T, An SS. Improved fibronectin-immobilized fibrinogen microthreads for the attachment and proliferation of fibroblasts. Int J Nanomedicine. 2013;8:1037–1049.Citation51
Abbreviations: Fbg, fibrinogen; SEM, scanning electron microscope.
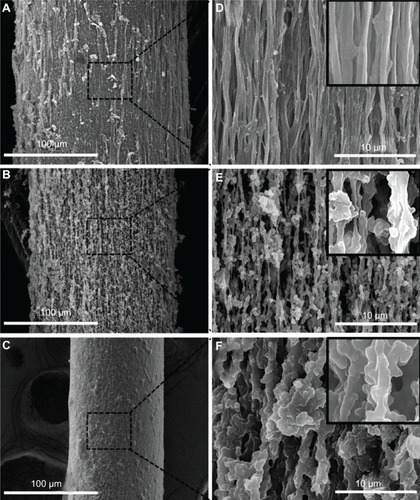
Fbg and Fbn hydrogel as scaffolds
Fbn hydrogels have been most widely used as scaffolds for the reconstruction of different tissue and have been used as a biological adhesive for various procedures, such as abdominal, vascular oral, thoracic, and endoscopic surgeries.Citation52–Citation54 Mostly, Fbn hydrogels have been prepared by mixing Fbg with thrombin solution in the presence of Ca2+. Thrombin can cleave peptide fragments from soluble Fbg, thereby yielding insoluble Fbn peptides that aggregate to form fibril hydrogel networks.Citation14 The characteristics of the Fbn fibril network formation were mainly based on the thrombin concentrations. For example, an increased thrombin concentration was found to be associated with shorter gelation time, the formation of more fiber bundles with thinner fibers size, and with low porosity. On other hand, lower thrombin concentrations were found to have longer gelation time, fewer fiber bundles with thicker fibers size, and more porous structures.Citation55–Citation57 In many cases, the constructed hydrogels have lacked mechanical strength, and to overcome this problem, the hydrogels were blended with biocompatible, biodegradable synthetic polymersCitation58 or were seeded with cells either by static or dynamic methods.Citation59
Rowe et al proposed a tubular, 3-D smooth muscle cell–seeded Fbn hydrogel construct for vascular tissue engineering. Here, the cells were directly mixed with the Fbg solution, were injected into an annular mold, and were then polymerized by the addition of thrombin. Further evaluation in this study concluded that the concentration of thrombin plays major roles in the outcome, influencing mechanical properties, fibril morphology, and cellular behavior.Citation14 In another study, Almany and Seliktar developed unique Fbg-based biosynthetic hybrid hydrogels. The Fbg fragments were cross-linked with a dysfunctional polyethylene glycol (PEG) side chain to make a PEG-Fbg hybrid hydrogel. The developed hydrogel had both good cell adhesion and mechanical properties because Fbg offers cellular adhesive domains, while PEG provides mechanical and structural properties.Citation60 PLGA microsphere–immobilized Fbn hydrogel provides an improved elastic modulus for chondrocyte proliferation and ECM production.Citation61 Fbn hydrogel containing BMP-2 loaded poly(l-lysine) nanoparticles and human mesenchymal stem cells (MSCs) evidenced that the Fbn constructs significantly improved the proliferation of human MSCs in bone regeneration. The study also suggested that this might be one of the most promising methods for enhancing bone regeneration.Citation6 Recently, Lei et al developed a novel Fbn-based hydrogel to deliver concentrated and nonaggregated nonviral genes, demonstrated by in vivo study.Citation62 The hybrid hydrogel, made with Pluronic F127-Fbg, showed superior physical properties for in vitro cell culture. Moreover, the hybrid hydrogel retained Fbg biocompatibility with added mechanical strength, and at the same time, Pluronic F127 did not lose its unique self-assembly properties.Citation63 Fbg- and Fbn-based hydrogels have remarkable merit over other types of natural polymer hydrogels (gelatin and collagen) in that they can be made available autologously, thus avoiding viral infection transmission and the potential risks of foreign body reaction.Citation61 Fbn hydrogels seeded with smooth muscle cells,Citation64 skeletal muscle cells,Citation65 and chondrocyte cellsCitation66 have been used to produce engineered tissues with a high degree of mechanical strength.
Fbg and Fbn tubes as vascular graft
Our laboratory constructed small-diameter Fbg grafts as biodegradable and biocompatible scaffolds for use in microvascular tissue engineering, using a tubular gelsolvent extraction method. The tube showed a rough outer surface and smooth inner surface. The measured inner and outer diameters of the Fbg microtube were approximately 750–850 μm and 950–1050 μm, respectively () (data unpublished).
Figure 7 SEM images of a Fbg microtube. (A) The outer morphology of the tube shows the rough surfaces; (B) Magnified image of microtube shown in (A); (C) The tube’s inner surface was revealed to be much smoother than the outer surface; (D) The cross-sectional SEM image reveals the round shape, with approximately uniform wall thickness. (data unpublished).
Abbreviations: Fbg, fibrinogen; SEM, scanning electron microscope.
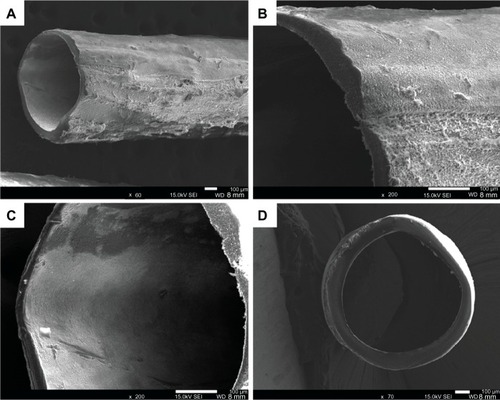
Fbg/Fbn–synthetic polymer composites as micro/nano scaffolds
Synthetic polymersCitation67 and copolymersCitation68 have been frequently used in the field of tissue engineering, as they have ability to precisely control the mechanical strength of the matrices, but often, these polymers provide inadequate cell regulation for specific cellular events. Fbg and Fbn have been widely mixed/blended with other natural/synthetic polymers to fabricate different composite biomaterials for vascular regeneration, bone regeneration, chronic wound healing, osteogenesis, and reepithelialization.Citation69–Citation71 In addition, biocomposite materials acquire adequate mechanical strength, enhanced cell adhesion, proliferation, and ECM production as well as the potential regulation of other cellular process activities for specific cellular events.
PEG-Fbg composites furnish attachment motifs for smooth muscle and endothelial cells adhesion; these cells have demonstrated their capability to pass through scaffolds to carry out their respective functions.Citation60 The PLGA microspheres immobilized with Fbg matrix have shown enhanced chondrocyte proliferation and ECM production.Citation61 PEGylated albumin combined with PEGylated Fbg hydrogel was shown to reveal comprehensive drug release and degradation properties.Citation72 Macro/microporous biphasic calcium phosphate granules combined with Fbn glue were shown to provide a moldable and self-hardening biocomposite, which has been used in types of orthopedic and maxillofacial applications, due to their osteoconductive properties.Citation73 Fbn-PLGA composites were shown to encourage early chondrogenesis in rabbit articular cartilage, and this indicates that Fbn-PLGA might act as a possible cell delivery vehicle to promote articular cartilage development.Citation58 Frisman et al reported a method for fabricating nanostructured biocompatible and biosynthetic polymeric hydrogel by cross-linking PEG and Fbg. The constructed 3-D hydrogel provided enhanced cellular behavior and superior mechanical strength.Citation74 Polycaprolactone valves coated with Fbn demonstrated proper opening and closing dynamics in cardiac tissue engineering, with good biocompatibility,Citation75 and in a further study, Fbn-polyurethane microporous hydrogel cultured with osteogenic cells exhibited promising results in articular tissue development in vivo.Citation76
The delivery of genetic material and DNA by polymer-based hydrogels would add to the applications in the field of gene therapy and cancer therapy.Citation77 However, the delivery of DNA through a synthetic cationic polymer (“polyplexes”) hydrogel has not previously succeeded because of high aggregation and inactivation of the cationic polymer inside the hydrogel matrix.Citation78 But, the same polymer hydrogel when functionalized with Fbg, could be effectively used to deliver concentrated DNA without aggregation.Citation62 Osathanon et al reported a novel method of constructing a calcium phosphate–Fbn hydroxyapatite-based composite scaffold, which revealed a tightly interconnected network and a more porous structure, and the biodegradable composite supported primary osteoblast-like cell adhesion, proliferation, and differentiation in bone tissue engineering, in vitro and in vivo.Citation79 Fbg and Fbn also have been combined with different types of synthetic polymers, such as polydioxanone,Citation48 poly(ε-caprolactone),Citation80 etc. Other composite materials that have been used in similar applications are listed in .
