Abstract
Although showing much promise for numerous tissue engineering applications, polyurethane and poly-lactic-co-glycolic acid (PLGA) have suffered from a lack of cytocompatibility, sometimes leading to poor tissue integration. Nanotechnology (or the use of materials with surface features or constituent dimensions less than 100 nm in at least one direction) has started to transform currently implanted materials (such as polyurethane and PLGA) to promote tissue regeneration. This is because nanostructured surface features can be used to change medical device surface energy to alter initial protein adsorption events important for promoting tissue-forming cell functions. Thus, due to their altered surface energetics, the objective of the present in vivo study was to create nanoscale surface features on a new polyurethane and PLGA composite scaffold (by soaking the polyurethane side and PLGA side in HNO3 and NaOH, respectively) and determine bladder tissue regeneration using a minipig model. The novel nanostructured scaffolds were further functionalized with IKVAV and YIGSR peptides to improve cellular responses. Results provided the first evidence of increased in vivo bladder tissue regeneration when using a composite of nanostructured polyurethane and PLGA compared with control ileal segments. Due to additional surgery, extended potentially problematic healing times, metabolic complications, donor site morbidity, and sometimes limited availability, ileal segment repair of a bladder defect is not optimal and, thus, a synthetic analog is highly desirable. In summary, this study indicates significant promise for the use of nanostructured polyurethane and PLGA composites to increase bladder tissue repair for a wide range of regenerative medicine applications, such as regenerating bladder tissue after removal of cancerous tissue, disease, or other trauma.
Introduction
Due to optimal mechanical properties but a frequent lack of suitable cytocompatibility, researchers have suggested several techniques to improve polyurethane (PU) for numerous tissue engineering applications.Citation1–Citation5 Similarly, due to its optimal degradation properties but lack of suitable cytocompatibility, various techniques have been proposed to improve poly-lactic-co-glycolic acid (PLGA) for tissue engineering applications.Citation1–Citation5 Whereas PU is hydrolytically stable, PLGA degrades in aqueous environments due to ester bond breakage. Importantly though, PU has been shown to degrade in vivo due to the presence of ether linkages in PU soft segments susceptible to chemical degradation. Ether and ester bond breakage allows both PU and PLGA, respectively, to be easily chemically and structurally modified to improve cellular responses.Citation3–Citation5
Thapa et al were the first to treat PU and PLGA separately with various concentrations of HNO3 and NaOH, respectively, for selected periods of time to promote nanoscale surface features to enhance in vitro bladder cell responses.Citation1,Citation2 Miller et al followed and demonstrated greater in vitro vascular cell responses while Vance et al provided evidence of decreased in vitro fibroblast responses leading to decreased prolonged fibrous tissue growth on such nanostructured films compared with conventional PU and PLGA films.Citation6,Citation7 Kay et al also provided evidence of greater function of chondrocytes (cartilage-forming cells) on nanostructured polymers compared with conventional polymers and polymer composites.Citation8 All of the aforementioned studies used cell adhesion and proliferation as indicators of enhanced cell function.
In an attempt to understand the properties of HNO3-treated PU and NaOH-treated PLGA that enhanced cellular responses, Thapa et al and Miller et al created separate PU and PLGA molds of conventional and HNO3-etched PU and NaOH-etched PLGA, respectively (thereby eliminating chemical changes and studying only nanometer roughness changes), and found similar increases in in vitro bladder and vascular cell functions, respectively, on the nanostructured molds compared with conventional molds.Citation1,Citation2,Citation6,Citation7
Thus, to date, the most promising consequence of HNO3 treatment of PU and NaOH treatment of PLGA has been the creation of nanometer surface roughness promoting selected cell responses important for the regeneration of numerous tissues, but no data have been provided to date concerning the ability of these nanostructured polymers to regenerate bladder tissue in vivo. Moreover, several studies have indicatedCitation9–Citation15 that PU does not possess desirable biodegradability or mechanical properties compared with natural bladder tissues.Citation1–Citation5 For this and all of the above reasons, the objective of the present study was to create novel graded PLGA-PU scaffolds with nanoscale surface roughness and characterize their ability to regenerate bladder tissue using a well established minipig model.
