Abstract
Silica nanoparticles (SNPs) are one of the most important nanomaterials, and have been widely used in a variety of fields. Therefore, their effects on human health and the environment have been addressed in a number of studies. In this work, the effects of amorphous SNPs were investigated with regard to multinucleation in L-02 human hepatic cells. Our results show that L-02 cells had an abnormally high incidence of multinucleation upon exposure to silica, that increased in a dose-dependent manner. Propidium iodide staining showed that multinucleated cells were arrested in G2/M phase of the cell cycle. Increased multinucleation in L-02 cells was associated with increased generation of cellular reactive oxygen species and mitochondrial damage on flow cytometry and confocal microscopy, which might have led to failure of cytokinesis in these cells. Further, SNPs inhibited cell growth and induced apoptosis in exposed cells. Taken together, our findings demonstrate that multinucleation in L-02 human hepatic cells might be a failure to undergo cytokinesis or cell fusion in response to SNPs, and the increase in cellular reactive oxygen species could be responsible for the apoptosis seen in both mononuclear cells and multinucleated cells.
Introduction
Nanotechnology can be defined as the science and engineering involved in the design, synthesis, characterization, and application of materials and devices, the smallest organization of which in at least one dimension is on the nanometer scale.Citation1 The rapid development of nanotechnology in recent years has created a myriad of engineered nanomaterials, including silica nanoparticles (SNPs), which are increasingly being used in industrial processes as well as in scientific, biological, and medical research.Citation2,Citation3 The direct impact of these materials on human health and the environment is of great concern to the population.Citation4
Recent investigations have indicated that SNPs can enter the body through the respiratory system, digestive tract, skin, and blood circulation, and accumulate in vital internal organs, including the lung, liver, spleen, and kidney, causing inflammation and severe tissue damage.Citation5–Citation7 It has been confirmed in previous in vitro studies that SNPs can cross the cell membrane, distribute into the cytoplasm and nucleus,Citation8,Citation9 and cause cytotoxicity and genotoxicity in many cell lines.Citation10–Citation12
Multinucleation can be divided into syncytium and plasmodium.Citation13 A syncytium is generated by cell fusion,Citation14 and is frequently observed in normal tissue. Multinucleated osteoclasts are formed by fusion of mononuclear cells in the process of bone resorption.Citation15 Macrophages can fuse to become multinuclear giant cells when adequately stimulated extracellularly.Citation16 A plasmodium is formed by karyokinesis without cytokinesis, and results in polyploidy or aneuploidy, such as in normal hepatocytes during growth of liver tissue.Citation17,Citation18 Plasmodium seems to be due to failure to form the central spindle and contractile ring, resulting from many mechanisms, such as lipid metabolism and abnormalities of the actin filaments and microtubules.Citation19 Further, formation of polyploidy and aneuploidy is the early event and main mark for a majority of solid tumors.Citation20 Abnormal cell division can decrease genomic stability and increase the risk of tumors.Citation21
It has been reported that some types of nanomaterials can enter cells, cause cytoskeletal dysfunction,Citation22,Citation23 and result in formation of multinucleated cells.Citation24 In general, reactive oxygen species (ROS) generation induced by nanoparticles is considered to be one of the important mechanisms associated with their toxicity.Citation25 Research indicates that SNPs increase intracellular ROS levels, and result in cellular dysfunction in several cultured cell lines.Citation26–Citation28 Meanwhile, additional ROS could induce amplification of multipolar spindles and irregular centrosomes,Citation29 which might lead to formation of multinucleated cells.
In a previous study, we reported for the first time that SNPs could induce multinucleation in HepG2 cells.Citation30 Interestingly, a similar result was obtained in L-02 cells in the present study. It was hypothesized that multinucleation in L-02 cells represents a failure to undergo cytokinesis or cell fusion resulting from extracellular ROS induced by SNPs. In this paper, we demonstrate that multinucleation of L-02 human liver cells can be induced in vitro in response to SNPs. Intracellular ROS generation, mitochondrial damage, arrest of the cell cycle, and apoptosis of L-02 cells were also investigated to clarify further the possible mechanisms of multinucleation.