Table 2 Delivery systems for different biomolecules and cell types using Fbg/Fbn composites, and their tissue engineering applications
Fbg/Fbn–natural polymer composites as micro/nano scaffolds
These combination natural polymer matrix–based cell delivery systems have become a promising approach in the development of many current cell therapies, as such systems exhibit synergistic effects on cellular interaction, promoting integration into host tissue, as well as offering variable material properties with good degradation kinetics.Citation81 In this section, we discuss Fbg/Fbn-natural polymer composites and their application in tissue regeneration therapies. In one study, Fbn-gelatin composite film was shown to exhibit a high equilibrium content of water, which could effectively improve the efficacy and properties of the matrix when applied as a wound dressing.Citation82 In other studies, a physiologically coated Fbn-chitosan gelatin composite film was shown to provide a fibrous and porous structure with a high water absorption capacity, which could improve the encapsulation efficacy of hydrophilic protein and peptides.Citation83 Hepatic cell-seeded Fbg-gelatin matrix stabilized by thrombin exhibited a very high compressive strength. The study also suggested that Fbn could be a favorable biological component for cell assembly/delivery compared with other natural protein-based scaffolds.Citation84 Fbg-and thrombin-coated collagen patches were used as a topical hemostatic agent and were applied for rapid hemostasis and wound closure after procedures such as liver, thoracic, neuro-, kidney, spleen, plastic, and minimally invasive surgery, in vivoCitation85. By combining collagen, chitosan, and Fbn, Han et al prepared novel asymmetric porous scaffolds.Citation86 In other studies, Lorusso et al reported that Fbg/thrombin-coated collagen patches were effectively used for the control of severe intraoperative pulmonary hemorrhage and air leakage after correction of a ruptured thoracic aortic aneurysm.Citation87 Recently, a Fbn-chitosan composite loaded with human fibroblasts showed good cells spreading effect and ECM interaction.Citation88 Both Fbn-and collagen-based vascular grafts could be used as substitutes for bypass grafts or as models for vascular studies.Citation89 Very recently, Zhou et al successfully demonstrated the controlled delivery of recombinant human epidermal growth factor (rhEGF) using a Fbn-chitosan composite – the composite achieved a high protein loading efficacy due to the presence of chitosan and a lower initial burst release due to the Fbn.Citation90 Fbg and Fbn have also been blended or coated with different natural polymers, such as poly(L-lactide),Citation61 silk fibroin,Citation91 alginate,Citation92 etc. Other such composites are listed in .
Cross-linking of Fbg and Fbn micro/nanoscaffolds
In tissue engineering and regenerative medicine, shape stability is a significant factor for specific medical applications, such as those relating to bone, blood vessel, and nerve regeneration.Citation11,Citation96 Fbg micro/nanoscaffolds can lose their structure within a few days through degradation by various protease enzymes, such as plasmin and trypsin, in vitro and in vivo.Citation97,Citation98 For complete regeneration of various body tissues, including cartilage and bone, scaffolds should maintain their size and shape in the long term in order to deliver comprehensive mechanical strength. In order to achieve such strength, Fbg and Fbn scaffolds have been cross-linked with different chemical and biological agents.
In 1963, Mihalyi was the first to describe a cross-linking procedure for bovine Fbg, using formaldehyde.Citation99 A few years later, in 1975, human Fbg cross-linking was introduced by Furlan and Beck, using glutaraldehyde and tetranitromethane. They further confirmed the participation of different chains (α, β, and γ) in intermolecular cross-linking, by performing sodium dodecyl sulfate-polyacrylamide gel electrophoresis (SDS-PAGE), the results of which evidenced that enhanced intermolecular cross-linking occurred when high concentrations of glutaraldehyde were used.Citation100 Fbn clots are naturally cross-linked through the inclusion of activated transglutaminase (factor XIII), which forms covalent cross-linking between glutamyl and lysyl residues of adjacent Fbn molecules,Citation45 but factor XIII is comparatively expensive. On the other hand, glutaraldehyde is commonly used as a cross-linking agent for natural proteins and is currently used to hasten tissue regeneration by fixing collagenous tissue.Citation101,Citation102 Glutaraldehyde is also reasonably inexpensive and has the ability to be cross-linked rapidly over large distances, with a broad range of amino groups.Citation103 However, aldehydes are non-zero-length cross-linkers that can induce poly- or bifunctional cross-links into the protein structure during cross-linking. Moreover, aldehydes could leach toxic substance after carrier degradation, thereby presenting an increased risk of cytotoxicity and material calcification.Citation101,Citation102 To compare cellular toxicity and other physical properties, Sell et al cross-linked bovine Fbg scaffolds using two different cross-linkers, namely glutaraldehyde vapor and 1-ethyl-3-(3-dimethylaminopropyl) carbodiimide hydrochloride) (EDC).Citation45 The EDC cross-linked scaffolds showed considerably increased peak stress modulus values and less toxicity with significant sustained degradation, when compared with glutaraldehyde cross-linked scaffolds, in vitro. Dare et al cross-linked Fbn hydrogels incorporated with human articular chondrocytes using naturally-occurring genipin, and the result demonstrated a superior mechanical strength, with excellent gene expression, ECM production, and a slower rate of degradation. Moreover, genipin cross-linked-Fbn hydrogel did not noticeably affect cell viability and importantly, increased the shear moduli and dynamic compression of the hydrogel.Citation104 Recently, Fbg nanoparticles were cross-linked with calcium chloride and were shown to have potential as a promising therapeutic carrier for sustained anticancer drug release in vitro.Citation12
EDC is a water soluble and zero-length cross-linker. Most of all, EDC cannot be incorporated into protein molecules, as it can be simply washed away as a water soluble urea derivative, thereby decreasing the potential for cytotoxic effects.Citation101,Citation102 Fbg microspheres that were cross-linked with various concentrations of EDC (1, 3, 5 and 10 mM) revealed a slower rate of degradation with increasing EDC concentrations.Citation9 Compared with this, nanostructure aligned and highly porous Fbg microfibers that were cross-linked with EDC showed slower fibrinolytic degradation. Thus, increased Fbg cross-linking is not only based on the concentration of cross-linkers, but also depends on scaffold structural characteristics. For example, Fbg scaffolds made with nanostructures attained higher cross-linking compared with microstructures because of their higher exposure of Arginine and Lysine residues, which are abundantly present on the Fbg surface. To minimize or reduce the proteolytic degradation of Fbn, researchers have commonly used Fbn degradation inhibitors and their stabilizers, such as factor XIII, aprotinin, and e-amino-n-caproic acids,Citation59,Citation65,Citation93,Citation105 along with sufficient amounts of thrombin and calcium, which all prevent excessive degradation of Fbn.Citation106
Tissue plasminogen activator can activate plasminogen to active plasmin (serine protease), which in turn, degrades Fbg and Fbn by cleaving the carboxyl terminal peptide bond of the Arginine or Lysine residues; therefore, for long-term degradation there needs to be an increase in the number of peptide bonds with suitable cross-linking agents.Citation99,Citation101,Citation102 Various studies have disclosed the potential of Fbg and Fbn scaffolds for cell viability, gene expression, and ECM production. However, to obtain better tissue engineering results, scaffolds should maintain their size and shape for prolonged periods, and to achieve that, it is necessary that they be stabilized with an appropriate cross-linking agent. Furthermore, the rate of degradation can be controlled by either increasing the concentration of cross-linking agents or by producing a nanostructured scaffold.
Cancer therapy
Cancer remains an incurable disease, and there are more than 10 million new patients identified every year. On the other hand, due to the development of new technology and surgical intervention (radiation and chemotherapy), the death rate related to cancer has been declining for the past few years. However, chemotherapeutics kill normal cells and cause a variety of toxicities to patients. To minimize this, many research groups have focused their research towards biodegradable polymer carrier (micro/nano) drug delivery systems, and many have attained satisfactory results.Citation107 Among the biodegradable polymer carriers, Fbg-based micro/nano carriers are widely used in cancer treatment. Fbg and Fbn can inhibit the progression of cancer cell growth by countering excessive coagulation in and around tumor tissues.Citation108 Because of this, Fbg-based micro- or nanocarriers are widely used in cancer treatment.
The anticancer drug-loaded Fbg micro/nano carriers have shown promising effects on various cancer cells. In one study, water soluble, 5-fluorouracil-loaded Fbg microspheres were effectively used as a novel injectable drug delivery system for anticancer treatment and expressed sustained release for more than 5 days, as demonstrated by in vitro experiment.Citation39 The same research group demonstrated doxorubicin release in mice, and positive results were observed on the basis of animal survival data. The doxorubicin solution–injected mice showed decreased lifespan, with 50% dead at 10 days and no survivors after 28 days, whereas doxorubicin-loaded-Fbg-microsphere-injected mice showed significantly increased lifespan, and 50% mortality occurred after 60 days.Citation36 Similarly, micronized droplets of olive oil containing docetaxel coated with Fbg markedly improved the therapeutic efficacy of docetaxel for the treatment of mammary tumor growth.Citation109 Recently, Fbg nanoparticles were effectively used as a carrier for 5-fluorouracilCitation42 and in curcumin delivery.Citation110
Fbg and Fbn micro/nano scaffolds integrated with biomolecules and cells for tissue engineering applications
An engineered scaffold should be biocompatible, provide sufficient mechanical strength for the specific application, and give the necessary functional support for cell growth and survival. In 3-D matrices, cell growth would be different from that on a 2-D/flat scaffold surface. When cells are growing in a 3-D scaffold, the third dimension provides another direction for cell–cell interactions, cell migration, and cell morphogenesis, all of which are essential in regulating cell cycles and tissue functions. For enhanced tissue regeneration the scaffold also needs to be grafted/immobilized with growth factors, other biomolecules, or different cells that can accelerate tissue regeneration and improve the function of the regenerated tissue through either direct release of biomolecules or via the secretion of proteins from immobilized cells. Growth factors are tissue-promoting agents that show therapeutic potential for various types of tissues regeneration (skin, nerve, vascular, dental, cartilage, bone, oral, and craniofacial) as well as for “therapeutic regeneration” processes.Citation111–Citation113 For example, in the treatment of chronic wounds, FGF and VEGF were successfully used in a bioartificial dermal substitute to improve angiogenesis through soft-tissue formation and collagen synthesis, in preclinical trials.Citation114,Citation115 Similarly, VEGF has been used in the treatment of new blood vessel formation and cardiac ischemia, in different clinical trials and animal models.Citation112,Citation116,Citation117 Furthermore, local administration of FGF-basic can stimulate wound healing, as has been detailed by clinical trials.Citation118,Citation119 However, the direct administration of growth factors have drawbacks, such as rapid clearance from the injected or implanted area (due to the administration of a more soluble form), faster rates of degradation, and short half-lives (usually less than 1 hour).Citation120 To overcome this problem, the natural polymer–based delivery system has emerged, as biopolymers can stabilize growth factors, allowing for their release in a controlled manner.Citation121 The following section describes growth factor- and cell-loaded Fbg and Fbn scaffolds which have been used in a diverse range of tissue regeneration applications. The schematic diagram in illustrates the general representation of growth factors and cell delivery from designed scaffolds, for a number of tissue engineering applications.
