Abstract
Background
The aim of this study was to synthesize Gd3+-based silica nanoparticles that conjugate easily with glucosamine and to investigate their use as a nanoprobe for detection of human fibrosarcoma cells.
Methods
Based on the structure of the 2-fluoro-2-deoxy-D-glucose molecule (18FDG), a new compound consisting of D-glucose (1.1 nm) was conjugated with a Gd3+-based mesoporous silica nanoparticle using an N-5-azido-2-nitrobenzoyloxy succinimide (ANB-NOS) crosslinker The contrast agent obtained was characterized using a variety of methods, including Fourier transform infrared spectroscopy, nitrogen physisorption, thermogravimetric analysis, scanning and transmission electron microscopy, and inductively coupled plasma atomic emission spectrometry (ICP-AES). In vitro studies included cell toxicity, apoptosis, tumor necrosis factor-alpha, and hexokinase assays, and in vivo tests consisted of evaluation of blood glucose levels using the contrast compound and tumor imaging. The cellular uptake study was validated using ICP-AES. Magnetic resonance relaxivity of the contrast agent was determined using a 1.5 Tesla scanner.
Results
ANB-NOS was found to be the preferred linker for attaching glucosamine onto the surface of the mesoporous silica nanospheres. The r1 relaxivity for the nanoparticles was 17.70 mM−1s−1 per Gd3+ ion, which is 4.4 times larger than that for Magnevist® (r1 approximately 4 mM−1s−1 per Gd3+ ion). The compound showed suitable cellular uptake (75.6% ± 2.01%) without any appreciable cytotoxicity.
Conclusion
Our results suggest that covalently attaching glucosamine molecules to mesoporous silica nanoparticles enables effective targeted delivery of a contrast agent.
Introduction
Targeted delivery of a contrast agent mediated by functionalized nanoparticles is expected to improve imaging of cancer cells, enabling rapid diagnosis of the disease in its early stages.Citation1–Citation3 In the past few years, mesoporous silica nanoparticles (MSNs) have emerged as an ideal nanoplatform in view of their biocompatibility and biodegradability Numerous studies of the biocompatibility and toxicity of MSNs have been reported.Citation1–Citation4 MSNs have a high surface area and large pore volumes for pay loading of magnetic centers and tunable particle size for efficient cellular uptake.Citation5–Citation11 There has been interest in developing smart MSN nanocarriers with external and internal surfaces that can be selectively functionalized with multiple groups.Citation5,Citation7–Citation12 Contrast agents could also be covalently linked to these particles via cleavable bonds.Citation5,Citation9,Citation13,Citation14 Further, abundant silanol groups facilitate modification on their surfaces following synthesis. Most recently, surface-functionalized MSNs using cancer-specific agents have also been used for selectively targeting cancer cells.Citation15 Antibodies, peptides, aptamers,Citation16 carbohydrates,Citation17 and folic acidCitation18 are some of these agents. Glucose analogs are excellent agents for diagnosis of cancer, showing excessive cellular uptake of glucose due to overexpression of glucose transporters in cancer cells. Moreover, glucose consumption in these cells is very high because of their high rate of proliferation and angiogenesis.Citation19 Over the past decade, surface scientists have found that click reactions are powerful tools for binding functional groups onto a surface.Citation20,Citation21 Clickable materials are species with click reaction capability, such as azide or alkyne groups. Click reactions are suitable for functionalization of silicon wafers as well as silicon rugate filters and the surface of mesoporous silica.Citation22 Some research work on clickable mesoporous silica nanoparticles has recently been reported.Citation23–Citation26
3-Aminopropyl trimethoxysilane (APTES) is an organosilane that is widely used for attaching targeting groups to the surfaces of MSNs. However, APTES does not contain a reactive functional group that could be used for covalent bonding with glucosamine. A stronger linkage could be achieved by attaching a heterobifunctional crosslinker, such as N-5-azido-2-nitrobenzoyloxy succinimide (ANB-NOS), to APTES for binding to glucosamine. ANB-NOS can covalently bind glucosamine through its aryl azide group following activation with ultraviolet light.Citation27
This paper reports a simple novel method for synthesizing a nanoprobe containing MSNs loaded with Gd3+ and glucosamine (MSN-Gd3+-DG) as a selective molecular imaging agent for human fibrosarcoma cells. Our in vitro and in vivo studies of conjugation of MSNs with glucosamine and Gd3+ loading indicate a novel nanoprobe with potential imaging effects in cancer cells.
Materials and methods
Materials
GdCl3 · 6H2O (99%), tetraethylorthosilicate (98%), methanol, anhydrous ethanol (99.5%), sodium hydroxide (NaOH), bromoacetic acid, cetyltrimethylammonium bromide (98%), APTES (99%), 3-(trimethoxysilylpropyl) diethylene triamine, anhydrous N,N dimethyl formamide (99.8%), toluene (99.8%), [4-(2-hydroxyethyl)-1-piperazineethanesulfonic acid] (HEPES), Tween 20, phosphate-buffered saline, and Infinity™ glucose reagent were purchased from Sigma-Aldrich (Seelze, Germany) and used without further purification. 3-(trimethoxysilylpropyl) diethylenetriamine was obtained from Gelest Inc (Morrisville, PA, USA). A dialysis bag with a 500–1000 Da cutoff was obtained from Spectrum Labs (Torrance, CA, USA). ANB-NOS was purchased from Pierce (Rockford, IL, USA). Other materials were sourced from Merck (Frankfurt, Germany). Fetal bovine serum and penicillin-streptomycin were also purchased from Sigma-Aldrich.
Human fibrosarcoma cells (HT1080) and murine mammary adenocarcinoma cells (derived from an M05 cell line) were purchased from the National Cell Bank of Pasteur Institute (Tehran, Iran). The HT1080 cells were cultured in Dulbecco’s Modified Eagle’s Medium supplemented with 5% fetal bovine serum (without heat inactivation) and 1% penicillin-streptomycin, and incubated at 37°C and 5% CO2.
