Abstract
Idiopathic pulmonary fibrosis (IPF) poses a formidable clinical challenge, characterized by the thickening of alveolar septa and the onset of pulmonary fibrosis. The pronounced activation of oxidative stress emerges as a pivotal hallmark of inflammation. Traditional application of exogenous antioxidants proves inadequate in addressing oxidative stress, necessitating exploration into strategies to augment their antioxidant efficacy. Exosomes, nano-sized extracellular vesicles harboring a diverse array of bioactive factors, present as promising carriers with the potential to meet this challenge. Recent attention has been directed towards the clinical applications of exosomes in IPF, fueling the impetus for this comprehensive review. We have compiled fresh insights into the role of exosomes in modulating oxidative stress in IPF and delved into their potential as carriers for regulating endogenous reactive oxygen species generation. This review endeavors to bridge the divide between exosome research and IPF, traversing from bedside to bench. Through the synthesis of recent findings, we propose exosomes as a novel and promising strategy for improving the outcomes of IPF therapy.
Introduction
Idiopathic pulmonary fibrosis (IPF) presents a complex and debilitating pulmonary pathology characterized by the unexplained scarring of lung tissue.Citation1 This chronic and progressive interstitial lung disease not only poses a significant threat to affected individuals but also imposes a substantial burden on global healthcare systems.Citation2 The insidious nature of IPF lies in its capacity to progressively diminish lung function, resulting in respiratory distress and compromised quality of life, particularly among individuals in their 50s and 60s.Citation3 As the aging global population and persistent environmental risk factors contribute to an increasing prevalence of respiratory-related health concerns, urgent research efforts are warranted to develop innovative therapeutic approaches to mitigate the impact of this epidemic.Citation4
The pathogenesis of IPF unfolds through an intricate cascade of events disrupting the delicate equilibrium of the lung’s cellular microenvironment.Citation5 Commencing with recurrent injuries to the alveolar epithelium, the response triggers abnormal wound healing, initiating the involvement of oxidative stress as a pivotal factor in the disease’s progression.Citation6 Characterized by an imbalance between the production of reactive oxygen species (ROS) and the body’s counteracting mechanisms, oxidative stress plays a crucial role in instigating and perpetuating fibrotic processes within lung tissue.Citation7 Various sources contribute to ROS production, including environmental factors, inflammatory cells, and dysfunctional mitochondria, setting off a cascade that activates fibroblasts, the key contributors to tissue repair.Citation8 These activated fibroblasts perpetuate the fibrotic cascade, generating excessive collagen and other matrix components, leading to scar tissue formation and the gradual distortion of lung architecture.Citation9
Oxidative stress induces immune dysregulation, drawing immune cells into the inflamed and fibrotic lung milieu, while inflammation contributes to oxidative stress, establishing a self-sustaining loop that perpetuates lung tissue damage.Citation10 Oxidative stress’s association with cellular senescence accelerates aging processes, further impairing cellular repair mechanisms, intensifying the cycle of injury and fibrosis.Citation11 Mitochondrial dysfunction, another consequence of oxidative stress, amplifies ROS production and disrupts cellular energy balance, impacting normal physiological functions.Citation12
In the context of IPF, the conventional application of exogenous antioxidants proves insufficient in addressing overwhelming oxidative stress.Citation13 This inadequacy prompts exploration into strategies to enhance the anti-oxidative capacity of antioxidants, presenting a potential therapeutic avenue for mitigating oxidative stress in IPF. Research efforts are ongoing to identify compounds that can modulate oxidative stress, either by targeting specific pathways or by promoting the overall antioxidant defense mechanisms of the body.Citation14 Exosomes, nano-sized extracellular vesicles enriched with a diverse array of bioactive factors, emerge as innovative messengers with therapeutic potential.Citation15 Their unique structure facilitates the delivery of a molecular toolkit, including proteins and nucleic acids, to target cells, presenting an opportunity to modulate redox homeostasis and counteract ROS.Citation16,Citation17 As research progresses, exosomes emerge as a novel and transformative strategy, offering a promising approach to combat the intricate molecular mechanisms underlying this challenging pulmonary disorder.Citation18
Therefore, in this review, we aim to elucidate the considerable potential of exosomes as an effective antioxidant for improving the therapeutic outcome of IPF. By thoroughly investigating the intricate biogenesis and multifaceted functional capacities inherent in exosomes, we aim to offer a comprehensive understanding of the latest state-of-the-art advances, the associated challenges, and the potential frontiers within advanced strategies for addressing IPF.
Exosomes: Biology and Therapeutic Potential
Origin and Secretion of Exosomes
Exosomes, minute extracellular vesicles, play a pivotal role in cellular communication, serving as dynamic messengers that convey bioactive molecules between cells.Citation19 The biogenesis of exosomes is intricately linked to the endosomal pathway, a cellular process that begins with endocytosis within early endosomes.Citation20 During this phase, the cell engulfs membrane proteins and extracellular materials, setting the foundation for the formation of intraluminal vesicles (ILVs).Citation21 These ILVs encapsulate a selectively packaged cargo, comprising proteins, lipids, and nucleic acids originating from the cytoplasm of the cell.Citation21 The maturation of early endosomes into multivesicular bodies (MVBs) marks a critical juncture in the exosome biogenesis pathway.Citation22 MVBs can follow two distinct fates: fusion with lysosomes for degradation or movement towards the cells’ plasma membrane for exosome secretion.Citation22 The latter results in the release of ILVs into the extracellular space through a process known as exocytosis. This intricate mechanism ensures that exosomes, upon release, carry a diverse and selectively packaged composition of bioactive molecules, including tetraspanins, heat shock proteins, and various nucleic acids ().Citation23
Figure 1 Exosome biogenesis involves the interaction of these vesicles with target cells. These vesicles originate from the endocytic pathway, arising from the inward budding of the MVB membrane. Reproduced from Xie X, Xiong Y, Panayi AC, et al. Exosomes as a Novel Approach to Reverse Osteoporosis: a Review of the Literature. Front Bioeng Biotechnol. 2020;8:594247. Creative Commons.Citation23

Acting as potent messengers, exosomes can be transported to neighboring or distant cells, where they interact with target cells through surface receptor binding or direct fusion with the cell membrane.Citation24 The cargo within exosomes, comprised of proteins, lipids, and nucleic acids, is then delivered to recipient cells, influencing various cellular processes.Citation24 This functional cargo transfer plays a crucial role in the regulation of physiological functions and contributes to both homeostasis and the response to pathological conditions.Citation25 The diverse composition of exosomes, coupled with their ability to traverse biological barriers and selectively target cells, positions them as valuable mediators in cell-to-cell communication.Citation22 Understanding the intricacies of exosome biology not only sheds light on fundamental cellular processes but also opens avenues for innovative diagnostic and therapeutic applications.
Biological Functions of Exosomes
Exosomes are instrumental in orchestrating a broad spectrum of biological functions that profoundly impact intercellular communication and cellular homeostasis.Citation26 One of the paramount roles of exosomes is their ability to facilitate intercellular communication.Citation27 Exosomes act as carriers of a diverse cargo, encompassing proteins, lipids, and nucleic acids, that can be selectively delivered to target cells.Citation28 This cargo transfer is a dynamic process that influences cellular signaling pathways, modulating various cellular processes.Citation28 By binding to surface receptors or undergoing fusion with the cell membrane, exosomes can activate specific cellular responses, contributing to tissue development, immune regulation, and the maintenance of overall cellular homeostasis.Citation29
In the process of immune regulation, exosomes emerge as pivotal players.Citation30 Exosomes derived from antigen-presenting cells participate in immune responses by presenting antigens to immune cells.Citation31,Citation32 This process is integral to the initiation and modulation of immune reactions.Citation31,Citation32 Moreover, exosomes exhibit immunomodulatory effects by influencing the activity of immune cells.Citation33 Depending on the context, exosomes can either enhance or suppress immune responses, a feature with implications for autoimmune diseases, infections, and cancer.Citation34 The intricate interplay between exosomes and the immune system underscores their role in sculpting the immune landscape, and ongoing research is elucidating the mechanisms that govern these immunoregulatory functions.Citation30
Exosomes also contribute significantly to tissue repair and regeneration.Citation35 Stem cell-derived exosomes, for instance, harbor factors that influence the differentiation of target cells, thereby contributing to tissue repair processes.Citation36 Additionally, exosomes play a role in angiogenesis, the formation of new blood vessels, which is crucial for supplying nutrients and oxygen during tissue repair.Citation37 This regenerative potential positions exosomes as promising candidates for therapeutic interventions aimed at enhancing tissue repair and regeneration.Citation38–40 Furthermore, the involvement of exosomes in cancer progression is noteworthy. Cancer-derived exosomes actively participate in remodeling the tumor microenvironment, influencing factors such as inflammation, angiogenesis, and immune responses.Citation41 Additionally, these exosomes contribute to the formation of pre-metastatic niches, creating a supportive environment for the dissemination and metastasis of cancer cells.Citation42,Citation43 Unraveling the complexities of exosome-mediated processes in cancer is imperative for developing targeted therapeutic strategies that could impede disease progression.