Nerve tissue regeneration
Peripheral nerve damage/injury often requires neural reconstruction, and the ability of the spinal cord to regenerate after injury is limited. From a clinical viewpoint, a number of scientific articles suggested that tissue engineering is a favorable option to facilitate the process of nerve repair/regeneration.Citation7,Citation24,Citation121,Citation122 The neurotrophins are a family of growth factors that maintain neuron survival and axonal elongation,Citation123 and include NGF, neurotrophin-4/5, neurotrophin-3 (NT-3), and brain-derived neurotrophic factor (BDNF).Citation124 NGF can be used to treat various neurodegenerative disorders, such as Alzheimer’s disease, Huntington’s disease, and peripheral nerve injuries; NGF also plays an essential role in sensory and sympathetic neuron endurance and preservation.Citation125 NGF has been explored as a significant drug for the treatment of spinal cord injury, to stimulate neural sensory fiber growth around chronic wounds.Citation126 NT-3 fulfills a central role in neurogenesis (by stimulating the differentiation of new neurons) and promotes corticospinal axonal growth.Citation127 NT-3 has been used as a potential therapeutic agent for spinal cord injury because it encourages neural cell growth and survival in the development of the corticospinal tract.Citation123 The direct injection of neurotrophins at the site of spinal cord injury has been shown to promote neuronal growth; however, the regenerative efficacy was reduced by various factors, such as loss of neurotrophins into the cerebrospinal fluid, aggregation, and proteolysis, and so this may not be applicable for continuous functional restoration and neuron regeneration.Citation122,Citation128 To achieve long-term protein release as well as neuronal fiber sprouting, a suitable polymer scaffold will release NGF at the specific site and at a specific rate for a specific duration.Citation121,Citation129,Citation130 The specific cell- and protein-binding characteristics of Fbg and Fbn scaffolds could be suitable for application to nerve conduit, providing an optimal microenvironment as well as molecular and electrical cues for efficient neural cell regeneration.Citation7,Citation131
NT-3-loaded Fbn scaffolds initiate regenerative responses and promote axonal growth by increasing neuronal fiber sprouting and cell migration into the injury site; moreover, the scaffold can dramatically reduce glial scar formation at the white matter border of the lesion, as demonstrated in one in vivo animal experiment.Citation122 In another study, a heparin-functionalized Fbn scaffold immobilized with NT-3, via noncovalent interactions, delivered protein in a controlled fashion to an injured spinal cord, as studied in an adult rat model. Furthermore, the heparin-Fbn-affinity-based system released the drug through cell-mediated processes and restricted drug loss by diffusion. Therefore, the controlled delivery of NT-3 from Fbn scaffolds indicated improved neuronal fiber development/growth.Citation132 The β-NGF-incorporated Fbn matrix provided enhanced nerve regeneration by effectively releasing NGF via the proteolytic activity of plasmin, and this kind of delivery system represents an improvement in nerve regeneration.Citation133 Further, it was shown that Fbn glue soaked with NT-3 expressed better dorsal root regeneration in the spinal cord and offered a strategy for injured spinal reflex arc stimulation. This study also suggested that Fbn glue is an effective exogenous vehicle for the intraspinal delivery of neurotrophic growth factors.Citation134 Fbn implantation with NT-3 induced neuronal fiber formation in rat spinal cord lesions.Citation124
In artificial nerve regeneration, other than nerve stimulating factors, stem cells, such as Schwann cells (SCs) and MSCs, also could enhance nerve growth by, themselves, secreting nerve stimulating factors.Citation135 MSCs can be induced to differentiate into SCs and to promote the regeneration of injured sciatic nerves.Citation136 SCs have a prominent specific role in nerve tissue regeneration because they can secrete NGF and other nerve regenerating proteins, which can enhance the survival of cholinergic neurons.Citation137 Because of their protein secretion advantage, these cells have a potential use in the treatment of various neurodegenerative disorders, such as Alzheimer’s and Huntington’s disease.Citation126 Both MSC- and SC-seeded polyhydroxybutyrate-fibrin (PBH-Fbn) nerve conduits have demonstrated enhanced continuous proliferation and differentiation throughout the conduit over conduits fabricated using only PBH, in vivo.Citation7 Fbn scaffolds cultured with embryonic stem cells stimulate neurons and oligodendrocytes and have been used as a platform for neural tissue engineering.Citation125 It has been suggested that both Fbg and Fbn can be an excellent scaffold for nerve regeneration, and in particular, Fbn gel has been demonstrated to encourage axonal regeneration and cell migration in short-gap nerve injury.Citation138
Bone and cartilage tissue regeneration
The poor healing potential of cartilage and bone has been a well-known concern in orthopedic surgery.Citation139 On the other hand, studies of bone tissue engineering have looked at regeneration of osteoblasts to accelerate fracture healing, correction of major defects in bones that cannot usually be rebuilt, spinal cord strengthening, and bone mineral density loss by aging.Citation6,Citation25,Citation79,Citation140 The treatment of bone loss has been conventionally managed using direct bolus injection of cells, open implantation of bone-forming cells, and scaffold-based drug delivery systems.Citation141 The skeletal system holds numerous growth factors, including TGF-β, FGF, VEGF, insulin-like growth factors (IGF-I and IGF-II), platelet-derived growth factor (PDGF) and BMPs, that contribute to cartilage and bone repairs.Citation141 Among these peptide growth factors, BMPs play a significant role in mediating the growth, remodeling, and regeneration of the skeleton system, due to their better osteoinductive properties,Citation142 and also in stimulating osteoblast proliferation and skeletal muscle component synthesis (collagen).Citation143 bFGF has mitogenic and angiogenic properties for cartilage regeneration that have been consistently confirmed.Citation144 TGF can increase bone thickness,Citation145 and the anti-inflammatory properties of TGF-β1 have been shown to stimulate cartilage regeneration via chondrocyte differentiation.Citation146 The IGFs are also viewed as one of the most significant groups of skeletal growth factors, due to their important actions on bone and cartilage cell function, repair, and regeneration.Citation147
Since 1980, Fbn has been extensively used for bone repair/regeneration; its various characteristics, including slow drug release and enhanced cellular growth, have made it a suitable carrier for different orthopedic surgery applications.Citation148 Fbn provides a natural environment for bone-forming cells,Citation149 and Fbn glue was effectively used because of its chemotactic, hemostatic, and mitogenic properties.Citation148 Recently, in bone tissue engineering, Fbn scaffolds loaded with different osteogenic growth factors and bone forming cells have been found to promote cartilage structure and viability.Citation68 In one study, BMP-2-immobilized Fbn gel, via cell-activated plasmin, resulted in a significant improvement in bone-defect healing.Citation150 Elsewhere, a heparin-incorporated Fbn-FN matrix model was reported to provide an optimal delivery system for BMPs, where the Fbn matrix acted as the platform to accommodate the infiltrating tissue, while FN established a connection between Fbn and heparin and also provided an adhesion site for tissue-repair cells.Citation151 Kang et al reported that Fbn scaffold itself has good bone cell inductive properties, and when it’s loaded with peptide growth factors, such as with BMP-2 shows enhanced bone regeneration.Citation152
Recently, bone marrow cells and MSCs were incorporated into Fbn scaffolds to encourage meniscal healing in an animal model.Citation153–Citation156 MSCs are multipotent and are capable of producing mesenchymal tissues like bone, cartilage, and tendon.Citation50 The MSC-loaded Fbn matrix was used as an autogenous bone graft because MSCs can easily undergo many cycles of cell division without losing their osteogenic capacity/properties, a feature that holds strong implications for orthopedic surgery and oral and maxillofacial reconstructions.Citation157 MSCs-Fbn microbeads secrete mineralized ECM around the implantation area, and bone formation is promoted by secretion of osteogenesis (ascorbic acid, β-glycerophosphate, and dexamethasone) and osteoblast growth factors.Citation158 Isogai et al reported that periosteal cell–cultured Fbn glue stimulated new bone formation at heterotopic sites, in an in vivo animal study.Citation159 In another study, Fbn hydrogel mixed with freshly isolated bovine chondrocytes showed coherent cartilaginous ECM production and distribution.Citation106 Currently, studies concerned with the fabrication of Fbn-based scaffolds for bone and cartilage regeneration have observed the advantages of this material when used to deliver multiple osteogenic growth factors and cells.Citation6,Citation92,Citation104 These advantages include the stimulation of osteoblasts, osteocytes, and osteoid cell proliferation, extending the application of such procedures beyond the treatment of isolated defects and fractures. However, effective bone and cartilage regeneration depend on the associated drug/cell delivery systems, the duration of biological cues release, and biochemical interactions between cells and the polymer carrier.
