Abstract
Background
Patupilone (EPO906) is a microtubule stabilizer with a potent antitumor effect. Integrin αVβ3-binding (RGD) liposomes were loaded with EPO906, and their antitumor efficacy was evaluated in two pediatric tumor models, ie, neuroblastoma and rhabdomyosarcoma.
Methods
Integrin αVβ3 gene expression, RGD-liposome cellular association, and the effect of EPO906 and liposomal formulations of EPO906 on cell viability were assessed in vitro in human umbilical vein endothelial cells (HUVEC), in the RH-30 rhabdomyosarcoma cell line, and in the Kelly neuroblastoma cell line. In vivo, mice bearing neuroblastoma or rhabdomyosarcoma tumors were treated with EPO906, EPO906-liposomes, or EPO906-RGD-liposomes. Tumor growth, cumulative survival, and toxicity were monitored.
Results
Integrin αVβ3 was highly expressed in HUVEC and RH-30, but not in Kelly cells. Accordingly, RGD-liposomes were highly associated with HUVEC and RH-30 cells in vitro, but not with the Kelly cells. EPO906 and its liposomal formulations inhibited HUVEC, RH-30, and Kelly cell viability to the same extent. In vivo, EPO906 1.5 mg/kg and liposomal EPO906 potently inhibited tumor growth in both xenograft models without triggering major toxicity. At this dose, liposomal EPO906 did not enhance the antitumor effect of EPO906 in neuroblastoma, but tended to have an increased antitumor effect in rhabdomyosarcoma. Using a lower dose of EPO906-RGD-liposomes significantly enhanced cumulative survival in rhabdomyosarcoma compared with EPO906 alone.
Conclusion
EPO906 shows a strong antitumor effect in neuroblastoma and rhabdomyosarcoma, without triggering major side effects. Its liposomal encapsulation does not alter its activity, and enhances cumulative survival when EPO906-RGD-liposomes are used at low dose in rhabdomyosarcoma.
Introduction
Patupilone, also known as epothilone B (EPO906), is a potent microtubule stabilizer. It belongs to the class of microtubule-binding drugs known as epothilones, initially discovered as secondary metabolite macrolides produced by the myxobacterium Sorangium cellulosum,Citation1 and rapidly shown to be taxane-like agents causing microtubule stabilization in vitro.Citation2 Like the taxanes, patupilone binds to β-tubulin on the interior surface of microtubules but with higher affinity, although the binding site on the microtubules is probably the same. This alters spindle formation, resulting in arrest of mitotic cells and cell death via apoptosis.Citation3,Citation4 In comparison with taxanes such as paclitaxel, patupilone has been shown to have more potent in vivo anticancer activity at tolerated dose levels in several human xenograft models.Citation3 Further, patupilone retains activity in vitro and in vivo in taxane-resistant cancer cells overexpressing P-glycoprotein or bearing β-tubulin mutations.Citation2,Citation5
In addition to being directly cytotoxic to tumor cells, patupilone has antivascular and antiangiogenic effects. The tumor vasculature, which is important for tumor progression, has characteristics different to those of quiescent endothelium. Immature angiogenic endothelial cells rely on a stable microtubule cytoskeleton to support their elongated shape,Citation6 making them sensitive to microtubule-binding agents. An antiproliferative effect and induction of apoptosis in endothelial cells has been observed in vitro using protracted low doses of patupilone.Citation7 Patupilone has also been recently described as a potent radiosensitizer in medulloblastoma.Citation8 Moreover, it showed potency in inhibition of the invasive angiogenic response in tumor extracts and in inducing vessel and tumor regression.Citation9 Further, a study using in situ magnetic resonance imaging and measuring interstitial fluid pressure in different tumor models showed a vascular disruptive effect of patupilone in vivo.Citation10 These combined antivascular and antiangiogenic properties suggest that patupilone has the potential to treat solid tumors successfully by its dual activity against tumor and endothelial cells.
Pediatric solid tumors are rare cancers and drug development is not focused on these life-threatening diseases due to a lack of demand. However, intensive collaborations and elaboration of protocols used in treatment-optimizing studies and clinical trials have helped to increase survival rates by up to 75% in young patients suffering from cancer in recent decades.Citation11,Citation12 Neuroblastoma and rhabdomyosarcoma are among the most frequent and aggressive tumors of childhood, and still have unsatisfactory outcomes, especially in the advanced stages.Citation13,Citation14 New molecules and therapeutic approaches are therefore urgently needed. Both entities show particular characteristics in their molecular biology, and angiogenesis has been identified to be a potential new target in neuroblastoma as well as in rhabdomyosarcoma.Citation15,Citation16
The aim of efficient tumor therapy should be to improve the specificity of drug delivery in order to have a better effect without destroying or damaging healthy cells. Among the targeting strategies, encapsulation of drugs into liposomes is a possibility for increasing the amount of drug delivered to the tumor with fewer side effects, as described, eg, with liposomal doxorubicin.Citation17,Citation18 Liposomes are spherical nanoparticles containing a phospholipid bilayer surrounding an aqueous core. Addition of a poly(ethylene glycol) (PEG) coating delays uptake by the mononuclear phagocyte system, resulting in a prolonged systemic circulation time.Citation19 In addition, liposomes exhibit preferential extravasation and accumulation at the site of a solid tumor because of increased endothelial permeability and reduced lymphatic drainage in the tumor tissue (ie, the enhanced permeability and retention effect).Citation20 Liposomes can be modified further by coupling peptides or antibodies to the outer surface to bind selectively to targeted cells to be taken up specifically by these cells. For example, small peptide ligands harboring a RGD (argininelysine-aspartate) motif that is found in the extracellular matrix can bind to integrin αVβ3. These integrins are transmembrane proteins expressed by a number of tumor cells as well as by endothelial cells actively proliferating in and around tumor tissue.Citation21–Citation23 Integrin αVβ3 is overexpressed in the tumor endothelium, but is absent or barely detectable in established blood vessels.Citation24 A number of RGD-grafted liposomal delivery systems have been developed in the last few years, not only to target tumor cells but also to have an antiangiogenic effect.Citation17,Citation25
In the present study, we investigated patupilone (EPO906) in two pediatric tumor models, ie, neuroblastoma and rhabdomyosarcoma. Inhibition of tumor growth and survival were analyzed when EPO906 was encapsulated into long-circulating PEG-coated liposomes, as well as PEG-coated liposomes additionally coupled with cyclic RGD-peptides.