Instrumentation
The Gd3+ ions were quantified using inductively coupled plasma atomic emission spectrometry (ICP-AES, Optima 2300, Perkin-Elmer, Boston, MA, USA). The zeta potential of MSN-Gd3+-DG dispersed in an aqueous solution (pH 7–8) was measured using a Zetasizer analyzer (Malvern Instruments Ltd, Worcestershire, UK). Transmission and scanning electron microscopic images were obtained on a Philips CM-120 (Eindhoven, The Netherlands) at 200 kV and a Hitachi 4160 (Tokyo, Japan), respectively. The textural properties of the MSN-Gd3+-DG were obtained from nitrogen adsorption isotherms at 77 K using a Belsorp Mini device (Toyonaka City, Japan). Brunauer-Emmett-Teller surface areas were determined from the recorded adsorption data in the range of intermediate partial pressures 0.05 ≤ P/P0 ≤ 0.3, and pore volume and pore size distribution were determined using the Barrett-Joyner-Halenda method. Thermogravimetric analysis was performed using a TGA-50 device (Shimadzu, Kyoto, Japan) equipped with a platinum pan at a heating rate of 3°C per minute under air.
Fourier transform infrared spectra were recorded using an Equinox 55 spectrophotometer (Bruker, Ettlingen, Germany). Magnetic resonance imaging (MRI) was performed on a 1.5 Tesla scanner (Siemens, Erlangen, Germany).
Flow cytometry was performed with a FACScan cytometer equipped with an Epics Altra HyPerSort 2 system (Beckman Coulter Inc, Fullerton, CA, USA) by counting 30,000 events, and the data were analyzed using Beckman Coulter software. Absorbance was measured at 450 nm using an ELX800 absorbance microplate reader (Bio-Tek Instruments Inc, Winooski, VT, USA). Blood glucose levels were measured using a glucometer (Bayer, Leverkusen, Germany).
Animals
BALB/C mice were sourced from the Laboratory Animal Center Institute of Cancer Research at Tehran University of Medical Sciences. The animal experiments were approved by the Imam Khomeini Cancer Research Center, Tehran, Iran. A total of 3 × 105 murine mammary adenocarcinoma cells (derived from an M05 cell line) were subcutaneously injected into the right flank of each mouse. Tumor growth was visible 4–5 weeks following injection.
Synthesis of MSN-Gd3+-DG
Gd3+-based MSNs
The MSNs used in this study were synthesized using the Stober method.Citation4 Briefly, 1.0 g of cetyltrimethylammonium bromide was dissolved in 500 mL of distilled water containing 3.5 mL of 2 M NaOH, and the solution was heated to 80°C. Next, 5.0 mL of tetraethylorthosilicate was added to the mixture. After stirring for a further three hours at 80°C, the MSNs were isolated by filtration and washed with distilled water and ethanol.
Amino-functionalized MSNs
First, 1.0 g of MSNs was dispersed in 100 mL of anhydrous ethanol with ultrasonic treatment for 10 minutes. Next, 1.0 mL of APTES was added dropwise to the solution over approximately 10 minutes and the reaction was stirred for 12 hours. The resulting solution was centrifuged at 6000 rpm for 20 minutes and redispersed in water to remove unreacted APTES.Citation28 The Kaiser test was performed to confirm that silanization was successful.Citation27
Extraction of surfactant
The MSNs (1.5 g) were refluxed in 162 mL of methanol solution with 1.57 M hydrochloric acid for 12 hours to remove the surfactant (cetyltrimethylammonium bromide). The extracted MSN-NH2 was washed three times with ethanol and stored as a dispersion in ethanol for future use.Citation29 Thermogravimetric analysis was used to characterize the amount of Gd3+-diethylenetriamine tetraacetic acid (DTTA) embedded in the MSNs.
Synthesis of Si-DTTA
First, 0.4 g of 3-(trimethoxysilylpropyl) diethylene triamine and 1.0 g of bromoacetic acid were dissolved in 2.0 mL of distilled water and 4.0 mL of 2 M NaOH. The reaction solution was heated to 50°C. NaOH (6.0 mL, 2 M) was added drop-wise over 20 minutes. After stirring for a further three hours at 50°C, the solvent was removed under reduced pressure to obtain a viscous yellowish oil. After washing with ethanol, a hygroscopic powder was obtained in high yield (>90%).Citation14
Synthesis of Gd3+-Si-DTTA
Si-DTTA (100.0 mg) was dissolved in 4.0 mL of distilled water by stirring at room temperature. Next, 300.0 μL of 50 M GdCl3 was added dropwise to the solution. The pH of the solution was adjusted to about 9 by addition of 1 M NaOH. After stirring for three hours at room temperature, the resulting solution was then concentrated to 1.0 mL ().Citation8,Citation14
Loading Gd3+-Si-DTTA into MSN-NH2
MSN-NH2 (200.0 mg) was refluxed with 0.5 mL of the Gd3+-Si-DTTA complex (0.1 M) in toluene for 48 hours. The resulting MSN-NH2-Gd3+-Si-DTTA was centrifuged at 8000 rpm for 15 minutes, then washed with water and ethanol. The resulting nanoparticles were dialyzed against phosphate-buffered saline to ensure removal of any free complexes.Citation8,Citation14
Treatment of silanized surface with heterobifunctional ANB-NOS
ANB-NOS was dissolved in dimethyl formamide. Next, 500 mg of MSN-NH2 and 50 mg of ANB-NOS were reacted in 20 mM HEPES at pH 8 and room temperature for two hours in the dark. The Kaiser test was performed to show successful reaction with ANB-NOS by confirming the absence of free amines.Citation27,Citation29
Conjugation of glucosamine
MSNs functionalized with 100.0 mg of ANB-NOS (MSN-ANB-NOS) was incubated at 37°C for 30 minutes with a saturated solution of glucosamine. Glucosamine was attached to the MSN surfaces by exposure to 302 nm light for five minutes at room temperature. Unbound glucosamine molecules were removed by washing three times with phosphate-buffered saline containing 0.05% Tween 20 (pH 7.5), with dialysis against phosphate-buffered saline to ensure removal of any free glucosamine molecules ().Citation30,Citation31
Preparation for MRI in vitro
AnHT1080 cell line (1 × 105) was incubated with two different doses of MSN-Gd3+-DG (0.2 μM and 0.4 μM) for one hour. The labeled cells were washed with phosphate-buffered saline, trypsinized and then centrifuged at 1000 rpm for five minutes at 37°C. The cells were then resuspended in an equal volume of Dulbecco’s Modified Eagle’s Medium and 2% agarose gel at 37°C, and were immediately translocated into phantom tubes for the MRI procedure. Unlabelled cells were used as the control group.
