Abstract
Objective
To observe the influence of Fe3O4-dextran-anti-β-human chorionic gonadotropin (HCG) carrying heparanase (Hpa) antisense oligodeoxynucleotide (ASODN), via the invasion, proliferation, and Hpa expression of JEG-3 cell lines and inhibitory effect of transplanted choriocarcinoma tumor growth.
Methods
The different abilities of invasion and proliferation between transfected JEG-3 and untransfected JEG-3 were measured by Matrigel invasion assay and 3-(4,5-dimethylthiazol-2-yl)-2,5-diphenyltetrazolium bromide assay in vitro. The effect of Hpa ASODN transfection on the expression of Hpa mRNA and protein was measured by reverse-transcription polymerase chain reaction and Western blot. The transplanted choriocarcinoma tumors were taken out to calculate the inhibitory effect on tumor growth of Hpa ASODN.
Results
In this study, we found that: (1) the invasive ability of JEG-3 cells was inhibited sufficiently (P < 0.05) after JEG-3 cells were transfected by Fe3O4-dextran-anti-βHCG carrying Hpa ASODN; (2) after JEG-3 cells were transfected by Fe3O4-dextran-anti-βHCG carrying Hpa ASODN at 48 and 72 hours, the proliferative ability of JEG-3 cells was inhibited sufficiently (P < 0.05); (3) the expression of Hpa mRNA and protein in JEG-3 cells was inhibited efficiently after JEG-3 cells were transfected by Fe3O4-dextran-anti-βHCG carrying Hpa ASODN (P < 0.05); and (4) Fe3O4-dextran-anti-βHCG carrying Hpa ASODN had an inhibitory effect on the transplanted choriocarcinoma tumor growth (P < 0.05) and was harmless on nude mice.
Conclusion
Fe3O4-dextran-anti-βHCG carrying Hpa ASODN weakened the invasive and proliferative ability of choriocarcinoma, with a significant inhibitory effect on the transplanted choriocarcinoma tumor. Therefore, Fe3O4-dextran-anti-βHCG carrying Hpa ASODN is an effective gene therapy, and Fe3O4-dextran-anti-βHCG nanoparticles are a harmless and effective gene vector.
Introduction
Heparanase (Hpa) is a mammalian endo-β-D-glucuronidase responsible for heparan sulfate degradation.Citation1 Hpa activity may therefore play an important role in fundamental biological processes associated with extracellular matrix remodeling and cell invasion.Citation2 In our study, we have found out that the expression of Hpa plays an important role in the metastatic capability of human choriocarcinoma.Citation3 Therefore, we deem that Hpa is the therapeutic target of choriocarcinoma and try to prove this hypothesis.
Gene therapy is the insertion of genes into an individual’s cells and tissues to treat a disease, and hereditary diseases in particular. Gene therapy typically aims to supplement a defective mutant allele with a functional one. Although the technology is still in its infancy, it has been used with some success. In antisense therapy, an antisense copy of the messenger RNA (mRNA) of the target gene is used to inhibit translation and production of the protein. In gene therapy, a carrier (vector) must be used to deliver the therapeutic gene to the patient’s target cells. Ideally, a gene-delivery system should be stable, biocompatible, nontoxic, cost-effective, and capable of transferring exogenous highly anionic genetic materials (ie, DNA, antisense oligonucleotides [ASODNs], short interfering RNA) into tissue-specific sites. A major goal of gene therapy is to obtain targeted vectors that transfer genes efficiently to specific cell types. The appearance of nanoparticles provides a new idea for gene vector. As a gene vector, nanoparticles possess several advantagesCitation4: (1) nanoparticles are not biologic materials, so they will not induce immune responses to human beings; (2) unlike viral carriers, nanoparticles have no genotoxicity or cytotoxicity; (3) nanoparticles are an effective gene vector; (4) nanoparticles can insert foreign genes in DNA in chromosomes of host cells, and as a result, foreign genes are expressed in host cells stably; (5) nanoparticles can defend foreign genes from complements, enzymes, etc; and (6) nanoparticles can counteract or kill some viruses. Having so many advantages, nanoparticles are potentially the most promising gene vector.