Materials and methods
Cell lines
Human umbilical vein endothelial cells (HUVEC), obtained from PromoCell GmbH (Heidelberg, Germany) were grown in endothelial basal medium containing 0.1 ng/mL human epidermal growth factor, 1 ng/mL basic fibroblast growth factor, 1 μg/mL hydrocortisone, 90 μg/mL heparin, and 2% fetal calf serum (endothelial cell growth medium kit, Lonza, Walkersville, MD) under 5% CO2 at 37°C. Human neuroblastoma Kelly cells and RH-30 human rhabdomyosarcoma cells were obtained from DSMZ (Braunschweig, Germany). Both cell lines were cultured in RPMI 1640 medium (Gibco-BRL, Eggenstein, Germany) supplemented with 10% fetal calf serum, 50 U/mL penicillin G, and 50 μg/mL of streptomycin under 5% CO2 at 37°C.
Analysis of integrin αVβ3 expression
Total RNA was isolated using Trizol reagent (Invitrogen GmbH, Darmstadt, Germany) according to the manufacturer’s instructions. cDNA synthesis was performed with 1 μg of total RNA using a reverse transcription kit (QuantiTect, Qiagen, Hilden, Germany). Polymerase chain reactions were performed on a thermal cycler system (C1000™, BioRad, Hercules, CA). Primer sets for integrin αV (INTαV), integrin β3 (INTβ3), and β-actin were custom-synthesized by Thermo Fisher Scientific GmbH (Erlangen, Germany) and are described elsewhere.Citation23 Polymerase chain reaction analyses were carried out in a reaction volume of 20 μL containing 18 μL of GoTaq® DNA polymerase mix (Promega, Madison, WI) and 2 μL of cDNA.
Preparation of EPO906-encapsulated liposomes
Patupilone was prepared by the chemical department at Novartis (Basel, Switzerland). The following reagents were used for preparation of the liposomes: unsaturated soybean phosphatidylcholine (Lipoid S100) from Lipoid (Ludwigshafen, Germany); N-carbonyl-methoxy-(polyethyleneglycol2000)-1,2-distearoyl-sn-glycero-3-phosphoethanolamine sodium salt (mPEG2000-DSPE) from Genzyme Pharmaceuticals (Liestal, Switzerland); cholesterol from Fluka (Buchs, Switzerland); cyclo-(Arg-Gly-Asp-D-Phe-Cys) (RGD-peptide) and cyclo-(Arg-Ala-Asp-D-Phe-Cys) (RAD-peptide) from Peptides International Inc (Louisville, KY); 1,2-distearoyl-sn-glycero-3-phosphoethanolamine-N-[maleimide(polyethylene glycol-2000)] (ammonium salt) (Mal-PEG2000-DSPE) from Avanti Polar Lipids Inc (Alabaster, AL); and ethanol of high-performance liquid chromatography grade from Merck (Darmstadt, Germany). For the hydration buffer, potassium monobasic and disodium hydrogen phosphate dihydrate were obtained from Fluka. For the fluorescent placebo liposomes, rhodamine B-1,2-dihexadecanoyl-sn-glycero-3-phosphoethanolamine (triethylammonium salt, rhodamine-PE) was obtained from Molecular Probes (Leiden, The Netherlands).
All Mal-PEG-liposome batches were produced according to a thin film hydration method described previouslyCitation26,Citation27 Briefly, the excipients (S100/mPEG2000-DSPE/Mal-PEG2000-DSPE/cholesterol 17.71/0.69/0.52/3.48 mol/mol) were dissolved in ethanol and the solvent was evaporated using a rotavapor (Rotavap R 215, Büchi, Flawil, Switzerland) for one hour, slowly decreasing the pressure to 30 mbar, and dried further for two hours to remove the solvent completely. The lipid film was then hydrated with a phosphate buffer (pH 7.4) to yield a liposomal dispersion containing 22.4 mM total lipid. The liposomal dispersion was extruded first through a polycarbonate membrane with 100 nm pores (Millipore, Billerica, MA) three times and then through a polycarbonate membrane with 50 nm pores (Millipore) six times. A solution of RGD-peptide (1.6 mM) in phosphate buffer (pH 7.4) was added to the liposomal dispersion and the preparation was incubated at room temperature overnight using a thermomixer at 750 rpm to couple the RGD-peptides with the maleimide groups on the liposomal surface. The molar ratio of RGD-peptide to anchor was optimized to 1:6 using in vitro uptake experiments (data not shown). For a negative peptide targeting control, the inactive RAD-peptide was replaced with RGD as the targeting motif. In the RAD-peptide structure, glycine is replaced by alanine. Therefore, the peptide does not bind to the receptor-binding pocket. The free peptide was separated from the coupled fraction by size exclusion chromatography using a Sephadex CL-4B column (Sigma-Aldrich, St Louis, MO).
The average hydrodynamic diameter of the liposomes and the particle size distribution were analyzed by photon correlation spectroscopy using a Zetasizer 3000HS from Malvern (Worcestershire, UK). First, 5 μL of the liposome dispersion were diluted with 2 mL of phosphate buffer (pH 7.4) and then measured at 20°C with a 90° angle. The average hydrodynamic diameter (z-average) was calculated from 10 subruns. All liposomal preparations were smaller than 100 nm and showed a narrow particle size distribution (polydispersity index < 0.1, ). The entrapment efficiency of EPO906 was assessed using a dialysis cassette (Slide-A-Lyzer®, 10 kDa, Thermo Fisher Scientific, Lausanne, Switzerland) to separate the free drug from the liposomal fraction. Liposomes remained inside the dialysis membrane (cutoff 10 kDa) while the free drug dissolved in the excess phosphate buffer (pH 7.4) volume of 2000 mL surrounding the dialysis chamber. The liposomal fraction was taken for high-performance liquid chromatography measurements to define the amount of entrapped drug substance.Citation27 The entrapment efficiency of EPO906 was shown to be higher than 95% for all liposomal preparations.
