Abstract
Background
Graphene is a single-atom thick, two-dimensional sheet of hexagonally arranged carbon atoms isolated from its three-dimensional parent material, graphite. One of the most common methods for preparation of graphene is chemical exfoliation of graphite using powerful oxidizing agents. Generally, graphene is synthesized through deoxygenation of graphene oxide (GO) by using hydrazine, which is one of the most widespread and strongest reducing agents. Due to the high toxicity of hydrazine, it is not a promising reducing agent in large-scale production of graphene; therefore, this study focused on a green or sustainable synthesis of graphene and the biocompatibility of graphene in primary mouse embryonic fibroblast cells (PMEFs).
Methods
Here, we demonstrated a simple, rapid, and green chemistry approach for the synthesis of reduced GO (rGO) from GO using triethylamine (TEA) as a reducing agent and stabilizing agent. The obtained TEA reduced GO (TEA-rGO) was characterized by ultraviolet (UV)–visible absorption spectroscopy, X-ray diffraction (XRD), particle size dynamic light scattering (DLS), scanning electron microscopy (SEM), Raman spectroscopy, and atomic force microscopy (AFM).
Results
The transition of graphene oxide to graphene was confirmed by UV–visible spectroscopy. XRD and SEM were used to investigate the crystallinity of graphene and the surface morphologies of prepared graphene respectively. The formation of defects further supports the functionalization of graphene as indicated in the Raman spectrum of TEA-rGO. Surface morphology and the thickness of the GO and TEA-rGO were analyzed using AFM. The presented results suggest that TEA-rGO shows significantly more biocompatibility with PMEFs cells than GO.
Conclusion
This is the first report about using TEA as a reducing as well as a stabilizing agent for the preparation of biocompatible graphene. The proposed safe and green method offers substitute routes for large-scale production of graphene for several biomedical applications.
Introduction
Graphene is a two-dimensional (2D) atomic crystal which consists of carbon atoms arranged in a hexagonal lattice.Citation1–Citation3 Graphene and related nanomaterials have received considerable attention due to their high surface area and unique physical and chemical properties.Citation4 This unique chemical structure gives graphene excellent electrical, mechanical, and optical properties, thus attracting much commercial and academic research interest.Citation2 Therefore, many synthetic strategies for graphene have been developed with the goal of making large quantities of high-quality graphene in a cost effective manner.Citation5 For the synthesis of high quality graphene, epitaxial growth and chemical vapor deposition methods are generally employed; however, these methods are not compatible with large-scale synthesis in solution-processable form and they require expensive high-temperature processes.Citation6,Citation7 Effective and scalable approaches are essential processes required for various practical applications of graphene.Citation1 However, most applications depend on availability of processable reduced graphene oxide (rGO) in large quantities; therefore, the development of efficient and cost-effective approaches has been the focus of substantial attention. Several methodologies are available, including micromechanical exfoliationCitation2 and chemical reduction of graphene oxide (GO).Citation8,Citation9 Although several techniques are available, the chemical reduction of GO has proven to be a promising method due to its low cost, the ease of bulk quantity production, and because it is well suited for chemical modification and subsequent processing.Citation10–Citation13 In general, hydrazine and hydrazine derivatives were usually employed as a strong reducing agent in the formation of graphene with high conductivity.Citation11–Citation16 The majority of the reducing agent is either toxic or explosive and is difficult to handle in larger scale production.
However, strong and toxic reduction agents and surfactants are essential to reduce GO fully in an aqueous phase.Citation17 To solve this problem, many studies have attempted to develop a new aqueous and environmentally-friendly reduction strategy using bacterial respiration,Citation18 polyallylamine,Citation19 potassium hydroxide (KOH),Citation20 polyvinylpyrrolidone,Citation21 ascorbic acid,Citation22 sugar,Citation23 Baker’s yeast,Citation24 and protein.Citation25 Among the various nontoxic reduction agents, protein has significant advantages because adsorbed proteins can be harnessed as a universal type of glue for various nanomaterials used in nanocomposite synthesis. Dextran is used as a multifunctional agent for GO reduction and functionalization.Citation26 Recently, our group developed an environmentally-friendly and simple approach for synthesis of water soluble graphene.Citation27–Citation29
Several studies have been published regarding toxicity of graphene both in bacteria and human cell types. The effect of GO and rGO on the growth of Escherichia coli has been noted, and they have been observed with minimal toxicity in human alveolar epithelial A549 cells as well.Citation30 Akhavan and GhaderiCitation31 compared the toxicity levels of graphene and GO nanowalls in E. coli and Staphylococcus aureus bacteria and found that GO and rGO caused bacterial membrane damage and that the hydrazine-rGO was more toxic than untreated GO. Akhavan et alCitation32 investigated the cytotoxic effects of the graphene incorporated in the titanium oxide (TiOCitation2) film against Caenorhabditis elegans nematodes, as a simple and well-established animal model, under solar light-induced stress conditions. Zhang et alCitation33 demonstrated that both GO and carbon nanotubes (CNTs) induced cytotoxic effects on pheochromocytoma (PC-12) cells; the authors concluded that CNTs are more toxic than graphene and that the shape of these carbon-based nanomaterials plays an important role in their cytotoxicity. Recently, Wang et alCitation34 demonstrated that GO has dose-dependent toxicity to human fibroblast cells, with GO causing obvious toxicity when the dose was higher than 50 μg/mL. Chang et alCitation35 suggested GO will not enter A549 cells and that GO shows no obvious toxicity to A549 cells, regardless of the size or dose of GO. Liao et alCitation36 demonstrated the biocompatibility of graphene-related materials with controlled physical and chemical properties and also explained that the toxicity of graphene and GO depends on their exposure environment. Further, Akhavan et alCitation37 demonstrated the size-dependent cytotoxic and genotoxic effects of the reduced graphene oxide nanoplatelets on human mesenchymal stem cells (hMSCs).