Materials and methods
Materials
Grafts were produced using a salt leaching method to achieve 80%-90% target porosity and the continuously graded composition as shown in . Specifically, the scaffolds were created through a salt leaching method to introduce pores into the polymers using 150–250 μm diameter salt (NaCl) particles (Sigma, St Louis, MO, USA) at a 10:90 polymer to salt ratio by weight. To create layers of increasing amounts of PLGA on PU-PLGA composites, layers of the next increasing weight percentage of PLGA to PU were placed by pipette on top of the lesser percentage of PLGA to PU ratio. This was done for every 20 wt% of PLGA to PU until the composite formed was complete PU (0% PLGA) to complete PLGA (0% PU). The PLGA used was 75:25 wt% (PLA to PGA, 12–16.5 × 103 molecular weight; Polysciences Inc, Warrington, PA, USA) and the PU used was Tecoflex® (medical grade SG80A, 90,000–140,000 kDa Noveon Inc, Minneapolis, MN, USA). Each polymer was dissolved in 1,1,1,3,3,3-hexafluoro-2-propanol at a concentration of 100 mg/mL. After forming each layer of the scaffold vacuum drying was used followed by rinsing with water to remove the salt (and thereby create pores). To induce nanoscale topologies on both the PLGA and PU layers, the scaffolds were exposed to 1.0 N NaOH on the PLGA side and then flipped and exposed to 10 N HNO3 on the PU side for 10 minutes each followed by rinsing in neutral pH after each exposure. The scaffolds were continuously rinsed in phosphate-buffered solution (pH 7.4) until the eluent pH was 7.4. The polymers were then functionalized with IKVAV and YIGSR to promote cellular functions important for the regeneration of bladder tissue. For this, standard aminophase silane chemistry was followed with N,N-(3-dimethylaminopropyl)-N′-ethyl-carbodiimide hydrochloride/N-hydroxysuccinimide (EDC/NHS, Sigma, St Louis, MO, USA) used to immobilize IKVAV and YIGSR (Sigma).Citation17 Lastly, radiofrequency glow discharge argon plasma was utilized for polymer scaffold sterilization for four minutes at 100 W.Citation9
Material characterization
The topography and porosity of the nanostructured polymeric scaffolds were evaluated using scanning electron microscopy (JSM-840, JEOL, New York, NY, USA) at an accelerating voltage of 5 kV and with Digital Scan Generator Plus software. Atomic force microscopy (NanoScope IIIa, Digital Instruments Inc, Boston, MA, USA) was also used to characterize surface roughness. Total porosity measurements were quantified using a SA 3100™ series surface area and pore size analyzer (Beckman Coulter, Fullerton, CA, USA) and the Brunauer-Emmett-Teller calculation. The chemistry of the polymers was characterized using x-ray photoelectron spectroscopy according to standard techniques.
The degradation rate of the nanostructured polymeric scaffolds was determined by placing them in cell buffer solutions (Dulbecco’s Modified Eagle Medium, Gibco, St Louis, MO, USA) under standard incubator conditions for up to 23 days and calculating the weight retained after certain time periods. Lastly, the surface energy of each side of the samples was determined using sessile (static) water contact angles by placing 100 μL of water onto each sample and using a camera to determine the contact angles.
Mechanical properties
Burst pressure (ASTM D3787)
To determine the ability of the bladder constructs to resist potential pressure increases representative of the bladder, a ball burst test was used. The pathological bladder wall tension range was found to be 9.8–11.7 N/cm2 and the normal physiological bladder wall tension was found to be 2.8 N/cm2.Citation1 The ball burst strength is defined as the force that is required to burst the material when applied perpendicular to the plane of the material. A mechanical testing system 810 (MTS Co, Eden Prairie, MN, USA) was used with a burst strength fixture for knitted goods (ASTM 3787) to measure the biaxial strength of the bladder constructs. The burst strength fixture and the mechanical testing machine were used according to standard test methods for determining the bursting strength of textiles during a constant-rate-of-traverse ball burst. A polished steel ball was advanced at a constant rate (25.4 mm per minute) through the mesh. Eleven samples of each material were cut into 10 cm × 5 cm each for testing. A mechanical burst pressure defined as between 9.8 N/cm2 and 11.7 N/cm2 will support the present design criteria for achieving favorable polymer graft characteristics for bladder applications.Citation1
Tensile test (ASTM D412)
In order to measure the tensile strength of the polymer constructs, uniaxial tensile tests were also performed. The ultimate tensile strain for the human bladder has been reported as 0.69 (mm/mm), the tensile strength as 0.27 (MPa), and the elastic modulus as 0.25 (MPa).Citation1 The samples were cut into dog-bone shapes 10 cm long and 2 cm wide in the middle. Constructs were clamped using a set of pneumatic piston clamps (Instron, Norwood, MA, USA) attached to the 810 mechanical testing system. The specimens were pulled at a rate of 25 mm per minute until failure. The force and displacement data were collected with computer software (Labview, Austin, TX, USA). Strength and stiffness were calculated from the stress-strain curves. Material thickness was assessed with a calibrated digital caliper (Mitutoyo, Tokyo, Japan). Eleven samples of each material were tested. A tensile strength defined as 0.52 (MPa) or two times that of normal human bladder tensile strength will support the design criteria of achieving favorable polymer graft characteristics.Citation1
Suture pullout strength