EPO906 dissolved in PEG 300 was used as a nonliposomal control formulation, and is referred to henceforth as EPO906, EPO906 encapsulated in PEG-liposomes as EPO906-lip, EPO906 encapsulated in RGD-PEG-liposomes as EPO906-RGD-lip, and EPO906 encapsulated in RAD-PEG-liposomes as EPO906-RAD-lip.
Selectivity of integrin targeting with RGD-liposomes in vitro
To study specifically the association between RGD-liposomes and integrin on endothelial and tumor cells, we prepared empty liposomes as described above and added a hydrophobic fluorescence marker (rhodamine-PE; 0.5 mol% of total lipid). HUVEC (120,000 per well), RH-30 (80,000 per well), and Kelly (80,000 per well) cells were seeded 24 hours before incubation with 300 nmol total lipid comprising empty fluorescent liposomes (PEG-lip, RAD-lip, or RGD-lip) for four hours at 4°C or 37°C. Flow cytometry analyses were performed with a FACSCalibur™ device (Becton Dickinson, Franklin Lakes, NJ; Software Cell Quest Pro, Heidelberg, Germany) equipped with an argon laser (488 nm). A total of 10,000 events per sample were recorded.
Cell viability
Cell viability was measured by MTS assay using the CellTiter96®aqueous non-radioactive cell proliferation assay kit (Promega). The inhibitory effect of EPO906 and the liposomal EPO906 formulations on cell viability was assessed in HUVEC, RH-30, and Kelly cells. The cells were seeded in 96-well plates (8000 HUVEC per well and 15,000 tumor cells per well) and incubated with EPO906 or the liposomal EPO906 formulations (from 0.1 nM to 100 nM) for four hours. Unbound liposomes were washed away three times, and the MTS assay was performed 48 or 72 hours later.
In vivo therapeutic efficacy studies
All experiments were conducted using protocols and conditions approved by the animal care and use committee at Freiburg’s University Medical Hospital. Female SCID bg/bg mice aged 5–6 weeks obtained from Charles River (Sulzfeld, Germany) were kept in groups of five animals per cage under normal conditions with access to food and water ad libitum. Tumors were induced by subcutaneous injection of Kelly cells or RH-30 cells (20 × 106 cells in 150 μL of phosphate-buffered solution in the right flank), and tumor growth and body weight were monitored three times weekly. Tumor sizes were determined according to the following formula: length × d2 × π/6; where “length” is the longest dimension and “diameter d” is the shortest dimension.
All treatments were started when the tumor size was 150–200 mm3. EPO906 and the liposomal EPO906 formulations were administered at different doses intravenously via the tail vein once weekly for four weeks. Intravenous injections of phosphate-buffered solution served as controls. Mice were euthanized after 28 days, if the tumor size exceeded 1000 mm3, or if loss of body weight reached 25%. In addition to changes in body weight, toxicity was assessed by scoring diarrhea, which is the dose-limiting toxicity of EPO906 observed in clinical trials. For this purpose, the mice were placed singly in a cage on white filter paper for 30 minutes, and diarrhea was graded from 0 (no diarrhea) in steps of 0.5 up to a maximum of grade 3 (liquid stool).
Statistical analysis
The results are presented as the mean ± standard error of the mean. The data were statistically analyzed using the Kruskal-Wallis H test, with the post hoc Mann-Whitney U test used to compare all groups. The differences in cumulative survival (Kaplan-Meier curves) were analyzed statistically using the log-rank test. P values < 0.05 were considered to be statistically significant.
Results
Integrin αVβ3 expression and selective targeting using RGD-liposomes in vitro
Semiquantitative reverse transcription PCR showed equal expression of integrin subunit αV in the HUVEC, Kelly, and RH-30 cell lines (), whereas expression of integrin β3 was much lower in Kelly cells compared with HUVEC and RH-30 cells. Stability of the particle size for EPO906-lip and EPO906-RGD-lip was investigated, and it was shown that the size for all preparations was stable over time ().
Figure 1 Integrin αV and β3 expression and integrin receptor targeting using RGD-liposomes in vitro. (A) Reverse transcription polymerase chain reaction for integrin αV and β3 fragments and the housekeeping gene β-actin in HUVEC, RH-30, and Kelly cells. (B–D) Association between RGD-liposomes and HUVEC (B), RH-30 (C), and Kelly cells (D).
Notes: HUVEC, RH-30, and Kelly cells were incubated with 300 nmol rhodamine-PE-labeled placebo liposomes (PEG-lip, RGD-lip, or RAD-lip) for four hours at 4°C and 37°C, and analyzed by flow cytometry. Data are presented as the percentage of fluorescent cells (mean ± standard error of the mean).
Abbreviations: lip, liposomes; RGD, cyclo-(Arg-Gly-Asp-D-Phe-Cys) peptide; RAD, cyclo-(Arg-Ala-Asp-D-Phe-Cys) peptide; HUVEC, human umbilical vein endothelial cells; PEG, poly(ethylene glycol).
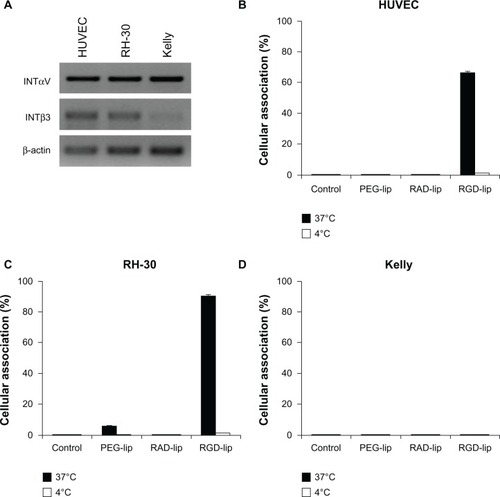
Flow cytometry analysis performed after four hours of incubation showed that RGD-lip were highly associated with HUVEC () and RH-30 () cells at 37°C, whereas no cellular association was observed with Kelly cells (). Moreover, none of the three cell lines showed an association with PEG-lip or RAD-lip, or with RGD-lip at 4°C, indicating high internalization of RGD-lip at 37°C. Therefore, using Kelly and RH-30 cells for xenograft tumors, we could study the effects of RGD-lip interacting with integrin αVβ3 present on endothelial and rhabdomyosarcoma cells, but missing on neuroblastoma tumor cells.