In bone tissue engineering and orthopedic surgery, Fbn composites have been widely used because of their good adhesive properties and their reduction of blood loss intra- and postoperatively as well as for their ease of manipulation.Citation160,Citation161 Fbn-coated calcium phosphate granules implanted in male Swiss albino mice depicted new bone formation and good healing responses at the extraskeletal site. Furthermore, bone formation was found to be conspicuous on Fbn-coated granules, whereas the uncoated granules did not show any de nova bone formation.Citation162 The Fbn-based biocomposite scaffolds seemed to stimulate mineralization on the Fbn network that was present within the ceramic grains; moreover, it was suggested Fbn glue could stabilize the granules at the implantation site, to mold the bone defects without an empty space and also provide favorable microenvironmental conditions for the osteogenic cells, leading to rapid bone healing effects.Citation73 Fbn, calcium phosphate, and hydroxyapatite–based composites could effectively induce bone repair. However, for better bone tissue regeneration, scaffolds should have well-controlled micro/nanopore interconnected networks, so that they can provide ease of cell attachment and proliferation.Citation73 In one study, the porous scaffold developed by a Fbn–calcium phosphate composite showed tightly interconnected networks and porous structure. The biodegradable composite supported primary osteoblast-like cell adhesion, proliferation, and differentiation in bone tissue engineering, in vitro and in vivo experiments.Citation79 In another study, the combination of Fbn sealant and ceramic depicted a synergic effect in bone and cartilage tissue engineering; also the bioceramics showed enhanced physical properties compared with ceramic alone.Citation163
Vascular tissue regeneration
The selection of suitable biomaterials that are capable of inducing endothelialization and blood vessel formation is significant in therapeutic regeneration, especially for vascular tissue engineering. Angiogenesis is another eminent concern for vascular tissue engineering; angiogenesis is the process by which the preexisting vasculature gives form to new blood vessels. The stimulation of neovascularization is based on the various angiogenic factors, and each angiogenic factor would function respectively, to make perfect functional blood vessels. Several growth factors have been applied to therapeutic angiogenesis, including the angiopoietins, hepatocyte growth factor (HGF), FGF (acidic and basic), and VEGF. Among these stimulators, VEGF is one of the major groups of proteins involved in blood vessel formation, by directly stimulating endothelial cells migration and proliferation; the VEGFs include VEGF-A, -B, -C, -D, -E and -F.Citation164 After VEGF, the FGFs are the most widely applied proteins for restoration of the blood supply in ischemic tissues, and these stimulate reendothelialization of wounded arteries;Citation165–Citation167 in particular, bFGF binds to endothelial cells and enhances their migration and proliferation for new blood vessel formation.Citation168,Citation169 HGF, neurotrophin, erythropoietin, and IGF are all also used for targeted vascular repair.Citation68 In addition to other growth factors, such as PDGF, epherin B2, and angiopoietin 1, these help to stabilize the newly shaped blood vessels, by recruiting smooth muscle cells to the nascent endothelial capillary.Citation170 Angiopoietins 1 and 2 function through the Tie2 receptor, which controls signal survival, migration of endothelial cells, regulation of blood vessel remodeling, and maintenance of vascular integrity.Citation171 Generally, for new blood vessel formation around ischemic tissues or associated with implants, it is presumed that efficient neovascularization requires the sustained delivery of angiogenic proteins to settled tissue over a period of weeks.Citation172,Citation173
Fbg/Fbn is a blood protein which has the ability to augment the biochemical and mechanical integrity of the scaffold for vascular regeneration.Citation11 The enzymatic fragmentation of fibrin yields a variety of degradation products that are capable of inducing angiogenesis; in particular, Fbn fragment E is a potent angiogenic factor, which has been studied alongside chicken chorioallantoic membrane (CAM).Citation70,Citation164 In one study, the heparin-functionalized Fbn matrix immobilized with VEGF was shown to be highly effective in delivering the growth factor over a period of months. Moreover, the matrix maintained VEGF bioefficacy for long term better than the other polymeric systems.Citation35 VEGF-grafted Fbn gel also showed enhanced therapeutic angiogenic outcomes, in a rabbit ischemic hind limb model.Citation35 Elsewhere, covalently immobilized VEGF in a Fbn hydrogel revealed dose-dependent protein release with excellent growth of endothelial cells, which further stimulated an angiogenic response in an ischemic region.Citation174 The VEGF-modified Fbn gel was shown to induce new blood vessel formation in CAM.Citation174 Similarly, bFGF-incorporated Fbn scaffolds have shown a comparatively slow release, for continuous angiogenesis.Citation5 Fbn scaffolds derived from blood clots were used as a local injectable carrier for VEGF, as this approach prevents the initial burst-release issue and maintains a controlled release.Citation175 Both VEGF- and bFGF-immobilized Fbn-based scaffold systems have shown increased neovascularization, with the establishment of more mature blood vessels compared with the delivery of single growth factor.Citation176 Arkudas et al investigated whether VEGF-and bFGF-immobilized Fbn gels significantly induce higher absolute blood vessels formation in an arteriovenous loop mode.Citation177 Composite scaffolds made with poly(ether)urethane-polydimethylsiloxane/Fbn can also be effectively used as a sustained delivery system for angiogenetic growth factors.Citation94
Both Fbg and Fbn contain binding sites for various angiogenic factors, including adipose-derived stem cells and bone marrow stem cells.Citation178,Citation179 Both Fbg and Fbn provide a high seeding efficacy with uniform cell distribution for certain stem cells, such as hematopoietic stem cells, endothelial progenitor cells, smooth muscle cells, and MSCs, which are potentially differentiated into vascular-promoting cells in vitro.Citation180 Endothelial cell–seeded-Fbn-hydrogel-induced blood vessels were shown to achieve good mechanical strength, with significant production of collagen and elastin, and showed considerable vasoreactivity within 2 weeks when implanted into 12-week-old lambs.Citation11 Similarly, a 3-D Fbn gel containing human aortic myofibroblast cells also resulted in good vascular development, with controlled degradation in vitro.Citation65 Smooth muscle cells can easily bind with Fbn via integrin αvβ3, evoking a number of biochemical responses, such as secretion of proteolytic enzyme and production of the extracellular matrices collagen and elastin, which lead to Fbn degradation and increased mechanical strength, respectively, aiding in the formation of blood vessels.Citation181 Moreover, Fbn-based carriers immobilized with angioinductive cells and vascular-stimulating proteins can be effectively used in vascular repair and in the induction of angiogenesis.Citation182
Skin tissue regeneration
Skin injury requires a coordinated communication of hemostatic and inflammatory systems, influenced by cytokines and other growth factors, which locally regulate cellular proliferation and skin restoration.Citation183 A number of growth factors are involved in skin regeneration, such as EGF, FGF, keratinocyte growth factor (KGF), PDGF, and TGF-β, each of which has been studied for its promotion of wound healing. EGF is a powerful growth factor for epidermal cell growth and is widely used as a therapeutic agent for epidermal cell migration, proliferation, and for stimulation of skin reepithelialization.Citation184 EGF can also promote the synthesis of collagen and other cell-adhesive materials through epidermal cell proliferation, leading to increased rates of wound healing.Citation184,Citation68 FGF has therapeutic potential for skin tissue regeneration; specifically, bFGF stimulates collagen synthesis, soft-tissue formation, and reepithelialization, as shown by in vivo study of animal models.Citation118,Citation185 FN is another potent mitogen that plays a crucial role in wound healing; upon injury it is bound to Fbn and is deposited at the site of injury, coagulating blood and providing a provisional matrix for epidermal cell migration and differentiation.Citation28 KGF is also a member of the FGF family (FGF-7) and plays a significant role in epithelial development and the promotion of wound healing.Citation186,Citation187 The TGF-β members includes three isoforms namely, TGF-β1, TGF-β2, and TGF-β3, which are involved in either scar-free healing or scaring repair.
Fbg/Fbn is a natural wound healing matrix that provides 3-D cell-infiltration scaffolds and facilitates cell adhesion and migration within wound areas.Citation18 During wound healing, Fbg could improve the verity of ECM proteins (hyaluronan and laminin) secretion which may additionally encourage the properties and function of engineered skin tissues.Citation20 bFGF can be easily bound to Fbg and Fbn due to the specific interactions; the bFGF-grafted Fbg and Fbn play a role in fibroblast adhesion and spreading, as well as in proliferation, ECM synthesis, and reepithelialization.Citation19 Fbn scaffold supplemented with TGF-β2 was shown to improve the wound healing of cutaneous wounds compared with Fbn alone in an in vivo animal study.Citation26 In another, recent study it was shown that PDGF-embedded Fbn gel enhanced wound healing in excisional wounds.Citation188 Elsewhere, the application of an Fbn hydrogel cross-linked with KGF showed long-term, controlled release and accelerated healing, which are difficult to achieve through the normal healing process, in vitro and in vivo.Citation189 Gwak et al reported that the combined delivery of EGF and KGF accelerated rapid epidermal regeneration compared with therapy using either EGF or KGF alone.Citation190 The combined therapy also resulted in significantly improved epidermal thickness compared with each separate therapy alone. EGF-immobilized Fbn scaffolds resulted in effective regeneration of keratinocytes.Citation191 Zhou et al developed a novel Fbn-based delivery system for rhEGF delivery, which may have great potential in topical wound healing applications, such as burns, surface destructions, ulcer fssures, and blisters.Citation90 Finally, Fbg matrix covalently cross-linked to FN with factor XIII was shown to stimulate macrophage, fibroblast, and endothelial cell migration at the site of tissue injury and is involved in full expression of macrophage immobilization.Citation192–Citation195
Fibroblast cells enrolled to the site of tissue injury are considered to be essential for successful skin regeneration.Citation196 Human keratinocyte cells and melanocytes have also been evaluated for potential application in wound healing.Citation197 Fibrocytes are believed to have diverse biochemical functions in wound healing processes and also are believed to induce angiogenesis in vitro and in vivo.Citation198 Fibroblast-coated Fbn microbeads have been found to stimulate granulation in tissue formation,Citation8 and keratinocytes suspended in Fbn matrix were successful in reconstituting full-thickness wounds, in both mouse and human.Citation199 Keratinocyte cell–seeded Fbn gel secretes a major cytokine (TGF-α) that promotes keratinocyte proliferation and initiates reepithelialization via the EGF-receptor/TGF-α pathway.Citation200 A recent study revealed that transplantation of an autologous keratinocyte-suspended Fbn scaffold could be efficiently used in the treatment of chronic venous leg ulcers.Citation29 Similarly, Fbn scaffolds incorporated with adenovirus encoding endothelial nitric oxide synthase (eNOS) were shown to enhance NO production at the site of diabetic wounds.Citation201 The enhanced NO production stimulated angiogenesis, endothelial and epithelial cell proliferation, and cell migration.Citation202
Conclusion and future perspectives
In the last three or four decades, a number of outstanding reviews and research articles have been printed on natural and synthetic polymers and their combination, which have significant relevance toward the development of drug delivery systems, cancer treatment, and tissue engineering applications. Specifically, protein-based polymer scaffolds containing micro/nanoporous structures have been the focus of this review, where Fbg- and Fbn-based micro/nanoscaffold systems with micro/nanoporous structures, exclusively used for tissue engineering applications, have been discussed. The advantages of these materials include their biocompatibility, biodegradability, and nonimmunogenicity, and together with their various cell-adhesive/binding properties have resulted in their widespread and successful application as a biomaterial. Fbg/Fbn-immobilized growth factors have been shown to be continuously released for several days in a controlled manner, resulting in a significant improvement in cartilage/bone, nerve, vascular, and skin regeneration/repair. The release of growth factors and other tissue-inductive proteins is only sufficient when there are enough cells at the site of injury to stimulate or respond to respective signals or regeneration. Once the severity of a wound is deep/thick, the local cell population may be inadequate to regenerate the wound, and so, extra cells must be introduced in order for complete repair to take place. This is one of the major indications for tissue engineering applications, and Fbg and Fbn scaffolds have proven to be useful candidates in vascular, cartilage, bone, skin and nerve regeneration. Various scaffold developments in our laboratory, such as Fbg microspheres, Fbg nanospheres, Fbg microfibers, highly porous Fbg sheets, and Fbg microtubes, could serve in further innovations in tissue engineering applications. The developed scaffolds showed good results in terms of biomolecule grafting, cell attachment, and cell proliferation in tissue engineering, and could play a significant role in artificial tissue constructs, especially in wound dressing preparation and in artificial vascular development. Fbg/Fbn scaffolds have some limitations, such as weak mechanical strength and quicker degradation rate; however, their mechanical strength can be improved through either mixing with other natural/synthetic polymers or incorporation of micro/nanospheres and cells, while their quicker degradation can be controlled by various cross-linking methods. Next-generation scaffolds should be immobilized with growth factors, other biomolecules, and suitable cell lines so as to accelerate, augment, and support cell proliferation and differentiation, enhancing the regeneration of fully functional tissue. In conclusion, Fbg and Fbn as drug delivery carriers and tissue engineering scaffolds can be used to deliver the drug and growth factors in a controlled manner, and also can be used to deliver the variety of cells by local injection, which may accelerate tissue regeneration. However, the overall processes of wound healing and vascular repair are complex processes, and there are a plethora of factors that may be considered in the continuous advancement of tissue engineering.