Materials and methods
Preparation of amorphous SNPs
Amorphous SNPs were synthesized using the StöberCitation31 method. Tetraethoxysilane (Tiantai Chemical Co Ltd, Wuhan, People’s Republic of China) was distilled under reduced pressure before use. Pure grade ethanol (99%, Beijing Chemical Reagent Company, Beijing, People’s Republic of China) and ammonium hydroxide (25%, Beijing Chemical Reagent Company) were used as received. Water was of high purity grade with a resistivity of 18.2 MΩ cm. Briefly, the SNPs were prepared by adding 0.3 mL of tetraethoxysilane to 30 mL of premixed ethanol solution containing 1.0 mL0020of ammonium hydroxide and 0.6 mL of water. The mixture was kept in a 40°C bath and magnetically stirred for 3 hours. Another 0.3 mL of tetraethoxysilane was then added and reacted for 3 hours. Finally, the SNPs were isolated by centrifugation for 15 minutes at 12,000 rpm and washed twice with ethanol and water, then redispersed in 30 mL of high purity water. The suspension of SNPs was then sterilized by autoclaving at 121°C for 20 minutes before the biological experiments.
Characterization of SNPs
First, 1 mL of the suspension was allowed to dry at 80°C for 24 hours, the residue was weighed and the concentration of particles was calculated. The morphology and average size of the SNPs was observed using a transmission electron microscope (JEOL Ltd Tokyo, Japan). Purity of the SNPs was assessed by inductively coupled plasma atomic emission spectrometry (Thermo Fisher Scientific, ARL 3520, Ecublens, Switzerland). A zeta electric potential granulometer (Malvern Instruments, Malvern, UK) was used to determine the zeta potential and hydrodynamic size of the SNPs in distilled water and Roswell Park Memorial Institute (RPMI) 1640 culture medium (Gibco, Grand Island NY, USA).
Cell culture and treatment with SNPs
An L-02 human hepatic cell line obtained from the Cell Resource Center, Shanghai Institutes for Biological Sciences, Chinese Academy of Sciences, was cultured in RPMI 1640 medium supplemented with 10% fetal bovine serum (HyClone, Logan, UT, USA), 100 U/mL penicillin, and 100 μg/mL streptomycin, and grown at 37°C in a humidified 5% CO2 environment. Cells used in this study were in early passages (10–30).
For the experiments, the cells were seeded in culture plates at a density of 1 × 105 cells/mL and allowed to attach for 24 hours, then treated with SNPs suspended in RPMI 1640 culture medium for another 24 hours. SNP suspensions were formed using a sonicator (160 W, 20 kHz, 5 minutes, KQ-250B, Kunshan Ultrasonic Instruments Co Ltd Jiangsu, People’s Republic of China) and diluted to four concentrations (50, 100, 150, and 200 μg/mL), then applied immediately to the L-02 cells. Cells maintained in RPMI 1640 culture medium without SNPs were used as the control. Each group had five replicate wells.
Assessment of cytotoxicity
The effect of SNPs on cell viability was determined using a cell counting kit (CCK-8, Dojindo Laboratories, Kumamoto, Japan), which is more sensitive than the MTT assay. Briefly, L-02 cells were incubated with or without the various concentrations of SNPs for 24 hours. Next, 10 μL of CCK-8 solution was added to each well, and the cells were incubated for a further 2 hours at 37°C. The plate was then read using a Sunrise microplate reader (Tecan Austria GmbH, Grödig, Austria) at 450 nm.
Assessment of multinucleation
After attachment, the cells were incubated with 50, 100, 150, or 200 μg/mL of SNPs for 24 hours. The medium was then removed and the cells were washed twice with phosphate-buffered saline and stained with 10 μg/mL Hoechst 33258 solution (Sigma, St Louis, MO, USA) for 45 minutes and 5 μg/mL of fluorescein diacetate (Sigma) for 10 minutes at 37°C in the dark. The cells were visualized by ultraviolet excitation and photographed using a laser confocal microscope (model TCSNT, Leica, Heidelberg, Germany). Fields were selected at random, numbers of binucleated and multinucleated cells were counted manually by two independent observers, and the rate of total multinucleated cells was then calculated.
Assessment of cell cycle
Following treatment with different concentrations of SNPs for 24 hours, the cells were harvested by centrifugation for 5 minutes at 4°C and 1,000 rpm, then washed twice with ice-cold phosphate buffered saline-ethylenediamine tetraacetic acid (PBS-EDTA), fixed in ice-cold 70% ethanol, and frozen at 20°C overnight. On the following day, the cells were washed twice with PBS-EDTA and resuspended in 450 μL of PBS-EDTA solution containing 7.5 μM of propidium iodide (PI) and 100 μg/mL of RNase for 45 minutes at 37°C in the dark. The DNA content was analyzed using a flow cytometer (Becton Dickinson, BD Biosciences, Franklin Lakes, NJ, USA). At least 10,000 cells in each sample were collected and the population of cells in each phase of the cell cycle was determined using Cell ModiFIT software (Verity Software House, Topsham, ME, USA).