Inhibition of cell viability by EPO906 and liposomal EPO906 formulations
EPO906 and its liposomal formulations inhibited viability in a dose-dependent manner in the HUVEC, Kelly, and RH-30 cell lines (). No difference was observed between EPO906 and the liposomal formulations of any of the cell lines tested, but the efficacy of EPO906 and its liposomal formulations was more rapid and stronger in Kelly cells than in RH-30 cells, with inhibition of viability reaching 100% in Kelly cells after 48 hours at the highest dose tested (), whereas only 50% inhibition was observed in RH-30 cells after 72 hours at the highest dose tested ().
Figure 2 Inhibition of cell viability by EPO906 and EPO906 liposomal formulations in vitro. HUVEC (A and D), Kelly (B and E), and RH-30 (C and F) cells were incubated for four hours with EPO906 and EPO906 liposomal formulations (from 0.1 nM to 100 nM) and MTS assay was performed 48 hours or 72 hours later.
Note: Data are presented as the percentage of viable cells relative to untreated cells (mean ± standard error of the mean).
Abbreviations: lip, liposomes; RGD, cyclo-(Arg-Gly-Asp-D-Phe-Cys) peptide; RAD, cyclo-(Arg-Ala-Asp-D-Phe-Cys) peptide; HUVEC, human umbilical vein endothelial cells; EPO906, epothilone B; PEG, poly(ethylene glycol).
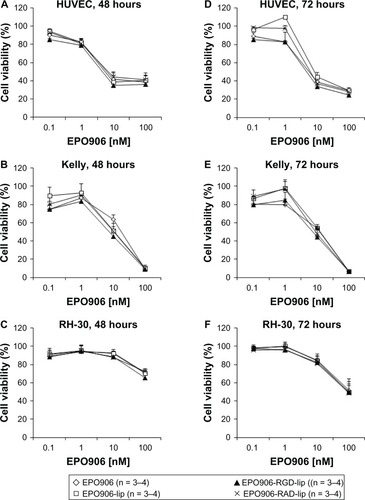
Inhibition of tumor growth by EPO906 and its liposomal formulations in a mouse model of neuroblastoma
Kelly xenograft tumors were grown in SCID mice until they reached 150–200 mm3. To define the most efficient and tolerated dose of EPO906 and liposomal EPO906 for further testing, we first treated mice with different doses of EPO906 and EPO906-lip (1.5 mg/kg, 2 mg/kg, 2.5 mg/kg, 3 mg/kg, ). No major differences in tumor growth were observed between the four doses used, indicating that 1.5 mg/kg was adequate to induce a potent antitumor effect. However, higher doses of EPO906 or EPO906-lip were associated with loss of body weight (), and diarrhea and mortality were proportionally increased ( and ).
Figure 3 Treatment of Kelly neuroblastoma xenografts with increasing doses (1.5 mg/kg to 3 mg/kg) of EPO906 and liposomal EPO906 formulations. A dose-finding study was performed to determine the optimal dose of EPO906 and EPO906-lip. Treatment with (A) 1.5 mg/kg, (B) 2 mg/kg, (C) 2.5 mg/kg, (D) and 3 mg/kg.
Notes: Tumors were induced by subcutaneous injection of 20 × 106 Kelly cells into SCID bg/bg mice. Treatment with EPO906 or EPO906-lip started when the tumor size reached 150–200 mm3, and was performed intravenously once a week for four weeks. Tumor size (mm3) and body weight (g) are presented as the mean ± standard error of the mean. *P < 0.05 versus PBS.
Abbreviations: EPO906, epothilone B; lip, liposomes; PBS, phosphate-buffered solution.
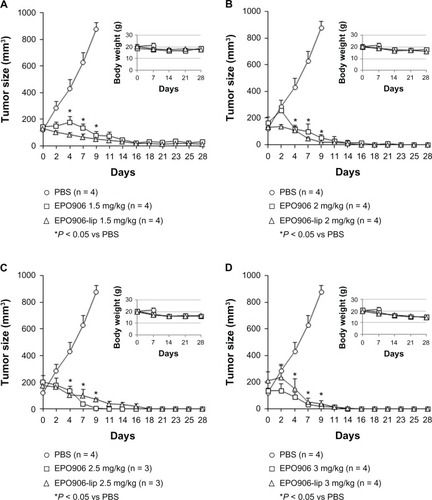
We further investigated the effects of 1.5 mg/kg EPO906 and its liposomal formulations. Treatment with EPO906 and the liposomal formulations rapidly and significantly inhibited tumor growth after two days (P < 0.01 versus controls, ). Moreover, body weight decreased slightly over time ().
Figure 4 Treatment of Kelly neuroblastoma xenografts with 1.5 mg/kg EPO906 and liposomal EPO906 formulations. (A) Tumor size (mm3), (B) body weight (g), and (C) cumulative survival (%).
Notes: Tumors were induced by subcutaneous injection of 20 × 106 Kelly cells into SCID bg/bg mice. Treatment with EPO906 or liposomal EPO906 formulations started when the tumor size reached 150–200 mm3, and was performed intravenously once a week for four weeks. The data are presented as the mean ± standard error of the mean. *P < 0.05 versus PBS; **P < 0.01 versus PBS.
Abbreviations: lip, liposomes; RGD, cyclo-(Arg-Gly-Asp-D-Phe-Cys) peptide; RAD, cyclo-(Arg-Ala-Asp-D-Phe-Cys) peptide; EPO906, epothilone B; PBS, phosphate-buffered solution.
