Abstract
Background
Silver nanoparticles (AgNPs) have been associated with the exacerbation of asthma; however, the immunological basis for the adjuvant effects of AgNPs is not well understood.
Objective
The aim of the study reported here was to investigate the allergic effects of AgNP inhalation using proteomic approaches.
Methods
Allergen provoked mice were exposed to 33 nm AgNPs at 3.3 mg/m3. Following this, bronchoalveolar lavage fluid (BALF) and plasma were collected to determine protein profiles.
Results
In total, 106 and 79 AgNP-unique proteins were identified in the BALF of control and allergic mice, respectively. Additionally, 40 and 26 AgNP-unique proteins were found in the plasma of control and allergic mice, respectively. The BALF and plasma protein profiles suggested that metabolic, cellular, and immune system processes were associated with pulmonary exposure to AgNPs. In addition, we observed 18 proteins associated with systemic lupus erythematosus that were commonly expressed in both control and allergic mice after AgNP exposure. Significant allergy responses were observed after AgNP exposure in control and allergic mice, as determined by ovalbumin-specific immunoglobulin E.
Conclusion
Inhaled AgNPs may regulate immune responses in the lungs of both control and allergic mice. Our results suggest that immunology is a vital response to AgNPs.
Introduction
Silver nanoparticles (AgNPs) are clusters of silver atoms with at least one dimension measuring <100 nm. AgNPs have antibacterial and antimicrobial properties that are advantageous for applications in medicine. Therefore, AgNPs are increasingly utilized substrates that have been highly commercialized. For example, clothing industries have added AgNPs into fabrics used to make socks, exploiting the antibacterial activity to neutralize odor-forming bacteria.Citation1 AgNPs have also been integrated into plastics and surface coatings. Recently, AgNPs have been investigated for treating asthma;Citation2,Citation3 however, the effects of AgNP inhalation on healthy and asthmatic individuals are unclear.
The potential for nanomedicine in allergen immunotherapy is currently being investigated and developed. AgNPs are candidates for the treatment of allergic asthma.Citation2,Citation3 AgNPs have larger surface area-to-volume ratios for interacting with bacteria and lower toxicity than other metal nanoparticles (NPs).Citation4 A recent study of the immunology of AgNPs suggested that they have significant adjuvant effects and that the mechanism of these effects is the recruitment and activation of local leukocytes, especially macrophages.Citation5 Park et al observed that AgNPs might have an antioxidant effect by attenuating antigen-induced airway inflammation and hyperresponsiveness.Citation2 Further, AgNPs suppressed mucus hypersecretion and the vascular endothelial growth factor signaling pathway during allergic airway inflammation.Citation3 However, biological applications employing AgNPs should be thoroughly studied for potential negative side effects.Citation6,Citation7
Toxicoproteomics is increasingly applied to discover diagnostic biomarkers of human disease, for example, lung cancer.Citation8 A “protein biomarker” is a protein or peptide that can be objectively and quantitatively measured and evaluated as an indicator of normal or pathological biological processes, exposure to environmental factors, lifestyle factors, the presence of pathogens, or responsiveness to a therapeutic intervention. In this regard, bronchoalveolar lavage fluid (BALF) and blood samples may be used to measure biomarkers for several purposes, including diagnosis, screening, evaluation of risk or predisposition, assessment of prognosis, or surrogate markers.Citation9 Several techniques have been developed for proteomic research. For example, two-dimensional gel electrophoresis is commonly used to identify unique protein expression profiles in biological samples. Mass spectrometry for protein identification has been successfully utilized in a number of toxicology studies. Further, gas-phase fractionation is an iterative mass spectrometry approach that examines multiple smaller mass-to-charge ranges. This process enables the ions selected for collision-induced dissociation to come from a greater number of unique peptides compared with the ions selected from the wide mass range scan in automated liquid chromatography-tandem mass spectrometry analysis.Citation10,Citation11 Proteomics approaches are becoming more popular in clinical medicine and environmental toxicology.