We have found out previously that Fe3O4-dextran-anti-β-human chorionic gonadotropin (HCG) nanoparticles represent a versatile vector for gene therapy with high stability towards degradation.Citation5 They combine a high introduction potential with good biocompatibility and low cytotoxicity. With magnetic and immune targeting, they offer the possibility of additional targeting strategies. In this study, we synthesized magnetic nanoparticles, and using those as a gene vector, we transfected Hpa ASODNs into choriocarcinoma JEG-3 cells. Afterwards, we estimated the curative effect of Hpa ASODNs on choriocarcinoma. We propose that the antitumor effect of Fe3O4-dextran-anti-βHCG nanoparticles carrying Hpa ASODN has been demonstrated as proof of principle.
Material and methods
Cells and animals
Cell-culture studies
JEG-3 and JAR are human choriocarcinoma cell lines, and were bought from the American Type Culture Collection (ATCC, Manassas, VA, USA). Both cell lines were cultured in Roswell Park Memorial Institute (RPMI) medium (Life Technologies, Carlsbad, CA, USA) supplemented with 10% fetal calf serum (FCS) in a humidified atmosphere of 5% CO2 and 37°C. Culture medium was replaced every other day.
Animals
Female BALB/c nude mice, 4 weeks old, were supplied and fed by the animal division of Central South University, Hunan, People’s Republic of China.
Preparation of magnetic nanoparticles of Fe3O4-dextran-anti-βHCG
Materials
Dextran with a molecular weight of 40,000 (T-40) and bovine serum albumin (BSA) were bought from Sigma-Aldrich, St Louis, MO, USA. Mouse anti-human βHCG monoclonal antibody was purchased from Wolwo Biotechnology, Shanghai, People’s Republic of China. FeCl3 · 6H2O, FeCl2 · 4H2O, and other chemicals were from local suppliers and were of analytical grade. Hpa ASODNs and non-sense oligonucleotides (NSODNs) were synthesized by Shanghai Sangon Biological Engineering Technology and Services (Shanghai, People’s Republic of China). Hpa ASODNs’ sequence was 5′-GGCTTCGAGCGCAGCAG CAT-3′, and Hpa NSODNs’ sequence was 5′-TCAGCTAGCGAGGCTGCGC A-3′. After being diluted to 100 Â μmol/L, all samples were preserved in −20°C. Mixtures of Fe3O4-dextran-anti-βHCG-Hpa ASODN or NSODN Fe3O4-dextran-anti-βHCG nanoparticles were prepared in a manner similar to Jingting et al.Citation4
Preparation of mixtures of Fe3O4-dextran-anti-βHCG-Hpa ASODNs or NSODNs
FeCl3 · 6H2O (0.552 g), FeCl2 · 4H2O (0.45 g), and dextran (5 g) were dispersed in 10 mL H2O. While under magnetism stirring with a velocity of 800 rpm, the mixture was titrated to alkaline by the dropwise addition of 10 mL 5 mol/L ammonia water and quickly heated to 60°C. After 30 minutes of reaction, the resulting black suspension was neutralized with acetic acid. Aggregates were removed by centrifugation for 10 minutes at 8,000 rpm. The dextran-coated iron oxide nanoparticles were separated from unbound dextran by magnetic separator and eluted with 10 mL sodium acetate (pH 6.5). The dextran-coated iron oxide suspension was oxidized with sodium periodate (NaIO4) under magnetism stirring in the dark at 4°C for 1 hour to obtain aldehyde termination, and the solution was dialyzed by magnetic separator again. Afterwards, 100 μg mouse anti-human βHCG monoclonal antibody and 200 μL 100 μmol/L Hpa ASODNs or NSODNs were put into 1 mL aldehyde dextran-coated iron oxide nanoparticles and mixed together fully. After being reacted in the dark at 4°C for 24 hours, the mixture was blocked by 50 μL 10 wt% BSA. For improved stability, 1 mol/L NaBH4 was added to the compound at 4°C for 3 hours and stirred. Lastly, the suspension was centrifuged for 20 minutes at 13,000 rpm three times. The production was dispersed again by 100 μL NaBO3 (pH 8.5).