In neuronal communication, exosomes play a pivotal role in synaptic plasticity—the ability of synapses to strengthen or weaken over time, critical for learning and memory.Citation44,Citation45 Neuronal-derived exosomes transport bioactive molecules that modulate synaptic function, contributing to the intricate network of neuronal communication.Citation46 Furthermore, exosomes may confer neuroprotection by delivering factors that promote cell survival and reduce inflammation in the central nervous system.Citation47,Citation48 The involvement of exosomes in neuroprotective mechanisms raises intriguing possibilities for therapeutic interventions in neurodegenerative disorders. Beyond their physiological roles, exosomes hold diagnostic potential. The analysis of exosomal cargo, particularly in bodily fluids such as blood and urine, has unveiled disease-specific biomarkers.Citation49,Citation50 This opens avenues for non-invasive diagnostic approaches, providing valuable insights into various diseases, including cancer and neurodegenerative disorders.
Isolation and Engineering of Exosomes for Therapeutic Application
Methods for Exosome Isolation and Purification
Exosome isolation and purification are pivotal steps in harnessing the therapeutic and research potential of these nano-sized extracellular vesicles.Citation51 Various methods have been developed to obtain highly enriched and purified exosome populations from complex biological fluidsCitation52 ().
Engineering Exosomes for Enhanced Therapeutic Efficacy
The engineering of exosomes for enhanced therapeutic efficacy represents a frontier in biomedical research, offering a versatile platform for targeted drug delivery and regenerative medicine.Citation53 One pivotal aspect of exosome engineering involves the strategic loading of therapeutic cargo.Citation54 Genetic modification of parent cells is a powerful strategy, allowing for the overexpression of specific therapeutic molecules within exosomes.Citation55,Citation56 This approach ensures that exosomes carry a predetermined payload, whether it be therapeutic proteins, RNA, or microRNAs.Citation55,Citation56 By harnessing the inherent cargo-loading capacity of exosomes, researchers can tailor these extracellular vesicles for precise therapeutic interventions. Additionally, exosomes can be loaded post-isolation through techniques like electroporation or sonication.Citation57 This versatility allows for the customization of exosome cargo, enabling researchers to address the unique requirements of diverse therapeutic applications.Citation57 In a recent study, Xiong et al constructed engineering exosomes by the encapsulation of didymin into ginseng-derived exosomes to form G-sEVsDM for stable and sustained therapy of diabetic wound.Citation58 The in vitro and in vivo results demonstrated the superior capacity of G-sEVsDM in promotion of neural regeneration and thereby enhance diabetic wound repair ().Citation58 Sun et al designed a clodronate (CLD)-loaded liposome and fibroblast-derived exosome (EL-CLD) hybrid drug delivery system with non-specific phagocytosis inhibition and fibroblast homing properties for the treatment of pulmonary fibrosis.Citation59 That system preferentially accumulated in the fibrotic lung, and significantly increased penetration inside pulmonary fibrotic tissue by targeted delivery due to the specific affinity for fibroblasts of the homologous exosome.Citation59
Table 1 Summary of the Methods for Exosome Isolation and Purification
Figure 2 (A) The augmented neurogenesis effect of engineered exosome-encapsulated hydrogel was examined through immunofluorescence staining. The scale bar is set at 50 µm. (B) The schematic representation of the tissue collection timeline. (C) General images depicting wound healing in various treatment groups. (D) Immunofluorescence images illustrating the expression of CGRP, NGF, and CRH in skin tissues on day 7 post-wounding, accompanied by corresponding statistical results. Scale bar: 100 µm. Reproduced from Xiong Y, Lin Z, Bu P, et al. A Whole-Course-Repair System Based on Neurogenesis-Angiogenesis Crosstalk and Macrophage Reprogramming Promotes Diabetic Wound Healing. Adv Mater. 2023;35(19):e2212300. © 2023 The Authors. Advanced Materials published by Wiley-VCH GmbH.Citation58
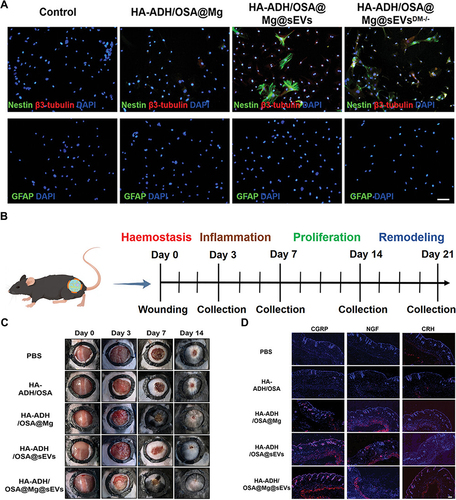
Surface modification is another key facet of exosome engineering, contributing to enhanced specificity and stability.Citation60 The surface of exosomes can be modified with targeting ligands, such as antibodies or peptides, facilitating a more specific interaction with target cells or tissues.Citation60,Citation61 This targeted approach ensures that engineered exosomes reach their intended destination, minimizing off-target effects and enhancing therapeutic precision.Citation60,Citation61 For example, Huang et al employed engineering technology to synergize exosomes with long non-coding RNA (lncRNA) for targeted therapy in osteosarcoma (OS).Citation62 They constructed c(RGDyK)-modified and MEG3-loaded exosomes (cRGD-Exo-MEG3).Citation62 These tailored exosomes exhibit enhanced delivery efficiency to OS cells both in vitro and in vivo. As a result, cRGD-Exo-MEG3 significantly augment the anti-OS effects of MEG3, showing improved tumor-targeting therapy. This research underscores the potential therapeutic impact of engineered exosomes serving as targeted carriers for delivering lncRNA MEG3 in the context of OS treatment ().Citation62
Figure 3 Schematic illustration of preparation of engineered exosome as targeted lncRNA MEG3 delivery vehicles for OS therapy. Reprinted from J Control Release, volume: 343, Huang X, Wu W, Jing D, et al. Engineered exosome as targeted lncRNA MEG3 delivery vehicles for osteosarcoma therapy. 107–11, Copyrihgt 2022, with permission from Elsevier.Citation62
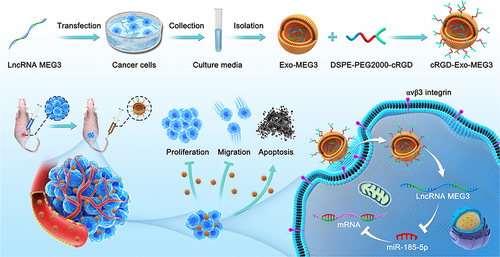
Fusion proteins represent an innovative approach in exosome engineering, involving the expression of fusion proteins that incorporate exosome-targeting peptides along with therapeutic agents.Citation63 This strategy allows for the seamless integration of targeting and therapeutic functionalities, streamlining the engineering process.Citation64 Engineered exosomes carrying these fusion proteins can be guided to specific cell types or tissues, ensuring a more targeted and efficient delivery of therapeutic cargo.Citation65 Additionally, the loading of exosomes with small molecules, such as chemotherapeutic drugs, takes advantage of their natural membrane permeability.Citation55 This intrinsic property allows exosomes to encapsulate hydrophobic compounds, providing protection during transport and facilitating their delivery to target cells.Citation55 Moreover, the loading of exosomes with various forms of RNA, including small interfering RNA (siRNA) or messenger RNA (mRNA), offers a powerful means to modulate gene expression in target cells.Citation66 This RNA cargo can be therapeutic by silencing specific genes or promoting the expression of therapeutic proteins, providing a versatile platform for gene-based therapies.Citation67
An integral aspect of exosome engineering involves manipulating the parent cells that produce exosomes. Cell engineering through genetic modification ensures that these cells not only produce exosomes with enhanced cargo but also that they produce exosomes more efficiently.Citation55,Citation68 This approach guarantees a continuous and sustainable supply of engineered exosomes for therapeutic applications. Additionally, the integration of magnetic nanoparticles into exosomes allows for their magnetic guidance to specific target sites.Citation69 This biophysical modification facilitates enhanced accumulation of exosomes at desired locations, improving therapeutic delivery and efficacy.Citation69 Furthermore, the utilization of hybrid systems comprising exosomes and biocompatible nanoparticles offers synergistic advantages. These hybrid platforms can be designed to enhance cargo loading, stability, and targeting capabilities, combining the strengths of both exosomes and nanoparticles for superior therapeutic outcomes.Citation70 Quality control measures, including the development of robust analytical tools, are crucial for characterizing engineered exosomes. These tools assess factors such as size distribution, cargo composition, and surface modifications, ensuring the reproducibility and safety of engineered exosomes for therapeutic applications.Citation67,Citation71