Kaiser test for free amines
The Kaiser test was used to detect the presence of free amino groups on the surface of the MSNs. The samples were washed to remove unreacted APTES and ANB-NOS. The MSNs were then centrifuged at 6000 rpm for 10 minutes to remove the supernatant. Solutions containing 50 mL of ninhydrin (0.5 g in 10 mL of ethanol), phenol (40 g in 10 mL of ethanol), and pyridine (1 mL of 0.001 M potassium cyanide in 49 mL of pyridine) were subsequently added to the MSNs. The MSN mixtures were then immersed in boiling water for two minutes. A blue color indicates the presence of free amine, whereas a yellow color indicates its absence.Citation27
Cell viability (MTT) assay
The HT1080 cell line was cultured in 96-well plates (5 × 105 cells/well), with each well containing 200 μL of Dulbecco’s Modified Eagle’s Medium and 10% fetal bovine serum. After culture for 24 hours, the medium was removed and replaced with Dulbecco’s Modified Eagle’s Medium containing 1% fetal bovine serum in the absence or presence of two concentrations of MSN-Gd3+-DG (100 nM, 200 nM) and incubated for 24 hours. Each concentration was tested in triplicate. MTT solution (20 μL of 5 mg/mL) was added to each well and incubated at 37°C for four hours in 5% CO2; cellular reduction of MTT by mitochondrial dehydrogenase in viable cells forms a blue formazan product, which can be measured quantitatively by a microplate reader at a wavelength of 540 nm.Citation32
TNF-α assay
The amount of tumor necrosis factor-alpha (TNF-α) secreted was analyzed using an enzyme-linked immunosorbent assay kit (U-CyTechR Biosciences, Utrecht, The Netherlands). MSN-Gd3+-DG at 20, 40, 60, and 100 μg/mL was incubated with the HT1080 cell line for 24 hours. The cells were then pelleted and the supernatants collected for quantitative determination of TNF-α secretion according to a specific protocol at 570 nm, using a Bio-Tek absorbance microplate reader at 450 nm. The negative control contained only Dulbecco’s Modified Eagle’s Medium.Citation33,Citation34
Apoptosis assays
Apoptosis is considered a different mechanism of certain cell death. Apoptosis was determined using an Annexin V-propidium iodide staining kit according to the manufacturer’s recommendations.Citation35
Intracellular uptake study
To detect intracellular uptake of MSN-Gd3+-DG, the cells were replated into six-well plates at a concentration of 2 × 105 cells per well and incubated at 37°C and 5% CO2 for 24 hours. MSN-Gd3+-DG (1 mL of 5.0 μg/mL) was added to the wells, each of which contained 1 mL of medium. The cells were incubated at 37°C with 5% CO2 for two hours. The cells were washed twice with 500 μL of phosphate-buffered saline, and then centrifuged at 1000 rpm for four minutes and reconstituted in 100 μL of phosphate-buffered saline. Cellular uptake of Gd3+ ions was determined quantitatively by ICP-AES. From the concentration of Gd3+ ions and the total number of cells, the average amount of Gd3+ ions taken up by each cell was calculated. These measurements were performed in triplicate and the mean and standard deviation of the results were calculated.
Hexokinase assay
The hexokinase assay experiments were carried out according to a previous report.Citation36 Briefly, 1.0 mg of MSN-Gd3+-DG and 1.0 mg of deoxyglucosamine were dissolved separately in 1.0 mL of water. Exactly 200 μL of each solution was diluted in 2.5 mL of water.Citation33 A 10 μL aliquot of each solution was mixed with 900 μL of Infinity glucose reagent and incubated at 37°C for three minutes. Changes in absorbance of Nicotinamide adenine dinucleotide phosphate (NADP) were measured using a Bio-Tek absorbance microplate reader at 450 nm.
Changes in blood glucose levels
Changes in blood glucose levels were investigated as described elsewhere.Citation33 Different doses of MSN-Gd3+-DG (1, 5, 10 mg/kg) were injected intravenously into BALB/C mice (six per group). Changes in blood glucose levels were determined using a glucometer 30, 60, 90, 120, and 180 minutes after injection and were compared with levels in the control groups. The control groups were treated with insulin (a glucose-lowering agent, negative control) or glucosamine (a glucose-increasing agent, positive control), or received no treatment.
Statistical analysis
Multigroup comparisons of the means were carried out using one-way analysis of variance. Statistical significance for cell toxicity was set at P < 0.05, and for the TNF-α and apoptosis assays was set at P < 0.05 and P < 0.01, respectively. The results are expressed as the mean ± standard deviation (n = 3–5).