Introduction of Hpa ASODN or NSODN to JEG-3 cells mediated by Fe3O4-dextran-anti-βHCG nanoparticles
When JEG-3 cells had grown to 70%–80%, the cells were washed with free Dulbecco’s Modified Eagle’s Medium (DMEM) (Sigma-Aldrich), and then 4.5 mL DMEM that contained 10% FCS and 500 μL Fe3O4-dextran-anti-βHCG-Hpa ASODN or NSODN compounds, which contained 20 μmol/L Hpa ASODN or NSODN in every culture bottle (37.5 μg/mL Fe3+ and 100 μg/mL mouse anti-human βHCG monoclonal antibody in introduction reagent). After the introduction (24 hours), the cells were used for later experimentation.
MTT assay
Transfected and untransfected JEG-3 cells were seeded in 96-well plates (5,000 cells/well) in DMEM containing 10% FCS. After 24, 48, and 72 hours, MTT (3-[4,5-dimethylthiazol-2-yl]-2,5-diphenyltetrazolium bromide; Sigma-Aldrich) was added to the culture medium at a concentration of 5 mg/mL for 4 hours at 37°C. Subsequently, the cells were washed with phosphate-buffered saline, and the purple formazan was solubilized in 100% dimethyl sulfoxide. Absorbance was read at 570 nm. Five separate sets of experiments were performed and averaged.
Matrigel invasion assay
A Matrigel invasion assay was prepared as described in Staff et al.Citation6 Briefly, diluted 1:2 Matrigel (1.75 μg/μL; BD Biosciences, Beit Ha’Emek, Israel) in serum-free cell-culture media was added to the upper chamber of a 24-well transwell plate and incubated at 37°C for 3–4 hours for gelling. Transfected and untransfected JEG-3 cells were harvested from tissue-culture flasks by trypsin/ethylenediaminetetraacetic acid, washed and resuspended in 0.1% FCS in DMEM, and added to upper wells at a density of 105 cells/well in 100 μL medium, while 600 μL medium was added to the lower wells. Plates were incubated at 37°C for 24 hours, and then the cells remaining on the upper surface of the membrane were removed with a cotton swab and the filters fixed by 95% ethanol for 30 minutes. Cells that had invaded the lower surface of the filter were counted under an inverted microscope; ten fields per well were counted. All experiments were performed five times, and the results from the five separate sets of experiments were averaged.
Reverse-transcription polymerase chain reaction
RNA extraction
The commercially available Trizol reagent (Life Technologies, Carlsbad, CA, USA) was used to extract total RNA from cells. In brief, cells were milled and lysed in 1 mL of Trizol and left at room temperature for 5–10 minutes. Subsequently, 0.2 mL of chloroform was added, followed by vigorous agitation for 15 seconds. The sample mixture was kept at room temperature for 2–3 minutes, and then centrifuged at 12,000 g for 15 minutes at 4°C. The aqueous phase was incubated with 0.5 mL of isopropanol for 10 minutes at room temperature, and then centrifuged at 12,000 g for 10 minutes at 4°C. The precipitated RNA was washed with 75% ethanol and dissolved in 0.1% diethylpyrocarbonate-treated water. RNA concentrations were measured spectrophotometrically (1 OD260 40 mg/mL of RNA).