Exosomes in Mitigating Oxidative Stress
Mechanisms by Which Exosomes Counteract Oxidative Stress
Exosomes, being essential components of intercellular communication, exhibit a multifaceted repertoire of mechanisms by which they counteract oxidative stress-a condition characterized by an imbalance between ROS production and the cellular antioxidant defense systemCitation72,Citation73 (). One key mechanism involves the transfer of antioxidant enzymes, such as Superoxide Dismutase (SOD), through exosomes.Citation74 SOD catalyzes the dismutation of superoxide radicals into hydrogen peroxide and molecular oxygen, playing a pivotal role in neutralizing one of the primary contributors to oxidative stress.Citation75 This enzymatic activity, when transferred via exosomes, enhances the cellular capacity to scavenge harmful free radicals.Citation76
Table 2 Mechanisms of Exosomes in Counteracting Oxidative Stress
Non-coding RNAs (ncRNAs), including microRNAs (miRNAs) and long non-coding RNAs (lncRNAs), constitute another critical facet of exosome-mediated oxidative stress regulation.Citation87 Exosomes encapsulate miRNAs that can modulate gene expression, particularly targeting genes involved in oxidative stress pathways.Citation88 This regulatory function allows for fine-tuning the cellular response to oxidative challenges. Similarly, lncRNAs carried by exosomes play a role in cellular processes, including those linked to oxidative stress. The transport of these ncRNAs provides an additional layer of complexity in the regulatory network that influences redox balance and cellular responses to oxidative stress conditions.Citation89
The cargo within exosomes extends beyond genetic material to include antioxidant molecules such as glutathione (GSH), vitamins, and cofactors essential for the activity of antioxidant enzymes.Citation90,Citation91 GSH, a paramount cellular antioxidant, is transported by exosomes and contributes to the cellular redox status by scavenging free radicals.Citation91 The transfer of antioxidant vitamins and cofactors further enhances the cellular defense against oxidative stress, providing a comprehensive antioxidant toolkit. Additionally, exosomes carry heat shock proteins (HSPs), including HSP70 and HSP90, which serve dual roles in protein folding and as antioxidants.Citation92,Citation93 These proteins contribute to cellular protection by preventing protein misfolding and by acting as molecular chaperones under conditions of oxidative stress.Citation94
Furthermore, exosomes play a pivotal role in maintaining mitochondrial quality and function—a crucial aspect of cellular resilience against oxidative stress.Citation95 Exosomes have been shown to contain mitochondrial components, including mitochondrial DNA (mtDNA).Citation96 This mitochondrial cargo contributes to the preservation of mitochondrial integrity and prevents the excessive generation of ROS within these cellular powerhouses.Citation97 In tandem, exosomes influence cellular signaling pathways associated with oxidative stress responses. The Nuclear Factor Erythroid 2-Related Factor 2 (Nrf2) pathway, a master regulator of antioxidant gene expression, can be activated by exosomal cargo in recipient cells.Citation98 This activation enhances the cellular antioxidant defense mechanisms, further emphasizing the diverse and integrated nature of exosome-mediated responses to oxidative stress.Citation99,Citation100
The DNA repair machinery is another critical arm of exosome-mediated defense against oxidative stress. Exosomes may carry proteins involved in DNA repair mechanisms, aiding in the restoration of oxidative stress-induced DNA damage. By supporting the repair of genomic instability caused by ROS, these exosome-borne proteins contribute to the overall cellular resilience against oxidative stress-induced cellular damage.Citation72,Citation101 Moreover, metal ion chelation is facilitated by exosomes through the transport of metallothioneins, proteins capable of binding metal ions. This mechanism helps prevent the generation of ROS through Fenton-type reactions, presenting an additional layer of protection against oxidative stress-induced cellular damage.
Application of Exosomes in Oxidative Stress Management in IPF
The application of exosomes in the management of oxidative stress in IPF represents a promising frontier in the pursuit of effective therapeutic interventions. Exosomes serve as natural carriers of a diverse cargo, making them ideal candidates for targeted and multifaceted approaches to counteract oxidative stress in the lungs. One primary mechanism involves the delivery of antioxidant enzymes, such as SOD, by exosomes. SOD catalyzes the breakdown of superoxide radicals, a primary contributor to oxidative stress in IPF.Citation102 By enhancing the delivery of antioxidant enzymes to the sites of oxidative stress, exosomes offer a localized and efficient strategy to neutralize harmful free radicals and mitigate oxidative damage.Citation103
Another facet of exosome-mediated oxidative stress management in IPF is the transfer of regulatory RNA molecules, including miRNAs and lncRNAs.Citation104 Shao et al found that miR-454-3p may serve as an exosomal biomarker and may be developed into a novel treatment for glioma.Citation105 By Valadi et al’s opinion, exosomes can deliver nucleic acids to cells at a distance and they are non-immunogenic, making them ideal candidates as vectors for gene therapy.Citation106 These molecules play a pivotal role in modulating gene expression and signaling pathways associated with oxidative stress responses. In the intricate pathogenesis of IPF, where aberrant oxidative stress is a driving force, the transfer of these regulatory RNA molecules by exosomes provides a means to modulate the cellular response to oxidative challenges.Citation107 The regulatory function of exosome-delivered miRNAs and lncRNAs adds a layer of complexity to the network that influences redox balance and cellular responses to oxidative stress conditions.Citation108 This approach is promising in developing therapeutic interventions to the specific molecular intricacies of oxidative stress in IPF.Citation109 Beyond direct antioxidant mechanisms, exosomes contribute to the reduction of inflammation and fibrosis, both of which are intertwined with oxidative stress in IPF. Certain exosomes possess anti-inflammatory and anti-fibrotic properties, delivering bioactive molecules that modulate immune responses and inhibit fibrotic processes in the lungs.Citation110–112 By targeting the underlying mechanisms that drive inflammation and fibrosis, exosomes indirectly alleviate oxidative stress. For instance, the Cheng et al group introduced a series of studies employing the lung spheroid cell-secretome (LSC-Sec) and exosomes (LSC-Exo) delivered through inhalation to address various models of lung injury and fibrosis.Citation113 They demonstrated that treatments with LSC-Sec and LSC-Exo can alleviate and resolve fibrosis induced by bleomycin and silica.Citation113 This therapeutic effect is achieved by restoring normal alveolar structure and reducing both collagen accumulation and myofibroblast proliferation. Notably, LSC-Sec and LSC-Exo surpass their mesenchymal stem cell-derived counterparts in certain efficacy measures.Citation113 The inhalation-based administration of secretome and exosomes presents a promising therapeutic approach for lung regeneration in experimental models of pulmonary fibrosis ().Citation113 This research sheds light on a potential avenue for developing treatments that may enhance lung function and alleviate the impact of pulmonary fibrosis.