Regarding biocompatibility of graphene materials, Yan et alCitation38 developed free-standing GO-Polyaniline(PANI) and graphene-PANI hybrid papers via rapid mixture polymerization of aniline on the surfaces of GO and graphene papers; these exhibit excellent biocompatibility, flexibility, electrochemical activity. Liu et alCitation39 have developed GO-based glucose sensors and have also shown excellent reproducibility and significant biocompatibility to human retinal pigment epithelial cells. In recent years, graphene sheets with various lateral dimensions have been applied in tissue engineering as scaffolds to support cellular attachment, proliferation, and differentiationCitation40–Citation42 in photothermal cancer therapy, as they are excellent near infrared (NIR) absorbent nanomaterials.Citation43,Citation44 Chen et alCitation45 have developed biocompatible, mechanically strong, electrically conductive graphene paper; its preliminary cytotoxicity tests suggest that biocompatibility of this material would result in attractive potential applications. Park et alCitation46 have fabricated a strong and biocompatible freestanding paper composed of Tween 20 and chemically reduced GO, which exhibits excellent stability in water. The Tween 20 paper and the rGO paper were noncytotoxic to three mammalian cell lines, while only the Tween 20 paper inhibited the nonspecific binding (NSB) of bacterial cells. Functionalized graphene shows excellent compatibility with red blood cells, platelets, and plasma coagulation pathways, and minimal alteration in the cytokine expression by human peripheral blood mononuclear cells.Citation47 Singh et alCitation48 have demonstrated that amine-modified graphene (G-NHCitation2) had neither a stimulatory effect on human platelets, nor did it induce pulmonary thromboembolism in mice.
In this study, we used primary mouse embryonic fibroblasts (PMEFs) as a model system. In broad-spectrum, PMEFs are used in the culturing of mouse embryonic stem (ES) cells, providing both a substrate for the ES cells to grow on and secreting many factors necessary for ES cells to maintain pluripotency. In addition, PMEFs cells are capable of undergoing a limited number of population doublings before entering crisis and senescence-like growth arrest; they are the ideal model system for studying aspects of cell growth control and functional aspects.
Synthesis of biocompatible graphene is essential for various biomedical applications. Herein, we demonstrate a simple, quick, and room temperature-mediated synthesis of highly soluble graphene using triethylamine (TEA); we also investigate the biocompatibility of synthesized graphene in PMEFs.
Materials and methods
Materials
Graphite powder was purchased from Sigma-Aldrich (St Louis, MO, USA). TEA, sodium hydroxide (NaOH), potassium permanganate (KMnO4), anhydrous ethanol, 98% sulfuric acid (H2SO4), 36% hydrochloric acid (HCl), and 30% hydrogen peroxide (H2O2) aqueous solution were purchased from Sigma-Aldrich and used directly without further purification. All aqueous solutions were prepared with deionized (DI) water. All other chemicals were purchased from Sigma-Aldrich unless stated otherwise.
Synthesis of GO
Natural graphite powder was utilized as the raw material to prepare graphite oxide according to suspension using a modified Hummers’ methodCitation10 and Esfandiar et al.Citation49 The prepared graphite oxide powder was dispersed in DI water to obtain an aqueous graphite oxide suspension with a yellow-brownish color. The suspension was centrifuged at 3000 rpm/minute for 10 minutes to eliminate unexfoliated graphitic plates, and then at 10,000 rpm/minute for 10 minutes to remove tiny graphite particles. Finally, GO suspension was achieved by exfoliation of the filtered graphite oxide suspension through its sonication.
Reduction of GO by TEA
Reduction of GO was followed as described earlier.Citation49 rGO was obtained from the reaction of TEA with GO. Typically, the final concentration of 0.1% (v/v) TEA was dissolved in 20 mL GO aqueous suspension (0.1 mg/mL) and then the mixture was kept in a tightly sealed glass bottle and stirred at 30°C for 1 hour. Using a magneto-stirrer heater, TEA-GO suspension was then stirred at 400 rpm, at a temperature of 30°C, for 30 minutes. A homogeneous TEA-rGO suspension was obtained without aggregation. Finally, the functionalized TEA-rGO was filtered and washed with DI water.
Characterization
Ultraviolet-visible (UV-vis) spectra were recorded using a WPA (Biowave II; Biochrom, Cambridge, UK). The aqueous suspension of GO and TEA-rGO was used as the UV–vis sample and the DI water was used as a reference. The particle size of dispersions was measured by Zetasizer Nano ZS90 (Malvern Instruments, Malvern, UK). X-ray diffraction (XRD) analyses were carried out on an X-ray diffractometer (Bruker D8 DISCOVER, Bruker AXS GmBH, Karlsruhe, Germany). The high resolution XRD patterns were measured at 3 kW with Cu target using a scintillation counter (λ = 1.5406°A) at 40 kV and 40 mA; they were recorded in the range of 2θ = 5°–80°. The solid samples were transferred to a carbon tape held in a scanning electron microscopy (SEM) sample holder for analyses. The analyses of the samples were carried out at an average working distance of 6 mm. Raman spectra of GO and rGO were measured by WITEC Alpha300 (Witec, Ulm, Germany) with a 532 nm laser. The calibration was initially made using an internal silicon reference at 500 cm−1 and gave a peak position resolution of less than 1 cm−1. The spectra were measured from 500–4500 cm−1. All samples were deposited on glass slides in powder form without using any solvent. Surface images were measured using tapping mode atomic force microscopy (TM-AFM) (SPA 400, SEIKO Instruments, Chiba, Japan) operating at room temperature. Height and phase images were recorded simultaneously using nanoprobe cantilevers (SI-DF20, SEIKO Instruments).
Cell culture and exposure to GO and TEA-rGO
PMEFs cells were routinely cultured in Dulbecco’s Modified Eagle’s low glucose medium (DMEM/low, Life Technologies, Carlsbad CA, USA) supplemented with 10% (v/v) fetal bovine serum (FBS), plus 2 mM L-glutamine, 1% (v/v) penicillin-streptomycin (10 U/mL penicillin and 0.1 mg/mL streptomycin); they were grown at 37°C in a 5% carbon dioxide (CO2) humidified environment. When the cells had reached 70% confluence, they were trypsinized (0.25% Trypsin–0.04% ethylenediaminetetraacetic acid [EDTA], Sigma-Aldrich) and passaged (1:3). Cells within three passages were used for experiments. GO or TEA-rGO suspensions were freshly prepared before the cells were exposed and diluted to appropriate concentrations from 20–100 μg/mL with the culture medium, then immediately applied to the cells. Cells that were not treated with GO or TEA-rGO served as controls in each experiment.
Cell viability assay
WST-8 assay was followed as described earlier by Liao et al.Citation36 Typically, 1 × 104 cells were seeded in a 96-well plate and cultured in DMEM supplemented with 10% serum at 37°C under 5% CO2 After 24 hours, the cells were washed with 100 μL of serum-free DMEM two times and incubated with 100 μL of different concentrations of GO or TEA-rGO suspensions in serum-free DMEM. After 24 hours exposure, the cells were washed twice with serum-free DMEM and 15 μL of WST-8 solution was added to each well containing 100 μL of serum-free DMEM. After 1 hour incubation at 37°C under 5% CO2, 80 μL of the mixture was transferred to another 96-well plate, because residual GO or TEA-rGO can affect the absorbance values at 450 nm. The absorbance of the mixture solutions was measured at 450 nm using a microplate reader. Cell-free control experiments were performed to see if the GO and rGO reacted directly with the WST-8 reagents. Typically, 100 μL of GO or TEA-rGO suspensions with different concentration (20–100 μg/mL) were added to a 96-well plate and 10 μL of WST-8 reagent solution was added to each well; the mixture solution was incubated at 37°C under 5% CO2 for 1 hour. After incubation, the GO or TEA-rGO were centrifuged and 50 μL of supernatant was transferred to another 96-well plate. The optical density was measured at 450 nm.