Reverse-transcription polymerase chain reaction
The expression levels of Hpa and glyceraldehyde-3-phosphodehydrogenase (GAPDH) genes were determined by reverse-transcription polymerase chain reaction (RT-PCR). Total RNA was reverse-transcribed in 30 mL of a solution containing 1 μg of total RNA random hexamer primers, 5× reaction buffer, RiboLock RNase inhibitor, 10 mM deoxyribonucleotide mix, Revert Aid Moloney murine leukemia virus reverse transcriptase, and diethylpyrocarbonate-treated water (Thermo Fisher Scientific, Waltham, MA, USA). PCR was performed to detect the expression of Hpa mRNA in transfected and untransfected JEG-3 cells. Based on published DNA sequences of human HPA and GAPDH genes, primers for HpaCitation1 (sense, HPU-355 5′-TTCGATCCCAAG AAGGAATCAAC-3′, antisense, HPL-229 5′-GTAGTGATGCCATGTAACTGAATC-3′) and GAPDH (sense, 5′GCTGGCGCTGAGTACGTCGT-3′, antisense, 5′–TGGGTGTCGCTGTTGAAGTC-3′) were obtained. PCR was performed using an MJ Research (Bio-Rad, Waltham, MA, USA) PCR system. The following conditions were applied to Hpa PCR amplifications: 94°C for 5 minutes, 38 cycles (denaturation at 94°C for 45 seconds, annealing at 55°C for 45 seconds, extension at 72°C for 1 minute), and 72°C for 10 minutes. The conditions for GAPDH PCR amplifications were 94°C for 5 minutes, 35 cycles (denaturation at 94°C for 45 seconds, annealing at 55°C for 50 seconds, extension at 72°C for 1 minute), and 72°C for 10 minutes. Both reactions were in 25 mL mixtures containing 5 μL of the reverse-transcription mix, 10× Taq buffer with KCl, 25 mM MgCl2, 10 mM deoxyribonucleotide mix, Taq DNA polymerase, 10 pmol/L of each sense and antisense primer, and ddH2O (Takara Bio, Otsu, Japan). Aliquots (10 μL) of the amplification products were resolved by 1.5% agarose gel (Promega, Fitchburg, WI, USA) electrophoresis and visualized by ethidium bromide staining. The fragment size and signal intensity were analyzed by GeneScan Analysis and GeneScan Genotyper software (Life Technologies).
Western blotting
Extracting proteins from tissues
Proteins were extracted in a homogenate suspension buffer consisting of 10 mM Tris-HCl, pH 7.6, 100 mM NaCl, and a protease-inhibitor cocktail (Complete; Roche Diagnostics, Rotkreuz, Switzerland). Protein concentration was measured with a BCA protein assay (Thermo Fisher Scientific).
Western blotting
About 50 μg of protein extract was subjected to sodium dodecyl sulfate–polyacrylamide gel electrophoresis and transferred to nitrocellulose filters by electroblotting. Nonspecific binding was blocked by incubating the membranes in 5% nonfat milk in TBS-T (20 mM Tris–HCl, 150 mM NaCl, 0.1% Tween-20). Membranes were incubated with the rabbit anti-Hpa polyclonal antibody (1:100 in a dilution) (Boster Biotechnology, Wuhan, People’s Republic of China) overnight at 4°C. Membranes were then washed with TBS-T and incubated with a horseradish peroxidase-conjugated rabbit antigoat immunoglobulin G (1:2000 dilution) (Boster Biotechnology) for 1 hour at room temperature. Signals were detected by enhanced chemiluminescence (GE Healthcare, Piscatawy, NJ, USA) according to the instructions of the manufacturer, and the data were analyzed using the Un-Scan-It program (Silk Scientific, Orem, UT, USA). The same membrane was reprobed with β-actin-specific antibody to ensure equal control.
Inhibitory effect of Fe3O4-dextran-anti-βHCG-Hpa ASODN on hypodermal transplant tumors in nude mice
Cultured JEG-3 cells were harvested and resuspended in unsupplemented RPMI medium at 4–6 × 106 cells/mL, then 100 μL was immediately injected into the flanks of 20 nude mice. The mice were divided into four groups, five in each, and further growth of induced tumors was monitored for 10 days. Tumor diameters measured 8–10 mm in all groups. Four different types of solutions were injected hypodermally in each nude mouse: group 1, 0.2 mL Fe3O4-dextran-anti-βHCG-Hpa ASODN solutions, which contained 20 μmol/L Hpa ASODN; group 2, 0.2 mL Fe3O4-dextran-anti-βHCG-Hpa NSODN solutions, which contained 20 μmol/L Hpa NSODN; group 3, 0.2 mL Fe3O4-dextran-anti-βHCG solutions; and group 4, 0.2 mL saline control. These solutions were injected every 2 days into all nude mice for 20 days (ten times) aggregately. We weighed the transplanted tumor of every nude mouse. Inhibition rate (%) = ([the weight of transplant tumor of control group − the weight of transplant tumor of curing group]/the weight of transplant tumor of control group) × 100%.