Measurements on MRI
Relaxation times for MSN-Gd3+-DG were measured at different concentrations of 0.6, 0.3, 0.15, 0.075, and 0.0375 mM. Different spin echo and gradient echo protocols were used with a 1.5 Tesla machine with a head coil. Multiple spin echo protocols were used for measurement of T2. Standard spin echo particulars were as follows: echoes 4; TE 16, 32, 48, 64 msec; TR 3000 msec; matrix 512*384; slice thickness 4 mm; field of view 25 cm; and NEX 3. A Flash protocol was used to compute the T1 maps. Standard spin echo was as follows: echoes 1; TE 15 msec; TR 100, 200, 400, 600, 1000, and 2000 msec; matrix 512*384; slice thickness 4 mm; field of view 25 cm; NEX 3; and pixel bandwidth 130. For quantitative analysis of the data, the MRI images obtained were transferred to DICOM (Digital Imaging and Communication in Medicine) Works software version 1.3.5 (Digital Imaging and Communications in Medicine, Rosslyn, VA, USA).Citation33
In vivo imaging of tumors
An MRI study was carried out in an animal model to evaluate the in vivo capability of the MSN-Gd3+-DG to discriminate between cancerous and normal tissue. The tumor images obtained five minutes after a 5 μmol/kg injection showed that the amount of MSN-Gd3+-DG internalized was large enough to have a significant effect on the intensity of the MRI signal.
Results
Qualitative characterization
The Kaiser test was used to detect the presence of priming amino groups on the surface of the MSNs.Citation23 APTES was used in this study to prepare priming amine groups for the particles by silanization. Once the reaction had taken place, the extracted MSN-NH2 was washed to remove excess APTES. On addition of Kaiser solution, the particles turned blue immediately, indicating that APTES had been anchored. However, after addition of Kaiser solution to MSN-ANB-NOS, appearance of a yellow color indicated the absence of free amine.
Fourier transform infrared analysis
Fourier transform infrared spectroscopy was used to confirm the existence of functionalized MSNs in the region of 400–4000 cm−1. The infrared spectrum of ANB-NOS showed absorption peaks at 1739 cm−1 and 1784 cm−1 attributable to C=O groups in the succinimide moiety, at 2120 cm−1 attributable to the N3 group, and at 1527 cm−1 and 1348 cm−1 attributable to the NO2 group.
When ANB-NOS was grafted onto the MSNs, the spectrum showed a new broad absorption band at 3200–3600 cm−1 corresponding to the silanol-OH bond and strong absorption of the siloxane (Si–O–Si) group at 1094 cm−1. An N3 absorption band was also observed at 2123 cm−1, with an amide carbonyl (–NH–C=O) stretch mode at about 1637 cm−1. These results indicate that ANB-NOS was incorporated into MSNs. When glucosamine was attached onto the MSN surface by exposure to light at 302 nm, the N3 absorption disappeared and the OH groups on the MSN nanoparticles and glucosamine showed a broad band at 3000–3600 cm−1 ().
Figure 3 FTIR spectra of: (—) ANB-NOS-MSN; (----) glucosamine grafted on ANB-NOS-MSN: the N3 absorption disappears when glucosamine was attached on the MSN surface.
Abbreviations: FTIR, fourier transform infrared spectroscopy; ANB-NOS-MSN, N-5-azido-2-nitrobenzoyloxy succinimide–mesoporous silica nanosphere; MSN, mesoporous silica nanospheres.
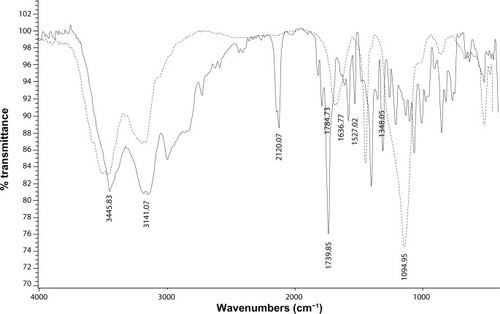
Adsorption and desorption of nitrogen
Measurements for adsorption and desorption of nitrogen gas indicated that the surfactant-extracted MSNs were highly porous, with a surface area of 970 m2/g, an average pore diameter of 2.5 nm, and a total pore volume of 0.57 cm3/g. In contrast, the MSN-Gd3+-DG had a reduced surface area of 26.54 m2/g, a mean pore diameter of 1.5 nm, and a total pore volume of 0.086 cm3/g ().
Table 1 Summary of Barrett-Joiner-Halenda (BJH) surface areas and pore sizes
Thermogravimetric analysis
Thermogravimetric analysis of the MSNs showed an initial weight loss of 13.7% from room temperature to 130°C for the adsorbed solvent species, and further weight loss of 46.99% in the temperature range of 160°C–290°C, indicating that the surfactant (cetyltrimethylammonium bromide) was removed whereas the extracted MSNs showed a weight loss of 1.0% across a temperature range of 160°C–290°C. This result confirms that the surfactant was removed during extraction ().
Figure 4 TGA curves of extracted MSN (—) and MSN (—). An initial weight loss of 13.7% from room temperature to 130°C for the adsorbed solvent species; Weight loss of 46.99% in the 160°C–290°C temperature range shows that the surfactant (CTABr) was removed.
Abbreviations: TGA, thermal gravimetric analysis; MSN, mesoporous silica nanospheres; extracted MSN, mesoporous silica nanospheres without surfactant; CTABr, cetyltrimethylammonium bromide.
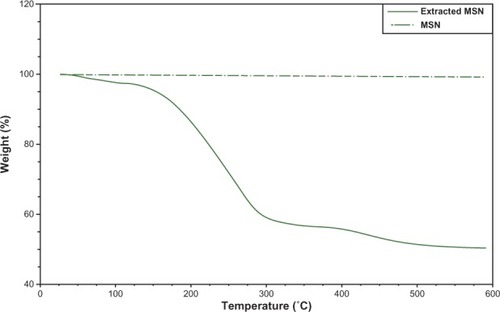
Electron microscopy
Scanning and transmission electron microscopy was used to evaluate MSN morphology and size. The images show that there was no change in MSN morphology after conjugation of glucose onto the surfaces of the MSNs, extraction of the surfactant, or upon grafting of the Gd3+-DTTA-chelated molecule ( and ).
Figure 5 SEM images of MSN-Gd3+-DG.
Abbreviations: SEM, scanning electron microscope; MSN-Gd3+-DG, mesoporous silica nanospheres loaded with Gd3+ and conjugated with glucosamine.