Membrane integrity
Cell membrane integrity of PMEFs cells was evaluated by determining the activity of lactate dehydrogenase (LDH) leaking out of the cell, according to the manufacturer’s instructions for the in vitro toxicology assay kit (TOX7, Sigma-Aldrich). The LDH assay is based on the release of the cytosolic enzyme, LDH, from cells with damaged cellular membranes. Thus, in cell culture, the course of GO and TEA-rGO induced cytotoxicity was followed quantitatively by measuring the activity of LDH in the supernatant. Briefly, cells were exposed to various concentrations of GO and TEA-rGO for 24 hours and then 100 μL per well of each cell-free supernatant was transferred in triplicate into wells in a 96-well plate; 100 μL of LDH-assay reaction mixture was then added to each well plate. After a 3-hour incubation under standard conditions, the optical density of the color generated was determined at a wavelength of 490 nm using a microplate reader.
Cell population growth assay
The cell population growth assay followed the method described earlier.Citation50 Cells were plated in six well culture plates at a density of 1 × 104/well in 1 mL of DMEM supplemented with 10% FBS. The cells were allowed to attach and media were replaced with various concentrations of GO or TEA-rGO (0–20 μg/mL) in triplicate. After treatment with three experimental conditions for 24 hours, cells were harvested with 0.05% trypsin and 0.02% EDTA in phosphate buffered saline (PBS), and counted. Data are expressed as number of cells.
Cell attachment assay
The cell attachment assay followed the method described earlier.Citation50 To coat the plates with GO and TEA-rGO, a 1 mL solution of 20 μg/mL in PBS was added in 35 mm tissue culture plates. Plates were allowed to sit at 37°C for 2 hours and then the solution was removed and plates were washed with PBS. To coat the plates with gelatin, a 1 mL solution of 0.2% gelatin in PBS was added to 35 mm tissue culture plates, and coated plates were left at room temperature for 2 hours. Cells were seeded at a density of 1 × 104 cells ⁄well in various coated plates and incubated for 24 hours at 37°C in 5% CO2. After incubation, unattached cells were gently removed with 1 mL PBS. Attached cells were harvested with trypsin and cells were counted as described above. Cells in uncoated plates were used as negative control. Data are expressed as percentage of attached cells compared to initial cells.
Results and discussion
Characterization of GO and TEA-rGO
shows the conversion of GO to graphene, which is confirmed by the color change from pale yellow to black, following the chemical reduction. Such observation provides a piece of evidence to support the formation of rGO.Citation51 The UV-vis absorption spectra of the GO and rGO suspensions are exhibited in . After reduction of the GO by TEA, the color of the GO suspension changed from light brown to black. The black color of the rGO indicates removal of oxygen-containing bonds, resulting in electronic conjugation within reduced sheets.Citation52 The optical absorption spectra also show that the absorption peak of the GO suspension is around 231 nm, while the absorption peaks of the reduced suspensions shift into wavelengths of around 260 nm, corresponding to deoxygenation of the GO suspension under the reduction processes. The results confirm the transition of GO to graphene.
Figure 1 Digital photographs of aqueous dispersions of GO before and after reduction with TEA.
Notes: Digital photographs of aqueous dispersions (0.5 mg/mL) of GO before (A) and after (B) the reduction with TEA, which were kept at 30°C for 60 minutes.
Abbreviations: GO, graphene oxide; TEA, triethylamine.
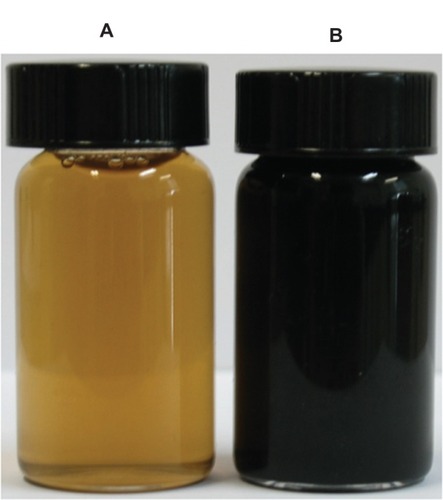
X-ray diffraction analysis
Further characterization of GO and TEA-rGO was carried out by X-ray diffraction. The characterized peak of GO appears at 2θ = 11.7° nm, corresponding to a d-spacing of 0.76 nm (). In contrast to GO, the TEA-rGO shows two peaks; one peak appears at 10.6°, corresponding to d-spacing of 2.1 nm; another broader peak is centered at 25.5°, corresponding to a d-spacing of 0.36 nm (), which may be a result of some restacked graphene layers. Note that this spacing is very close to pristine graphite, indicating that the functional groups of GO have been efficiently removed. The interlayer spacing decreased to 0.35 nm, which suggests that removal of oxygen and water from the interlayer occurred during exfoliation, at a large rate. This broad peak is also suggestive of a loss of the long range order in graphenes.Citation53 Though there is a decrease in the interlayer spacing compared with GO, the basal spacing of rGO is higher than that of well-ordered graphite (single-layer pristine graphene). The higher basal spacing may be due to the presence of residual oxygen functional groups, indicating incomplete reduction of GO.Citation54
Size distribution analysis by dynamic light scattering (DLS)
The DLS method was adopted to measure the size of GO and TEA-rGO in aqueous solution; DLS results could contribute to show the size differences between GO and rGO. As shown in , the results indicate that the hydrodynamic diameter of GO sheets in water is distributed from about 1645 nm in size. Upon reduction of GO by TEA, the particle size is shifted to an increase in size distribution with a hydrodynamic diameter centered around 2116 nm (). The rGO particles were formed by reducing GO nanosheets, and their size is slightly larger than that of GO particles because of the aggregation of TEA-rGO fragments. This obvious change in size distribution indicates that TEA not only acted as a reducing agent to prepare graphene, but it was also functionalized on the surfaces of the resulting rGO, leading to an increased Brownian motion rate after the reduction process. Wang et alCitation55 observed that after the reduction of GO with heparin, the average size of rGO was larger than GO. Liu et alCitation56 analyzed the size of various graphene based materials; among them GO nanosheets are the smallest in size. Although graphite oxide particles have similar chemical functionality to GO nanosheets, their average size is nearly 20 times larger than that of GO nanosheets. The rGO particles were formed by reducing GO nanosheets, and their size is about nine times larger than that of GO nanosheets because of the aggregation of rGO fragments.Citation56 In order to further confirm size, the dispersions were further dropped on aluminum foil and dozens of SEM images were taken randomly for each sample.