Assessment of intracellular ROS
Intracellular ROS levels were monitored using 2′,7′-dichlorofluorescein diacetate. After treatment with the different concentrations of SNPs for 24 hours, the cells were harvested by centrifugation for 5 minutes at 4°C and 1,000 rpm. Next, 2.5 μg/mL 2′,7′-dichlorofluorescein diacetate was added and staining was carried out for 20 minutes in the dark at 37°C. The cells were then washed with phosphate-buffered saline and analyzed by flow cytometry.
Assessment of mitochondrial damage
Mitochondrial damage in the L-02 cells was estimated using MitoTracker® Green (Invitrogen, Carlsbad CA, USA), which is a mitochondria-specific fluorescent dye. The cells were treated with different concentrations of SNPs for 24 hours. After washing with phosphate-buffered saline, the cells were stained with 50 μmol/L MitoTracker Green for 30 minutes in the dark at 37°C and then analyzed using a laser confocal microscope with Leica TCSNT software (Leica, Wetzlar, Germany).
Assessment of apoptosis
Cell apoptosis was determined using an Annexin V-FITC/PI apoptosis kit (Baosai Reagent, Baosai Biological Technology Co, Ltd Beijing, People’s Republic of China). Following treatment, the cells were harvested and resuspended in 500 μL of binding buffer and 10 μL of Annexin V/FITC conjugate, then incubated for 10 minutes in the dark at 4°C. Five minutes before detection, 5 μL of PI was added and the cells were analyzed using flow cytometry at an excitation wavelength of 488 nm and an emission wavelength of 630 nm. Next, 10,000 events in each sample were collected and the apoptotic percentages were analyzed by Cell ModiFIT software. The cell nuclei were then stained with 10 μg/mL Hoechst 33258 solution for 45 minutes in the dark at 37°C and then visualized and photographed using a laser confocal microscope. Five hundred binucleated and multinucleated cells were randomly selected and the number of apoptotic cells was counted manually by two independent observers, after which the apoptotic rate in multinucleated cells was calculated.
Statistical analysis
Data for multinucleated cell numbers and rate of apoptosis in multinucleated cells were reported as frequencies and analyzed by chi-square test. Other data are expressed as the mean±standard deviation. Statistical analysis was performed using one-way analysis of variance with Dunnett’s test. P < 0.05 was considered to be statistically significant.
Results
Characterization of SNPs
Transmission electron microscopy showed that the amorphous SNPs were uniform in size and evenly distributed with good monodispersity and a spherical shape (). The average size of these nanoparticles was 53 ± 5.1 nm, which was determined by measuring 300 particles with ImageJ software (National Institutes of Health, Bethesda, MD, USA). The purity of the amorphous SNPs was higher than 99.9%. Their hydrodynamic diameter and zeta potential were measured in distilled water as the stock medium and in RMPI 1640 as the exposure medium at 0, 3, 6, and 24 hours to reflect their dispersion throughout the experiments. As shown in , the hydrodynamic diameter of these particles was about 90 nm and did not change significantly with time. Zeta potential measurement showed that the SNPs were highly negatively charged (about −30 mV), indicating a fairly stable suspension in both types of dispersion medium.
Table 1 Time evolution of the hydrodynamic diameter and zeta potential of 53 nm silica nanoparticles in different dispersion medium
Cell viability
After exposure of the cells to various concentrations of SNPs for 24 hours, cytotoxicity was estimated using a CCK-8 kit. As shown in , the L-02 cell survival rate decreased with increasing silica exposure. Cell viability in the groups treated with 50, 100, 150, and 200 μg/mL SNPs decreased to 71.32%, 54.42%, 40.66%, and 31.89%, respectively. Significant differences in cell viability were seen between the group treated with 50 μg/mL SNPs and the control group after exposure (P < 0.05).
Figure 2 Viability of L-02 cells treated with different concentrations of silica nanoparticles for 24 hours. The results indicate that viability is reduced in a dose-dependent manner. Data are expressed as the mean ± standard deviation of three independent experiments. *P < 0.05 versus control group using analysis of variance.