Statistical analysis
Results were shown as means ± standard error of mean. The statistical significance of differences in mean values was assessed by Student’s t-test or one-way analysis of variance followed by the Dunnett’s multiple-comparison test using SAS software (SAS Institute, Cary, NC, USA). Differences among means were considered significant at P < 0.05.
Results
Effect of Fe3O4-dextran-anti-βHCG-Hpa ASODN compounds on proliferative ability of JEG-3
As shown in , after 24 hours there were not any significant differences among proliferative abilities of untransfected Hpa NSODN and Hpa ASODN JEG-3 cells (P > 0.05). At the same time, we found that after 48 and 72 hours, the proliferative ability of Hpa ASODN JEG-3 cells was obviously inhibited (P < 0.05), whereas there were not any significant differences between the untransfected group and the Hpa NSODN group (P > 0.05).
Table 1 Difference in proliferative ability of untransfected and transfected JEG-3 cells
Effect of Fe3O4-dextran-anti-βHCG-Hpa ASODN compounds on invasive ability of JEG-3 cells
As shown in , invasive ability was decreased in JEG-3 cells treated by Fe3O4-dextran-anti-βHCG-Hpa ASODN (the average cell number on the lower chamber was 109 ± 10) compared to Hpa NSODN (213 ± 18) and untransfected control JEG-3 cells (221 ± 25) (P < 0.01). There were not any significant differences between Fe3O4-dextran-anti-βHCG-Hpa NSODN-treated JEG-3 cells and untransfected control JEG-3 cells (P > 0.05).
Figure 1 (A–C) Effects of Fe3O4-dextran-anti-β-human chorionic gonadotropin (HCG) carrying heparanase (Hpa) antisense oligodeoxynucleotide (ASODN) on JEG-3 cell invasion. (A) Untransfected cells. Cells on the lower surface of the insert chamber were counted under microscope with 80× magnification. (B) Fe3O4-dextran-anti-βHCG-Hpa ASODN-treated cells. (C) Fe3O4-dextran-anti-βHCG-Hpa NSODN-treated cells.
Abbreviation: NSODN, non-sense oligonucleotides.
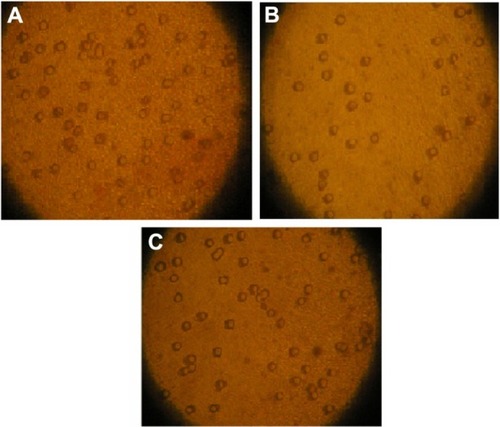
Effect of Fe3O4-dextran-anti-βHCG-Hpa ASODN compounds on Hpa mRNA expression in JEG-3 cells
As shown in , there was an almost 2.7-fold decrease in Hpa mRNA in JEG-3 cells when these cells were treated with Hpa ASODN (0.481 ± 0.04 vs 1.288 ± 0.12, P < 0.01). This decrease in Hpa mRNA is sequence-specific because the NSODN-treated JEG-3 cells produced equivalent amounts of Hpa mRNA transcripts (1.117 ± 0.10 vs 1.288 ± 0.12, P > 0.05).
Figure 2 (A–C) Effect of Fe3O4-dextran-anti-β-human chorionic gonadotropin (HCG) carrying heparanase (Hpa) antisense oligodeoxynucleotide (ASODN) compounds on Hpa messenger RNA (mRNA) expression in JEG-3 cells. (A) Hpa mRNA expression in JEG-3 cells. (B) Hpa mRNA expression in JEG-3 cells after been treated with Fe3O4-dextran-anti-βHCG-Hpa ASODNs. (C) Hpa mRNA expression in JEG-3 cells after been treated with Fe3O4-dextran-anti-βHCG-Hpa NSODNs.
Abbreviations: GAPDH, glyceraldehyde-3-phosphodehydrogenase; NSODN, non-sense oligonucleotides.