Figure 4 Hydrodynamic size distribution of GO and TEA-rGO.
Notes: Hydrodynamic size distribution of (A) GO and (B) TEA-rGO (500 μg/mL) measured by DLS at room temperature in DI water.
Abbreviations: GO, graphene oxide; TEA-rGO, triethylamine-reduced graphene oxide; DLS, dynamic light scattering; DI, deionized.
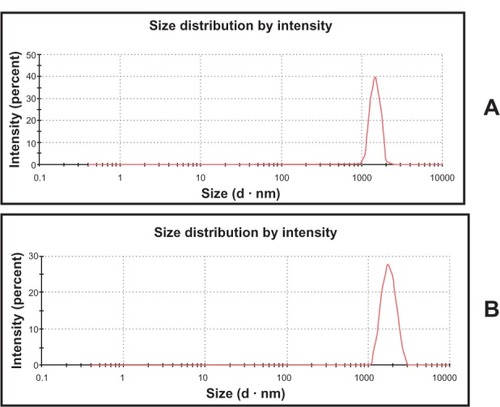
SEM analysis
SEM images of GO sheets were smooth with folded shapes at the edges; they also looked like waves (). A thin layer of TEA-rGO sheets displayed a crumpled and wrinkled structure; they look like typical folded paper (). The geometric wrinkling arising from π–π interaction within sheets of graphene not only minimizes the surface energy but also induces mechanical integrity with tensile strength and good film-forming ability.Citation57 In addition, the wrinkled structure of TEA-rGO sheets provides a large rough surface as a scaffold for further modification. Stankovich et alCitation13 observed that the reduced GO material consists of randomly aggregated, thin, crumpled sheets closely associated with each other and forming a disordered solid.
Raman spectroscopy
The Raman spectra further support the structural change before and after the reduction of graphite oxide.Citation58 Earlier reports suggested that graphene obtained by the chemical reduction method exhibits two characteristic main peaks: the D band at 1350 cm−1 and G band at 1575 cm−1.57,58 In our present work, we observed that GO showed a D band at 1395 cm−1 and G band at 1608 cm−1 respectively (). After reduction of GO, the rGO showed the corresponding bands of D and G band appearing at 1347 and 1575 cm−1, respectively (). The G band of TEA-rGO red-shifted from 1603 cm−1 to 1575 cm−1, which was recognized as recovery of the hexagonal network of the carbon atom. The intensity ratio of the D and G band (ID/IG) revealed structural defects and the indication of disorder. After reduction, the ID/IG ratio increased from 1.7 to 2.94 in TEA-rGO. Compared with the GO, the intensity ratio of TEA-rGO was high, which indicates high level defects. This change suggests that more SP2 domains are formed during the reduction of graphite oxide, which agrees very well with other reports.Citation14,Citation59,Citation60
Figure 6 Raman spectra of GO and TEA-rGO.
Notes: Raman spectra of (A) GO and (B) TEA-rGO.
Abbreviations: GO, graphene oxide; TEA-rGO, triethylamine-reduced graphene oxide; 2D, 2-dimensional; au, absorbance units.
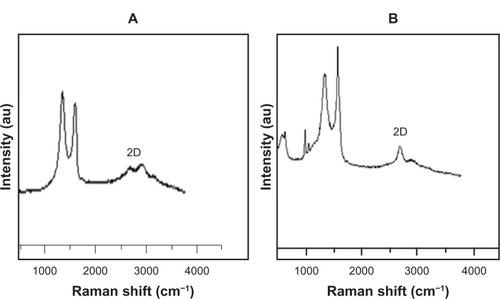
In order to see the quality of synthesized graphene, we used Raman spectroscopy, which is the potential candidate for nondestructive and quick inspection of multiple layers of graphene. Raman spectra of graphene-based materials showed a 2D band which was sensitive to stacking of graphene sheets. 2D Raman band shape and position are good fingerprints of single and bilayer graphene to distinguish them from the multilayer. We observed a significant feature and intensity of 2D bands 2670 located between 2600–3000 cm−1 in the Raman spectrum. As shown in , the 2D band of TEA-rGO centered about 2670 cm−1 with a low-intensity shoulder at the higher wave numbers, and we analyzed the peak intensity between 2D and G bands, the ratio of which is 2.2. The results suggest that the synthesized graphene could be a monolayer.Citation13,Citation49
Atomic force microscopy (AFM) analysis
We used an atomic force microscope to probe film morphology and thickness. AFM images confirm that evaporated dispersions of GO and graphene are comprised of isolated graphitic sheets. GO has lateral dimensions of several micrometers and a thickness of 1 nm, which is characteristic of a fully exfoliated GO sheet.Citation13 We have shown a typical AFM image of GO and rGO dispersion in water after their deposition on a freshly cleaved mica surface through drop casting method. We observed that the film morphology changes significantly with this dimension. are representative of the topology of films from GO and TEA-rGO solutions with concentrations in the 1 mg/mL range. GO and TEA-rGO sheets with the lateral size up to 5 μm were observed as shown in . As the prepared GO and rGO dispersion shows, the height profile of the AFM image is about 1.06 nm and 1.02 nm (), respectively. Compared with GO sheets, the rGO is less thick. We observed increased disorder and roughness and the appearance of micrometer-long wrinkles; it became increasingly hard to identify the edges of the separate sheets. Wrinkles on graphene’s surface, dried on the mica substrate, decreased its apparent thickness (1.02 nm) as measured by AFM (). The decrease in the lateral dimensions of graphene sheets is also attributed to excessive sonication during each step of the preparation of graphene.