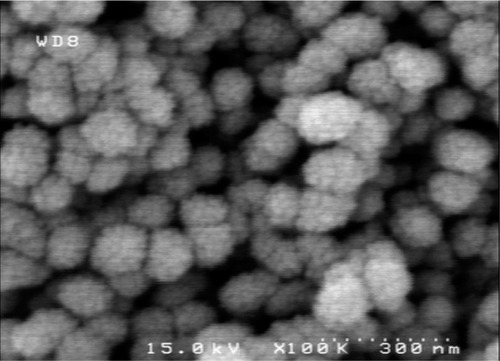
Figure 6 Transmission electron micrograph of an ultramicrotomed MSN-Gd3+-DG. The layer of DG can be visualized by the rim of amphorous structure surrounding the MSN core with mesopores packed in a hexgonal symmetry.
Abbreviations: DG, D glucosamine; MSN, mesoporous silica nanospheres; MSN-Gd3+-DG, mesoporous silica nanospheres loaded with Gd3+ and conjugated with glucosamine.
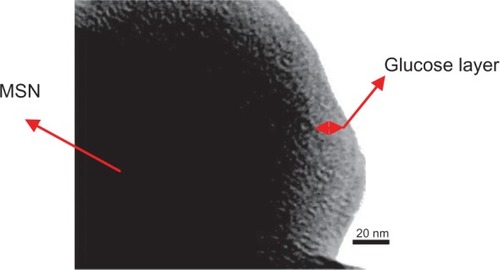
Zeta potential measurements
The zeta potentials for MSN, MSN-NH2, and MSN-Gd3+-DG are shown in , and indicate that the MSN-NH2 aggregates in water because of the point of zero charge when pH is 7–8. In our experiments, although the MSN-NH2 was washed three times after reaction, the ammonium hydroxide could not be completely removed. The solution appeared alkaline and had a low negative zeta potential of about −3 mV, indicating that the MSN-NH2 aggregated rapidly, which agrees with the results reported by other researchers.Citation35,Citation38,Citation39
Table 2 Characteristics of silica nanospheres (zeta potential)
Cell viability assay
MTT assays were performed using the HT1080 cell line to determine whether MSN-Gd3+-DG has a cytotoxic effect. shows a comparison of the HT1080 cell line when incubated for 24 hours at different concentrations of MSN-Gd3+-DG (100 μg/mL and 200 μg/mL). The MSN-Gd3+-DG concentrations were similar to that of the control (0 μg/mL), indicating that cell viability was not affected at the concentration range tested.
Figure 7 MTT results of 24 h of MSN-Gd3+-DG exposure to HT1080; No significant toxic effect was observed (P < 0.05).
Abbreviations: MTT, MTT cell proliferation assay; HT1080, human fibrosarcoma cells; MSN, mesoporous silica nanospheres; MSN-Gd3+-DG, mesoporous silica nanospheres loaded with Gd3+ and conjugated with glucosamine.
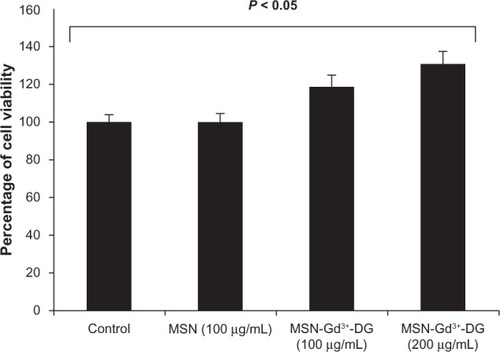
TNF-α assay
Characterization of physiological effects is required for the continued development of MSN-Gd3+-DG The TNF-α level usually rises when cells are injured or inflamed. We found that treatment with MSN-Gd3+-DG caused a significant increase in release of TNF-α up to a dose of 40 µg/mL (P < 0.01, ). However, the increased dose of MSN-Gd3+-DG did not have any toxic effects, such as causing cell death.
Figure 8 MSN-Gd3+-DG treated HT1080 cells showed an insignificant release of TNF-α (P < 0.05, P < 0.01).
Abbreviations: TNF-α, tumor necrosis factor alpha; MSN-Gd3+-DG, mesoporous silica nanospheres loaded with Gd3+ and conjugated with glucosamine; HT1080, human fibrosarcoma cells; MSN, mesoporous silica nanospheres.
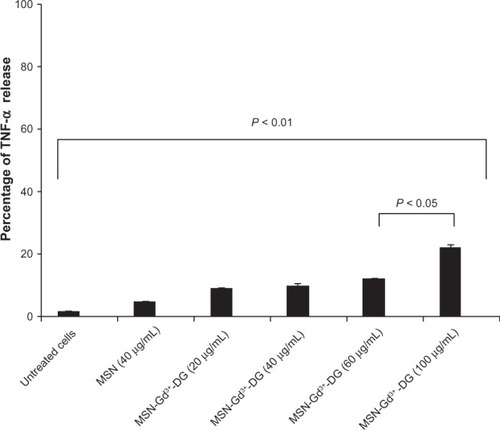
Apoptosis assay
Apoptosis is a mode of cell death that occurs under normal physiological conditions and the cell is an active participant in its own demise (ie, “cellular suicide”). Our findings demonstrate a statistically insignificant time-dependent increase in apoptotic cell responses to MSN-Gd3+-DG at doses up to 40 μg/mL (P < 0.05); however, increasing the dose to over 60 μg/mL resulted in a significant increase in apoptotic activity. Further, it should be remembered that the results of the MTT assay indicated no toxic effects at any doses of MSN-Gd3+-DG. Based on immunological assays, it can be hypothesized that if the exposure time is extended to over 24 hours, a significant increase in the rate of cell death is probable. The data analyzed are shown in detail in .