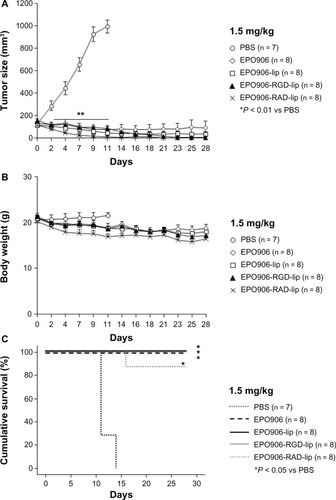
Cumulative survival was significantly increased in all the treatment groups compared with that in the control mice treated with phosphate-buffered solution, but no differences were observed between EPO906 and its liposomal formulations ().
Inhibition of tumor growth by EPO906 and liposomal EPO906 in a mouse model of rhabdomyosarcoma
RH-30 xenograft tumors were grown and treated as described above for the Kelly xenograft tumors.
Rapid tumor growth was seen in the control mice treated with phosphate-buffered solution (). In comparison, EPO906 and its liposomal formulations induced a statistically significant decrease in tumor growth four days after the start of therapy (P < 0.01 versus controls, ). This antitumor effect was more efficient when the animals were treated with EPO906-lip, and we had to stop evaluation of tumor size after 11 days of treatment with EPO906 and EPO906-RGD-lip because the tumors had reached 1000 mm3 in size. Further, body weight decreased slightly over time (). Cumulative survival was significantly increased in all the treatment groups compared with the control mice, but no differences were observed between EPO906 and its liposomal formulations ().
Figure 5 Treatment of RH-30 rhabdomyosarcoma xenografts with 1.5 mg/kg EPO906 and liposomal EPO906 formulations. (A) Tumor size (mm3), (B) body weight (g), and (C) cumulative survival (%).
Notes: Tumors were induced by subcutaneous injection of 20 × 106 RH-30 cells into SCID bg/bg mice. Treatment with EPO906 or liposomal EPO906 formulations was started when the tumor size reached 150–200 mm3, and was performed intravenously once a week for four weeks. The data are presented as the mean ± standard error of the mean. *P < 0.05 versus PBS; **P < 0.01 versus PBS.
Abbreviations: lip, liposomes; RGD, cyclo-(Arg-Gly-Asp-D-Phe-Cys) peptide; RAD, cyclo-(Arg-Ala-Asp-D-Phe-Cys) peptide; EPO906, epothilone B; PBS, phosphate-buffered solution.
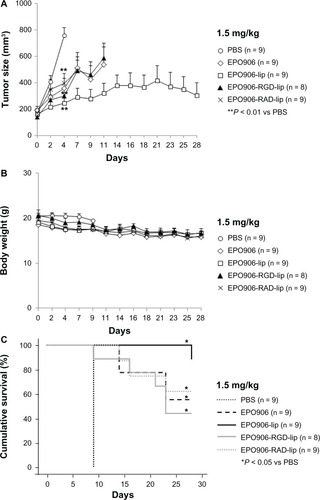
Inhibition of tumor growth and enhancement of cumulative survival by low-dose EPO906 and its liposomal formulations in a mouse model of rhabdomyosarcoma
The antitumor effect of 1.5 mg/kg EPO906 showed more potency when it was encapsulated in liposomes in the rhabdomyosarcoma model. Because EPO906 and its liposomal formulations were more toxic in the neuroblastoma model at higher doses, we oriented our study towards a low-dose therapy in rhabdomyosarcoma.
Low-dose (0.5 mg/kg and 1 mg/kg) EPO906 and its liposomal formulations were investigated. Treatment with 0.5 mg/kg showed no beneficial effects on tumor growth or survival (data not shown). With 1 mg/kg, most of the mice in the treatment groups were euthanized before the end of the 28-day investigation period because they reached our defined criteria for stopping therapy (tumor size > 1000 mm3). However, EPO906-RGD-lip induced faster and more potent tumor growth inhibition compared with the other formulations, but this was not observed at the dose of 1.5 mg/kg (). Body weight decreased slightly over time ().
Figure 6 Treatment of RH-30 rhabdomyosarcoma xenografts with 1 mg/kg EPO906 and liposomal EPO906 formulations. (A) Tumor size (mm3), (B) body weight (g), and (C) cumulative survival (%).
Notes: Tumors were induced by subcutaneous injection of 20 × 106 RH-30 cells into SCID bg/bg mice. Treatment with EPO906 or liposomal EPO906 formulations started when the tumor size reached 150–200 mm3, and was performed intravenously once a week for four weeks. The data are presented as the mean ± standard error of the mean. *P < 0.05 versus PBS; §P < 0.05 versus EPO906; #P < 0.05 versus EPO906-lip.
Abbreviations: lip, liposomes; RGD, cyclo-(Arg-Gly-Asp-D-Phe-Cys) peptide; RAD, cyclo-(Arg-Ala-Asp-D-Phe-Cys) peptide; EPO906, epothilone B; PBS, phosphate-buffered solution.
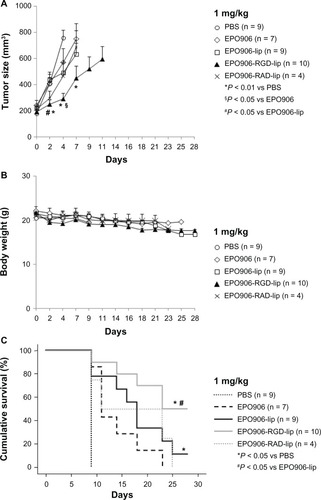
With regard to cumulative survival, use of low-dose EPO906 and its liposomal formulations resulted in death of most of the mice before the end of the treatment period, in accordance with our defined criteria for stopping therapy (), except in the EPO906-lip group where one of nine mice was still alive after 28 days (P < 0.05 versus controls, ) and in the EPO906-RGD-lip group where five of 10 mice were still alive after 28 days (P < 0.05 versus controls, ). Moreover, cumulative survival was significantly longer when mice were treated with EPO906-RGD-lip than when they were treated with EPO906 (P < 0.05 versus EPO906, ).