Acknowledgments
This work was supported by a National Research Foundation of Korea (NRF) grant funded by the Korean government (MEST) (2012R1A2A2A03046819: Development of biodegradable and biocompatible nano-patterned microfibers and microtubes for skin and vascular tissue engineering) and the GRRC program of Gyeonggi province [GRRCKyungwon 2013-A01, Development of Microfluidic Chip for diagnosis of disease].
Disclosure
The authors report no conflicts of interest in this work.
References
- BlombäckBHesselBHoggDTherkildsenLA two-step fibrinogen – fibrin transition in blood coagulationNature19782755680501505692730
- BensaïdWTriffittJTBlanchatCOudinaKSedelLPetiteHA biodegradable fibrin scaffold for mesenchymal stem cell transplantationBiomaterials200324142497250212695076
- SellSAWolfePSGargKMcCoolJMRodriguezIABowlinGLThe use of natural polymers in tissue engineering: a focus on electrospun extracellular matrix analoguesPolymers201024522553
- MeinhartJFusseneggerMHöblingWStabilization of fibrin-chondrocyte constructs for cartilage reconstructionAnn Plast Surg199942667367810382807
- SahniAFrancisCWVascular endothelial growth factor binds to fibrinogen and fibrin and stimulates endothelial cell proliferationBlood200096123772377811090059
- ParkKHKimHMoonSNaKBone morphogenic protein-2 (BMP-2) loaded nanoparticles mixed with human mesenchymal stem cell in fibrin hydrogel for bone tissue engineeringJ Biosci Bioeng2009108653053719914589
- KalbermattenDFKinghamPJMahayDFibrin matrix for suspension of regenerative cells in an artificial nerve conduitJ Plast Reconstr Aesthet Surg200861666967518218346
- GorodetskyRClarkRAAnJFibrin microbeads (FMB) as biodegradable carriers for culturing cells and for accelerating wound healingJ Invest Dermatol1999112686687210383731
- RajangamTPaikHJAnSSADevelopment of fibrinogen microspheres as a biodegradable carrier for tissue engineeringBioChip J201152175183
- RajangamTPaikH-jAnSFabricating fibrinogen microfibers with aligned nanostructure, as biodegradable threads for tissue engineeringBull Korean Chem Soc201233620752078
- SwartzDDRussellJAAndreadisSTEngineering of fibrin-based functional and implantable small-diameter blood vesselsAm J Physiol Heart Circ Physiol20052883H1451H146015486037
- RejinoldNSMuthunarayananMDeepaNChennazhiKPNairSVJayakumarRDevelopment of novel fibrinogen nanoparticles by two-step co-acervation methodInt J Biol Macromol2010471374320380849
- WnekGECarrMESimpsonDGBowlinGLElectrospinning of nanofiber fibrinogen structuresNano Lett200232213216
- RoweSLLeeSStegemannJPInfluence of thrombin concentration on the mechanical and morphological properties of cell-seeded fibrin hydrogelsActa Biomater200731596717085089
- MosessonMWSiebenlistKRMehDAThe structure and biological features of fibrinogen and fibrinAnn N Y Acad Sci2001936113011460466
- RybarczykBJLawrenceSOSimpson-HaidarisPJMatrix-fibrinogen enhances wound closure by increasing both cell proliferation and migrationBlood2003102124035404312920033
- DrewAFLiuHDavidsonJMDaughertyCCDegenJLWound-healing defects in mice lacking fibrinogenBlood200197123691369811389004
- GailitJClarkeCNewmanDTonnesenMGMosessonMWClarkRAHuman fibroblasts bind directly to fibrinogen at RGD sites through integrin alpha(v) beta3Exp Cell Res199723211181269141628
- SahniAOdrljinTFrancisCWBinding of basic fibroblast growth factor to fibrinogen and fibrinJ Biol Chem199827313755475599516457
- JanmeyPAWinerJPWeiselJWFibrin gels and their clinical and bioengineering applicationsJ R Soc Interface200963011018801715
- StegemannJPKaszubaSNRoweSLReview: advances in vascular tissue engineering using protein-based biomaterialsTissue Eng200713112601261317961004
- EhrbarMMettersAZammarettiPHubbellJAZischAHEndothelial cell proliferation and progenitor maturation by fibrin-bound VEGF variants with differential susceptibilities to local cellular activityJ Control Release20051011–39310915588897
- BreenAO’BrienTPanditAFibrin as a delivery system for therapeutic drugs and biomoleculesTissue Eng Part B Rev200915220121419249942
- BhangSHJeonOChoiCYKwonYHKimBSControlled release of nerve growth factor from fibrin gelJ Biomed Mater Res A2007804998100217117469
- LeeTCHoJTHungKSChenWFChungYHYangYLBone morphogenetic protein gene therapy using a fibrin scaffold for a rabbit spinal-fusion experimentNeurosurgery2006582373380 discussion 373–38016462492
- PetratosPBFelsenDTrierweilerGPrattBMcPhersonJMPoppasDPTransforming growth factor-beta2 (TGF-beta2) reverses the inhibitory effects of fibrin sealant on cutaneous wound repair in the pigWound Repair Regen200210425225812191008
- YangHSBhangSHHwangJWKimDIKimBSDelivery of basic fibroblast growth factor using heparin-conjugated fibrin for therapeutic angiogenesisTissue Eng Part A20101662113211920136400
- ClarkRALaniganJMDellaPellePManseauEDvorakHFColvinRBFibronectin and fibrin provide a provisional matrix for epidermal cell migration during wound reepithelializationJ Investig Dermatol19827952642696752288
- HartmannAQuistJHammHBröckerEBFriedlPTransplantation of autologous keratinocyte suspension in fibrin matrix to chronic venous leg ulcers: improved long-term healing after removal of the fibrin carrierDermatol Surg200834792292918384617
- JaySMSaltzmanWMControlled delivery of VEGF via modulation of alginate microparticle ionic crosslinkingJ Control Release20091341263419027807
- HiemstraCZhongZvan SteenbergenMJHenninkWEFeijenJRelease of model proteins and basic fibroblast growth factor from in situ forming degradable dextran hydrogelsJ Control Release20071221717817658651
- CaiSLiuYZheng ShuXPrestwichGDInjectable glycosaminoglycan hydrogels for controlled release of human basic fibroblast growth factorBiomaterials200526306054606715958243
- VranaNELiuYMcGuinnessGBCahillPACharacterization of poly(vinyl alcohol)/chitosan hydrogels as vascular tissue engineering scaffoldsMacromol Symp20082691106110
- ZhongYZhangLDingAGRescue of SCID murine ischemic hindlimbs with pH-modified rhbFGF/poly(DL-lactic-co-glycolic acid) implantsJ Control Release2007122333133717706831
- ChungYIKimSKLeeYKEfficient revascularization by VEGF administration via heparin-functionalized nanoparticle-fibrin complexJ Control Release2010143328228920093158
- MiyazakiSHashiguchiNSugiyamaMTakadaMMorimotoYFibrinogen microspheres as novel drug delivery systems for antitumor drugsChem Pharm Bull (Tokyo)1986343137013753731352
- HoHOHsiaoCCChenCYSokoloskiTDSheuMTFibrin-based drug delivery systems III: The evaluation of the release of macromolecules from microbeadsJ Control Release19953416570
- LeviMFriederichPWMiddletonSFibrinogen-coated albumin microcapsules reduce bleeding in severely thrombocytopenic rabbitsNat Med1999511071119883848
- MiyazakiSHashiguchiNHouWMYokouchiCTakadaMPreparation and evaluation in vitro and in vivo of fibrinogen microspheres containing adriamycinChem Pharm Bull (Tokyo)1986348338433933791511
- KulkarniMMGreiserUO’BrienTPanditAA temporal gene delivery system based on fibrin microspheresMol Pharm20118243944621171649
- HoHOHsiaoCCChenCYSokoloskiTDSheuMTFibrin-based drug delivery systems. II. The preparation and characterization of microbeadsDrug Dev Ind Pharm1994204535546
- RejinoldNSMuthunarayananMChennazhiKPNairSVJayakumarR5-fluorouracil loaded fibrinogen nanoparticles for cancer drug delivery applicationsIntl J Biol Macromol201148198105
- McManusMCBolandEDKooHPMechanical properties of electrospun fibrinogen structuresActa Biomater200621192816701855
- McManusMCBolandEDSimpsonDGBarnesCPBowlinGLElectrospun fibrinogen: feasibility as a tissue engineering scaffold in a rat cell culture modelJ Biomed Mater Res A200781229930917120217
- SellSAFrancisMPGargKMcClureMJSimpsonDGBowlinGLCross-linking methods of electrospun fibrinogen scaffolds for tissue engineering applicationsBiomed Mater20083404500118824779
- CarlisleCRCoulaisCNamboothiryMCarrollDLHantganRRGutholdMThe mechanical properties of individual, electrospun fibrinogen fibersBiomaterials20093061205121319058845
- McManusMBolandESellSElectrospun nanofibre fibrinogen for urinary tract tissue reconstructionBiomed Mater20072425726218458483
- McManusMCSellSABowenWCKooHPSimpsonDGBowlinGLElectrospun fibrinogen-polydioxanone composite matrix: potential for in situ urologic tissue engineeringJ Eng Fiber Fabr2008321221
- NgRZangRYangKKLiuNYangSTThree-dimensional fibrous scaffolds with microstructures and nanotextures for tissue engineeringRSC Adv20122271011010124