Lastly, polymer constructs were tested for suture retention by inserting four sutures into one end of each test sample, 2 mm from the end. Ethicon 2-0 Prolene™ sutures (Johnson and Johnson, Raynam, MA, USA) were used with an SH taper needle. The top pneumatic piston grip of the mechanical testing system was tightened to distribute the holding pressure equally along the surface of the sample. The sutures were inserted into the clamps of the lower grip, parallel to the direction of the force application. A mark made across the sample at the front inner edge of each grip was allowed, checking for sample slippage. Samples were tested at a constant rate of 25 mm per minute until the sample broke or until the suture material tore through the sample. Eleven samples of each material were tested. An adequate suture pullout strength defined as 25 N or better will support the present design criteria of achieving favorable graft characteristics.Citation1
In vivo study
This part of the study tested the aforementioned PU-PLGA scaffolds for bladder augmentation in a partial cystoplasty model. All protocols were approved by the Brown University Institutional Animal Care and Use Committee per National Institutes of Health regulations. A total of eight female minipigs, divided into two groups (those receiving control ileal segments and those receiving the novel nanostructured synthetic grafts) at two time periods (five and 11 weeks) were used. Preoperatively, the animals were acclimatized for a minimum of two days before surgery. Oral antibiotics were administered preoperatively and continued for 10 days postoperatively. Each animal was rendered unconscious using an intramuscular injection of ketamine 15–20 mg/kg and xylazine 2 mg/kg and were then intubated and anesthetized with 1%–3% isoflurane. For the ileal segment reconstruction, a 12–18 cm segment of ileum located proximal to the ileocecal valve was measured and tagged by nondegradable sutures. The mesentery of the iliac segment selected was incised and prepared in a sequential manner using Kelly clamps and 3–0 free ties. The bowel segment was divided and the end of the ileal segment to be exteriorized was marked. Proximal and distal ends of the ileum were then anastomosed. The distal closed end of the ileal segment was excised and opened to allow copious irrigation of its lumen with saline solution. The ileal loop was oriented to allow peristalsis to proceed in the antegrade direction towards the cutaneous stoma. Next, the left and right ureters were isolated and anastomosed to the ileal segment. A circular skin excision in the previously marked stoma location was performed and an adequate crossed window was provided through the rectus fascia. The ileal end with a 2 cm nipple was secured to the rectus fascia with 3–0 Vicryl™ sutures (Ethicon). The stoma was then completed by folding the distal margin of the conduit to obtain a 1 cm nipple by suturing the mucosa to the skin with multiple interrupted 4–0 Vicryl sutures. Urethral catheters were left after surgery for seven days.
For the synthetic polymer scaffolds, the same process was followed, except for the harvesting of the ileal segment where the novel nanostructured polymer grafts were sutured rather than the ileal segment sutured into the bladder wall.
At time 0 and at the completion of the recovery period, urine and blood samples were collected and analyzed. After five and 11 weeks, the designated animals were euthanized and necropsy performed for harvesting the scaffolds and associated tissues for histological preparation and pathological examination. Standard hematoxylin and eosin stains were performed to assess bladder tissue generation and photographs were taken during biomaterial removal. Lastly, immediately after removal of the reconstructed bladder, the amount of water held by the reconstructed bladder was determined by filling the bladder with water until leakage by visual inspection. The amount of water held was then measured and reported as bladder capacity.
Statistical analysis
Quantitative data were analyzed using analysis of variance techniques with factorial designs. P values < 0.05 were considered to be statistically significant. All studies, unless otherwise noted, were run in triplicate per substrate type.
Results and discussion
Material characterization
When using a 9:1 salt to polymer ratio, the theoretical porosity of the PU-PLGA graft synthesized in the present study was 82.6%. The measured porosity from scanning electron microscopic images was 87.6%, which may be a result of the slightly larger amount of salt particles added during experiments. The size of the pores was determined by the size of the sieved salt particles which was between 150 μm and 250 μm, as shown in . Regardless, the porosity and pore size achieved meet the design criteria of creating a significant number of pores to increase bladder tissue growth and alter the mechanical properties to match that of bladder tissue.Citation1
Figure 2 Porous structures of the PU-PLGA graft. Pore size was determined by sieved salt particle sizes, which had a size range of 150–250 μm.
Abbreviations: PU, polyurethane; PLGA, poly-lactic-co-glycolic acid.
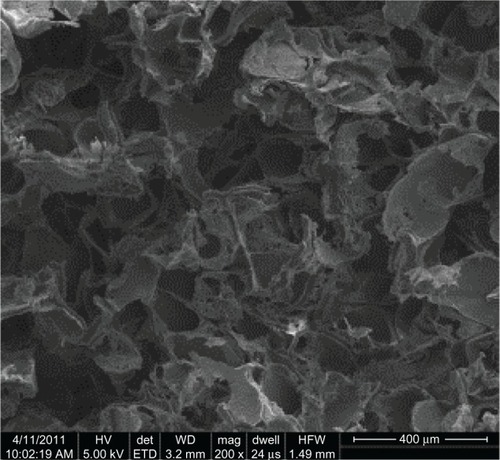
As mentioned, both the PU and PLGA sides of the graft were modified by chemical etching to possess nanometer rough surface features. The nontreated PU surface was very smooth without any noticeable features under 10 μm (). In comparison, HNO3-etched PU had many submicron to nanometer rough pores on the surface (). Similarly, the PLGA surfaces were modified by soaking in NaOH to have nanometer rough surface features (). From the images, as expected, it is obvious that some PLGA was etched away by the chemical treatment. Thus, it was clear that the present PU-PLGA composites possessed the desired nanoscale surface features previously shown to promote bladder cell responses.Citation1 Atomic force microscopic images () and roughness analysis () further confirmed the qualitative findings from scanning electron microscopy that the nanotreated PLGA and PU were much more rough at the nanoscale than their original surfaces, respectively.