Figure 4 (A) Schematic representation of the experimental design for the exosome study in SD rats, with a total of 12 biological independent animals per group. (B) Representative H&E staining images, with the upper panel showing a scale bar of 100 μm and the lower panel showing a scale bar of 50 μm. (C) Representative Gomori’s trichrome staining images, illustrating muscle fibers (red), collagen (blue), nuclei (black-purple), and erythrocytes (red). The scale bar is set at 100 μm for the upper panel and 50 μm for the lower panel. (D) Representative picrosirius red staining images depicting collagen types I and III in red. The scale bar is set at 50 μm. (E) Quantification of fibrosis using the Ashcroft score. Each data point represents information from one animal, with a total of 12 biological independent animals. The Ashcroft score was determined by averaging the scores provided by one blinded and one non-blinded scorer. (F) Quantification of pulmonary hydroxyproline levels. Each data point represents information from one animal, with a total of 4 biological independent animals. Statistical significance is denoted by P < 0.05. Reproduced from Dinh PC, Paudel D, Brochu H, et al. Inhalation of lung spheroid cell secretome and exosomes promotes lung repair in pulmonary fibrosis. Nat Commun. 2020;11(1):1064. Creative Commons.Citation113
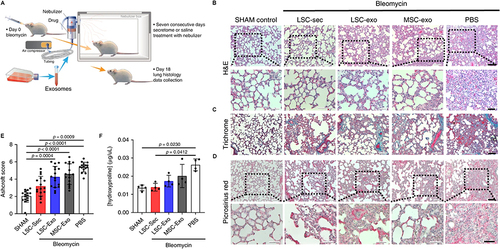
Furthermore, the maintenance of mitochondrial quality is crucial in conditions associated with oxidative stress, and exosomes play a role in this aspect as well.Citation114 Mitochondrial components, including mtDNA, transported by exosomes contribute to preserving mitochondrial integrity.Citation115,Citation116 This preservation helps regulate the production of ROS within mitochondria, addressing a significant source of oxidative stress in IPF. Moreover, the potential for exosomes to stimulate endogenous antioxidant defense mechanisms in recipient cells adds a layer of adaptability to their therapeutic impact. The cargo within exosomes, encompassing antioxidant molecules and signaling factors, may activate cellular pathways involved in antioxidant responses.Citation28 This activation not only addresses existing oxidative stress but also promotes sustained cellular resilience against future oxidative challenges.
Additionally, the prospect of engineered exosomes for targeted drug delivery is a frontier with transformative potential.Citation57,Citation61 By designing exosomes to carry specific antioxidant compounds or drugs targeting oxidative stress pathways, researchers can ensure that therapeutic agents reach the affected lung tissue efficiently, minimizing off-target effects and maximizing the efficacy of oxidative stress management in IPF. For example, Sun et al developed a hybrid drug delivery system, combining clodronate (CLD)-loaded liposomes with fibroblast-derived exosomes (EL-CLD).Citation59 This innovative system possesses properties that inhibit non-specific phagocytosis and exhibit homing capabilities toward fibroblasts, offering a targeted approach for pulmonary fibrosis treatment.Citation59 Importantly, the hybrid system shows a preferential accumulation in fibrotic lungs, enhancing penetration into pulmonary fibrotic tissue due to the specific affinity of homologous exosomes for fibroblasts. The therapeutic agent, Nintedanib (NIN), known for its anti-fibrotic properties, loaded into the EL-CLD system, demonstrates remarkable improvements in curative effects. This enhanced efficacy is attributed to increased accumulation and delivery of NIN in pulmonary fibrotic tissue, coupled with a reduced macrophage-induced inflammatory response ().Citation59
Figure 5 (A) Transmission electron microscopy (TEM) image capturing the morphology of the exosome. (B) Determination of the size and size distribution of L-929 cell-derived exosomes using dynamic light scattering (DLS). (C) Western blot analysis depicting the protein levels of CD9, HSP70, calnexin, and β-actin in the exosomes secreted by L-929 cells. (D) Schematic representation of the procedure employed to produce the EL-CLD hybrid, involving the hybridization of exosomes with L-CLD using membrane extrusion. (E) TEM image illustrating the morphology of the EL-CLD hybrid, showing the successful integration of exosomes with clodronate-loaded liposomes through the described procedure. (F) Micro-CT images depicting the condition of the lung following the respective treatments. (G) Lung Index quantifying pulmonary fibrosis in mice post-treatments, with an inset providing visual comparisons between healthy lungs and those affected by fibrosis at the conclusion of the treatments. The study includes a sample size of n=5. *p<0.05, **p<0.01. Bar: 200 nm. Reproduced from Biomaterials, volume 271, Sun L, Fan M, Huang D, et al. Clodronate-loaded liposomal and fibroblast-derived exosomal hybrid system for enhanced drug delivery to pulmonary fibrosis. 120761, Copyright 2021, with permission from Elsevier.Citation59
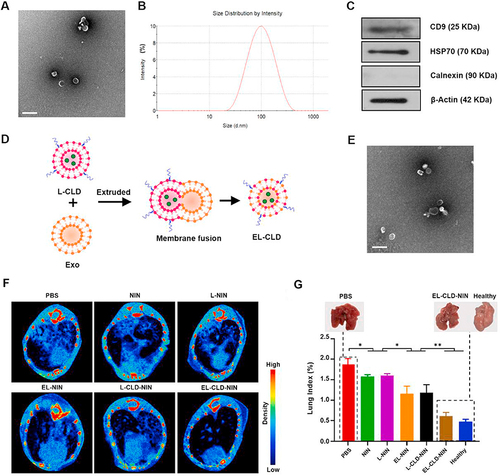
Recent Reports on Studies of EVs/Exosomes Role in IPF
Recent studies have increasingly shed light on the role of EVs, particularly exosomes, in the pathogenesis of IPF. These tiny membrane-bound vesicles, released by various cell types including epithelial cells, fibroblasts, endothelial cells, and immune cells, carry a cargo of proteins, lipids, and nucleic acids that can influence cellular communication and modulate the microenvironment within the lungs.Citation117
In IPF, epithelial cells play a central role in the disease process, undergoing aberrant activation and apoptosis, leading to the formation of fibroblastic foci and excessive deposition of extracellular matrix. Recent research suggests that exosomes released by injured or activated epithelial cells may contribute to the fibrotic response by promoting fibroblast activation, myofibroblast differentiation, and collagen production.Citation118,Citation119 These exosomes contain pro-fibrotic mediators such as TGF-β, PDGF, and various microRNAs that can induce fibroblast proliferation and collagen synthesis.Citation118,Citation119
Moreover, fibroblasts themselves are potent producers of exosomes, which can propagate fibrotic signaling cascades in an autocrine and paracrine manner. Studies have demonstrated that fibroblast-derived exosomes carry fibrogenic cargoes, including TGF-β, Wnt proteins, and connective tissue growth factor (CTGF), which can exacerbate the fibrotic phenotype and promote the persistence of myofibroblasts.Citation120
Endothelial cell-derived exosomes have also emerged as key players in IPF pathogenesis, contributing to vascular remodeling, endothelial dysfunction, and the perpetuation of fibrosis. These exosomes may carry angiogenic factors, inflammatory cytokines, and microRNAs that influence endothelial cell behavior and contribute to the dysregulated angiogenesis observed in IPF.Citation121
In addition to their role in disease pathogenesis, EVs, particularly exosomes, hold promise as novel biomarkers for IPF. The cargo of circulating exosomes reflects the pathological processes ongoing in the lungs, offering insights into disease activity and progression. Recent studies have identified specific exosomal microRNAs, proteins, and lipids that show differential expression patterns in IPF patients compared to healthy individuals or those with other lung diseases.Citation122 These findings suggest that exosomal biomarkers could potentially aid in early diagnosis, prognostication, and monitoring of IPF patients, providing valuable clinical tools for disease management.Citation123
Future Directions
In the pursuit of harnessing the therapeutic potential of exosomes, researchers are increasingly focused on precision targeting of oxidative stress pathways implicated in IPF. This targeted approach aims to neutralize ROS and interrupt the oxidative stress cascade integral to IPF progression. Future research should try to elucidate the optimal cargo composition within exosomes, ensuring their payload is not only effective in mitigating oxidative stress but also tailored to the unique molecular characteristics of individual patients. By understanding the intricacies of redox imbalance in IPF, researchers can design exosome-based interventions that act as finely tuned molecular tools, disrupting the fibrotic process at its core.
To augment the therapeutic efficacy of exosome-based interventions, innovative loading strategies have emerged as a focal point of exploration.Citation124 This involves refining techniques such as pre-conditioning donor cells or leveraging nanotechnology for cargo loading. The goal is to enhance the delivery and stability of exosomes, ensuring their payload reaches target cells with precision. In the context of oxidative stress mitigation, researchers are investigating the most effective combination of antioxidants and anti-fibrotic agents within exosomes.Citation125 This synergistic approach may hold the key to a more potent and comprehensive therapeutic impact, disrupting not only the oxidative stress pathways but also intervening at multiple points in the fibrotic cascade. By refining loading strategies, researchers aim to harness the full potential of exosomes as therapeutic vehicles, offering a multifaceted and targeted approach to address the complexities of IPF pathophysiology.