- PittengerMFMackayAMBeckSCMultilineage potential of adult human mesenchymal stem cellsScience1999284541114314710102814
- RajangamTAnSSImproved fibronectin-immobilized fibrinogen microthreads for the attachment and proliferation of fibroblastsInt J Nanomedicine201381037104923515334
- CurrieLJSharpeJRMartinRThe use of fibrin glue in skin grafts and tissue-engineered skin replacements: a reviewPlast Reconstr Surg200110861713172611711954
- MatrasHFibrin seal: The state of the artJ Oral Maxillofac Surg19854386056113891930
- SaltzRSierraDFeldmanDSaltzMBDimickAVasconezLOExperimental and clinical applications of fibrin gluePlast Reconstr Surg199188610051015 discussion 1016–10171946751
- BlombäckBBarkNFibrinopeptides and fibrin gel structureBiophys Chem20041122–314715115572242
- BlombäckBCarlssonKHesselBLiljeborgAProcykRÅslundNNative fibrin gel networks observed by 3D microscopy, permeation and turbidityBiochim Biophys Acta19899971–2961102752057
- KubotaKKogureHMasudaYGelation dynamics and gel structure of fibrinogenColloids Surf B Biointerfaces2004383–410310915542309
- Sha’banMKimSHIdrusRBKhangGFibrin and poly(lactic-co-glycolic acid) hybrid scaffold promotes early chondrogenesis of articular chondrocytes: an in vitro studyJ Orthop Surg Res200831718435862
- MolAvan LieshoutMIDam-de VeenCGFibrin as a cell carrier in cardiovascular tissue engineering applicationsBiomaterials200526163113312115603806
- AlmanyLSeliktarDBiosynthetic hydrogel scaffolds made from fibrinogen and polyethylene glycol for 3D cell culturesBiomaterials200526152467247715585249
- ZhaoHMaLGongYGaoCShenJA polylactide/fibrin gel composite scaffold for cartilage tissue engineering: fabrication and an in vitro evaluationJ Mater Sci Mater Med200920113514318704656
- LeiYRahimMNgQSeguraTHyaluronic acid and fibrin hydrogels with concentrated DNA/PEI polyplexes for local gene deliveryJ Control Release2011153325526121295089
- ShachafYGonen-WadmanyMSeliktarDThe biocompatibility of PluronicF127 fibrinogen-based hydrogelsBiomaterials201031102836284720092890
- IkariYFujikawaKYeeKOSchwartzSMAlpha(1)-proteinase inhibitor, alpha(1)-antichymotrypsin, or alpha(2)-macroglobulin is required for vascular smooth muscle cell spreading in three-dimensional fibrin gelJ Biol Chem200027517127991280510777577
- YeQZündGBenediktPFibrin gel as a three dimensional matrix in cardiovascular tissue engineeringEur J Cardiothorac Surg200017558759110814924
- HaughMGThorpeSDVinardellTBuckleyCTKellyDJThe application of plastic compression to modulate fibrin hydrogel mechanical propertiesJ Mech Behav Biomed Mater201216667223149099
- KimSJJangDHParkWHMinBMFabrication and characterization of 3-dimensional PLGA nanofiber/microfiber composite scaffoldsPolymer201051613201327
- MarimuthuMKimSSurvey of the state of the art in biomaterials, cells, genes and proteins integrated into micro- and nanoscaffolds for tissue regenerationCurrent Nanoscience200952189203
- TakeiATashiroYNakashimaYSueishiKEffects of fibrin on the angiogenesis in vitro of bovine endothelial cells in collagen gelIn Vitro Cell Dev Biol Anim19953164674728589891
- Bootle-WilbrahamCATazzymanSThompsonWDStirkCMLewisCEFibrin fragment E stimulates the proliferation, migration and differentiation of human microvascular endothelial cells in vitroAngiogenesis20014426927512197472
- HerrickSBlanc-BrudeOGrayALaurentGFibrinogenInt J Biochem Cell Biol199931774174610467729
- Oss-RonenLSeliktarDPolymer-conjugated albumin and fibrinogen composite hydrogels as cell scaffolds designed for affinity-based drug deliveryActa Biomater20117116317020643230
- Le NihouannenDSaffarzadehAAguadoEOsteogenic properties of calcium phosphate ceramics and fibrin glue based compositesJ Mater Sci Mater Med200718222523517323153
- FrismanISeliktarDBianco-PeledHNanostructuring of PEG-fibrinogen polymeric scaffoldsActa Biomater2010672518252419615475
- Van LieshoutMPetersGRuttenMBaaijensFA knitted, fibrin-covered polycaprolactone scaffold for tissue engineering of the aortic valveTissue Eng200612348148716579681
- LeeCRGradSGornaKGogolewskiSGoesslAAliniMFibrin-polyurethane composites for articular cartilage tissue engineering: a preliminary analysisTissue Eng2005119–101562157316259610
- De LaporteLYanALSheaLDLocal gene delivery from ECM-coated poly(lactide-co-glycolide) multiple channel bridges after spinal cord injuryBiomaterials200930122361236819144400
- LeiYHuangSSharif-KashaniPChenYKavehpourPSeguraTIncorporation of active DNA/cationic polymer polyplexes into hydrogel scaffoldsBiomaterials201031349106911620822811
- OsathanonTLinnesMLRajacharRMRatnerBDSomermanMJGiachelliCMMicroporous nanofibrous fibrin-based scaffolds for bone tissue engineeringBiomaterials200829304091409918640716
- PankajakshanDPhiliposeLPPalakkalMKrishnanKKrishnanLKDevelopment of a fibrin composite-coated poly(epsilon-caprolactone) scaffold for potential vascular tissue engineering applicationsJ Biomed Mater Res B Appl Biomater200887257057918546199
- Thein-HanWWSaikhunJPholpramooCMisraRDKKitiyanantYChitosan-gelatin scaffolds for tissue engineering: physico-chemical properties and biological response of buffalo embryonic stem cells and transfectant of GFP-buffalo embryonic stem cellsActa Biomater2009593453346619460465
- NoorjahanSESastryTPAn in vivo study of hydrogels based on physiologically clotted fibrin-gelatin composites as wound-dressing materialsJ Biomed Mater Res B Appl Biomater200471230531215386397
- NatarajanNShashirekhaVNoorjahanSERameshkumarMRoseCSastryTPFibrin–chitosan–gelatin composite film: preparation and characterizationJournal of Macromolecular Science, Part A: Pure and Applied Chemistry2005427945953
- XuWWangXYanYRapid prototyping three-dimensional cell/gelatin/fibrinogen constructs for medical regenerationJ Bioact Compat Polym2007224363377
- ErdoganDvan GulikTMEvolution of fibrinogen-coated collagen patch for use as a topical hemostatic agentJ Biomed Mater Res B Appl Biomater200885127227817806107
- HanCMZhangLPSunJZShiHFZhouJGaoCYApplication of collagen-chitosan/fibrin glue asymmetric scaffolds in skin tissue engineeringJ Zhejiang Univ Sci B201011752453020593518
- LorussoRDe CiccoGVizzardiEGelsominoSHuman fibrinogen/thrombin-coated collagen patch to control intraoperative severe pulmonary hemorrhage and air leakage after correction of a ruptured thoracic aortic aneurysmAnn Thorac Surg201191391791921353031
- ChenZWangLStegemannJPPhase-separated chitosan-fibrin microbeads for cell deliveryJ Microencapsul201128534435221736519
- CummingsCLGawlittaDNeremRMStegemannJPProperties of engineered vascular constructs made from collagen, fibrin, and collagen-fibrin mixturesBiomaterials200425173699370615020145
- ZhouWZhaoMZhaoYMouYA fibrin gel loaded with chitosan nanoparticles for local delivery of rhEGF: preparation and in vitro release studiesJ Mater Sci Mater Med20112251221123021445654
- ParkSHChoiBHParkSRMinBHChondrogenesis of rabbit mesenchymal stem cells in fibrin/hyaluronan composite scaffold in vitroTissue Eng Part A2011179–101277128621189070
- PerkaCSchultzOLindenhaynKJoint cartilage repair with transplantation of embryonic chondrocytes embedded in collagen-fibrin matricesClin Exp Rheumatol2000181132210728439
- ParkSHParkSRChungSIPaiKSMinBHTissue-engineered cartilage using fibrin/hyaluronan composite gel and its in vivo implantationArtif Organs2005291083884516185347
- BrigantiESpillerDMirtelliCA composite fibrin-based scaffold for controlled delivery of bioactive pro-angiogenetic growth factorsJ Control Release20101421142119811766
- FilováEJelinekFHandlMNovel composite hyaluronan/type I collagen/fibrin scaffold enhances repair of osteochondral defect in rabbit kneeJ Biomed Mater Res B Appl Biomater200887241542418435405
- VenkatramanSBoeyFLaoLLImplanted cardiovascular polymers: Natural, synthetic and bio-inspiredProg Polym Sci2008339853874
- MarderVJIdentification and purification of fibrinogen degradation products produced by plasmin: considerations on the structure of fibrinogenScand J Haematol Suppl19711321364258202
- StändkerLSillardRBenschKWIn vivo degradation of human fibrinogen Aα: detection of cleavage sites and release of antithrombotic peptidesBiochem Biophys Res Commun199521538969027488058
- MihályíECross-linking of bovine fibrinogen with formaldehydeActa Chem Scand196317SupplS277S281
- FurlanMBeckEACross-linking of human fibrinogen with glutaraldehyde and tetranitromethaneThromb Res197576827838812201