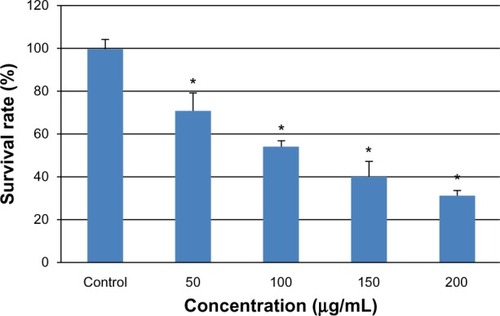
Cell multinucleation
shows the results of a representative multinucleation experiment in which L-02 cells were incubated with 100 μg/mL SNPs for 24 hours. The use of Hoechst 33258 as a DNA labeling agent enabled determination of the DNA content in all cells by measuring the integrated fluorescence emitted by each nucleus. Cells in the control group were normal with round and homogeneously stained nuclei, with only a few multinucleated cells observed, whereas giant cells containing more than one nucleus were frequently observed upon exposure to different concentrations of SNPs, suggesting that these cells had become multinucleated. Multinucleation increased in a concentration-dependent manner, increasing from 1.6% in the control group to 19.2% in the group treated with 150 μg/mL SNPs, and decreasing slightly to 12.6% in the group treated with 200 μg/mL SNPs ().
Table 2 Number of binucleated and multinucleated cells induced by silica nanoparticles
Figure 3 Multinucleation in L-02 cells induced by silica nanoparticles. (A) Control group and (B) group treated with100 μg/mL silica nanoparticles. The cell nucleus and cytoplasm were stained by Hoechst 33258 and fluorescein diacetate, respectively. Binucleated cells (arrows) and multinucleated cells (amplification) were observed.
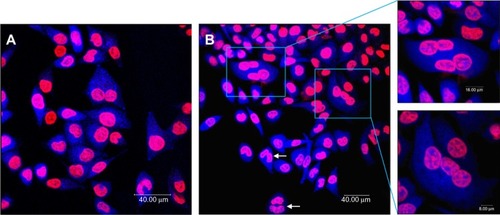
Arrest of cell cycle
To investigate the basis for multinucleation further, we examined cell cycle progression by flow cytometry. Arrest of the cell cycle was observed in all four treatment groups (). As the silica dose increased, the percentage of cells in G0/G1 phase gradually decreased, whereas the percentage of cells in G2/M phase increased significantly compared with the control group (P < 0.05). This arrest of G2/M phase corresponded to an increase in the number of multinucleated cells observed by laser confocal microscopy.
Figure 4 G2/M phase cell cycle arrest of L-02 cells induced by silica nanoparticles. After exposure to 50, 100, 150, and 200 μg/mL silica nanoparticles for 24 hours, the L-02 cell cycle was determined by flow cytometry. As the silica dose increased, the percentage of cells in G0/G1 phase decreased and the percentage of cells in G2/M phase increased significantly. Data are expressed as the mean ± standard deviation of three independent experiments. *P < 0.05 versus control group using analysis of variance.
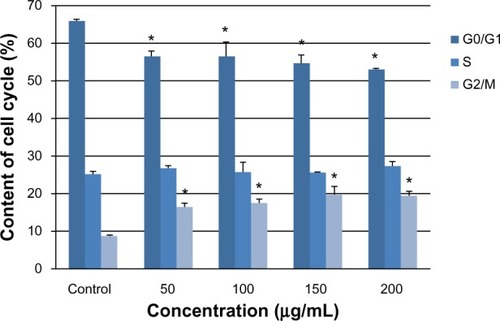
Increased generation of intracellular ROS
Intracellular ROS was determined by flow cytometry after exposing the L-02 cells to 0–200 μg/mL SNPs for 24 hours. The results showed a marked shift in the M2 peak of fluorescence intensity for 2′,7′-dichlorofluorescein diacetate (), suggesting that the SNPs caused an increase in generation of ROS in L-02 cells. The fluorescence intensity in the groups treated with 0, 50, 100, 150, and 200 μg/mL SNPs was 218.1, 385.8, 441.3, 455.4, and 588.2, respectively (). Concentration dependency of ROS induction was clearly observed.
Figure 5 Change in intracellular ROS levels in L-02 cells after 24 hours of incubation with different concentrations of silica nanoparticles. The results of flow cytometry (A) and the corresponding bar graph (B) are shown. Intracellular ROS levels increased in a dose-dependent manner. Data are expressed as the mean ± standard deviation of three independent experiments. *P < 0.05 versus control group using analysis of variance.
Abbreviation: ROS, reactive oxygen species.
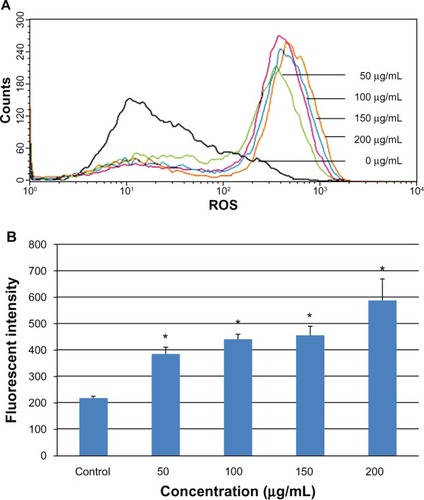
Mitochondrial damage
Mitochondrial damage in L-02 cells was monitored by laser confocal microscopy using the mitochondria-specific probe, Mito Tracker Green. shows that mitochondrial fluorescence intensity decreased in a dose-dependent manner on incubation of L-02 cells with SNPs for 24 hours, with increasing numbers of inactive or damaged mitochondria appearing in the group treated with SNPs.