As the field advances, researchers are increasingly recognizing the importance of tailoring exosome characteristics for optimal delivery. This involves understanding the biodistribution, pharmacokinetics, and stability of exosomes to enhance their ability to traverse the pulmonary barrier and reach target cells effectively. Surface modification and size control are emerging as critical parameters in this pursuit, offering avenues to fine-tune exosomes for enhanced therapeutic delivery.Citation126 Simultaneously, the integration of exosome-based therapies with emerging technologies presents an exciting frontier in IPF research. Gene editing tools such as CRISPR/Cas9 can be harnessed within exosomes to modulate oxidative stress-related genes, providing a level of precision and specificity previously unimaginable.Citation127 This integration of exosomes with cutting-edge technologies represents a convergence of biological and engineering principles, opening new dimensions for the development of highly targeted interventions in the realm of pulmonary fibrosis.
Recognizing the heterogeneity of IPF, there is a growing emphasis on personalized medicine approaches in the development of exosome-based therapies.Citation110,Citation128,Citation129 This entails identifying biomarkers that can guide the customization of treatments based on the unique oxidative stress profiles of individual patients. By utilizing patient-derived exosomes for therapy, researchers aim to tailor interventions to the specific molecular characteristics of each IPF patient, ushering in a new era of precision medicine. As we continue to unravel the complexities of exosome biology and oxidative stress mechanisms, the translation of these insights into clinical applications may offer new hope for patients battling this devastating disease.
Conclusion
In conclusion, the future of exosome-based therapies in IPF, particularly in mitigating oxidative stress, holds immense promise. The integration of advanced technologies, refinement of delivery strategies, and a personalized medicine approach collectively position exosome-based therapies at the forefront of innovative and targeted interventions for IPF. The journey from bench to bedside is ongoing, but with each discovery, we inch closer to a new era in IPF therapy—one where the complexities of the disease are met with equally sophisticated and tailored solutions.
Disclosure
The authors report no conflicts of interest in this work.
Acknowledgments
This work is sponsored by Shanghai Pudong New Area Health Commission Research Project (NO.PW2021A-42), the National Science Foundation of China (No.82270064), Clinical Research Youth Project of Shanghai Health Commission (20204Y0383) and Foundation of Shanghai Pulmonary Hospital (FKZR2112).
References
- Kataoka K, Nishiyama O, Ogura T, et al. Long-term effect of pulmonary rehabilitation in idiopathic pulmonary fibrosis: a randomised controlled trial. Thorax. 2023;78(8):784–791. doi:10.1136/thorax-2022-219792
- Sauleda J, Núñez B, Sala E, Soriano JB. Idiopathic Pulmonary Fibrosis: epidemiology, Natural History, Phenotypes. Med Sci. 2018;6(4):548.
- Chitnis A, Vyas PK, Chaudhary P, Ghatavat G. Case-based discussion: lymphocytic interstitial pneumonia a rare presentation in an immunocompetent adult male. Lung India. 2015;32(5):500–504.
- Shen Q, Peng H. Clinical evaluation on idiopathic pulmonary fibrosis. Zhong Nan Da Xue Xue Bao Yi Xue Ban. 2021;46(3):309–315.
- Gadkowski LB, Stout JE. Cavitary pulmonary disease. Clin Microbiol Rev. 2008;21(2):305–333.
- Wilson MS, Wynn TA. Pulmonary fibrosis: pathogenesis, etiology and regulation. Mucosal Immunol. 2009;2(2):103–121.
- Walters DM, Cho HY, Kleeberger SR. Oxidative stress and antioxidants in the pathogenesis of pulmonary fibrosis: a potential role for Nrf2. Antioxid Redox Signal. 2008;10(2):321–332.
- Mittal M, Siddiqui MR, Tran K, Reddy SP, Malik AB. Reactive oxygen species in inflammation and tissue injury. Antioxid Redox Signal. 2014;20(7):1126–1167. doi:10.1089/ars.2012.5149
- Podolanczuk AJ, Noth I, Raghu G. Idiopathic pulmonary fibrosis: prime time for a precision-based approach to treatment with N-acetylcysteine. Eur Respir J. 2021;57(1):98.
- Araneda OF, Tuesta M. Lung oxidative damage by hypoxia. Oxid Med Cell Longev. 2012;2012:856918.
- Iakovou E, Kourti M. A Comprehensive Overview of the Complex Role of Oxidative Stress in Aging, The Contributing Environmental Stressors and Emerging Antioxidant Therapeutic Interventions. Front Aging Neurosci. 2022;14:827900. doi:10.3389/fnagi.2022.827900
- Cheresh P, Kim SJ, Tulasiram S, Kamp DW. Oxidative stress and pulmonary fibrosis. Biochim Biophys Acta. 2013;1832(7):1028–1040.
- Kinnula VL, Fattman CL, Tan RJ, Oury TD. Oxidative stress in pulmonary fibrosis: a possible role for redox modulatory therapy. Am J Respir Crit Care Med. 2005;172(4):417–422.
- Estornut C, Milara J, Bayarri MA, Belhadj N, Cortijo J. Targeting Oxidative Stress as a Therapeutic Approach for Idiopathic Pulmonary Fibrosis. Front Pharmacol. 2021;12:794997. doi:10.3389/fphar.2021.794997
- Kholafazad Kordasht H, Hasanzadeh M. Biomedical analysis of exosomes using biosensing methods: recent progress. Anal Methods. 2020;12(22):2795–2811.
- Wei D, Zhan W, Gao Y, et al. RAB31 marks and controls an ESCRT-independent exosome pathway. Cell Res. 2021;31(2):157–177.
- Mittelbrunn M, Sánchez-Madrid F. Intercellular communication: diverse structures for exchange of genetic information. Nat Rev Mol Cell Biol. 2012;13(5):328–335.
- Luan X, Sansanaphongpricha K, Myers I, Chen H, Yuan H, Sun D. Engineering exosomes as refined biological nanoplatforms for drug delivery. Acta Pharmacol Sin. 2017;38(6):754–763.
- Wang L, Li J, Yang X, et al. The effects of intravenous remifentanil on umbilical artery serum-derived exosomes in parturients undergoing epidural anesthesia: a randomized trail. BMC Pregnancy Childbirth. 2023;23(1):29. doi:10.1186/s12884-023-05360-8
- Jin Y, Ma L, Zhang W, Yang W, Feng Q, Wang H. Extracellular signals regulate the biogenesis of extracellular vesicles. Biol Res. 2022;55(1):35. doi:10.1186/s40659-022-00405-2
- Wandrey M, Jablonska J, Stauber RH, Gül D. Exosomes in Cancer Progression and Therapy Resistance: molecular Insights and Therapeutic Opportunities. Life. 2023;13(10):2033. doi:10.3390/life13102033
- Gurung S, Perocheau D, Touramanidou L, Baruteau J. The exosome journey: from biogenesis to uptake and intracellular signalling. Cell Commun Signal. 2021;19(1):47. doi:10.1186/s12964-021-00730-1
- Xie X, Xiong Y, Panayi AC, et al. Exosomes as a Novel Approach to Reverse Osteoporosis: a Review of the Literature. Front Bioeng Biotechnol. 2020;8:594247. doi:10.3389/fbioe.2020.594247
- Dilsiz N. Hallmarks of exosomes. Future Sci OA. 2022;8(1):FSO764. doi:10.2144/fsoa-2021-0102
- Gurunathan S, Kang MH, Kim JH. A Comprehensive Review on Factors Influences Biogenesis, Functions, Therapeutic and Clinical Implications of Exosomes. Int J Nanomed. 2021;16:1281–1312. doi:10.2147/IJN.S291956