Inflammation, thrombosis and coagulation, and vascular function and heart rate variability are the human pathophysiological responses to particulate pollution exposure.Citation12 However, there is no standard guidance for AgNP inhalation exposure. Nonetheless, the protein profiles in body fluids and their biological pathways following AgNP exposure remain unclear. The objective of this study was to investigate the protein profiles and potential biological pathway in biological fluids in healthy and allergic subjects using proteomic analyses. We established an ovalbumin (OVA)-sensitized allergic mouse model to evaluate the allergenicity of AgNPs in healthy and susceptible mice. The animals inhaled AgNPs that were generated by an evaporation–condensation method. After AgNP inhalation, the BALF and plasma protein profiles were identified in allergic and healthy mice. Finally, we analyzed the biological processes, protein functional classifications, and pathways based on the identified proteins.
Materials and methods
Animals
Female 6-week-old BALB/c mice were obtained from BiOLASCO (Taipei, Taiwan). The mice were maintained at a constant temperature and a relative humidity of 22°C ± 2°C and 55% ± 10%, respectively; a light–dark cycle (12–12 hours) was used throughout the study. The animals were housed in plastic cages and were provided the Lab Diet 5001 (PMI Nutrition International, St Louis, MO, USA) and water ad libitum during acclimatization and pre- and post-exposure. The animal experiments were performed in compliance with the animal and ethics review committee of the Laboratory Animal Centre at the National Taiwan University (Taipei, Taiwan).
AgNP generation and characterization
An evaporation–condensation method was used to generate AgNPs, as described previously.Citation13,Citation14 In brief, silver powder (>99%, Merck KGaA, Darmstadt, Germany) was evaporated at the centre of a 1,100°C tube furnace (model T11-301, SJ Ltd, Taipei, Taiwan), and the generated silver vapor was transported continuously using nitrogen at a flow rate of 6 L/minutes. Because the furnace outlets were equipped with a chilling system, the silver vapor was quenched suddenly and condensed to form AgNPs. In this study, an additional condition section was introduced between the generation system and the exposure chamber to guarantee that the temperature, relative humidity, and composition of the exposed AgNP stream were close to the constant environmental conditions. The oxygen and nitrogen air (5 L/minute at a flow rate of 1:1) was delivered and mixed with the exposed AgNP stream. Next, the exposed AgNP stream was split into two routes for filtration with or without high-efficiency particulate air filters before its introduction into whole-body exposure chambers, which have been described previously.Citation15
A TSI Scanning Mobility Particle Sizer™ Spectrometer 3936 with nano-differential mobility analyzer (DMA) (TSI Incorporated, Shoreview, MN, USA) was used to continuously monitor the size distribution of AgNPs in the exposure chamber throughout the entire exposure period. The flow rates of the aerosol stream and sheath air of the scanning mobility particle sizer were fixed at 0.3 and 3.0 L/minute, respectively. The scanning mobility particle sizer was calibrated with 100 nm National Institute of Standards and Technology-traceable polystyrene latex spheres standard particles before the experiment. The total particle number concentrations, the number-based geometric mean diameter, and the geometric standard deviation were recorded. To physicochemically characterize the generated AgNPs, AgNPs were collected onto 37 mm Teflon substrates (Merck Millipore, Darmstadt, Germany), quarters of which were fixed onto 13 mm aluminum scanning electron microscopy (SEM) stubs.Citation16 The samples were platinum-coated to an average thickness of 10 nm using a sputter coater and were imaged using an Inspect™ SEM (FEI, Hillsboro, OR, USA) at an accelerating voltage of 3 kV and a spot size of 2.5.