Intracellular uptake study
The affinity of MSN-Gd3+-DG for HT1080 cells was determined and compared with that obtained from the nonspecific precursor MSN-Gd3+, as shown in . The ICP-AES results show that cellular uptake of MSN-Gd3+-DG was about 3.2 times more than that for the nanospheres alone. The data confirm the important role of glucose in MSN-Gd3+-DG ().
Figure 10 Cell uptake assay of MSN-Gd3+-DG: Results indicated the glucose effect on intracellular uptake (P < 0.05).
Abbreviations: MSN-Gd3+-DG, mesoporous silica nanospheres loaded with Gd3+ and conjugated with glucosamine; HT1080, human fibrosarcoma cells; ICP-AES, inductively coupled plasma atomic emission spectrometry; MSN, mesoporous silica nanospheres.
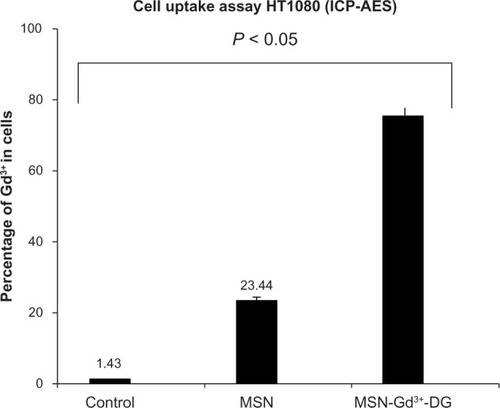
Hexokinase assay
Absorption at 340 nm for glucosamine and MSN-Gd3+-DG () was a sign of glucose phosphorylation due to the presence of the reduced form of nicotinamide adenine dinucleotide.Citation36,Citation37
Table 3 Positive hexokinase enzyme response to glucosamine, and MSN-Gd3+-DG
Blood glucose levels
A statistically insignificant (P < 0.05) increase in blood glucose levels was observed after intravenous injection of MSN-Gd3+-DG at the various doses.Citation36 Coadministration of MSN-Gd3+-DG and insulin caused a marked decrease in blood glucose levels. This confirms the effectiveness of insulin on cell/tissue uptake of MSN-Gd3+-DG ().
In vitro MRI experiments
To evaluate whether the amount of MSN-Gd3+-DG internalized was enough for visualization of cancerous cells with respect to healthy ones, an “in vitro” MRI experiment was performed. For this purpose, HT1080 cells were incubated for one hour in the presence of an equal amount (100 μM) of MSN-Gd3+-DG shows that cells labeled with MSN-Gd3+-DG showed a more intense signal on the T1-weighted spin echo image than the unlabeled cells. This demonstrates efficient uptake of the MSN-Gd3+-DG for cell imaging.
Relaxivity
The MRI relaxation times for MSN-Gd3+-DG were measured using a 1.5 Tesla MRI scanner (). The nanoparticles showed large longitudinal (r1) and transverse (r2) relaxivities. The r1 and r2 values were 17.70 mM−1s−1 and 26.095 mM−1s−1, respectively and the r2:r1 ratio was 1.5 ().
In vivo tumor imaging
The efficacy of MSN-Gd3+-DG as an in vivo MRI contrast agent was evaluated. The tumor images obtained a few minutes after injection of MSN-Gd3+-DG 5.0 μmol/kg via the tail vein indicate the usefulness of MSN-Gd3+-DG as a potential MRI contrast agent ().
Figure 15 MR images (A) pre-injection and (B) 5 minutes post-injection (5 μmol/kg). A total of 3 × 105 Murine Mammary Adenocarcinoma Cells were subcutaneously injected into the right flank of a mouse. Tumor growth was visible 4–5 weeks post injection. This result shows the ability of MSN-Gd3+-DG in enhancing the T1-weighted images.
Abbreviations: MRI, magneic resonance imaging; MSN-Gd3+-DG, mesoporous silica nanospheres loaded with Gd3+ and conjugated with glucosamine.
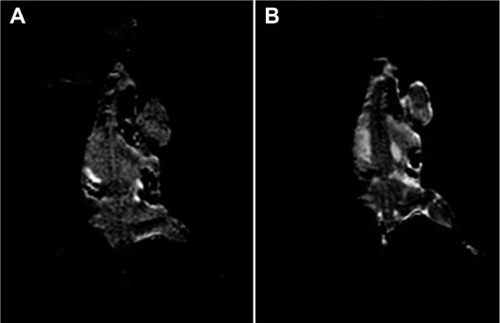
Discussion
Gd3+-based MSNs represent a nonselective contrast agent. Conjugation of glucosamine onto the surfaces of MSNs can be used to increase specific accumulation of these nanoparticles within the target cancerous tissue. Tumor cells consume more glucose than normal cells due to their higher rate of proliferation and overexpression of glucose transporters.Citation19
Amino-functionalized MSNs (MSN-NH2) were prepared by silanization with 3-aminopropyltriethoxysilane. ANB-NOS is a heterobifunctional crosslinker containing an amine-reactive N-hydroxysuccinimide ester and a photoactivatable nitrophenyl azide. N-hydroxysuccinimide esters react with primary amino groups of MSN-NH2 at pH 7–9 by forming a strong amide bond. When exposed to ultraviolet light, nitrophenyl azides form a nitrene group that can initiate additional reactions with double bonds and subsequent ring expansion to react with primary amines of glucosamine, which is a nucleophile. ANB-NOS is water-insoluble, so it was first dissolved in dimethyl formamide and then added to the aqueous reaction mixture. ANB-NOS is lipophilic and does not contain any charged groups. These properties are useful for intracellular conjugation.
MSN-NH2 has many amine groups, which should be partially charged at pH 8.5. Since MSN-NH2 does not have the ability to attach covalently to glucosamine directly the ANB-NOS is used for treating the surface of MSNs. ANB-NOS binds substantially more glucosamine molecules because the aryl azide group can link covalently with glucosamine. The crosslinking of ANB-NOS is initiated by ultraviolet irradiation because ultraviolet light activates the aryl azide groups to nitrene. Via nitrene, some amino groups in glucosamine can be crosslinked to form amides. Nitrene is a very reactive functional group, so its participation in the cross-linking process takes less than 10−4 of a second,Citation37 which minimizes nonspecific binding.