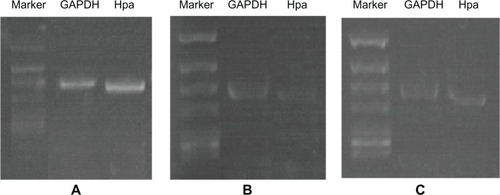
Effect of Fe3O4-dextran-anti-βHCG-Hpa ASODN compounds on Hpa protein expression in JEG-3 cells
Confirmation of decreased translation of Hpa protein was demonstrated by Western blot analysis. As shown in , Hpa protein decreased in JEG-3 cells treated with magnetic nanoparticle Hpa ASODNs (16.1 ± 1.4) compared to Fe3O4-dextran-anti-βHCG-Hpa NSODNs (35.3 ± 3.2) and untransfected control JEG-3 cells (39.7 ± 2.9) (P < 0.05). But there was no significant difference between Hpa protein in JEG-3 cells treated with Fe3O4-dextran-anti-βHCG-Hpa NSODNs and that in untransfected control JEG-3 cells (P > 0.05).
Figure 3 (A–C) Effect of Fe3O4-dextran-anti-β-human chorionic gonadotropin (HCG) carrying heparanase (Hpa) antisense oligodeoxynucleotide (ASODN) compounds on Hpa protein expression in JEG-3 cells. (A) Hpa protein expression in JEG-3 cells. (B) Hpa protein expression in Fe3O4-dextran-anti-βHCG-Hpa ASODN-treated cells. (C) Hpa protein expression in Fe3O4-dextran-anti-βHCG-Hpa nonsense oligonucleotides-treated cells.
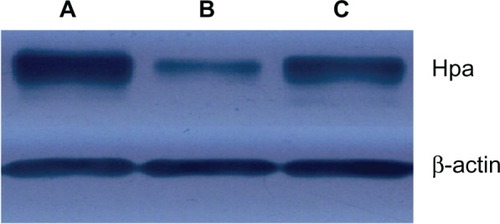
Effect of Fe3O4-dextran-anti-βHCG-Hpa ASODN on hypodermal transplant tumors of nude mice
As shown in , after being treated by Fe3O4-dextran-anti-βHCG-Hpa ASODN, the growth of hypodermal transplant tumors was obviously inhibited (P < 0.01; the average weight was 0.615 ± 0.061 g). The inhibitory rate was 58.6%, and compared with the control group (the average weight was 1.487 ± 0.159 g), there was a significant difference (P < 0.01). The average weight of hypodermal transplant tumors in the Fe3O4-dextran-anti-βHCG-Hpa NSODN group and the Fe3O4-dextran-anti-βHCG nanoparticle group was 1.417 ± 0.136 g and 1.428 ± 0.145 g, respectively, and their inhibitory rates were 4.7% and 4.0%, respectively. Compared with the control group, the growth of hypodermal transplant tumors of the Fe3O4-dextran-anti-βHCG-Hpa NSODN group and the Fe3O4-dextran-anti-βHCG nanoparticle group was not obviously inhibited (P > 0.05).
Figure 4 (A–D) Effect of Fe3O4-dextran-anti-β-human chorionic gonadotropin (HCG) carrying heparanase (Hpa) antisense oligodeoxynucleotide (ASODN) on hypodermal transplant tumors in nude mice. (A) Saline solution-treated hypodermal transplant tumor. (B) Fe3O4-dextran-anti-βHCG nanoparticle-treated hypodermal transplant tumor. (C) Fe3O4-dextran-anti-βHCG-Hpa nonspecific oligodeoxynucleotide-treated hypodermal transplant tumor. (D) Fe3O4-dextran-anti-βHCG-Hpa ASODN-treated hypodermal transplant tumor.