Figure 3 Scanning electron microscopic images of (A) PU control, (B) nanomodified PU, (C) PLGA control, and (D) nanomodified PLGA. Scale bars = 10 μm. Insets in (C) and (D) are unmodified and nanomodified porous PLGA layers under low magnification, respectively.
Abbreviations: PU, polyurethane; PLGA, poly-lactic-co-glycolic acid.
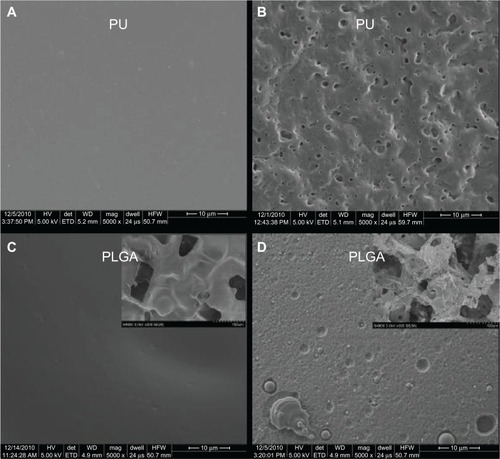
Figure 4 Atomic force micrographs of (A) conventional PU, (B) nanomodified PU, (C) conventional PLGA, and (D) nanomodified PLGA. Scale bars = 1 μm.
Abbreviations: PU, polyurethane; PLGA, poly-lactic-co-glycolic acid.
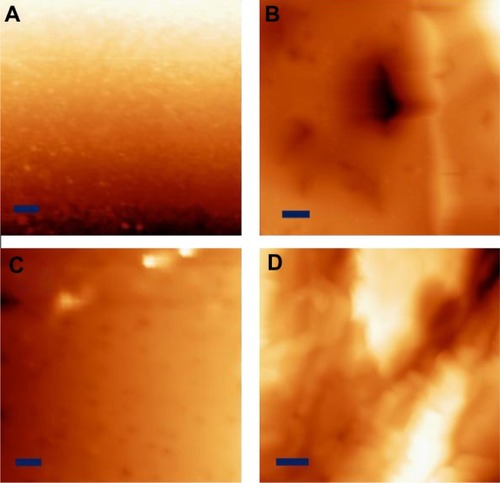
Figure 5 RMS roughness of PU and PLGA polymers at different scales.
Abbreviations: AFM, atomic force microscopy; PU, polyurethane; PLGA, poly-lactic-co-glycolic acid; RMS, root mean square.
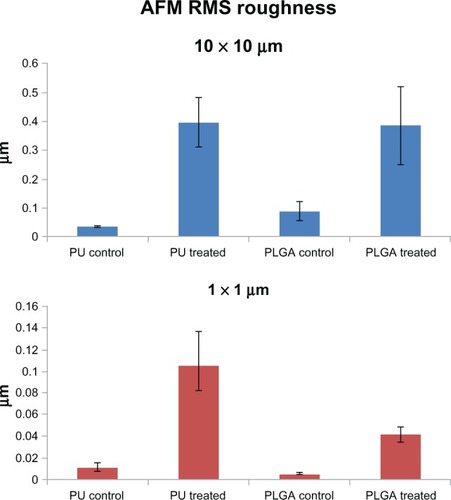
As a result of this change in surface roughness at the nanoscale, as expected, the chemically etched PU showed greater hydrophobicity (transitioning from contact angles of 74–85 degrees, ). In contrast, the fat PLGA was hydrophobic (around 101 degrees) while the porous (150–250 μm) nanorough PLGA was hydrophilic (84 degrees). Such results are expected due to the ether bond breakage in PU and ester bond breakage in PLGA. The reference material, ie, small intestinal submucosa, had a water contact angle about 60 degrees.Citation16
Figure 6 Water contact angles for PU and PLGA surfaces before and after surface modification.
Abbreviations: PU, polyurethane; PLGA, poly-lactic-co-glycolic acid.
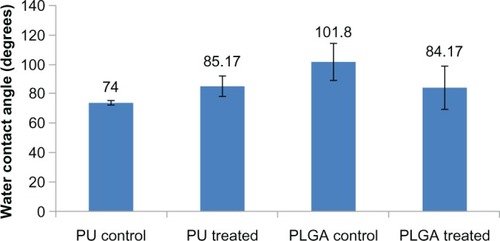
Lastly, x-ray photoelectron spectroscopy was used to confirm covalent bonding of the two peptides of interest in the present study, IKVAV and YIGSR, onto the polymer surfaces (). With the presence of only EDC and NHS, there was no significant increase in nitrogen content after an hour of soaking with polymer graft and rinsing afterwards (, 0.2% for PLGA and 0.3% for PLGA soaked with EDC/NHS). In comparison, with the presence of both EDC/NHS and the abovementioned peptides, there was a significant increase in nitrogen content (1.3%) on the polymer surface after an hour of reaction and rinsing afterwards, indicating that both peptides were present.