Discussion
Drugs that target microtubules are among the most commonly prescribed anticancer therapies. Vinca alkaloids, which depolymerize microtubules, are routinely administered in the treatment of pediatric solid tumors.Citation28 The role of taxanes in pediatric oncology is not as clear. Paclitaxel and docetaxel have achieved a very moderate response in pediatric solid tumors and brain tumors at relapse,Citation29,Citation30 which is in contrast with their activity in adult carcinomas. Epothilones have emerged as a new class of microtubule-targeting drugs with the ability to overcome some of the problems associated with taxane-based therapy, and have shown potent antitumor activity.Citation2,Citation10 However, little is known about their effect in pediatric cancers. Ixabepilone, an epothilone B derivative, showed a broad spectrum of activity against a panel of pediatric tumor xenograft models.Citation31 However; ixabepilone was inactive in a Phase II clinical trial in children and young adults with refractory solid tumors.Citation32
Our study was designed to investigate the potential antitumor effect of EPO906 in two pediatric tumor models. In addition, a liposomal formulation of EPO906 was developed and characterized in vitro and in vivo. Given that EPO906 has antivascular and antiangiogenic propertiesCitation7,Citation10 in addition to cytotoxicity in tumor tissue, we assumed that it could be a good candidate for active targeting of tumor endothelium. For this purpose, we modified liposomes by adding cyclic RGD-peptides to their surface, forming integrin-targeting liposomes. Because neuroblastoma and rhabdomyosarcoma are both known to be highly vascularized,Citation13,Citation14 we predicted an additional benefit from this antiangiogenic therapeutic approach in addition to the antitumor effect.
Our results using a 1.5 mg/kg dose demonstrate for the first time the potent antitumor activity of EPO906 in vivo in its native or liposomal form in neuroblastoma and to a more moderate extent in rhabdomyosarcoma. This study also shows the feasibility of encapsulation of liposomal EPO906. This procedure did not enhance drug cytotoxicity assessed in vitro but did not negatively affect it neither, and no differences were observed between the different formulations. Moreover, the extent of its toxicity to tumor cells in vitro was correlated with the antitumor effect of EPO906 in vivo, with a very strong and rapid effect in neuroblastoma, leading to complete tumor regression, whereas a more moderate and heterogeneous response was observed in rhabdomyosarcoma. EPO906 was well tolerated, with no high-grade diarrhea or significant body weight loss observed. In addition, explanted organs (ie, liver, spleen) at the end of treatment did not show any macroscopic damage. It has been demonstrated that EPO906 is rapidly distributed from plasma to all tissues and eliminated slowly, with a longer retention in tumor and brain tissue.Citation28 In the present study, pharmacokinetic profiling was not performed. It is important to point out that all our experiments were performed with EPO906 dissolved in PEG 300 as a control for the PEG-coated long-circulating liposomes encapsulating EPO906. We cannot exclude the possibility that this preparation prolongs the circulation time of EPO906 and increases its bioavailability in comparison with its nonpegylated form. This probably also explains the limitation of side effects compared with other studies.Citation28
Tumors generally have leaky blood vessels and poor lymphatic drainage. While free drugs may diffuse nonspecifically, a carrier molecule can extravasate into tumor tissue via leaky vessels by the enhanced permeability and retention effect.Citation19,Citation20 Tissue distribution using 111In-labeled pegylated liposomes, RGD-liposomes, and RAD-liposomes showed that their localization in tumor tissue was the same. However, circulating levels of RGD-liposomes were lower than those of RAD-liposomes and pegylated liposomes, demonstrating their specific interaction with the tumor vasculature.Citation17 Further, liposomes conjugated with RGD-peptides have been reported to target tumor endothelial cells without extravasation,Citation25 and are located at the rim of the tumor, whereas RAD-liposomes are found throughout the whole tumor.Citation33
In the neuroblastoma model, empty RGD-liposomes did not associate in vitro with Kelly cells, which have low expression of the integrin β3 subunit, essential for binding of the RGD ligand with the specific α-β active site pocket,Citation34 but we cannot exclude that RGD-liposomes bound to the tumor endothelium, as shown in vitro with HUVEC. However, their use to deliver EPO906 in neuroblastoma did not enhance the already strong antitumor effect of EPO906 or EPO906 encapsulated in PEG-liposomes. Moreover, the antitumor effects of EPO906-RAD-liposomes and EPO906-RGD-liposomes were exactly the same in vivo, while the effect of RAD-liposomes was expected to be the same as PEG-liposomes. Treatment of SCID mice with neuroblastoma using EPO906 dissolved in PEG 300 was already strong enough to inhibit tumor growth effectively, without triggering major side effects. In consequence, any effort to improve its delivery was not required. However, we did not treat the mice for longer than 28 days or monitor the animals after reducing and/or stopping the treatment.
Interestingly, in the mouse model of rhabdomyosarcoma, using RGD-liposomal EPO906 increased the antitumor effect as well as cumulative survival in the animals. Surprisingly, this beneficial effect was seen when we used a lower dose of EPO906 and its liposomal formulations. In general, cytotoxic agents are used at their maximum tolerated dose to induce rapid reduction of tumors, but this strategy often causes side effects and tumor resistance. Metronomic dosing, ie, continuous administration of low-dose chemotherapy over a protracted time course, targets the endothelial compartment of the tumor vasculature instead of having a direct cytotoxic effect on tumor cells. This ultimately deprives the tumor of its nutrient supply.Citation35 A recent study using a human tumor-derived angiogenesis model confirmed a beneficial effect of EPO906 on tumor angiogenesis when used at very low doses for a prolonged period of time.Citation36 In our study, use of low-dose EPO906-RGD-liposomes enhanced the antitumor effect and increased cumulative survival significantly compared with EPO906 and EPO906-lip. This result could be explained by the sensitivity of endothelial cells to low-dose chemotherapeutic drugs, ie, the metronomic chemotherapy dosing concept. Whether targeting liposomes improve uptake by cells in vivo is widely debated, but it seems that receptor-ligand interaction facilitates internalization and delivery of drugs into the cells.Citation37,Citation38 In our study, 1 mg/kg of RGD-liposomes showed greater endothelial cytotoxicity compared with PEG-liposomes, possibly because of a more appropriate ratio of integrins/RGD-ligands when a low dose was used, reducing the risk of receptor saturation and loss of receptor efficiency. Of note, the low dose of EPO906 (1 mg/kg) used in our study is 2.5–10 times lower than that described so far in preclinical studies.Citation28
Targeted drug delivery has been tested extensively in recent years. For example, targeted toxins are now available in brain tumor therapy and clinically approved nanoparticle-based cancer therapeutics,Citation39 including liposomal formulations of anthracyclines, cytarabine, the nab formulation of paclitaxel, and the polymeric nanoparticle formulation of paclitaxel.Citation40 However, in pediatric oncology, none of these has been studied in clinical trials nor approved by the regulatory authorities, although drug delivery exclusively to tumor cells is widely desired. Therefore, the RGD-liposomes described here could help to introduce these concepts, especially when treating young children and adolescents with cancer.