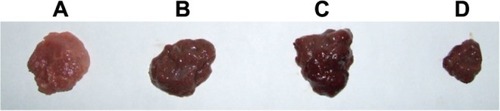
Discussion
The key role played by Hpa in malignancy was confirmed by antisense complementary DNA introduction studies that showed a significant reduction in the invasive and metastatic properties of tumor cells.Citation7 Expression of an anti-Hpa ribozyme construct in human MDA-MB-435 breast carcinoma cells significantly reduced Hpa enzyme activity as well as invasion through a Matrigel basement membrane.Citation8 A large number of publications now clearly link Hpa expression to the process of tumorigenesis in a wide range of cancers, including bladder,Citation9 colon,Citation10 gastric,Citation11 breast,Citation12 oral,Citation13 esophageal,Citation14 pancreatic,Citation15 brain,Citation16 thyroid,Citation17 prostate,Citation18 and acute myeloid leukemia.Citation19 Collectively, this evidence suggests that Hpa plays a fundamental role in sustaining the pathology of malignant diseases, and therefore that it may provide a potential target for anticancer therapy.
Human gene therapy is one of those new therapeutic approaches emerging from this molecular biology and biotechnology revolution.Citation20 ASODN is one gene-therapy approach; it offers the ability to regulate genes involved in cancer progression, especially those that are not amenable to small-molecule or antibody inhibition.Citation21 ASODNs are designed to be complementary to a selected gene’s mRNA and thereby specifically inhibit expression of that gene. It is estimated that any sequence of at least 13 bases in RNA and 17 bases in DNA is represented only once within the human genome. Thus, the specificity implicit in the design of ASODNs theoretically leads to decreased off-target toxicity. ASODN technology enables rapid movement from preclinical models to testing in the clinic. Challenges remain to optimize tissue exposure and cellular uptake, and to demonstrate mechanisms and antitumor activity. Therefore, it is very important for us to find a targeted vector.
Magnetic Fe3O4 nanoparticles are considered ideal gene vectors, because they are simple to manufacture, have diameters of 10 nm, are able to absorb significant amounts of DNA, demonstrate limited cellular toxicity, and can be guided to specific sites via magnets. In addition, to improve biocompatibility, Fe3O4 nanoparticles can be embedded in various macromolecules, such as polysaccharides and proteins. However, human organs, such as liver, spleen, and lymph (among others), are infused with a reticuloendothelial system and have the ability to absorb nanoparticles passively through phagocytosis. Because βHCG is expressed only in choriocarcinomas, anti-βHCG monoclonal antibodies were attached to magnetic particles to allow the nanoparticles to target the tissues of interest.
We have confirmed that magnetic nanoparticles represent a versatile vector for gene therapy, with high stability towards degradation. They combine a high introduction potential with good biocompatibility and low cytotoxicity. With magnetic and immune targeting, they offer the possibility of additional targeting strategies.Citation5 In this study, we used MTT and Matrigel invasion assay to detect changes in the proliferative and invasive abilities of choriocarcinoma JEG-3 cells after being treated by magnetic nanoparticle Hpa ASODN compounds. The results revealed that the invasive ability of JEG-3 cells was inhibited, and that after 48 and 72 hours, the proliferative ability was also obviously inhibited. Subsequently, we found that magnetic nanoparticle Hpa ASODN compounds can weaken the expression of Hpa mRNA and protein. Finally, we tested the effect of magnetic nanoparticle Hpa ASODN compounds on choriocarcinoma hypodermal tumors of nude mice. The results indicated that magnetic nanoparticle Hpa ASODN compounds can inhibit the growth of choriocarcinoma tumors. All of these results were identical with expectations. However, an unexpected outcome was the finding that proliferative ability after 24 hours was not affected. It was difficult for us to explain this result. Our initial view was that magnetic nanoparticle Hpa ASODN compounds did not produce any effect on JEG-3 cell proliferation after 24 hours.
Transfected by Fe3O4-dextran-anti-βHCG magnetic nanoparticles, Hpa ASODNs inhibited the expression of JEG-3 cells’ Hpa directly, which hints that Fe3O4-dextran-anti-βHCG magnetic nanoparticles are a kind of effective gene vector. Subsequently, we found that the invasive and proliferative abilities of JEG-3 cells and the growth of choriocarcinoma hypodermal tumors of nude mice had been obviously controlled, and this result should be connected with the inhibition of Hpa expression. The reason is that the growth of tumors is not only relevant to the proliferation of tumor cells but is also associated with the invasion of cells. If tumor cells want to invade surrounding organs, it is necessary for them to dissolve extracellular matrix. Thus when the expression of Hpa is inhibited, the solvency of tumor cells is weakened, and so the growth of tumor cells is inhibited also. On the other hand, we found that magnetic nanoparticle Hpa ASODN compounds do not have any toxicity to nude mice, so it is reasonable for us to consider this as a safe and effective tumor remedy.