Table 1 X-ray photoelectron spectroscopic analysis of compositions on peptide-functionalized PLGA showing the same results for peptides
Graft mechanical strength
Equally as important to determining material surface properties of the proposed PU-PLGA scaffolds, it is critical to determine their mechanical properties for assessment of their suitability for application in bladder tissue regeneration. For this, as mentioned, the mechanical strength of the graft materials was assessed using three mechanical tests (tensile, ball burst, and suture pullout) and carried out using the aforementioned American Society of Testing Material standards. The results showed that the tensile strength of the seven-layer PU-PLGA construct was about 0.71 MPa, which was two times higher than normal bladder tissue strength (0.54 MPa). When compared with multilayered small intestinal submucosa, the synthetic polymer scaffold showed similar load at yield but a lower stiffness (slope of curve).Citation16 It is noted that there were small drops along the curve that could be indications of PU-PLGA layer breakage (). Moreover, the PU-PLGA polymer graft showed maximum force similar to that of four-layer small intestinal submucosa material in the ball burst and suture pullout tests ( and ).Citation16 Again, the polymer graft showed much larger displacement and, thus, lower stiffness. In summary, the mechanical tests provided evidence of mechanical properties (tensile, ball burst, and suture pullout) similar to those of natural bladder tissue and small intestinal submucosa ().Citation16
Table 2 Summary of mechanical testing of proposed nanostructured PU-PLGA graft versus design criteria
Figure 7 Tensile test plot of PU-PLGA grafts (left) compared with single and multilayered SIS grafts.Citation16
Abbreviations: PU, polyurethane; PLGA, poly-lactic-co-glycolic acid; SIS, single layered small intestinal submucosa; MLSIS, multilayered small intestinal submucosa.
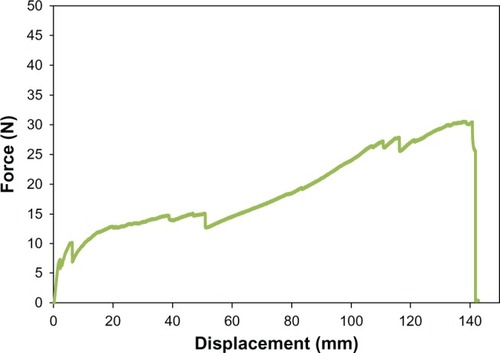
Figure 8 Ball burst test plot of PU-PLGA grafts (left) compared with single and multilayered SIS grafts.Citation16
Abbreviations: PU, polyurethane; PLGA, poly-lactic-co-glycolic acid; SIS, single layered small intestinal submucosa; MLSIS, multilayered small intestinal submucosa.
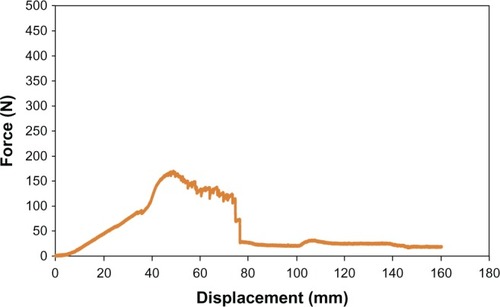
Figure 9 Suture pullout test plot of PU-PLGA grafts (left) compared with single and multilayered SIS grafts.Citation16
Abbreviations: PU, polyurethane; PLGA, poly-lactic-co-glycolic acid; SIS, single layered small intestinal submucosa; MLSIS, multilayered small intestinal submucosa.
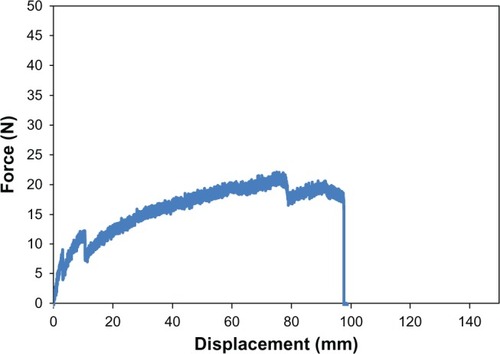
Degradation
The results of the present study also provide evidence of similar degradation properties between nanomodified PLGA-PU and control composites for up to 23 weeks, with approximately 40 wt% degradation for both materials after 23 weeks ().