- López de Las Hazas MC, Gil-Zamorano J, Cofán M, et al. One-year dietary supplementation with walnuts modifies exosomal miRNA in elderly subjects. Eur J Nutr. 2021;60(4):1999–2011. doi:10.1007/s00394-020-02390-2
- Kumari M, Anji A. Small but Mighty-Exosomes, Novel Intercellular Messengers in Neurodegeneration. Biology (Basel). 2022;11(3):413. doi:10.3390/biology11030413
- Chen H, Wang L, Zeng X, et al. Exosomes, a New Star for Targeted Delivery. Front Cell Dev Biol. 2021;9:751079. doi:10.3389/fcell.2021.751079
- Yates AG, Pink RC, Erdbrügger U, et al. In sickness and in health: the functional role of extracellular vesicles in physiology and pathology in vivo: part II: pathology: part II: pathology. J Extracell Vesicles. 2022;11(1):e12190. doi:10.1002/jev2.12190
- Gangadaran P, Madhyastha H, Madhyastha R, et al. The emerging role of exosomes in innate immunity, diagnosis and therapy. Front Immunol. 2022;13:1085057. doi:10.3389/fimmu.2022.1085057
- Shenoda BB, Ajit SK. Modulation of Immune Responses by Exosomes Derived from Antigen-Presenting Cells. Clin Med Insights Pathol. 2016;9(Suppl 1):1–8. doi:10.4137/CPath.S39925
- Buzas EI. The roles of extracellular vesicles in the immune system. Nat Rev Immunol. 2023;23(4):236–250. doi:10.1038/s41577-022-00763-8
- Hong CS, Sharma P, Yerneni SS, et al. Circulating exosomes carrying an immunosuppressive cargo interfere with cellular immunotherapy in acute myeloid leukemia. Sci Rep. 2017;7(1):14684. doi:10.1038/s41598-017-14661-w
- Kugeratski FG, Kalluri R. Exosomes as mediators of immune regulation and immunotherapy in cancer. FEBS J. 2021;288(1):10–35. doi:10.1111/febs.15558
- Wan R, Hussain A, Behfar A, Moran SL, Zhao C. The Therapeutic Potential of Exosomes in Soft Tissue Repair and Regeneration. Int J Mol Sci. 2022;23(7). doi:10.3390/ijms23073869
- Prasai A, Jay JW, Jupiter D, Wolf SE, El Ayadi A. Role of Exosomes in Dermal Wound Healing: a Systematic Review. J Invest Dermatol. 2022;142(3 Pt A):662–678.e8. doi:10.1016/j.jid.2021.07.167
- Olejarz W, Kubiak-Tomaszewska G, Chrzanowska A, Lorenc T. Exosomes in Angiogenesis and Anti-angiogenic Therapy in Cancers. Int J Mol Sci. 2020;21(16). doi:10.3390/ijms21165840
- Muthu S, Bapat A, Jain R, Jeyaraman N, Jeyaraman M. Exosomal therapy-a new frontier in regenerative medicine. Stem Cell Investig. 2021;8:7. doi:10.21037/sci-2020-037
- Lu Y, Mai Z, Cui L, Zhao X. Engineering exosomes and biomaterial-assisted exosomes as therapeutic carriers for bone regeneration. Stem Cell Res Ther. 2023;14(1):55. doi:10.1186/s13287-023-03275-x
- Kwon HH, Yang SH, Lee J, et al. Combination Treatment with Human Adipose Tissue Stem Cell-derived Exosomes and Fractional CO2 Laser for Acne Scars: a 12-week Prospective, Double-blind, Randomized, Split-face Study. Acta Derm Venereol. 2020;100(18):adv00310. doi:10.2340/00015555-3666
- Yang E, Wang X, Gong Z, Yu M, Wu H, Zhang D. Exosome-mediated metabolic reprogramming: the emerging role in tumor microenvironment remodeling and its influence on cancer progression. Signal Transduct Target Ther. 2020;5(1):242. doi:10.1038/s41392-020-00359-5
- Gao J, Li S, Xu Q, et al. Exosomes Promote Pre-Metastatic Niche Formation in Gastric Cancer. Front Oncol. 2021;11:652378. doi:10.3389/fonc.2021.652378
- Guo Y, Ji X, Liu J, et al. Effects of exosomes on pre-metastatic niche formation in tumors. Mol Cancer. 2019;18(1):39. doi:10.1186/s12943-019-0995-1
- Bahrini I, Song JH, Diez D, Hanayama R. Neuronal exosomes facilitate synaptic pruning by up-regulating complement factors in microglia. Sci Rep. 2015;5:7989. doi:10.1038/srep07989
- Chivet M, Hemming F, Pernet-Gallay K, Fraboulet S, Sadoul R. Emerging role of neuronal exosomes in the central nervous system. Front Physiol. 2012;3:145. doi:10.3389/fphys.2012.00145
- Huo L, Du X, Li X, Liu S, Xu Y. The Emerging Role of Neural Cell-Derived Exosomes in Intercellular Communication in Health and Neurodegenerative Diseases. Front Neurosci. 2021;15:738442. doi:10.3389/fnins.2021.738442
- Zhang N, He F, Li T, et al. Role of Exosomes in Brain Diseases. Front Cell Neurosci. 2021;15:743353. doi:10.3389/fncel.2021.743353
- Sun K, Zheng X, Jin H, Yu F, Zhao W. Exosomes as CNS Drug Delivery Tools and Their Applications. Pharmaceutics. 2022;14(10). doi:10.3390/pharmaceutics14102252
- Mosquera-Heredia MI, Morales LC, Vidal OM, et al. Exosomes: potential Disease Biomarkers and New Therapeutic Targets. Biomedicines. 2021;9(8). doi:10.3390/biomedicines9081061
- Boukouris S, Mathivanan S. Exosomes in bodily fluids are a highly stable resource of disease biomarkers. Proteomics Clin Appl. 2015;9(3–4):358–367. doi:10.1002/prca.201400114
- Muller L, Hong CS, Stolz DB, Watkins SC, Whiteside TL. Isolation of biologically-active exosomes from human plasma. J Immunol Methods. 2014;411:55–65. doi:10.1016/j.jim.2014.06.007
- Zhang Y, Bi J, Huang J, Tang Y, Du S. Exosome: a Review of Its Classification, Isolation Techniques, Storage, Diagnostic and Targeted Therapy Applications. Int J Nanomed. 2020;15:6917–6934. doi:10.2147/IJN.S264498
- Liang Y, Duan L, Lu J, Xia J. Engineering exosomes for targeted drug delivery. Theranostics. 2021;11(7):3183–3195. doi:10.7150/thno.52570
- Zeng H, Guo S, Ren X, Wu Z, Liu S, Yao X. Current Strategies for Exosome Cargo Loading and Targeting Delivery. Cells. 2023;12(10). doi:10.3390/cells12101416
- Liu C, Su C. Design strategies and application progress of therapeutic exosomes. Theranostics. 2019;9(4):1015–1028. doi:10.7150/thno.30853
- Kar R, Dhar R, Mukherjee S, et al. Exosome-Based Smart Drug Delivery Tool for Cancer Theranostics. ACS Biomater Sci Eng. 2023;9(2):577–594. doi:10.1021/acsbiomaterials.2c01329
- Huang L, Wu E, Liao J, Wei Z, Wang J, Chen Z. Research Advances of Engineered Exosomes as Drug Delivery Carrier. ACS Omega. 2023;8(46):43374–43387. doi:10.1021/acsomega.3c04479
- Xiong Y, Lin Z, Bu P, et al. A Whole-Course-Repair System Based on Neurogenesis-Angiogenesis Crosstalk and Macrophage Reprogramming Promotes Diabetic Wound Healing. Adv Mater. 2023;35(19):e2212300. doi:10.1002/adma.202212300
- Sun L, Fan M, Huang D, et al. Clodronate-loaded liposomal and fibroblast-derived exosomal hybrid system for enhanced drug delivery to pulmonary fibrosis. Biomaterials. 2021;271:120761. doi:10.1016/j.biomaterials.2021.120761
- Salunkhe S, Chitkara D, Mittal A. Surface functionalization of exosomes for target-specific delivery and in vivo imaging & tracking: strategies and significance. J Control Release. 2020;326:599–614. doi:10.1016/j.jconrel.2020.07.042
- He J, Ren W, Wang W, et al. Exosomal targeting and its potential clinical application. Drug Deliv Transl Res. 2022;12(10):2385–2402. doi:10.1007/s13346-021-01087-1
- Huang X, Wu W, Jing D, et al. Engineered exosome as targeted lncRNA MEG3 delivery vehicles for osteosarcoma therapy. J Control Release. 2022;343:107–117. doi:10.1016/j.jconrel.2022.01.026
- Sadeghi S, Tehrani FR, Tahmasebi S, Shafiee A, Hashemi SM. Exosome engineering in cell therapy and drug delivery. Inflammopharmacology. 2023;31(1):145–169. doi:10.1007/s10787-022-01115-7