Effect of GO and TEA-rGO on cell viability
The evaluation of biocompatibility of graphene materials is an important factor in biological and biomedical applications, including drug delivery and gene transfection. It has been found that WST-8 is an appropriate assay for cell viability and proliferation tests of graphene materials.Citation36 As shown in , GO shows a dose-dependent significant toxicity in PMEFs cells (P < 0.05); however the lower concentration of 20 μg/mL displayed neither toxic nor stimulatory effect, whereas TEA-rGO didn’t show an obvious decrease in cell viability up to the concentrations of 100 μg/mL, which suggests that functionalization of GO by TEA plays an important role in cell biocompatibility. Interestingly, the lower concentration of TEA-rGO (20 μg/mL) shows a significant stimulatory effect when compared to control (P < 0.05). Schrand et alCitation61 examined biocompatibility of various carbon nanomaterials such as nanodiamonds (NDs), single- and multi-walled carbon nanotubes (SWNTs, MWNTs), and carbon black (CB), at concentrations ranging from 25–100 μg/mL for 24 hours in neuronal and lung cell lines; the results indicated that these carbon nanomaterials displayed differential biocompatibility in these two different cell lines; however, the greatest biocompatibility was found after incubation with NDs and both cell types. Gelatin functionalized grapheme nanosheets shows nontoxicity in MCF-7 cells, even at a high concentration of 200 μg/mL. Mejías Carpio et alCitation62 investigated the toxicity of polyvinyl-N-carbazole (PVK)-graphene oxide (GO) with planktonic microbial cells, biofilms, and NIH 3T3 fibroblast cells, and the results suggested that the PVK–GO nanocomposite presents higher antimicrobial effects than the pristine GO; however, the same nanocomposite didn’t induce significant cytotoxicity in fibroblast cells. The cells exposed to the suspension of GO and functioning with polyethylene glycol (PEG) in the rGO/PEG concentration range between 3.125 μg/mL and 25 μg/mL show relatively high viability in mouse fibroblast cells.Citation63 Chen et alCitation64 reported that grapheme and GO can support mouse induced pluripotent stem cell (iPSC) culture and allow for spontaneous differentiation. Another report suggests that GO is a great support for mammalian cell attachment, growth, and proliferation.Citation65
Figure 8 Effect of GO and TEA-rGO on cell viability of PMEFs cells.
Notes: Cell viability of PMEFs cells was determined using WST-8 assay after 24 hours exposure to different concentrations of GO or TEA-rGO. The results represent the means of three separate experiments, and error bars represent the standard error of the mean. GO treated groups showed statistically significant differences from the control group by the Student’s t-test (P < 0.05).
Abbreviations: GO, graphene oxide; TEA-rGO, triethylamine-reduced graphene oxide; PMEFs, primary mouse embryonic fibroblast cells; au, absorbance units; WST, water-soluble tetrazolium salt.
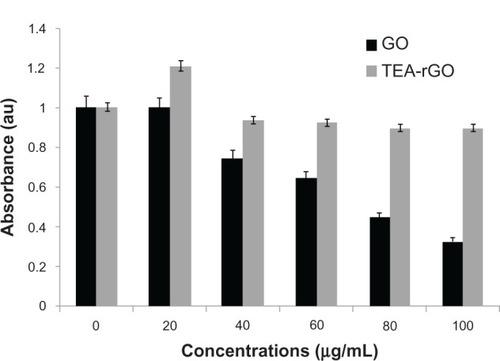
Impact of GO and TEA-rGO on membrane integrity
LDH is a soluble cytosolic enzyme which when released into the extracellular medium (due to membrane damage) consequently leads to apoptosis. It is widely accepted as an indicator of lytic cell death. LDH release measures the membrane damage, a hallmark of necrosis. To further support the biocompatibility of TEA-rGO in PMEFs cells, the cells were treated with various concentrations of GO and TEA-rGO for 24 hours and extracellular LDH activity was then measured. The results suggest that cell membrane integrity in PMEFs cells was compromised by GO and increasing the concentration of the GO leads to a higher amount of leakage in the LDH assay; this indicates the membrane integrity was compromised and cellular contents were found in the media, and in turn suggests that GO induces significant toxicity (P < 0.05) when compared to untreated cases. Interestingly TEA-rGO shows no effects on membrane damage up to 100 μg/mL (). LDH activity was slightly decreased when compared to control. Similar to our results, Chang et alCitation35 observed that the LDH levels of GO-treated cells are even slightly lower than that of the control cells. Park et alCitation66 demonstrated that nitrogen doped graphitic hollow spheres had little effect on cell proliferation/viability and LDH release compared to graphitic hollow spheres, suggesting that nitrogen-doping could reduce cytotoxicity of graphitic hollow spheres. The data obtained revealed that PMEFs cells had significant biocompatibility with TEA-rGO treatment. These results clearly suggest that TEA-rGO exhibited no toxicity, implying its great potential for biological and pharmaceutical applications.
Figure 9 Effect of GO and TEA-rGO on LDH activity in PMEFs cells.
Notes: LDH activity was measured by changes in optical densities due to NAD+ reduction, monitored at 490 nm, as described in Materials and methods, using the Cytotoxicity Detection Lactate Dehydrogenase kit. The results represent the means of three separate experiments, and error bars represent the standard error of the mean. GO treated groups showed statistically significant differences from the control group by the Student’s t-test (P < 0.05).
Abbreviations: GO, graphene oxide; TEA-rGO, triethylamine-reduced graphene oxide; LDH, lactate dehydrogenase; PMEFs, primary mouse embryonic fibroblast cells; NAD+, nicotinamide adenine dinucleotide.
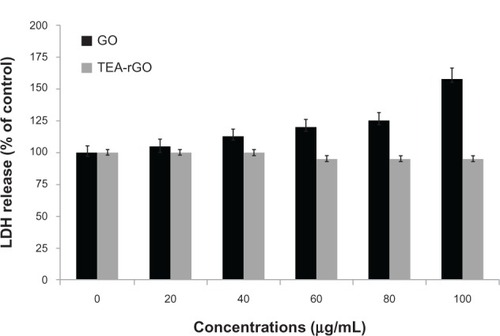
The effect of lower concentrations of TEA-rGO on proliferation of cells
Biocompatibility is an important factor for the development of any new nanomaterials in biological and biomedical applications. Surprisingly, cell viability assays suggest that TEA-rGO had a stimulatory effect on PMEFs at a concentration of 20 μg/mL. Furthermore, we were interested in evaluating whether or not lower concentrations of GO and TEA-rGO promote proliferation; we therefore selected various concentrations between 5 and 20 μg/mL for both GO and TEA-rGO, and the cells were seeded 1 × 104 at the same density in all plates. After 24 hours of incubation, we measured the number of cells after culturing in the medium containing various concentrations of GO and TEA-rGO (from 5–20 μg/mL) on cells treated with GO and TEA-rGO. First, the effect of GO and TEA-rGO on PMEFs’ proliferation was assessed by cell population growth assay. Quantitative data suggest that TEA-rGO significantly increased proliferation of PMEFs when compared to negative controls and GO, in a concentration-dependent manner in TEA-rGO treated groups (). Furthermore, microscopic images show the evidence for proliferation in two different concentrations (). When compared to control and GO, the TEA-rGO treated plates show stronger effect, and the cells were crowded and forming networks due to overgrowth of cells, which indicates that the functionalization of the rGO could contribute to the proliferation of the cells. The biopolymer functionalized rGO exhibits an ultralow hemolysis ratio and good cytocompatibility in human umbilical vein endothelial cells (HUVECs), even at a high concentration of 100 μg/mL.Citation67 Liu et alCitation39 developed a glucose oxidase-immobilized GO electrode which shows reproducibility and good storage stability, as well as significant biocompatibility with retinal pigment epithelium (RPE) cells. Li et alCitation68 studied the cytotoxicity of the graphene oxide (GO)-hydroxyapatite (HA) and chitosan (CS)-GO-HA nanocomposites in the murine fibroblast L-929 cell line and the human osteoblast-like MG-63 cell line, and suggested that the novel synthesized GO–HA and CS-GO–HA nanocomposites induced no obvious inhibitory effects on the in vitro cell proliferation rate of L-929 and MG-63 cells; they exhibited excellent in vitro cytocompatibility within a concentration of 100μg/mL, especially for CS-GO–HA.