Blood and urine tests
As mentioned, urine and blood was collected from animals with either the ileal segment or polymer graft the day after surgery and before sacrifice five and 11 weeks later ( and ). For urinalysis, the four animals (labeled as 03–38 and 03–24 for the five-week implantation time and 00–18 and 00–89 for the 11-week implantation time) with the ileal grafts all had yellow hazy urine at a pH of around 6.5 the day after surgery. The 03–24 animal showed a trace of blood and a few red blood cells. The 00–18 animal showed a few white blood cells, a few bacteria, and a few epithelial cells. The 00–89 animal showed a few white blood cells and a few epithelial cells. After five weeks, the urinary pH was 7.5 or 8. The 03–38 animal showed blood, a moderate number of white blood cells, a moderate number of red blood cells, and a moderate number of epithelial cells. The other animal (03–24) showed a moderate number of white blood cells, a moderate number of epithelial cells, and a moderate amount of oxalate crystals. After 11 weeks, the 00–18 animal showed a trace of blood, a few white blood cells, a moderate number of red blood cells, and a few epithelial cells. The 00–89 animal showed a trace of blood, a marked number of white blood cells, a moderate number of red blood cells, and a moderate number of epithelial cells.
Table 3 Urinalysis of ileal-grafted and polymer-grafted animals
Table 4 Selected cells detected in blood samples from animals with an ileal or polymer graft
The four animals (labeled 00–40 and 06–13 for the five-week implantation time and 03–41 and 03–27 for the 11-week implantation time) with polymer grafts also had yellow hazy urine at a pH of 6.5–7.5 the day after surgery. The 03–41 animal showed a few white blood cells and a few epithelial cells. The 03–27 animal showed a trace amount of blood, a few white blood cells, a moderate number of red blood cells, a marked number of bacteria, a few epithelial cells, and a few crystals in the urine samples. After five weeks, the 06–13 animal showed blood, a marked number of white blood cells, a moderate number of red blood cells, a moderate number of bacteria, a moderate number of epithelial cells, and a marked number of crystals in the urine. After 11 weeks, the 03–41 animal showed a trace of blood, a moderate number of white blood cells, a few red blood cells, and a few epithelial cells. The 03–27 animal showed a moderate number of white blood cells, a moderate number of bacteria, a few epithelial cells, and moderate crystal formation. In summary, the results of urine tests showed no major differences between the use of an ileal segment versus a polymer graft.
Comparing the presence of immune cells in the blood between the animals receiving the ileal segment or the PLGA-PU nanocomposite, animals with polymer grafts had neutrophil, lymphocyte, and monocyte levels similar to or lower than those in animals with an ileal graft at the three different time points (0, five, and 11 weeks). For both groups, blood collected the day after surgery showed a higher number of neutrophils than that collected after five or 11 weeks. The number of reticulocytes and platelets showed no obvious trends. In summary, blood tests provided evidence of a stronger immune reaction (due to the presence of more immune cells in the blood) when using the ileal segment compared with the polymer grafts; of note, use of an ileal segment as a bladder replacement material required two separate surgeries which clearly increases the chance of an immune response.
There was one animal in the ileal segment group that showed signs of contamination in the urine the day after surgery. In addition, one animal in the nanostructured polymer graft group showed signs of contamination the day after surgery and moderate contamination after 11 weeks. Another animal with the polymer graft showed moderate contamination after five weeks. The appearance of bacteria may be attributed to either contamination during the procedures or, less probably, endotoxins inside the graft. The plasma sterilization method used in this study is recognized by different researchers but has not been validated for the present specific bladder regeneration application. It is important to note that previous in vitro studies have demonstrated less bacteria adhesion and growth on nanostructured PLGA and PU compared with conventional PLGA and PU as prepared in this study.
Bladder capacity
The ileal segment and polymer grafts were able to withstand the same pressure after five and 11 weeks of implantation (40 cm H2O, ). The average bladder capacity of the minipigs which underwent ileal segment augmentation was 425 mL after five weeks and 630 mL after 11 weeks. Importantly, the average bladder capacity of minipigs who underwent polymer graft augmentation was 295 mL after five weeks and 645 mL after 11 weeks, showing equivalence with the ileal segment group after 11 weeks.
Table 5 Capacity of bladder with an ileal segment graft or polymer graft after five and 11 weeks (n = 2)
Integration of ileal segments and polymer grafts into the bladder
Most importantly, macroscopic images () clearly showed that the ileal segments remained attached and viable in the resected portion of bladder wall after five and 11 weeks. Polymer grafts appeared to have partially formed tissue after five weeks and fully formed tissue across the entire resected area after 11 months. The estimated regenerated bladder area initially replaced by the ileal tissue segment and the polymer graft was 4 cm × 4 cm. Consistent with the observed equivalence of bladder capacity between the two groups after 11 weeks, the transplanted mucosal tissue of the ileal segment was estimated at 4 cm × 4 cm at 11 weeks while a 4 cm × 4 cm area of new bladder tissue had formed on the polymer graft after 11 weeks. Interestingly, after 11 weeks, a thin residual PU layer had started to separate from the newly formed bladder tissue. The thin residual PU layer was easily separated from the newly formed bladder tissue by gently pulling the PU.