- Masjedi MN, Sadroddiny E, Ai J, Balalaie S, Asgari Y. Targeted expression of a designed fusion protein containing BMP2 into the lumen of exosomes. Biochim Biophys Acta Gen Subj. 2023;1868(1):130505. doi:10.1016/j.bbagen.2023.130505
- Xu M, Feng T, Liu B, et al. Engineered exosomes: desirable target-tracking characteristics for cerebrovascular and neurodegenerative disease therapies. Theranostics. 2021;11(18):8926–8944. doi:10.7150/thno.62330
- Lu Y, Huang W, Li M, Zheng A. Exosome-Based Carrier for RNA Delivery: progress and Challenges. Pharmaceutics. 2023;15(2). doi:10.3390/pharmaceutics15020598
- Chen H, Yao H, Chi J, et al. Engineered exosomes as drug and RNA co-delivery system: new hope for enhanced therapeutics. Front Bioeng Biotechnol. 2023;11:1254356. doi:10.3389/fbioe.2023.1254356
- Kojima R, Bojar D, Rizzi G, et al. Designer exosomes produced by implanted cells intracerebrally deliver therapeutic cargo for Parkinson’s disease treatment. Nat Commun. 2018;9(1):1305. doi:10.1038/s41467-018-03733-8
- Chen Y, Hou S. Recent progress in the effect of magnetic iron oxide nanoparticles on cells and extracellular vesicles. Cell Death Discov. 2023;9(1):195. doi:10.1038/s41420-023-01490-2
- Chan MH, Chang ZX, Huang CF, Lee LJ, Liu RS, Hsiao M. Integrated therapy platform of exosomal system: hybrid inorganic/organic nanoparticles with exosomes for cancer treatment. Nanoscale Horiz. 2022;7(4):352–367. doi:10.1039/d1nh00637a
- Bhatia R, Chang J, Munoz JL, Walker ND. Forging New Therapeutic Targets: efforts of Tumor Derived Exosomes to Prepare the Pre-Metastatic Niche for Cancer Cell Dissemination and Dormancy. Biomedicines. 2023;11(6). doi:10.3390/biomedicines11061614
- Wang T, Jian Z, Baskys A, et al. MSC-derived exosomes protect against oxidative stress-induced skin injury via adaptive regulation of the NRF2 defense system. Biomaterials. 2020;257:120264. doi:10.1016/j.biomaterials.2020.120264
- Ranjit S, Patters BJ, Gerth KA, Haque S, Choudhary S, Kumar S. Potential neuroprotective role of astroglial exosomes against smoking-induced oxidative stress and HIV-1 replication in the central nervous system. Expert Opin Ther Targets. 2018;22(8):703–714. doi:10.1080/14728222.2018.1501473
- Shao X, Zhang M, Chen Y, Sun S, Yang S, Li Q. Exosome-mediated delivery of superoxide dismutase for anti-aging studies in Caenorhabditis elegans. Int J Pharm. 2023;641:123090. doi:10.1016/j.ijpharm.2023.123090
- Calvani N, De Marco Verissimo C, Jewhurst HL, Cwiklinski K, Flaus A, Dalton JP. Two Distinct Superoxidase Dismutases (SOD) Secreted by the Helminth Parasite Fasciola hepatica Play Roles in Defence against Metabolic and Host Immune Cell-Derived Reactive Oxygen Species (ROS) during Growth and Development. Antioxidants (Basel). 2022;11(10). doi:10.3390/antiox11101968
- Stavely R, Nurgali K. The emerging antioxidant paradigm of mesenchymal stem cell therapy. Stem Cells Transl Med. 2020;9(9):985–1006. doi:10.1002/sctm.19-0446
- Yan Y, Jiang W, Tan Y, et al. hucMSC Exosome-Derived GPX1 Is Required for the Recovery of Hepatic Oxidant Injury. Mol Ther. 2017;25(2):465–479. doi:10.1016/j.ymthe.2016.11.019
- Yao J, Zheng J, Cai J, et al. Extracellular vesicles derived from human umbilical cord mesenchymal stem cells alleviate rat hepatic ischemia-reperfusion injury by suppressing oxidative stress and neutrophil inflammatory response. FASEB J. 2019;33(2):1695–1710. doi:10.1096/fj.201800131RR
- Guo L, Chen Y, Feng X, et al. Oxidative stress-induced endothelial cells-derived exosomes accelerate skin flap survival through Lnc NEAT1-mediated promotion of endothelial progenitor cell function. Stem Cell Res Ther. 2022;13(1):325. doi:10.1186/s13287-022-03013-9
- Liu X, Yuan W, Yang L, Li J, Cai J. miRNA Profiling of Exosomes from Spontaneous Hypertensive Rats Using Next-Generation Sequencing. J Cardiovasc Transl Res. 2019;12(1):75–83. doi:10.1007/s12265-017-9784-7
- Keerthikumar S, Chisanga D, Ariyaratne D, et al. ExoCarta: a Web-Based Compendium of Exosomal Cargo. J Mol Biol. 2016;428(4):688–692. doi:10.1016/j.jmb.2015.09.019
- Pathan M, Fonseka P, Chitti SV, et al. Vesiclepedia 2019: a compendium of RNA, proteins, lipids and metabolites in extracellular vesicles. Nucleic Acids Res. 2019;47(D1):D516–D519. doi:10.1093/nar/gky1029
- van Balkom BW, Eisele AS, Pegtel DM, Bervoets S, Verhaar MC. Quantitative and qualitative analysis of small RNAs in human endothelial cells and exosomes provides insights into localized RNA processing, degradation and sorting. J Extracell Vesicles. 2015;4:26760. doi:10.3402/jev.v4.26760
- Vats S, Galli T. Introducing secretory reticulophagy/ER-phagy (SERP), a VAMP7-dependent pathway involved in neurite growth. Autophagy. 2021;17(4):1037–1039. doi:10.1080/15548627.2021.1883886
- Wojnacki J, Nola S, Bun P, et al. Role of VAMP7-Dependent Secretion of Reticulon 3 in Neurite Growth. Cell Rep. 2020;33(12):108536. doi:10.1016/j.celrep.2020.108536
- Hu X, He C, Zhang L, et al. Mesenchymal stem cell-derived exosomes attenuate DNA damage response induced by cisplatin and bleomycin. Mutat Res Genet Toxicol Environ Mutagen. 2023;889:503651. doi:10.1016/j.mrgentox.2023.503651
- Wang ZY, Wen ZJ, Xu HM, Zhang Y, Zhang YF. Exosomal noncoding RNAs in central nervous system diseases: biological functions and potential clinical applications. Front Mol Neurosci. 2022;15:1004221. doi:10.3389/fnmol.2022.1004221
- Soccio P, Moriondo G, Lacedonia D, et al. EVs-miRNA: the New Molecular Markers for Chronic Respiratory Diseases. Life. 2022;12(10):1544. doi:10.3390/life12101544
- Hashemian SM, Pourhanifeh MH, Fadaei S, Velayati AA, Mirzaei H, Hamblin MR. Non-coding RNAs and Exosomes: their Role in the Pathogenesis of Sepsis. Mol Ther Nucleic Acids. 2020;21:51–74. doi:10.1016/j.omtn.2020.05.012
- Liu X, Lin J, Zhang H, et al. Oxidative Stress in Autism Spectrum Disorder-Current Progress of Mechanisms and Biomarkers. Front Psychiatry. 2022;13:813304. doi:10.3389/fpsyt.2022.813304
- Xiong F, Jia J, Ma J, Jia Q. Glutathione-functionalized magnetic thioether-COFs for the simultaneous capture of urinary exosomes and enrichment of exosomal glycosylated and phosphorylated peptides. Nanoscale. 2022;14(3):853–864. doi:10.1039/d1nr06587d
- Linder M, Pogge von Strandmann E. The Role of Extracellular HSP70 in the Function of Tumor-Associated Immune Cells. Cancers (Basel). 2021;13(18):4721. doi:10.3390/cancers13184721
- Reddy VS, Madala SK, Trinath J, Reddy GB. Extracellular small heat shock proteins: exosomal biogenesis and function. Cell Stress Chaperones. 2018;23(3):441–454. doi:10.1007/s12192-017-0856-z
- Hu C, Yang J, Qi Z, et al. Heat shock proteins: biological functions, pathological roles, and therapeutic opportunities. MedComm. 2022;3(3):e161. doi:10.1002/mco2.161
- Sayeed N, Sugaya K. Exosome mediated Tom40 delivery protects against hydrogen peroxide-induced oxidative stress by regulating mitochondrial function. PLoS One. 2022;17(8):e0272511. doi:10.1371/journal.pone.0272511
- Wang X, Weidling I, Koppel S, et al. Detection of mitochondria-pertinent components in exosomes. Mitochondrion. 2020;55:100–110. doi:10.1016/j.mito.2020.09.006