- Olde DaminkLHDijkstraPJvan LuynMJvan WachemPBNieuwenhuisPFeijenJCross-linking of dermal sheep collagen using a water-soluble carbodiimideBiomaterials19961787657738730960
- SungHWChangWHMaCYLeeMHCrosslinking of biological tissues using genipin and/or carbodiimideJ Biomed Mater Res A200364342743812579556
- JayakrishnanAJameelaSRGlutaraldehyde as a fixative in bioprostheses and drug delivery matricesBiomaterials19961754714848991478
- DareEVGriffithMPoitrasPGenipin cross-linked fibrin hydrogels for in vitro human articular cartilage tissue-engineered regenerationCells Tissues Organs2009190631332519287127
- FusseneggerMMeinhartJHöblingWKullichWFunkSBernatzkyGStabilized autologous fibrin-chondrocyte constructs for cartilage repair in vivoAnn Plast Surg200351549349814595186
- EyrichDBrandlFAppelBLong-term stable fibrin gels for cartilage engineeringBiomaterials2007281556516962167
- OsadaKChristieRJKataokaKPolymeric micelles from poly(ethylene glycol)-poly(amino acid) block copolymer for drug and gene deliveryJ R Soc Interface20096Suppl 3S325S33919364722
- CostantiniVZacharskiLRThe role of fibrin in tumor metastasisCancer Metastasis Rev19921132832901423819
- EinhausCMRetzingerACPerrottaAOFibrinogen-coated droplets of olive oil for delivery of docetaxel to a fibrin(ogen)-rich ascites form of a murine mammary tumorClin Cancer Res200410207001701015501980
- RejinoldNSMuthunarayananMChennazhiKPNairSVJayakumarRCurcumin loaded fibrinogen nanoparticles for cancer drug deliveryJ Biomed Nanotechnol20117452153421870456
- AsaharaTBautersCZhengLPSynergistic effect of vascular endothelial growth factor and basic fibroblast growth factor on angio-genesis in vivoCirculation199592Suppl 9S365S371
- HughesGCBiswasSSYinBTherapeutic angiogenesis in chronically ischemic porcine myocardium: comparative effects of bFGF and VEGFAnn Thorac Surg200477381281814992878
- MimuraTImaiSKuboMA novel exogenous concentration-gradient collagen scaffold augments full-thickness articular cartilage repairOsteoarthritis Cartilage20081691083109118378169
- OkumuraMOkudaTNakamuraTYajimaMAcceleration of wound healing in diabetic mice by basic fibroblast growth factorBiol Pharm Bull19961945305358860952
- WilckeILohmeyerJALiuSVEGF(165) and bFGF protein-based therapy in a slow release system to improve angiogenesis in a bioartificial dermal substitute in vitro and in vivoLangenbecks Arch Surg2007392330531417404752
- HasegawaTKimuraAMiyatakaMInagakiMIshikawaKBasic fibroblast growth factor increases regional myocardial blood flow and salvages myocardium in the infarct border zone in a rabbit model of acute myocardial infarctionAngiology199950648749510378825
- LosordoDWValePRHendelRCPhase 1/2 placebo-controlled, double-blind, dose-escalating trial of myocardial vascular endothelial growth factor 2 gene transfer by catheter delivery in patients with chronic myocardial ischemiaCirculation2002105172012201811980678
- GibranNSIsikFFHeimbachDMGordonDBasic fibroblast growth factor in the early human burn woundJ Surg Res19945632262348145539
- RobsonMCPhillipsLGLawrenceWTThe safety and effect of topically applied recombinant basic fibroblast growth factor on the healing of chronic pressure soresAnn Surg19922164401406 discussion 406–4081417189
- LeeKYPetersMCMooneyDJComparison of vascular endothelial growth factor and basic fibroblast growth factor on angiogenesis in SCID miceJ Control Release2003871–3495612618022
- Sakiyama-ElbertSEHubbellJAControlled release of nerve growth factor from a heparin-containing fibrin-based cell ingrowth matrixJ Control Release200069114915811018553
- TaylorSJRosenzweigESMcDonaldJW3rdSakiyama-ElbertSEDelivery of neurotrophin-3 from fibrin enhances neuronal fiber sprouting after spinal cord injuryJ Control Release2006113322623516797770
- DaviesAMThe role of neurotrophins in the developing nervous systemJ Neurobiol19942511133413487852989
- TaylorSJMcDonaldJW3rdSakiyama-ElbertSEControlled release of neurotrophin-3 from fibrin gels for spinal cord injuryJ Control Release200498228129415262419
- WillerthSMSakiyama-ElbertSEApproaches to neural tissue engineering using scaffolds for drug deliveryAdv Drug Deliv Rev2007594–532533817482308
- RomeroMIRangappaNGarryMGSmithGMFunctional regeneration of chronically injured sensory afferents into adult spinal cord after neurotrophin gene therapyJ Neurosci200121218408841611606629
- GrillRMuraiKBleschAGageFHTuszynskiMHCellular delivery of neurotrophin-3 promotes corticospinal axonal growth and partial functional recovery after spinal cord injuryJ Neurosci19971714556055729204937
- SchnellLSchneiderRKolbeckRBardeYASchwabMENeurotrophin-3 enhances sprouting of corticospinal tract during development and after adult spinal cord lesionNature199436764591701738114912
- SakiyamaSESchenseJCHubbellJAIncorporation of heparin-binding peptides into fibrin gels enhances neurite extension: an example of designer matrices in tissue engineeringFASEB J199913152214222410593869
- Sakiyama-ElbertSEHubbellJADevelopment of fibrin derivatives for controlled release of heparin-binding growth factorsJ Control Release200065338940210699297
- BennetDKimSImplantable microdevice for peripheral nerve regeneration: materials and fabricationsJ Mater Sci2011461447234740
- TaylorSJSakiyama-ElbertSEEffect of controlled delivery of neurotrophin-3 from fibrin on spinal cord injury in a long term modelJ Control Release2006116220421016919351
- Sakiyama-ElbertSEPanitchAHubbellJADevelopment of growth factor fusion proteins for cell-triggered drug deliveryFASEB J20011571300130211344120
- IwayaKMizoiKTesslerAItohYNeurotrophic agents in fibrin glue mediate adult dorsal root regeneration into spinal cordNeurosurgery1999443589595 discussion 595–59610069596
- TohillMTerenghiGStem-cell plasticity and therapy for injuries of the peripheral nervous systemBiotechnol Appl Biochem200440Pt 1172415270703
- CaddickJKinghamPJGardinerNJWibergMTerenghiGPhenotypic and functional characteristics of mesenchymal stem cells differentiated along a Schwann cell lineageGlia200654884084916977603
- SiegelGJChauhanNBNeurotrophic factors in Alzheimer’s and Parkinson’s disease brainBrain Res Rev2000332–319922711011066
- WilliamsLRLongoFMPowellHCLundborgGVaronSSpatial-temporal progress of peripheral nerve regeneration within a silicone chamber: parameters for a bioassayJ Comp Neurol198321844604706619324
- BuckwalterJAMankinHJArticular cartilage: tissue design and chondrocyte-matrix interactionsInstr Course Lect1998474774869571449
- MalafayaPSilvaGABaranETReisRLDrug delivery therapies I – General trends and its importance on bone tissue engineering applicationsCurr Opin Solid State Mater Sci200264283295
- LeeKSilvaEAMooneyDJGrowth factor delivery-based tissue engineering: general approaches and a review of recent developmentsJ R Soc Interface201185515317020719768
- SmoljanovićTGrgurevićLJelićMRegeneration of the skeleton by recombinant human bone morphogenetic proteinsColl Antropol200731392393218041408
- CentrellaMMassaguéJCanalisEHuman platelet-derived transforming growth factor-beta stimulates parameters of bone growth in fetal rat calvariaeEndocrinology19861195230623123464414
- CuevasPBurgosJBairdABasic fibroblast growth factor (FGF) promotes cartilage repair in vivoBiochem Biophys Res Commun198815626116183190672
- NodaMCamilliereJJIn vivo stimulation of bone formation by transforming growth factor-betaEndocrinology19891246299129942721454
- SarafAMikosAGGene delivery strategies for cartilage tissue engineeringAdv Drug Deliv Rev200658459260316766079
- KunduBKhareSKSinghGRole of polypeptides in the treatment and diagnosis of osteoporosisPeptides199920452353710458525
- PatelSRodriguez-MerchanECHaddadFSThe use of fibrin glue in surgery of the kneeJ Bone Joint Surg Br201092101325133120884966
- IshimuraMOhgushiHHabataTTamaiSFujisawaYArthroscopic meniscal repair using fibrin glue. Part I: Experimental studyArthroscopy19971355515579343641
- SchmoekelHGWeberFESchenseJCGrätzKWSchawalderPHubbellJABone repair with a form of BMP-2 engineered for incorporation into fibrin cell ingrowth matricesBiotechnol Bioeng200589325326215619323
- HanDLiuWAoQWangGOptimal delivery systems for bone morphogenetic proteins in orthopedic applications should model initial tissue repair structures by using a heparin-incorporated fibrin-fibronectin matrixMed Hypotheses200871337437818565684