Figure 11 Observation of ileal and polymer grafts five and 11 weeks after implantation. (A) Ileal graft at five weeks, (B) polymer graft at five weeks, (C) ileal graft at 11 weeks, and (D) polymer graft at 11 weeks. The polyurethane layer was easily separated from the newly formed bladder tissue with a gentle pull.
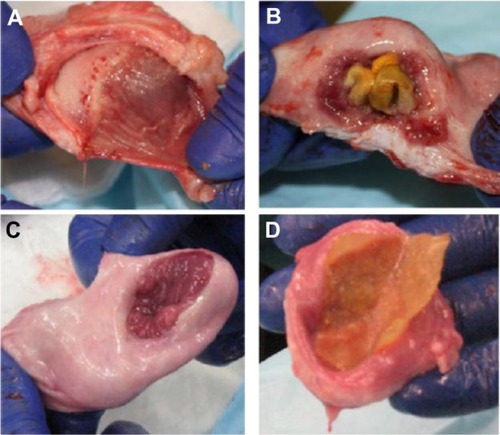
The thickness of the PU-PLGA polymer grafts decreased from around 2.5 mm to 0.5/0.3 mm after five weeks and to 0.3/0.3 mm after 11 weeks. Because the estimated thickness of the 100% PU layer was about 0.2 mm, it is reasonable to conclude that most of the PLGA components in the graft had been degraded after 11 weeks. Clearly, hydrolysis of PLGA in vivo is much faster than that in vitro. The nanostructured polymer graft lost most of its thickness after 11 weeks in the minipig but maintained the majority of its mass in vitro. This is due to the significant difference in conditions in the bladder (ie, dynamic fluid flow and composition of fluid) compared with the static in vitro testing conditions used. However, when compared with small intestinal submucosa, the PU-PLGA behaved similarly; that is, less than 10% of small intestinal submucosa remained three months after implantation in a pig studyCitation6 while 40% remained after one month with complete loss after three months in a canine study.Citation7
Of intrigue, is the separation of the nanostructured PU-PLGA graft from the bladder wall during bladder wall regeneration. Of course, this may allow for easy removal of the graft after integration (for example, using laparoscopy); however, care will need to be taken to match regeneration time carefully with graft removal. Clearly, future studies will be needed to take full advantage of this novel property of nanostructured PU-PLGA grafts to separate from bladder tissue after bladder tissue regeneration.
Histological analysis of urothelial and smooth muscle tissue regeneration
On histological examination, the transplanted ileal segment itself appeared to integrate well into the bladder tissue after five and 11 weeks of implantation ( and ). Specifically, after five weeks, a layer of urothelium was observed across the site of the nondegradable sutures used. However, no smooth muscle cell layer had formed after five weeks. After 11 weeks, both urothelium and smooth muscle formed on the newly created bladder tissue when using the ileal segment. The remodeling process between the original ileal epithelial cells and the regenerated urothelial cells is unclear and will require further experiments.
Figure 12 Ileal segment histology showing newly formed urothelial layers after five weeks (A and B) and formation of both urothelial and smooth muscle tissue after 11 weeks (C and D) on the transplanted ileal segment. Scale bar = 1 μm.
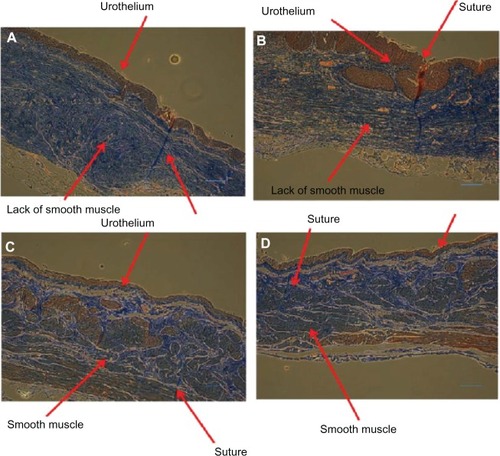
Figure 13 Nanomodified polymer graft histology showing newly formed urothelial and smooth muscle layers after five weeks (A–D) and continued maturation of urothelial and smooth muscle tissue after 11 weeks (E and F) on the newly formed tissue around the nanomodified polymer graft. Scale bar = 1 μm.
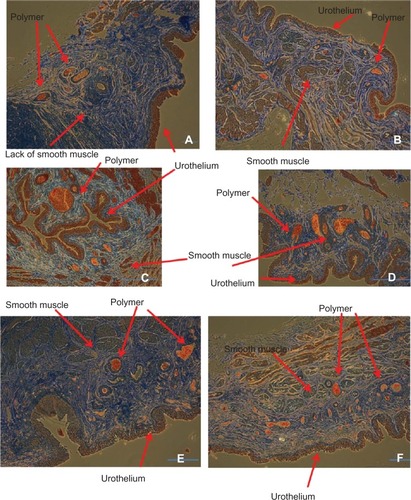
In contrast, after five weeks, both urothelial and smooth muscle cell layers formed on the nanostructured polymer graft ( and ). Remnants of the polymeric material (one micron or less) were observed next to the newly formed bladder walls, suggesting successful incorporation of new tissue into the pores of the graft. The composition of the polymer observed in the histological segments is not known, and will need to be determined in future studies. If it is residual PLGA (as expected), the polymer remnants would be expected to degrade completely with sufficient time. After 11 weeks, both the urothelium and smooth muscle were consistently and continuously formed on the new bladder wall tissue with the incorporated polymer present.