Figure 10 The effect of lower concentration of TEA-rGO on proliferation of PMEFs cells.
Notes: (A) PMEFs cells were treated with various concentrations of GO and TEA-rGO for 24 hours, cells were harvested with 0.05% trypsin and 0.02% EDTA in PBS, and counted. Data are expressed as number of cells. The results represent the means of three separate experiments and error bars represent the standard error of the mean. GO and TEA-rGO treated groups showed statistically significant differences from the control group by the Student’s t-test (P < 0.05). (B) Representative microscopic images of GO and TEA-rGO treated cells (0.5 and 25 μg/mL).
Abbreviations: GO, graphene oxide; TEA-rGO, triethylamine-reduced graphene oxide; EDTA, ethylenediaminetetraacetic acid; PBS, phosphate buffered saline; PMEFs, primary mouse embryonic fibroblast cells.
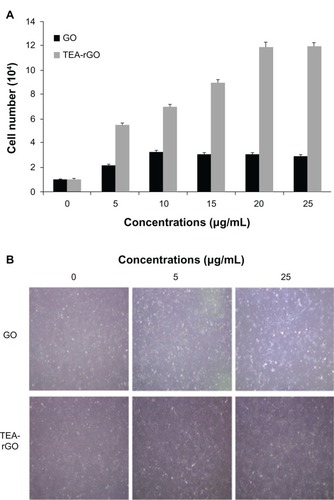
TEA-rGO enhances the attachment of PMEFs cells
Graphene has been employed as a substrate to be interfaced with various biomolecules and cells. Biological modification in turn benefits graphene by improving its biocompatibility, solubility, and selectivity.Citation34 The cell viability results suggest that TEA-rGO shows significantly better biocompatibility than GO. Therefore, because we were further interested in evaluating whether TEA-rGO-coated substrates can promote the attachment of cells when compared to uncoated plates, we selected a particular dose (5 μg/mL) which is the lowest concentration for GO and TEA-rGO respectively. The typical positive control (gelatin) was also used as a benchmark for the attachment assay. The cells were seeded 1 × 104 at the same density in all the plates. The cells were allowed to attach and develop on the substrates. The cells were treated with the same concentration of GO and TEA-rGO for 24 hours. Quantitative analysis was carried out to determine the number of attached cells on each substrate for 24 hours of incubation (). The microscopic images indicate that the cell densities on the dish without coating and the GO-coated dish were lower than that on the TEA-GO coated surface, which indicates more efficient cell adherence onto TEA-rGO than the control and GO surfaces (). Although GO promoted the attachment of cells, the effect was significant (P < 0.05) in TEA-rGO coated plates, which could be the reason for functionalization of GO by TEA. Chen et alCitation65 reported that graphene and GO can support mouse iPSCs culture and allow for spontaneous differentiation. Graphene and CNTs supported behavior of NIH-3T3 fibroblast cells in response to proliferation, cell shape, focal adhesion, and cell adhesion-related gene expression levels; gene transfection efficiency of cells grown on the substrates was improved up to 250% compared to that of cells grown on a cover glass.Citation69 The results suggest that TEA-rGO could provide a wider surface for cell adhesion. These results clearly indicated that TEA-rGO did not induce any cytotoxic effects on the cells, but that it promoted cell attachment. Ruiz et alCitation65 demonstrated that mammalian colorectal adenocarcinoma HT-29 cells attached more efficiently in 10 μg/mL of GO than noncoated dishes. Pretreating rats with amine-modified SWNTs can protect neurons and enhance the recovery of behavioral functions in rats with induced stroke.Citation70 Previous findings suggested that positively charged modifications such as amine (2 NHCitation2) groups can stimulate neurite outgrowth.Citation71 Plasma treatment may have increased surface defects on the nanotubes, resulting in greater surface energy and polarity, which favor the growth of neural tissue.Citation72
Figure 11 Effect of GO and TEA-rGO on attachment of PMEFs cells.
Notes: (A) The cells were grown in coated dishes for 24 hours. The numbers of cells attached to coated dishes were counted after 24 hours. Triplicate cell counts were performed in each experiment, and the experiment was repeated three times. The results represent the means of three separate experiments and error bars represent the standard error of the mean. GO and TEA-rGO (5 μg/mL) treated groups showed statistically significant differences from the control group by the Student’s t-test (P < 0.05). Data are expressed as percentage of attached cells compared to initial cells. (B) Representative microscopic images of GO and TEA-rGO treated cells.
Abbreviations: GO, graphene oxide; TEA-rGO, triethylamine-reduced graphene oxide; PMEFs, primary mouse embryonic fibroblast cells; CON, control.
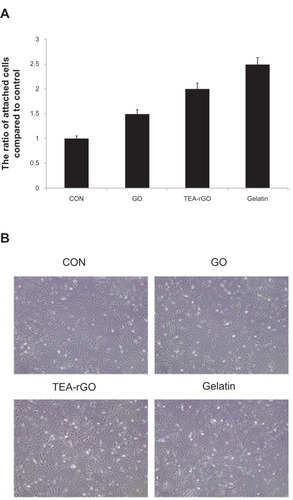
Our recent studies demonstrated that graphene nanomaterials play an extremely important role in toxicity. Bacterially-reduced graphene oxide (B-rGO) induces cytotoxic effects on human breast cancer cell lines, and these effects are concentration-dependent.Citation73 In contrast, we also demonstrated that the biocompatibility of microbially rGO (M-rGO) in PMEFs cells.Citation28 Altogether, our findings and those of other research groups suggest that the biocompatibility of graphene is dependent on the physicochemical properties of graphene-based materials, including density of functional groups, size, conductivity, the type of reducing agents used for the deoxygenation of GO, the degree of functionalization, and the cell types.