- KangSWKimJSParkKSSurface modification with fibrin/hyaluronic acid hydrogel on solid-free form-based scaffolds followed by BMP-2 loading to enhance bone regenerationBone201148229830620870047
- RoeddeckerKNagelschmidtMKoebkeJGuenscheKMeniscal healing: a histological study in rabbitsKnee Surg Sports Traumatol Arthrosc19931128338535993
- IzutaYOchiMAdachiNDeieMYamasakiTShinomiyaRMeniscal repair using bone marrow-derived mesenchymal stem cells: experimental study using green fluorescent protein transgenic ratsKnee200512321722315911296
- HashimotoJKurosakaMYoshiyaSHirohataKMeniscal repair using fibrin sealant and endothelial cell growth factor. An experimental study in dogsAm J Sports Med19922055375411443321
- ScottiCPozziAMangiaviniLHealing of meniscal tissue by cellular fibrin glue: an in vivo studyKnee Surg Sports Traumatol Arthrosc200917664565119296087
- YamadaYBooJSOzawaRBone regeneration following injection of mesenchymal stem cells and fibrin glue with a biodegradable scaffoldJ Craniomaxillofac Surg2003311273312553923
- GurevichOVexlerAMarxGFibrin microbeads for isolating and growing bone marrow-derived progenitor cells capable of forming bone tissueTissue Eng20028466167212202005
- IsogaiNLandisWJMoriRExperimental use of fibrin glue to induce site-directed osteogenesis from cultured periosteal cellsPlast Reconstr Surg2000105395396310724255
- AlbrektssonTBachAEdshageSJönssonAFibrin adhesive system (FAS) influence on bone healing rate: a microradiographical evaluation using the bone growth chamberActa Orthop Scand19825357577637136585
- RousouJLevitskySGonzalez-LavinLRandomized clinical trial of fibrin sealant in patients undergoing resternotomy or reoperation after cardiac operations. A multicenter studyJ Thorac Cardiovasc Surg19899721942032464722
- AbiramanSVarmaHKUmashankarPRJohnAFibrin glue as an osteoinductive protein in a mouse modelBiomaterials200223143023303112069345
- WittkampfARFibrin glue as cement for HA-granulesJ Craniomaxillofac Surg19891741791812471712
- ThompsonJAAndersonKDDiPietroJMSite-directed neovessel formation in vivoScience19882414871134913522457952
- AsaharaTBautersCPastoreCLocal delivery of vascular endothelial growth factor accelerates reendothelialization and attenuates intimal hyperplasia in balloon-injured rat carotid arteryCirculation19959111279328017758186
- AsaharaTChenDTsurumiYAccelerated restitution of endothelial integrity and endothelium-dependent function after phVEGF165 gene transferCirculation19969412329133028989143
- Van BelleETioFOCouffinhalTMaillardLPasseriJIsnerJMStent endothelialization. Time course, impact of local catheter delivery, feasibility of recombinant protein administration, and response to cytokine expeditionCirculation19979524384489008462
- DeBloisCCôtéMFDoillonCJHeparin-fibroblast growth factor-fibrin complex: in vitro and in vivo applications to collagen-based materialsBiomaterials19941596656727948588
- BrownKJMaynesSFBezosAMaguireDJFordMDParishCRA novel in vitro assay for human angiogenesisLab Invest19967545395558874385
- ZischAHLutolfMPHubbellJABiopolymeric delivery matrices for angiogenic growth factorsCardiovasc Pathol200312629531014630296
- WuXMaJHanJDWangNChenYGDistinct regulation of gene expression in human endothelial cells by TGF-beta and its receptorsMicrovasc Res2006711121916405919
- FasolRSchumacherBSchlaudraffKHauensteinKHSeitelbergerRExperimental use of a modified fibrin glue to induce site-directed angiogenesis from the aorta to the heartJ Thorac Cardiovasc Surg19941076143214397515132
- GreislerHPCziperleDJKimDUEnhanced endothelialization of expanded polytetrafluoroethylene grafts by fibroblast growth factor type 1 pretreatmentSurgery19921122244254 discussion 254–2551641764
- ZischAHSchenkUSchenseJCSakiyama-ElbertSEHubbellJACovalently conjugated VEGF – fibrin matrices for endothelializationJ Control Release2001721–310111311389989
- ShiremanPKGreislerHPMitogenicity and release of vascular endothelial growth factor with and without heparin from fibrin glueJ Vasc Surg200031593694310805884
- WongCInmanESpaetheRHelgersonSFibrin-based biomaterials to deliver human growth factorsThromb Haemost200389357358212624643
- ArkudasATjiawiJBleizifferOFibrin gel-immobilized VEGF and bFGF efficiently stimulate angiogenesis in the AV loop modelMol Med2007139–1048048717762899
- DiMuzioPTulenkoTTissue engineering applications to vascular bypass graft development: the use of adipose-derived stem cellsJ Vasc Surg200745Suppl AA99A10317544030
- CharwatSGyöngyösiMLangIRole of adult bone marrow stem cells in the repair of ischemic myocardium: current state of the artExp Hematol200836667268018358589
- ZhangGSuggsLJMatrices and scaffolds for drug delivery in vascular tissue engineeringAdv Drug Deliv Rev2007594–536037317513003
- JonesJIPrevetteTGockermanAClemmonsDRLigand occupancy of the alpha-V-beta3 integrin is necessary for smooth muscle cells to migrate in response to insulin-like growth factorProc Natl Acad Sci U S A1996936248224878637900
- ChristmanKLFokHHSieversREFangQLeeRJFibrin glue alone and skeletal myoblasts in a fibrin scaffold preserve cardiac function after myocardial infarctionTissue Eng2004103–440340915165457
- FuXBGrowth factors and skin repair and regenerationInt J Cosmet Sci20052714041
- TsurumaATanakaMYamamotoSShimomuraMControl of neural stem cell differentiation on honeycomb filmsColloids Surf A Physicochem Eng Asp2008313–314536540
- ChenWYRogersAALydonMJCharacterization of biologic properties of wound fluid collected during early stages of wound healingJ Invest Dermatol19929955595641431216
- AaronsonSABottaroDPMikiTKeratinocyte growth factor. A fibroblast growth factor family member with unusual target cell specificityAnn N Y Acad Sci199163862771664700
- WernerSKeratinocyte growth factor: a unique player in epithelial repair processesCytokine Growth Factor Rev1998921531659754709
- MogfordJETawilBJiaSMustoeTAFibrin sealant combined with fibroblasts and platelet-derived growth factor enhance wound healing in excisional woundsWound Repair Regen200917340541019660049
- GeerDJSwartzDDAndreadisSTBiomimetic delivery of keratinocyte growth factor upon cellular demand for accelerated wound healing in vitro and in vivoAm J Pathol200516761575158616314471
- GwakSJKimSSSungKHanJChoiCYKimBSSynergistic effect of keratinocyte transplantation and epidermal growth factor delivery on epidermal regenerationCell Transplant2005141080981716454355
- KitajimaTSakuragiMHasudaHOzuTItoYA chimeric epidermal growth factor with fibrin affinity promotes repair of injured keratinocyte sheetsActa Biomater2009572623263219376761
- AliIUHynesROEffects of LETS glycoprotein on cell motilityCell1978142439446667950
- PostlethwaiteAEKeski-OjaJBalianGKangAHInduction of fibroblast chemotaxis by fibronectin. Localization of the chemotactic region to a 140,000-molecular weight non-gelatin-binding fragmentJ Exp Med198115324944997241050
- BowersoxJCSorgenteNChemotaxis of aortic endothelial cells in response to fibronectinCancer Res1982427254725517083147
- RemoldHGShawJEDavidJRA macrophage surface component related to fibronectin is involved in the response to migration inhibitory factorCell Immunol19815811751877013990
- BucalaRSpiegelLAChesneyJHoganMCeramiACirculating fibrocytes define a new leukocyte subpopulation that mediates tissue repairMol Med19941171818790603
- LiuYSuwaFWangXReconstruction of a tissue-engineered skin containing melanocytesCell Biol Int200731998599017467308
- HartlappIAbeRSaeedRWFibrocytes induce an angiogenic phenotype in cultured endothelial cells and promote angiogenesis in vivoFASEB J200115122215222411641248
- HorchREBannaschHStarkGBTransplantation of cultured autologous keratinocytes in fibrin sealant biomatrix to resurface chronic woundsTransplant Proc2001331–264264411266996
- YamamotoMYanagaHNishinaHWatabeSMambaKFibrin stimulates the proliferation of human keratinocytes through the autocrine mechanism of transforming growth factor-alpha and epidermal growth factor receptorTohoku J Exp Med20052071334016082153
- BreenAMDockeryPO’BrienTPanditASThe use of therapeutic gene eNOS delivered via a fibrin scaffold enhances wound healing in a compromised wound modelBiomaterials200829213143315118442851
- YamasakiKEdingtonHDMcCloskyCReversal of impaired wound repair in iNOS-deficient mice by topical adenoviral-mediated iNOS gene transferJ Clin Invest199810159679719486966