In conclusion, EPO906 showed a potent antitumor effect in vivo in two pediatric tumor models, ie, neuroblastoma and rhabdomyosarcoma. This effect was maintained when EPO906 was delivered to integrin-expressing cells using RGD-liposomes. Moreover, tumor targeting of EPO906 using RGD-liposomes enhanced its antitumor activity in rhabdomyosarcoma. Finally, the fact that low-dose EPO906-RGD-liposomes had a potent antitumor effect, in addition to significantly increasing cumulative survival, opens up new opportunities for targeting liposomes as low-dose therapeutics in pediatric oncology.
Acknowledgments
We would like to acknowledge Peter Nöllke for the statistical analyses, Leah Bury for her support with the in vitro experiments, Celine Simonin for preparation and characterization of the liposome batches, and Paul MJ McSheehy for his strong support and recommendations concerning the design of the in vivo experiments. Jochen Rössler and Regine Süss received a grant from the Deutsche Forschungsgemeinschaft (RO 1263/9-1, PE 708/3-1).
Supplementary materials
Figure S1 Particle size and polydispersity index of EPO906-lip and EPO906-RGD-lip over time.
Notes: EPO906-lip and EPO906-RGD-lip preparations were mixed with rat plasma (1:1) and stored at 37°C. Samples were taken at various time points to measure particle size and polydispersity index. In parallel, pure plasma was diluted in the same manner prior to measurement of size, and the plasma components showed an average diameter of 70 nm, which was about half the size of the liposomal structures. Therefore, we consider that the plasma components did not interfere with measurements of particle size or polydispersity index.
Abbreviations: EPO906, epothilone B; lip, liposomes; PI, polydispersity index; PEG, poly(ethylene glycol); RGD, cyclo-(Arg-Gly-Asp-D-Phe-Cys) peptide; RAD, cyclo-(Arg-Ala-Asp-D-Phe-Cys) peptide.
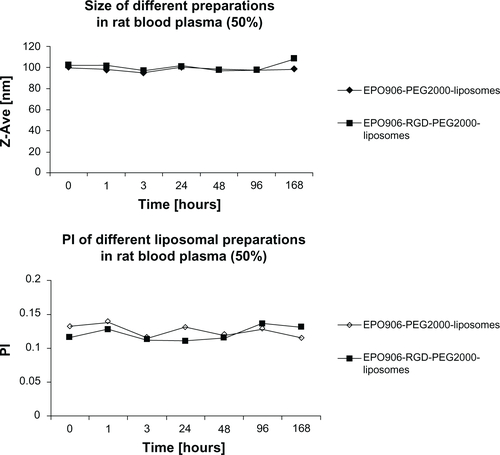
Figure S2 Effect of increasing doses of EPO906 and EPO906-lip on stool consistency in neuroblastoma (diarrhea score).
Notes: Tumor-bearing mice were singly placed in a cage on white filter paper for 30 minutes and diarrhea was graded from 0 (no diarrhea) in increments of 0.5 up to a maximum of grade 3 (liquid stool). Diarrhea was scored every 2–3 days. The results are presented as mean arbitrary units ± standard error of the mean.
Abbreviations: au, arbitary unit; EPO906, epothilone B; lip, liposomes; PBS, poly(ethylene glycol); SEM, standard error of the mean.
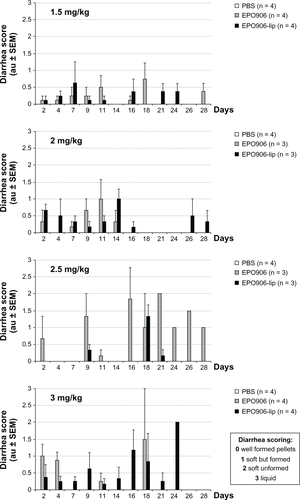
Table S1 Effect of increasing doses of EPO906 and EPO906-lip on survival of mice with neuroblastoma (dose-finding study) showing numbers of dead animals/treated animals
Disclosure
The authors report no conflicts of interest in this work.