Figure 6 Mitochondrial damage to L-02 cells after exposure to different concentrations of silica nanoparticles for 24 hours detected by Mito-Tracker® green. (A) Control group, (B) group treated with 50 μg/mL silica nanoparticles, (C) group treated with 100 μg/mL silica nanoparticles, (D) group treated with 150 μg/mL silica nanoparticles, and (E) group treated with 200 μg/mL silica nanoparticles. The corresponding bar graph for flow cytometry is shown in (F). Mitochondrial damage increased in a dose-dependent manner. Data are expressed as the mean ± standard deviation of three independent experiments. *P < 0.05 versus control group using analysis of variance.
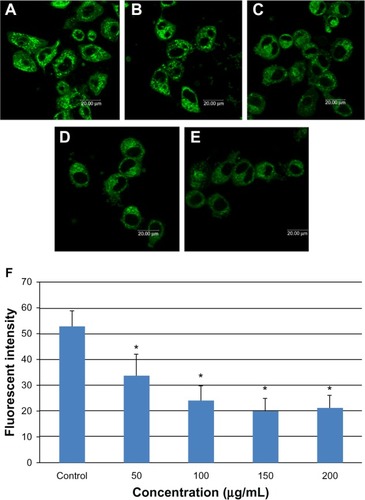
Apoptosis of L-02 cells
As shown in , the SNPs induced obvious morphologic changes in the L-02 cell nuclei. Intact and evenly stained nuclei were visualized in untreated cells, whereas condensed and aggregated chromatin was found in the groups treated with SNPs, indicating apoptotic nuclei. The apoptotic rate elevated significantly from the group treated with 50 μg/mL SNPs (). Compared with the control group, the apoptotic rate increased by 13.0%, 29.2%, 31.3%, and 33.4%, respectively, after exposure to 0, 50, 100, 150 and 200 μg/mL SNPs for 24 hours.
Figure 7 Apoptosis of L-02 cells after 24 hours of incubation with silica nanoparticles. Hoechst 33258 was used to observe morphologic changes in the cell nucleus. (A) Control group and (B) group treated with 100 μg/mL silica nanoparticles. Nuclear condensation (arrowhead) and chromatin margination (arrow) are seen in the silica nanoparticle-treated group. The corresponding bar graph for apoptotic rate detected by flow cytometry is shown in (C). Apoptosis induced by silica nanoparticles increased in a dose-dependent manner. Data are expressed as the mean ± standard deviation of three independent experiments. *P < 0.05 versus control group using analysis of variance.
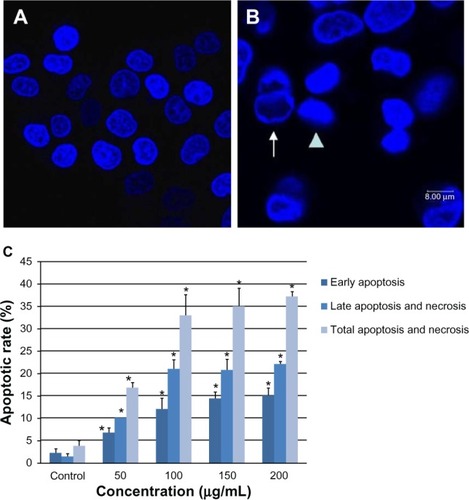
Apoptosis of multinucleated cells
We also stained the L-02 cells with Annexin V-FITC/PI. Interestingly, some multinucleated cells could also be stained by Annexin V-FITC or PI, indicating these cells were undergoing early or late apoptosis (). Compared with the control group, the multinuclear apoptotic rate increased significantly with increasing SNP doses (). In the group treated with 200 μg/mL SNPs, the multinuclear apoptotic rate increased to 42.85%, suggesting that apoptosis was occurring in almost half of the multinuclear cells.
Figure 8 Apoptosis of multinucleated L-02 cells induced by silica nanoparticles. Early (A) and late (B) apoptosis of multinucleated cells was observed (arrowhead) in the group treated with100 μg/mL silica nanoparticles. (a) Hoechst 33258, (b) FITC-Annexin V (c) propidium iodide, and (d) merged image. The corresponding bar graph for apoptotic rate of multinucleated cells is shown in (C). The results indicate that the apoptotic rate of multinucleated cells also increased in a dose-dependent way. *P < 0.05 versus control group using Chi-square test.