MSNs have rigid and strained siloxyl groups on their surface, with glucosamine molecules bonded to the outside of a curved surface, which tend to have considerable freedom of movement.Citation38 Notwithstanding, ANB-NOS is relatively “bulky” (7.7 Å) and can probably saturate the curved surface. In this case, glucosamine fits onto the curved area, with multiple electrostatic interactions and without spatial restriction. Therefore, MSN-NH2 can bind to glucosamine. The ANB-NOS-treated surface involves a covalent interaction, so glucosamine will remain attached. Accessibility of the surface to ANB-NOS is expected to be greater for a curved surface in comparison to a flatter surface.
MSN-Gd3+-DG is transported inside the cell by specific glucose transporters and phosphorylated by hexokinase, but is not metabolized further to any significant extent. MSN-Gd3+-DG-6-phosphate thereby accumulates in the cell, allowing distribution of MSN-Gd3+-DG in the tissues to be imaged by MRI.
Glucosamine and MSN-Gd3+-DG were tested for their ability to inhibit hexokinase competitively (). The behavior of MSN-Gd3+-DG was similar to that of glucosamine for inhibition of hexokinase. Amanlou et al also conjugated D-glucose to diethylenetriamine penta-acetic acid labeled it with Gd3+ (GDD), and examined the results in vitro and in vivo.Citation36 Their findings indicated that application of GDD to cancer cells also increased levels of TNF-α, but did not alter blood glucose levels. Interestingly, no toxicological findings were seen in normal human kidney cells.
Relaxivities were also measured and the r2:r1 ratio was 1.3, indicating that MSN-NH2-Gd3+ was a good T1-weighted contrast agent. We attributed this enhanced MRI relaxivity to the accessibility of cellular water molecules at paramagnetic centers.
Detection of primary tumors is a critical issue in the treatment of cancer. We have designed a tumor-oriented MRI contrast agent based on glycosylated Gd3+-based MSNs targeted to the glucose receptors overexpressed in tumor cells. Our results indicate that MSN-Gd3+-DG can enhance T1-weighted images. Taylor et al also prepared MSNs with grafted Gd3+ chelates (MSNs-Gd3+) as T1 contrast agents without any targeting group,Citation5 and demonstrated the efficacy of MSNs-Gd3+ as T1 contrast agents both in vitro and in vivo. Their results suggested that MSNs-Gd3+ have good potential in the diagnosis of early disease.Citation5,Citation11
Comparison of the relaxivities of MSN-NH2-Gd3+ and Magnevist® (Bayer HealthCare Pharmaceuticals, Inc, Mont-ville, NJ, USA) (which has an r1 value of 4.1 mM−1s−1 under the same conditions) shows that the former can be a better contrast agent. The same results were found comparing the relaxivity values of MSN-NH2-Gd3+ with those of recently reported solid silica nanoparticles coated with multilayers of Gd3+-DTTA.Citation8
The effectiveness of MSN-NH2-Gd3+ as an in vivo MRI contrast agent was evaluated using a 1.5 Tesla scanner. Upon injection of MSN-NH2-Gd3+ 5 μmol/kg into the tail vein, significant T1-weighted enhancement was clearly visible in the aortic and tumor tissue of a BALB/C mouse five minutes after injection (), indicating the usefulness of MSN-NH2-Gd3+ as an intravascular MRI contrast agent. This dose is much lower than what is typically required for currently used contrast agents (0.1–0.3 mmol/kg for Magnevist).Citation5,Citation8,Citation11 We attribute this to the large pore volume of MSNs for pay loading of magnetic centers (Gd3+-Si-DTTA).
Conclusion
As expected glucosamine did attach onto the MSN surface via the ANB-NOS crosslinker. The covalent bond formed between the silica surface and glucosamine is very resistant to disruption, and glucosamine has greater freedom of motion on the outside of a small sphere. Glucose-functionalized groups can be easily coupled to the surface of silica nanoparticles. Compared with existing methods, it is evident that covalent bonding of glucosamine to the MSN surface is an effective way of modifying MSNs. Immobilization of the Gd3+ ion inside the channels of MSNs enhances the transverse relaxation rate, leading to improved r1 relaxivity. Using this design strategy, we expect that a new cellular imaging contrast enhancer can be available in the near future.
Acknowledgments
This work was supported by Shahid Beheshti Medical University and Tehran University of Medical Science. Authors thank Behroz Rafiei for valuable help with the 1.5 Tesla MRI system, and Maryam Shahzad Shirazi for valuable assistance with characterization analysis.
Disclosure
This research forms the major part of a PhD thesis by BM in nanomedicine. Otherwise, the authors report no conflicts of interest in this work.