Conclusion
We presented a simple, nontoxic and room temperature mediated synthesis of water-soluble graphene. We used TEA as a reducing and stabilizing agent for effective preparation of well-stable and highly soluble graphene. The prepared graphene was characterized using various analytical techniques and was evaluated for cytotoxicity. Cell viability assays exhibited GO-caused dose-dependent toxicity, whereas TEA-rGO displayed significant biocompatibility with PMEFs cells. The lower concentration of GO and TEA-rGO enhances proliferation and cell attachment. Our present findings provide a cost effective and environmentally-friendly approach to the production of stable aqueous dispersions of rGO; this approach is amenable to bulk production. To the best of our knowledge, this is the first such example for TEA reduced GO. Graphene could be a significant biocompatible scaffold substrate for stem cells due to its cost effectiveness, easy availability, solubility, and the high quality of continuous graphene sheets, producible on a large scale.
Acknowledgments
This paper was supported by the SMART-Research Professor Program of Konkuk University. Dr Sangiliyandi Gurunathan was supported by a Konkuk University SMART-Full time Professorship. This work was supported by the Woo Jang-Choon project (PJ007849) and the next generation of Biogreen 21 (PJ009107) from the Rural Development Administration (RDA), Republic of Korea.
Disclosure
The authors report no conflicts of interest in this work.
References
- NovoselovKSGeimAKMorozovSVElectric field effect in atomically thin carbon filmsScience2004306569666666915499015
- GeimAKNovoselovKSThe rise of grapheneNat Mater20076318319117330084
- RaoCNSoodAKSubrahmanyamKSGovindarajAGraphene: the new two-dimensional nanomaterialAngew Chem Int Ed Engl200948427752777719784976
- DikinDAStankovichSZimneyEJPreparation and characterization of graphene oxide paperNature2007448715245746017653188
- DreyerDRParkSBielawskiCRuoffRSThe chemistry of graphene oxideChem Soc Rev200939122824020023850
- BergerCSongZLiXElectronic confinement and coherence in patterned epitaxial grapheneScience200631257771191119616614173
- KimKSZhaoYJangHLarge-scale pattern growth of graphene films for stretchable transparent electrodesNature2009457723070671019145232
- LiDMüllerMBGiljeSKanerRBWallaceGGProcessable aqueous dispersions of graphene nanosheetsNat Nanotechnol20083210110518654470
- WangZZhouXZhangJBoeyFZhangHDirect Electrochemical Reduction of Single-Layer Graphene Oxide and Subsequent Functionalization with Glucose OxidaseJ Phys Chem C2009113321407114075
- HummersWSOffemanREPreparation of Graphitic OxideJ Am Chem Soc19588061339
- XuYBaiHLuGLiCShiGFlexible graphene films via the filtration of water-soluble noncovalent functionalized graphene sheetsJ Am Chem Soc2008130185856585718399634
- PhamTAKimJSKimJSJeongYTOne-step reduction of graphene oxide with L-glutathioneColloids Surf A20113841–3543548
- StankovichSPinerRDChenXWuNNguyenSTRuoffRSStable aqueous dispersions of graphitic nanoplatelets via the reduction of exfoliated graphite oxide in the presence of poly(sodium 4-styrenesulfonate)J Mater Chem200616155158
- StankovichSDikinDADommettGHGraphene-based composite materialsNature2006442710028228616855586
- TungVCAllenMJYangYKanerRBHigh-throughput solution processing of large-scale grapheneNature Nanotech2009412529
- HigginbothamALKosynkinDVSinitskiiASunZTourJMLower-defect graphene oxide nanoribbons from multiwalled carbon nanotubesACS Nano2010442059206920201538
- ZhouXLiuZA scalable, solution-phase processing route to graphene oxide and graphene ultra large sheetsChem Commun (Camb)201046152611261320449324
- SalasECSunZLüttgeATourJMReduction of graphene oxide via bacterial respirationACS Nano2010484852485320731460
- MinKHanTHKimJA facile route to fabricate stable reduced graphene oxide dispersions in various media and their transparent conductive thin filmsJ Colloid Interface Sci20123831364222795947
- FanXPengWLiYDeoxygenation of exfoliated graphite oxide under alkaline conditions: a green route to graphene preparationAdv Mater2008202344904493
- TangLALeeWCShiHHighly wrinkled cross-linked graphene oxide membranes for biological and charge-storage applicationsSmall20128342343122162356
- ZhangJYangHShenGChengPZhangJGuoSReduction of graphene oxide via L-ascorbic acidChem Commun (Camb)20104671112111420126730
- ZhuCGuoSFangYDongSReducing sugar: new functional molecules for the green synthesis of graphene nanosheetsACS Nano2010442429243720359169
- KhanraPKuilaTKimNHBaeSHYuDSLeeJHSimultaneous bio-functionalization and reduction of graphene oxide by baker’s yeastChem Eng J2012183526533
- LiuJHAnilkumarPCaoLCytotoxicity evaluations of fluorescent carbon nanoparticlesNano Life201011&2153161
- KimJEHanTHLeeSHGraphene Oxide Liquid CrystalsAngew Chem Int Ed2011501330433047
- GurunathanSHanJWDayemAAEppakayalaVKimJHOxidative stress-mediated antibacterial activity of graphene oxide and reduced graphene oxide in Pseudomonas aeruginosaInt J Nanomedicine201275901591423226696
- GurunathanSHanJWEppakayalaVKimJHMicrobial reduction of graphene oxide by Escherichia coli: a green chemistry approachColloids Surf B Biointerfaces201310277277723107955
- GurunathanSHanJWEppakayalaVKimJHAn Environmentally Friendly Approach to the Reduction of Graphene Oxide by Escherichia fergusoniJ Nanosci Nanotechnol20131332091209823755651
- HuWPengCLuoWGraphene-based antibacterial paperACS Nano2010474317432320593851
- AkhavanOGhaderiEToxicity of graphene and graphene oxide nano-walls against bacteriaACS Nano20104105731573620925398
- AkhavanOGhaderiERahimiKAdverse effects of graphene incorporated in TiO2 photocatalyst on minuscule animals under solar light irradiationJ Mater Chem2012222326023266
- ZhangYAliSFDervishiECytotoxicity effects of graphene and single-wall carbon nanotubes in neural phaeochromocytoma-derived PC12 cellsACS Nano2010463181318620481456
- WangKRuanJSongHBiocompatibility of Graphene OxideNanoscale Res Lett2011618