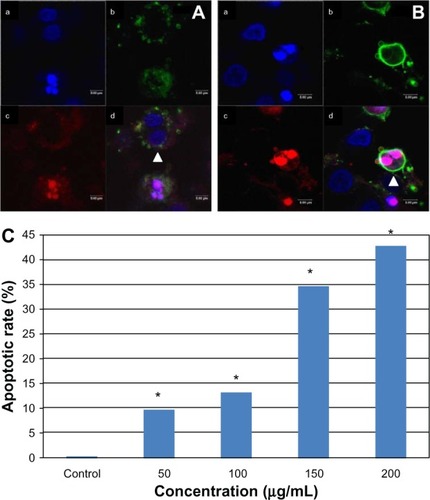
Discussion
In this study, we investigated multinucleation in L-02 cells after exposure to SNPs. Significant enlargement and multinucleation of L-02 cells was observed following exposure, in comparison to control cells (). Consistent with multinucleation data, flow cytometry showed that L-02 cells treated with the different concentrations of SNPs for 24 hours exhibited a significant increase in the percentage of cells arrested in G2/M phase, and in a dose-dependent manner (). G2/M arrest could be G2 or M phase arrest according to the flow cytometry analysis. However, considering multinucleation in cells, we can presume that these cells were arrested in M phase with two or more separate nuclei in one single cell, rather than in G2 phase which is basically one single 4n nucleus in the cell. Segregation of replicated chromosomes in mitosis must be precisely coordinated with the process of cytokinesis in space and time. Failure to separate the proper complement of chromosomes into each daughter cell before cytokinesis can result in polyploidy or aneuploidy,Citation32 which is linked to hepatocyte ploidy status during liver growthCitation20 and development of cancer.Citation21
Cytokinesis is the cell division process at the end of mitosis in which the membrane is physically cleaved and the daughter cells are separated after segregation of the chromosomes. Correct cytoplasmic separation involves sequential alterations in the microtubule cytoskeleton. During this process, adherent cells undergo notable changes in shape that are driven by the actin cytoskeleton and microtubule network.Citation33 Nuclear division (karyogenesis) which is not followed by cytokinesis can lead to multinucleation. Our results show that the rate of multinucleation in L-02 cells induced by SNPs increased in a dose-dependent manner (), and the intracellular ROS level also increased as the silica dose increased (). This suggests that the SNPs used in our study might have caused structural injury and dysfunction of microfilaments, microtubules, or centrosomes via intracellular ROS generation, leading to failure of cytokinesis and multinucleation in L-02 cells.
An insufficient supply of adenosine triphosphate (ATP) for energy during cell division might be another reason for multinucleation. Cells actively provide contractile forces for many processes, including cytokinesis and motility. Contractility is known to depend on myosin II motors which convert chemical energy from hydrolysis of ATP into forces on actin filaments.Citation34 The mitochondria provide most of the energy required for cellular function. This energy comes from ATP, which is produced by the Krebs cycle and the electron transport chain in mitochondria through oxidation of fats, carbohydrates, and proteins.Citation35 In our study, the number of damaged mitochondria in L-02 cells dramatically increased following treatment with SNPs for 24 hours (), which would subsequently reduce the energy supply in the form of ATP in cells, resulting in failure of cytokinesis.
Cell fusion could also be involved in the multinucleation of L-02 cells induced by SNPs. Research has suggested that after one cell divides to generate two daughter cells, it is possible that a thin cellular bridge connecting the daughter cells still remains after cell division and the cells do not separate completely, with the daughter cells fusing to form one cell again.Citation13 In vitro, nanoparticles can penetrate the cell membrane by diffusion or adhesion,Citation36 and the size of these particles could be an important factor. The reaction between the particles and the cell membrane results in generation of ROS, and the oxidative stress generated might cause breakdown of the membrane lipids,Citation37,Citation38 which might lead to fusion of the cell membrane and result in multinucleation. Therefore, increased cellular ROS after exposure to silica could be one of the reasons for cell fusion and subsequent cell multinucleation.
In summary, multinucleation in L-02 cells is generated mainly by karyokinesis without cytokinesis and accumulation of ROS, and lack of sufficient mitochondria might contribute to this process. Cell fusion is another possible reason and should be taken into account.