References
- HöfeGBedorfNSteinmetzHSchomburgDGerthKReichenbachHEpothilone A and B – novel 16-membered macrolides and cytotoxic activity: isolation, crystal structure, and conformation in solutionAngew Chem Int Engl19963515671569
- BollagDMMcQueneyPAZhuJEpothilones, a new class of microtubule-stabilizing agents with a Taxol-like mechanism of actionCancer Res199555232523337757983
- AltmannKHWartmannMO’ReillyTEpothilones and related structures – a new class of microtubule inhibitors with potent in vivo antitumor activityBiochem Biophys Acta20001470M79M9110799747
- GoodinSKaneMPRubinEHEpothilones: mechanism of action and biologic activityJ Clin Oncol2004222015202515143095
- GiannakakouPSackettDLKangYKPaclitaxel resistant human ovarian cancer cells have mutant β-tubulins that exhibit impaired paclitaxel-driven polymerizationJ Biol Chem199527217118171259202030
- ThorpePEVascular targeting agents as cancer chemotherapeuticsClin Cancer Res20041041542714760060
- BocciGNicolaouKCKerbelRSProtracted low-dose effects on human endothelial cell proliferation and survival in vitro reveal a selective antiangiogenic window for various chemotherapeutic drugsCancer Res2002626938694312460910
- OehlerCvon BuerenAOFurmanovaPThe microtubule stabilizer patupilone (epothilone B) is a potent radiosensitizer in medulloblastoma cellsNeuroOncol20111310001010
- WolteringEALewisJMMaxwellPJ4thDevelopment of a novel in vitro human tissue-based angiogenesis assay to evaluate the effect of antiangiogenic drugsAnn Surg200323779079812796575
- FerrettiSAllegriniPRO’ReillyTPatupilone induced vascular disruption in orthotopic rodent tumor models detected by magnetic resonance imaging and interstitial fluid pressureClin Cancer Res2005117773778416278399
- SchrappeMReiterAZimmermannMLong-term results of four consecutive trials in childhood ALL performed by the ALL-BFM study group from 1981 to 1995. Berlin-Frankfurt-MünsterLeukemia2000142205222211187912
- MörickeAZimmermannMReiterALong-term results of five consecutive trials in childhood acute lymphoblastic leukemia performed by the ALL-BFM study group from 1981 to 2000Leukemia20102426528420010625
- ZwannCMKearnsPCaronHThe role of the ‘innovative therapies for children with cancer’ (ITCC) European consortiumCancer Treat Rev20103632833420231057
- RengaswamyVKontnyURösslerJNew approaches for pediatric rhabdomyosarcoma drug discovery: targeting combinatorial signalingExpert Opin Drug Discov201161103112522646865
- RösslerJTaylorMGeoergerBAngiogenesis as a target in neuroblastomaEur J Cancer2008441645165618614349
- AndréNVerschuurARosslerJSterbaJAnti-angiogenic therapies for children with cancerCurr Cancer Drug Targets20101087988920718702
- SchiffelersRMKoningGAten HagenTLMAnti-tumor efficacy of tumor vasculature-targeted liposomal doxorubicinJ Control Release20039111512212932643
- PloskerGLPegylated liposomal doxorubicin: a review of its use in the treatment of relapsed or refractory multiple myelomaDrugs2008682535255119016577
- PeerDKarpJMHongSFarokhzadOCMargalitRLangerRNanocarriers as an emerging platform for cancer therapyNat Nanotechnol2007275176018654426
- MaedaHWuJSawaTMatsumuraYHoriKTumor vascular permeability and the EPR effect in macromolecular therapeutics: a reviewJ Control Release20006527128410699287
- DechantsreiterMAPlankerEMathäEN-methylated cyclic RGD peptides as highly active and selective alpha(V)beta(3) integrin antagonistsJ Med Chem1999423033304010447947
- KokRJSchraaAJBosEJPreparation and functional evaluation of RGD-modified proteins as alpha(V)beta(3) integrin directed therapeuticsBioconjug Chem20021312813511792188
- DingemansAMvan den BoogaartVVosseBAvan SuylenRJGriffioenAWThijssenVLIntegrin expression profiling identifies integrin alpha5 and beta1 as prognostic factors in early stage non-small cell lung cancerMol Cancer2010915216020565758
- HynesROA reevaluation of integrins as regulators of experimental angiogenesisNat Med2002891892112205444
- MulderWJCastermansKvan BeijnumJRMolecular imaging of tumor angiogenesis using alphavbeta3-integrin targeted multimodal quantum dotsAngiogenesis200912172419067197
- SchönherrCToucheneSWilserGPeschka-SüssRFranceseGSimple and precise detection of lipid compounds present within liposomal formulations using a charged aerosol detectorJ Chromatogr20091216781786
- SchoenherrCHaefeleTPaulusKFranceseGConfocal Raman microscopy to probe content uniformity of a lipid based powder for inhalation: a quality by design approachEur J Pharm Sci200938475419501161
- O’ReillyTWartmannMBrueggenJPharmacokinetic profile of the microtubule stabilizer patupilone in tumor-bearing rodents and comparison of anti-cancer activity with other MTS in vitro and in vivoCancer Chemother Pharmacol2008621045105418301895
- HurwitzCARellingMVWeitmanSDPhase I trial of paclitaxel in children with refractory solid tumors: a Pediatric Oncology Group StudyJ Clin Oncol199311232423297902425
- MorrisPGFornierMNMicrotubule active agents: beyond the taxane frontierClin Cancer Res2008147167717219010832
- PetersonJKTuckerCFavoursEIn vivo evaluation of ixabepilone (BMS247550), a novel epothilone B derivative, against pediatric cancer modelsClin Cancer Res2005116950695816203787
- JacobsSFoxEKrailoMPhase II trial of ixabepilone administered daily for five days in children and young adults with refractory solid tumors: a report from the children’s oncology groupClin Cancer Res20101675075420068084
- MulderWJStrijkersGJHabetsJWMR molecular imaging and fluorescence microscopy for identification of activated tumor endothelium using a bimodal lipidic nanoparticleFASEB J2005192008201016204353
- HumphriesJDByronAHumphriesMJIntegrin ligands at a glanceJ Cell Sci20061193901390316988024
- BrowderTButterfieldCEKrälingBMAntiangiogenic scheduling of chemotherapy improves efficacy against experimental drug-resistant cancerCancer Res2000601878188610766175
- StalderMWAnthonyCTWolteringEAMetronomic dosing enhances the anti-angiogenic effect of epothilone BJ Surg Res201116924725620338584
- FedermanNDennyCTTargeting liposomes toward novel pediatric anticancer therapeuticsPediatr Res20106751451920118828
- XiongXBHuangYLuWLIntracellular delivery of doxorubicin with RGD-modified sterically stabilized liposomes for an improved antitumor efficacy: in vitro and in vivoJ Pharm Sci2005941782179315986461
- LiYMHallWATargeted toxins in brain tumor therapyToxins201022645266222069569
- WangAZLangerRSFarokhzadOCNanoparticle delivery of cancer drugsAnnu Rev Med20126318519821888516