References
- LuJLiongMLiZZinkJITamanoiFBiocompatibility, biodistribution, and drug-delivery efficiency of mesoporous silica nanoparticles for cancer therapy in animalsSmall201061794180520623530
- GuzmanELiggieriLSantiniEFerrariMRaveraFEffect of hydrophilic and hydrophobic nanoparticles on the surface pressure response of DPPC monolayersJ Phys Chem C20111152171521722
- GuzmanELiggieriLSantiniEFerrariMRaveraFInfluence of silica nanoparticles on dilational rheology of DPPC-palmitic acid Langmuir monolayersSoft Matter2012839383948
- YuTGreishKMcGillLDRayAGhandehariHInfluence of geometry, porosity, and surface characteristics of silica nanoparticles on acute toxicity: their vasculature effect and tolerance thresholdACS Nano201262289230122364198
- TaylorKMLKimJSRieterWJMesoporous silica nanospheres as highly efficient MRI contrast agentsJ Am Chem Soc20081302154215518217764
- LinWHyeonTLanzaGMZhangMMeadeTJMagnetic nanoparticles for early detection of cancer by magnetic resonance imagingMRS Bull200934441448
- LinYSTsaiCPHuangHYKuoCTHungYHuangDMWell-ordered mesoporous silica nanoparticles as cell markersChem Mater20051745704573
- LuJLiongMShermanSXiaTKovochichMMesoporous silica nanoparticles for cancer therapy: energy-dependent cellular uptake and delivery of paclitaxel to cancer cellsNanobiotechnology20083899519936038
- Taylor-PashowKMLRoccaJDLinWMesoporous silica nanoparticles with co-condensed gadolinium chelates for multimodal imagingNanomaterials20122114
- SlowingIIVivero-EscotoJLWuCWLinVSYMesoporous silica nanoparticles as controlled release drug delivery and gene transfection carriersAdv Drug Deliv Rev2008601278128818514969
- RieterWKimJSTaylorKMLAnHLinWTarrantTJHybrid silica nanoparticles for multimodal imagingAngew Chem Int Ed20074636803682
- KimJSRieterWJTaylorKMLAnHLinWSelf-assembled hybrid nanoparticles for cancer-specific multimodal imagingJ Am Chem Soc20071298962896317602632
- LiLLYinQChengJLuYPolyvalent mesoporous silica nanoparticleaptamer bioconjugates target breast cancer cellsAdv Healthc Mater2012156757223184791
- De la FuenteJMPenadésSGlyconanoparticles: types, synthesis and applications in glycoscience, biomedicine and material scienceBiochim Biophys Acta2006176063665116529864
- ShenZLiYKohamaKOneillBBiJImproved drug targeting of cancer cells by utilizing actively targetable folic acid-conjugated albumin nanospheresPharm Res2011635158
- GanapathyVThangarajuMPrasadPDNutrient transporters in cancer: relevance to Warburg hypothesis and beyondPharm Ther20091212940
- SchibliRDumasCPetrigJSynthesis and in vitro characterization of organometallic rhenium and technetium glucose complexes against glut 1 and hexokinaseBioconjug Chem20051610511215656581
- KhanianiYBadieiaAZiaraniGMApplication of clickable nanoporous silica surface for immobilization of ionic liquidsJ Mater Res201227931938
- HaenschCHoeppenerSSchubertUSChemical surface reactions by click chemistry: coumarin dye modification of 11-bromoundecyl-trichlorosilane monolayersNanotechnology20081903570321817588
- SchlossbauerASchaffertDKechtJWagnerEBeinTClick chemistry for high-density biofunctionalization of mesoporous silicaJ Am Chem Soc2008130125581255918759397
- GallantNDLaveryKAAmisEJBeckerMLUniversal gradient substrates for “click” biofunctionalizationAdv Mater200719965969
- HuangLDolaiSRajaKKrukM“Click” grafting of high loading of polymers and monosaccharides on surface of ordered mesoporous silicaLangmuir2010262688269320141209
- GuanBCiampiSLe SauxGGausKReecePJGoodingJJDifferent functionalization of the internal and external surfaces in mesoporous materials for biosensing applications using “click” chemistryLangmuir20112732833421141983
- WongCBurgessJPModifying the surface chemistry of silica nanoshells for immunoassaysJ Young Invest200361114
- BoiteauRMeadeTGadolinium-labeled nanoparticles for magnetic resonance imagingNanoscape200853339
- RaduDRLaiCYWienchJWPruskiMLinVSGatekeeping layer effect: a poly(lactic acid)-coated mesoporous silica nanospheres-based fluorescence probe for detection of amino-containing neurotransmittersJ Am Chem Soc20041261640164114871088
- McDonaldARDijkstraHPSuijkerbuijkBMJMvan KlinkGPMvan KotenG“Click” immobilization of organometallic pincer catalysts for C-C coupling reactionsOrganometallics20092846894699
- AdamsCAKarSRHopperJEFriedMGSelf-association of the amino-terminal domain of the yeast TATA-binding proteinJ Biol Chem20042791376138214534318
- ZhangCLiYShiXKimSKInhibition of the expression on MMP-2, 9 and morphological changes via human fibrosarcoma cell line by 6,6′-bieckol from marine alga Ecklonia cavaBMB Rep2009626819250605
- AmanlouMSiadatSDEbrahimiSEGd3+-DTTA-DG: novel nanosized dual anticancer and molecular imaging agentInt J Nanomedicine2011674776321589643
- MosmannTRapid colorimetric assay for cellular growth and survival: application for proliferation and cytotoxicity assayJ Immunol Methods19836555636606682
- MirzaeiMMohagheghiMShahbazi-GahroueiDKhatamiANovel nanosized Gd3+-ALGD-G2-C595: in vivo dual selective MUC-1 positive tumor molecular MR imaging and therapeutic agentJ Nanomed Nanotechnol20123147152
- PuechPABousselLBelfkihSLemaitreLDouekPBeuscartRDicomWorks: software for reviewing DICOM studies and promoting low-cost teleradiologyJ Digit Imaging20072012213017333414
- LewisRVRobertsMFDennisEAAllisonWSPhotoactivated heterobifunctional cross-linking reagents which demonstrate the aggregation state of phospholipaseBiochemistry19771656505654921957
- IlerRKThe Chemistry of SilicaNew York, NYJohn Wiley & Sons1979
- AmanlouMSiadatSDEbrahimiSEGd3+-DTPA-DG: novel nanosized dual anticancer and molecular imaging agentInt J Nanomed20116747763
- SchibliRDumasCPetrigJSynthesis and in vitro characterization of organometallic rhenium and technetium glucose complexes against GLUT 1 and hexokinaseBioconjug Chem20051610511215656581
- AnYChenMXueQLiuWPreparation and self-assembly of carboxylic acid functionalized silicaJ Colloid Interface Sci20073150751317383673
- SlowingITrewynBGLinVSEffect of surface functionalization of MCM-41-type mesoporous silica nanoparticles on the endocytosis by human cancer cellsJ Am Chem Soc2006128147921479317105274