Another concern is the fate of multinucleated cells. It is believed that excessive intracellular ROS levels could trigger redox signaling pathways, producing a series of cytotoxic events.Citation39 First, high amounts of oxidative stress induced by ROS are suspected to be involved in injuring the mitochondria and perturbing the mitochondrial permeability transition pore, resulting in cellular apoptosis.Citation25 Second, there is a clear relationship between ROS and oxidative damage to DNA.Citation40 Therefore, increased levels of ROS in L-02 cells could also be one of the reasons for arrest of G2/M phase because of the G2/M check point. Cell cycle checkpoints function to ensure that cells have time to complete DNA repair, whereas apoptotic cell death may function to eliminate damaged cells that are irreparable or unrepaired. Apoptosis, a unique type of programmed cell death, plays an important role in maintenance of homeostasis and elimination of damaged cells.Citation41 As shown in and , both mononuclear cells and multinucleated cells underwent apoptosis; in addition, the apoptosis rate increased significantly with increasing doses of silica ( and ); nearly half of the cells failing to progress to mitosis are destined for apoptosis after exposure to SNPs. These findings suggest that SNPs can lead not only to multinucleation but also to apoptosis in L-02 cells.
However, the specific pathways or molecular mechanisms of multinucleation in L-02 cells induced by SNPs are far from understood. In subsequent studies, we will investigate the relationship between multinucleation and failure of cytokinesis or cell fusion further. This phenomenon and the related mechanisms need to be confirmed in animal experiments in vivo.
Conclusion
In the present study, we demonstrated that exposure to SNPs can induce multinucleation in L-02 cells in a dose-dependent manner. Our results also indicate that interaction of SNPs with L-02 cells could increase intracellular ROS levels. Excessive ROS could induce mitochondrial or DNA damage, which would initiate the process of apoptosis in both mononuclear cells and multinucleated cells.
Acknowledgments
This work was supported by the National Natural Science Foundation of China (81172704), Innovative Team Project of Beijing Education Committee (PHR201107116), and Doctoral Program of Higher Education (20090061110062). The authors would like to thank Wensheng Yang from Jilin University for preparation of the silica nanoparticles.
Disclosure
The authors report no conflicts of interest in this work.
References
- OberdörsterGOberdörsterEOberdörsterJNanotoxicology: an emerging discipline evolving from studies of ultrafine particlesEnviron Health Perspect200511382383916002369
- OrrGPantherDJCassensKJPhillipsJLTarasevichBJPoundsJGSyndecan-1 mediates the coupling of positively charged submicrometer amorphous silica particles with actin filaments across the alveolar epithelial cell membraneToxicol Appl Pharmacol200923621022019371605
- BashirMMNavinKVAdrielePMActivation of stress-related signalling pathway in human cells upon SiO2 nanoparticles exposure as an early indicator of cytotoxicityJ Nanobiotechnology201192921801388
- NapierskaDThomassenLCLisonDMartensJAHoetPHThe nanosilica hazard: another variable entityPart Fibre Toxicol201073921126379
- ChoWSChoiMHanBSInflammatory mediators induced by intratracheal instillation of ultrafine amorphous silica particlesToxicol Lett2007175243317981407
- ChoMChoWSChoiMThe impact of size on tissue distribution and elimination by single intravenous injection of silica nanoparticlesToxicol Lett200918917718319397964
- XieGSunJZhongGShiLZhangDBiodistribution and toxicity of intravenously administered silica nanoparticles in miceArch Toxicol20108418319019936708
- MuQHondowNSKrzemińskiLBrownAPJeukenLJRoutledgeMNMechanism of cellular uptake of genotoxic silica nanoparticlesPart Fibre Toxicol201223929
- ChenMMikeczAFormation of nucleoplasmic protein aggregates impairs nuclear function in response to SiO2 nanoparticlesExp Cell Res2005305516215777787
- WangJJSandersonBJWangHCytotoxicity and genotoxicity of ultrafine crystalline SiO2 particulate in cultured human lymphoblastoid cellsEnviron Mol Mutagen20074815115717285640
- SohaebuddinSKThevenotPTBakerDEatonJWTangLNanomaterial cytotoxicity is composition, size, and cell type dependentPart Fibre Toxicol201072220727197