- Chianese R, Pierantoni R. Mitochondrial Reactive Oxygen Species (ROS) Production Alters Sperm Quality. Antioxidants (Basel). 2021;10(1). doi:10.3390/antiox10010092
- Chen J, Li X, Liu H, et al. Bone marrow stromal cell-derived exosomal circular RNA improves diabetic foot ulcer wound healing by activating the nuclear factor erythroid 2-related factor 2 pathway and inhibiting ferroptosis. Diabet Med. 2023;40(7):e15031. doi:10.1111/dme.15031
- Urzì O, Cafora M, Ganji NR, et al. Lemon-derived nanovesicles achieve antioxidant and anti-inflammatory effects activating the AhR/Nrf2 signaling pathway. iScience. 2023;26(7):107041. doi:10.1016/j.isci.2023.107041
- Ji S, Xiong M, Chen H, et al. Cellular rejuvenation: molecular mechanisms and potential therapeutic interventions for diseases. Signal Transduct Target Ther. 2023;8(1):116. doi:10.1038/s41392-023-01343-5
- Zuo R, Liu M, Wang Y, et al. BM-MSC-derived exosomes alleviate radiation-induced bone loss by restoring the function of recipient BM-MSCs and activating Wnt/β-catenin signaling. Stem Cell Res Ther. 2019;10(1):30. doi:10.1186/s13287-018-1121-9
- Ramírez-Hernández AA, Reyes-Jiménez E, Velázquez-Enríquez JM, et al. Zingiber officinale-Derived Extracellular Vesicles Attenuate Bleomycin-Induced Pulmonary Fibrosis Trough Antioxidant, Anti-Inflammatory and Protease Activity in a Mouse Model. Cells. 2023;12(14). doi:10.3390/cells12141852
- Shaba E, Landi C, Carleo A, et al. Proteome Characterization of BALF Extracellular Vesicles in Idiopathic Pulmonary Fibrosis: unveiling Undercover Molecular Pathways. Int J Mol Sci. 2021;22(11):5696. doi:10.3390/ijms22115696
- Qiu Y, Li P, Zhang Z, Wu M. Insights Into Exosomal Non-Coding RNAs Sorting Mechanism and Clinical Application. Front Oncol. 2021;11:664904. doi:10.3389/fonc.2021.664904
- Shao N, Xue L, Wang R, Luo K, Zhi F, Lan Q. miR-454-3p Is an Exosomal Biomarker and Functions as a Tumor Suppressor in Glioma. Mol Cancer Ther. 2019;18(2):459–469. doi:10.1158/1535-7163.MCT-18-0725
- Valadi H, Ekström K, Bossios A, Sjöstrand M, Lee JJ, Lötvall JO. Exosome-mediated transfer of mRNAs and microRNAs is a novel mechanism of genetic exchange between cells. Nat Cell Biol. 2007;9(6):654–659. doi:10.1038/ncb1596
- Otoupalova E, Smith S, Cheng G, Thannickal VJ. Oxidative Stress in Pulmonary Fibrosis. Compr Physiol. 2020;10(2):509–547. doi:10.1002/cphy.c190017
- Lan B, Dong X, Yang Q, et al. Exosomal MicroRNAs: an Emerging Important Regulator in Acute Lung Injury. ACS Omega. 2023;8(39):35523–35537. doi:10.1021/acsomega.3c04955
- Makiguchi T, Yamada M, Yoshioka Y, et al. Serum extracellular vesicular miR-21-5p is a predictor of the prognosis in idiopathic pulmonary fibrosis. Respir Res. 2016;17(1):110. doi:10.1186/s12931-016-0427-3
- Guiot J, Cambier M, Boeckx A, et al. Macrophage-derived exosomes attenuate fibrosis in airway epithelial cells through delivery of antifibrotic miR-142-3p. Thorax. 2020;75(10):870–881. doi:10.1136/thoraxjnl-2019-214077
- Mansouri N, Willis GR, Fernandez-Gonzalez A, et al. Mesenchymal stromal cell exosomes prevent and revert experimental pulmonary fibrosis through modulation of monocyte phenotypes. JCI Insight. 2019;4(21):e128060. doi:10.1172/jci.insight.128060
- Sun L, Zhu M, Feng W, et al. Exosomal miRNA Let-7 from Menstrual Blood-Derived Endometrial Stem Cells Alleviates Pulmonary Fibrosis through Regulating Mitochondrial DNA Damage. Oxid Med Cell Longev. 2019;2019:4506303. doi:10.1155/2019/4506303
- Dinh PC, Paudel D, Brochu H, et al. Inhalation of lung spheroid cell secretome and exosomes promotes lung repair in pulmonary fibrosis. Nat Commun. 2020;11(1):1064. doi:10.1038/s41467-020-14344-7
- Mathivanan S, Fahner CJ, Reid GE, Simpson RJ. ExoCarta 2012: database of exosomal proteins, RNA and lipids. Nucleic Acids Res. 2012;40(Database issue):D1241–1244. doi:10.1093/nar/gkr828
- Zhang Y, Tan J, Miao Y, Zhang Q. The effect of extracellular vesicles on the regulation of mitochondria under hypoxia. Cell Death Dis. 2021;12(4):358. doi:10.1038/s41419-021-03640-9
- Di Mambro T, Pellielo G, Agyapong ED, et al. The Tricky Connection between Extracellular Vesicles and Mitochondria in Inflammatory-Related Diseases. Int J Mol Sci. 2023;24(9):8181. doi:10.3390/ijms24098181
- Martin-Medina A, Lehmann M, Burgy O, et al. Increased Extracellular Vesicles Mediate WNT5A Signaling in Idiopathic Pulmonary Fibrosis. Am J Respir Crit Care Med. 2018;198(12):1527–1538. doi:10.1164/rccm.201708-1580OC
- Royce SG, Patel KP, Mao W, Zhu D, Lim R, Samuel CS. Serelaxin enhances the therapeutic effects of human amnion epithelial cell-derived exosomes in experimental models of lung disease. Br J Pharmacol. 2019;176(13):2195–2208. doi:10.1111/bph.14666
- Tan JL, Lau SN, Leaw B, et al. Amnion Epithelial Cell-Derived Exosomes Restrict Lung Injury and Enhance Endogenous Lung Repair. Stem Cells Transl Med. 2018;7(2):180–196. doi:10.1002/sctm.17-0185
- Shentu TP, Huang TS, Cernelc-Kohan M, et al. Thy-1 dependent uptake of mesenchymal stem cell-derived extracellular vesicles blocks myofibroblastic differentiation. Sci Rep. 2017;7(1):18052. doi:10.1038/s41598-017-18288-9
- Sun X, Nkennor B, Mastikhina O, Soon K, Nunes SS. Endothelium-mediated contributions to fibrosis. Semin Cell Dev Biol. 2020;101:78–86. doi:10.1016/j.semcdb.2019.10.015
- Guiot J, Henket M, Corhay JL, Moermans C, Louis R. Sputum biomarkers in IPF: evidence for raised gene expression and protein level of IGFBP-2, IL-8 and MMP-7. PLoS One. 2017;12(2):e0171344. doi:10.1371/journal.pone.0171344
- Njock MS, Guiot J, Henket MA, et al. Sputum exosomes: promising biomarkers for idiopathic pulmonary fibrosis. Thorax. 2019;74(3):309–312. doi:10.1136/thoraxjnl-2018-211897
- Karami Fath M, Azami J, Masoudi A, et al. Exosome-based strategies for diagnosis and therapy of glioma cancer. Cancer Cell Int. 2022;22(1):262. doi:10.1186/s12935-022-02642-7
- Zaazaa AM, El-Motelp BA A, Ali NA, Youssef AM, Sayed MA, Mohamed SH. Stem cell-derived exosomes and copper sulfide nanoparticles attenuate the progression of neurodegenerative disorders induced by cadmium in rats. Heliyon. 2022;8(1):e08622. doi:10.1016/j.heliyon.2021.e08622
- Choi H, Choi Y, Yim HY, Mirzaaghasi A, Yoo JK, Choi C. Biodistribution of Exosomes and Engineering Strategies for Targeted Delivery of Therapeutic Exosomes. Tissue Eng Regen Med. 2021;18(4):499–511. doi:10.1007/s13770-021-00361-0
- Deng J, Pan T, Lv C, et al. Exosomal transfer leads to chemoresistance through oxidative phosphorylation-mediated stemness phenotype in colorectal cancer. Theranostics. 2023;13(14):5057–5074. doi:10.7150/thno.84937
- Kuse N, Kamio K, Azuma A, et al. Exosome-Derived microRNA-22 Ameliorates Pulmonary Fibrosis by Regulating Fibroblast-to-Myofibroblast Differentiation in Vitro and in Vivo. J Nippon Med Sch. 2020;87(3):118–128. doi:10.1272/jnms.JNMS.2020_87-302
- Zhu L, Chen Y, Chen M, Wang W. Mechanism of miR-204-5p in exosomes derived from bronchoalveolar lavage fluid on the progression of pulmonary fibrosis via AP1S2. Ann Transl Med. 2021;9(13):1068. doi:10.21037/atm-20-8033