Acknowledgments
This project was supported by the National Natural Science Foundation of China (grant 81101995) and the Natural Science Foundation of Hunan Province (grant 13JJ4120).
Disclosure
The authors report no conflicts of interest in this work.
References
- VlodavskyIFriedmannYElkinMMammalian heparanase: gene cloning, expression and function in tumor progression and metastasisNat Med1999579380210395325
- VlodavskyIFriedmannYMolecular properties and involvement of heparanase in cancer metastasis and angiogenesisJ Clin Invest200110834134711489924
- JingtingCYangdeZYiZHuiningLRongYYuZHeparanase expression correlates with metastatic capability in human choriocarcinomaGynecol Oncol2007107222917688924
- ZhangYDNanobiotechnologyBeijingScientific Publishing2005
- JingtingCHuiningLYiZPreparation and characterization of magnetic nanoparticles containing Fe3O4-dextran-anti-β-human chorionic gonadotropin, a new generation choriocarcinoma-specific gene vectorInt J Nanomedicine2011628529421383853
- StaffACRanheimTHenriksenTHalvorsenB8-Iso-prostaglandin F2α reduces trophoblast invasion and matrix metalloproteinase activityHypertension2000351307131310856282
- UnoFFujiwaraTTakataYAntisense-mediated suppression of human heparanase gene expression inhibits pleural dissemination of human cancer cellsCancer Res2001617855786011691803
- EdovitskyEElkinMZchariaEPeretzTVlodavskyIHeparanase gene silencing, tumor invasiveness, angiogenesis, and metastasisJ Natl Cancer Inst2004961219123015316057
- GohjiKOkamotoMKitazawaSHeparanase protein and gene expression in bladder cancerJ Urol20011661286129011547059
- SatoTYamaguchiAGoiTHeparanase expression in human colorectal cancer and its relationship to tumor angiogenesis, hematogenous metastasis, and prognosisJ Surg Oncol20048717418115334632
- TakaokaMNaomotoYOhkawaTHeparanase expression correlates with invasion and poor prognosis in gastric cancersLab Invest20038361362212746471
- ZhangYLFuZRChenXYYangJHWangYHShenQThe inhibitory effect of heparanase antisense oligodeoxynucleotides on the invasiveness of human mammary carcinoma cell lineZhonghua Shi Yan Wai Ke Za Zhi20043367369
- IkutaMPodymaKAMaruyamaKEnomotoSYanagishitaMExpression of heparanase in oral cancer cell lines and oral cancer tissuesOral Oncol20013717718411167146
- MikamiSOhashiKUsuiYLoss of syndecan-1 and increased expression of heparanase in invasive esophageal carcinomasJpn J Cancer Res2001921062107311676857
- KimAWXuXHollingerEFGattusoPGodellasCVPrinzRAHuman heparanase-1 gene expression in pancreatic adenocarcinomaJ Gastrointest Surg2002616717211992801
- MarchettiDNicolsonGLHuman heparanase: a molecular determinant of brain metastasisAdv Enzyme Regul20014134335911384754
- XuXQuirosRMMaxhimerJBInverse correlation between heparan sulfate composition and heparanase-1 gene expression in thyroid papillary carcinomas: a potential role in tumor metastasisClin Cancer Res200395968597914676122
- OgishimaTShiinaHBreaultJEIncreased heparanase expression is caused by promoter hypomethylation and up-regulation of transcriptional factor early growth response-1 in human prostate cancerClin Cancer Res2005111028103615709168
- VlodavskyIGoldshmidtOZchariaEMammalian heparanase: involvement in cancer metastasis, normal development and angiogenesisSemin Cancer Biol20021212112912027584
- AndersonWFHuman gene therapyNature199839225309579858
- GleaveMEMoniaBPAntisense therapy for cancerNat Rev Cancer2005546847915905854