- RabolliVThomassenLCUwambayinemaFMartensJALisonDThe cytotoxic activity of amorphous silica nanoparticles is mainly influenced by surface area and not by aggregationToxicol Lett201120619720321803137
- YoshidaKObataSOnoMEsakiMMaejimaTSawadaHTPA-induced multinucleation of a mesenchymal stem cell-like clone is mediated primarily by karyokinesis without cytokinesis, although cell-cell fusion also occursEur J Cell Biol20078646147117599648
- AndersonJMMultinucleated giant cellsCurr Opin Hematol20007404710608503
- MiyamotoTSudaTDifferentiation and function of osteoclastsKeio J Med2003521712713016
- JunqueiraLCCarneiroJConnective tissueBasic Histology: Text and Atlas11th edNew York, NYThe McGraw-Hill Companies2005
- GuidottiJEBregerieORobertADebeyPBrechotCDesdouetsCLiver cell polyploidization: a pivotal role for binuclear hepatocytesJ Biol Chem2003278190951910112626502
- DuncanAWHanlon NewellAESmithLFrequent aneuploidy among normal human hepatocytesGastroenterology2012142252822057114
- CiminiDFioravantiDTanzarellaCDegrassiFSimultaneous inhibition of contractile ring and central spindle formation in mammalian cells treated with cytochalasin BChromosoma19981074794859914380
- AriizumiTOgoseAKawashimaHHottaTUmezuHEndoNMultinucleation followed by an acytokinetic cell division in myxofibrosarcoma with giant cell proliferationJ Exp Clin Cancer Res2009284419335880
- RaoCVYamadaHYYaoYDaiWEnhanced genomic instabilities caused by deregulated microtubule dynamics and chromosome segregation: a perspective from genetic studies in miceCarcinogenesis2009301469147419372138
- MöllerWHoferTZiesenisAKargEHeyderJUltrafine particles cause cytoskeletal dysfunctions in macrophagesToxicol Appl Pharmacol200218219720712183099
- JukkaSHarriAMinnamariVKaiSAnnePProteomic characterization of engineered nanomaterial-protein interactions in relation to surface reactivityACS Nano201154300430921528863
- HuangSChuehPJLinYWShihTSChuangSMDisturbed mitotic progression and genome segregation are involved in cell transformation mediated by nano-TiO2 long-term exposureToxicol Appl Pharmacol200924118219419695278
- NelAXiaTMadlerLLiNToxic potential of materials at the nanolevelScience200631162262716456071
- LiuXSunJEndothelial cells dysfunction induced by silica nanoparticles through oxidative stress via JNK/P53 and NF-kappaB pathwaysBiomaterials2011318198820920727582
- NabeshiHYoshikawaTMatsuyamaKAmorphous nanosilica induce endocytosis-dependent ROS generation and DNA damage in human keratinocytesPart Fibre Toxicol20118121235812
- HiromiNTomoakiYKeigoMAmorphous nanosilica induce endocytosis-dependent ROS generation and DNA damage in human keratinocytesPart Fibre Toxicol20118121235812
- ChaeSYunCUmHLeeJHChoHCentrosome amplification and multinuclear phenotypes are induced by hydrogen peroxideExp Mol Med20053748248716264273
- LiYSunLJinMSize-dependent cytotoxicity of amorphous silica nanoparticles in human hepatoma HepG2 cellsToxicol In Vitro2011251343135221575712
- StöberWFinkABohnEControlled growth of monodisperse silica spheres in the micronsize rangeJ Colloid Interface Sci1986266269
- LeversonJDHuangHKForsburgSLHunterTThe Schizosaccharomyces pombe aurora-related kinase Ark1 interacts with the inner centromere protein Pic1 and mediates chromosome segregation and cytokinesisMol Biol Cell2002131132114311950927
- PellinenTTuomiSArjonenAIntegrin trafficking regulated by Rab21 is necessary for cytokinesisDev Cell20081537138518804435
- BendixPMKoenderinkGHCuvelierDA quantitative analysis of contractility in active cytoskeletal protein networksBiophys J2008943126313618192374
- RicquierDBouillaudFMitochondrial uncoupling proteins: from mitochondria to the regulation of energy balanceJ Physiol200052931011080246
- KasperJHermannsMIBantzCInflammatory and cytotoxic responses of an alveolar-capillary coculture model to silica nanoparticles: comparison with conventional monoculturesPart Fibre Toxicol20118621272353
- LiSQZhuRRZhuHNanotoxicity of TiO(2) nanoparticles to erythrocyte in vitroFood Chem Toxicol2008463626363118840495
- ChoiSJOhJMChoyJHToxicological effects of inorganic nanoparticles on human lung cancer A549 cellsJ Inorg Biochem200910346347119181388
- SunLLiYLiuXCytotoxicity and mitochondrial damage caused by silica nanoparticlesToxicol In Vitro2011251619162921723938
- GerloffKAlbrechtCBootsAWFörsterISchinsRPFCytotoxicity and oxidative DNA damage by nanoparticles in human intestinal Caco-2 cellsNanotoxicology20093355364
- DoonanFCotterTGMorphological assessment of apoptosisMethods20084420020418314050