Conclusion
A synthetic polymer graft comprised of nanostructured PU and nanostructured PLGA was designed for partial bladder replacement. The graft was fabricated to have porosity to support infiltration of bladder tissue into all sides of the graft. Both polymer surfaces were modified to possess nanostructured features and with IKVAV and YIGSR to enhance their interactions with cells. Mechanical tests confirmed that this polymer graft has appropriate mechanical properties for bladder wall replacement. This synthetic PU-PLGA nanostructured graft was assessed in a well established minipig model, and showed similar bladder capacity, similar regeneration size, and similar tissue microstructure for newly formed bladder tissues up to 11 weeks between the ileal segment and polymer graft. The PLGA matrix portion of the graft was fully desorbed and a thin residual PU layer which could be gently peeled away from the new bladder wall was observed. Taken together, these findings support the continued development of this nanostructured resorbable synthetic graft for partial bladder and urogenital repair as well as neobladder replacement. Thus, this synthetic PU-PLGA nanostructured composite offers significant new clinical options for the treatment of patients suffering from numerous bladder problems, including congenital defects, bladder cancer, and other bladder trauma.
Acknowledgments
The authors would like to acknowledge the National Cancer Institute of the National Institutes of Health for financially supporting the present study (grant 1R41CA136033-01A2).
Disclosure
The authors CY, MH, and TJW are employees of Nanovis LLC, IN, USA. All other authors declare no conflicts of interest in this work.
References
- ThapaAWebsterTJHaberstrohKMNano-structured polymers enhance bladder smooth muscle cell functionBiomaterials2003242915292612742731
- ThapaAWebsterTJHaberstrohKMNano-structured polymers enhance bladder smooth muscle cell adhesionJ Biomed Mater Res200367A13741383
- TanziMCMantovaniDPetriniPGuidoinRLarocheGChemical stability of polyether urethanes versus polycarbonate urethanesJ Biomed Mater Res1997365505599294772
- TanziMCFareSPetriniPIn vitro stability of polyether and polycarbonate urethanesJ Biomater Appl20001432534810794506
- FareSPetriniPMottaACigadaATanziMCSynergistic effects of oxidative environments and mechanical stress on in vitro stability of polyetherurethanes and polycarbonateurethanesJ Biomed Mater Res199945627410397959
- MillerDCThapaAHaberstrohKMWebsterTJEnhanced functions of vascular cells on nanostructured polymersBiomaterials200425536114580908
- VanceRMillerDThapaAHaberstrohKWebsterTJDecreased fibroblast cell density on chemically degraded poly-lactic-glycolic acid, polycaprolactone, and polyurethaneBiomaterials2004252095210314741624
- KaySThapaAHaberstrohKMWebsterTJNanostructured polymer: nanophase ceramic composites enhance osteoblast and chondrocyte adhesionTissue Eng2002875376112459054
- MachadoMTarquinioKWebsterTJDecreased S. aureus biofilm formation on nanomodified ETTs: an airway dynamic modelInt J Nanomedicine201273741375022904622
- GeilichBWebsterTJReduced adhesion of Staphylococcus aureus to ZnO/PVC nanocompositesInt J Nanomedicine201381823293515
- HoltonPDShinarZBennaJPatzakisMJPorcine small intestine submucosa does not show antimicrobial propertiesClin Orthop Relat Res2004427182415552130
- ChunYWKhangDHaberstrohKMWebsterTJThe role of polymer nanosurface roughness and submicron pores in improving bladder urothelial cell density and inhibiting calcium oxalate stone formationNanotechnology20092008510419417440
- TaylorEWebsterTJReducing infections through nanotechnology and nanoparticlesInt J Nanomedicine201161463147321796248
- JerniganTWCroceMACagiannosCShellDHHandorfCRFabianTCSmall intestinal submucosa for vascular reconstruction in the presence of gastrointestinal contaminationAnn Surg200423973373815082978
- YaoCHedrickMWebsterTDecreased bacteria adhesion and growth on nanostructured polymersInt J Nanomedicine
- ArnoldGAMathewsKGRoeSMentePSeabochTBiomechanical comparison of four soft tissue replacement materials: an in vitro evaluation of single and multilaminate porcine small intestinal submucosa, canine fascia lata, and polypropylene meshVet Surg20093883484419781026
- DanczykRCKriederBNorthAWebsterTJHogeneschHRundellAComparison of antibody functionality using different immobilization methodsBiotechnol Bioeng20038421522312966578