- ChangYYangSTLiuJHIn vitro toxicity evaluation of graphene oxide on A549 cellsToxicol Lett2011200320121021130147
- LiaoKHLinYSMacoskoCWHaynesCLCytotoxicity of graphene oxide and graphene in human erythrocytes and skin fibroblastsACS Appl Mater Interfaces2011372607261521650218
- AkhavanOGhaderiEEmamyHAkhavanFGenotoxicity of graphene nanoribbons in human mesenchymal stem cellsCarbon201354419431
- YanXChenJYangJXueQMielePFabrication of free-standing, electrochemically active, and biocompatible graphene oxide-polyaniline and graphene-polyaniline hybrid papersACS Appl Mater Interfaces2010292521252920735069
- LiuYYuDZengCMiaoZDaiLBiocompatible Graphene Oxide-Based Glucose BiosensorsLangmuir20102696158616020349968
- LiNXiaTNelAEThe role of oxidative stress in ambient particulate matter-induced lung diseases and its implications in the toxicity of engineered nanoparticlesFree Radic Biol Med20084491689169918313407
- ParkSRuoffRSChemical methods for the production of graphenesNat Nanotechnol20104421722419350030
- AgarwalSZhouXYeFInterfacing live cells with nanocarbon substratesLangmuir20102642244224720099791
- RobinsonJTTabakmanSMLiangYYWangHLCasalongueHSVinhDUltrasmall reduced graphene oxide with high near-infrared absorbance for photothermal therapyJ Am Chem Soc2011133176825683121476500
- YangKZhangSAZhangGXSunXMLeeSTLiuZAGraphene in mice: ultrahigh in vivo tumor uptake and efficient photothermal therapyNano Lett20101093318332320684528
- ChenHMüllerMBGilmoreKJWallaceGGLiDMechanically Strong, Electrically Conductive, and Biocompatible Graphene PaperAdvanced Materials2008201835573561
- ParkSMohantyNSukJWBiocompatible, robust free-standing paper composed of a TWEEN/graphene compositeAdv Mater2010221517361740520496406
- SasidharanAPanchakarlaLSSadanandanARHemocompatibility and macrophage response of pristine and functionalized grapheneSmall2012881251126322334378
- SinghKSSinghMKKulkarniPPSonkarKVGrácioJJDashDAmine-modified graphene: thrombo-protective safer alternative to graphene oxide for biomedical applicationsACS Nano2012632731274022376049
- EsfandiarAAkhavanOIrajizadAMelatonin as a powerful bio-antioxidant for reduction of graphene oxideJ Mater Chem2011211090710914
- JiangSYShuRSongZCXieYFEffects of enamel matrix proteins on proliferation, differentiation and attachment of human alveolar osteoblastsCell Prolif201144437237921702859
- McAllisterMJLiJLAdamsonDHSingle sheet functionalized graphene by oxidation and thermal expansion of graphiteChem Mater2007191843964404
- KaniyoorABabyTTRamaprabhuSGraphene synthesis via hydrogen induced low temperature exfoliation of graphite oxideJ Mater Chem20102084678469
- LiDMüllerMBGiljeSKanerRBWallaceGGProcessable aqueous dispersions of graphene nanosheetsNat Nanotechnol20083210110518654470
- NethrvathiCRajamathiMChemically modified graphene sheets produced by the solvothermal reduction of colloidal dispersions of graphite oxideCarbon2008461419941998
- WangYZhangPLiuCFZhanLLiYFHuangCZGreen and easy synthesis of biocompatible graphene for use as an anticoagulantRSC Adv2012223222328
- LiuSZengTHHofmannMAntibacterial activity of graphite, graphite oxide, graphene oxide, and reduced graphene oxide: membrane and oxidative stressACS Nano2011596971698021851105
- LiSJDengDHShiQLiuSRElectrochemical synthesis of a graphene sheet and gold nanoparticle-based nanocomposite, and its application to amperometric sensing of dopamineMicrochim Acta2012177325331
- GaoJLiuFLiuYMaNWangZZhangXEnvironment-friendly method to produce graphene that employs vitamin C and amino acidChem Mater201022722132218
- LiBZhangXLiXPhoto-assisted preparation and patterning of large-area reduced graphene oxide-TiO2 conductive thin filmChem Commun (Camb)201046203499350120376387
- GuoSWenDZhaiYDongSWangEPlatinum nanoparticle ensemble-on-graphene hybrid nanosheet: one-pot, rapid synthesis, and used as new electrode material for electrochemical sensingACS Nano2010473959396820568706
- SchrandAMDaiLSchlagerJJHussainSMOsawaEDifferential biocompatibility of carbon nanotubes and nanodiamondsDiamond and Related Materials2007161221182123
- Mejías CarpioIESantosCMWeiXRodriguesDFToxicity of a polymer-graphene oxide composite against bacterial planktonic cells, biofilms, and mammalian cellsNanoscale20124154746475622751735
- WojtoniszakMChenXKalenczukRJSynthesis, dispersion, and cytocompatibility of graphene oxide and reduced graphene oxideColloids Surf B Biointerfaces201289798521962852
- ChenGYPangDWHwangSMTuanHYHuYCA graphene-based platform for induced pluripotent stem cells culture and differentiationBiomaterials201233241842722014460
- RuizONFernandoKAWangBGraphene oxide: a nonspecific enhancer of cellular growthACS Nano20115108100810721932790
- ParkJGrayferEDJungYPhotoluminescent nanographitic/nitrogen-doped graphitic hollow shells as a potential candidate for biological applicationsJ Mater Chem B2013112291234
- ChengCNieSLiSBiopolymer functionalized reduced graphene oxide with enhanced biocompatibility via mussel inspired coatings/anchorsJ Mater Chem B20131265275
- LiMWangYLiuQIn situ synthesis and biocompatibility of nano hydroxyapatite on pristine and chitosan functionalized graphene oxideJ Mater Chem B20131475484
- RyooSRKimYKKimMHMinDHBehaviors of NIH-3T3 fibroblasts on graphene/carbon nanotubes: proliferation, focal adhesion, and gene transfection studiesACS Nano20104116587689820979372
- LeeJHParkJYoonOJAmine-modified single-walled carbon nanotubes protect neurons from injury in a rat stroke modelNat Nanotechnol20116212112521278749
- MatsumotoKSatoCNakaYWhitbyRShimizuNStimulation of neuronal neurite outgrowth using functionalized carbon nanotubesNanotechnology2010211111510120173239
- WangWFishmanHADaiHHarrisJSNeural stimulation with a carbon nanotube micro electrode arrayNano Lett2006692043204816968023
- GurunathanSHanJWEppakayalaVKimJHGreen synthesis of graphene and its cytotoxiceffects in human breast cancer cellsInt J Nanomedicine201381015102723687445