Inductively coupled plasma mass spectrometry (ICP-MS; Elan 500, PerkinElmer, Waltham, MA, USA) was used to determine any contamination in the AgNPs. These samples were digested with concentrated nitric acid (Fisher Primar grade, specific gravity 1.48; Thermo Fisher Scientific, Waltham, MA, USA) carried out in a CEM MDS-2000 microwave digestor oven (CEM, SpectraLab Scientific Inc, Markham, ON, Canada), using CEM advanced Teflon-lined composite vessels.Citation17 The pressure was increased to 80 pounds per square inch for approximately 20 minutes, producing a digestion temperature of approximately 180°C. Samples were diluted to 10% nitric acid using deionized (> 18 MΩ) water. Nitric acid blanks were run to detect any contamination during the analysis process. A solution of a certified rock standard (BCR1) was used to check the accuracy of the analyses.
Allergic sensitization, AgNP inhalation, and OVA challenge
To assess the allergenicity of AgNPs, we used endotoxin-free OVA (Sigma-Aldrich, St Louis, MO, USA) as an allergen to induce systemic sensitization. The procedure used to develop allergic airway disease in mice after AgNP exposure was modified from a published method.Citation18 The experimental design is shown in . Mice were divided into four groups (control groups 1 and 2 and allergy groups 3 and 4 [OVA]). Group 1 and 3 mice were exposed to high-efficiency particulate air filtered air (FA; n = 5 each group), whereas Group 2 and 4 were exposed to AgNPs (n = 6 each group). On Day 0, the mice in the allergic groups received an intraperitoneal injection of 50 μg OVA in aluminum hydroxide adjuvant prepared in phosphate-buffered saline (PBS), whereas those in the control groups received the same volume of adjuvant prepared in PBS alone. On Day 8, the mice in the allergic groups received an intraperitoneal injection of 25 μg OVA in aluminum hydroxide adjuvant prepared in PBS, whereas the control groups received the same volume of adjuvant prepared in PBS alone. The mice in the control groups demonstrated a significantly lower level of OVA-specific immunoglobulin (Ig) E than the mice sensitized with OVA (P< 0.001). On Day 16, the allergic and control mice were exposed to 33 nm AgNPs (3.3 ± 0.7 mg/m3; Groups 2 and 4) and FA (Groups 1 and 3) in the whole-body chambers for 7 days (6 hours/day, from Day 16 to 22). The mice were challenged intranasally with 100 μg OVA between Days 23 and 25, and the animals were euthanized on Day 27.
Figure 1 Experimental design for investigating the effects of silver nanoparticles (AgNPs) on the allergic response to ovalbumin (OVA) antigen using proteomic approaches. OVA: OVA in aluminum hydroxide adjuvant prepared in phosphate-buffered saline (PBS); PBS (control): aluminum hydroxide adjuvant prepared in PBS.
Abbreviation: HEPA, high-efficiency particulate air.
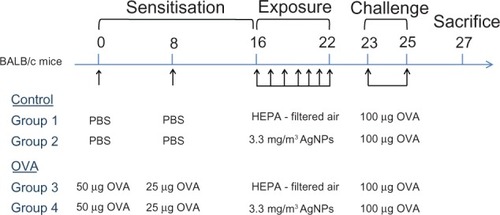
Animal necropsy and sample collection
Animal necropsy, and BALF and blood collections were performed as described previouslyCitation19 Facial vein blood collection was performed before euthanasia. For BALF collection, animals were euthanized with a single intraperitoneal injection of sodium pentobarbitone (200 mg/mL). A single 0.1 mL volume of PBS was used to lavage the lungs. All samples were centrifuged at 1,500 × g for 5 minutes at 4°C and the cell pellet was removed. For proteomic analyses, 0.5 mL of BALF or a plasma sample from each group was pooled together following protein preparation.
Trichloroacetic acid precipitation
The BALF samples were diluted in 120 μL dd-H2O, dried with a SpeedVac concentrator (Thermo Scientific, Asheville, NC, USA), denatured and reduced with 1% sodium dodecyl sulfate/10 mM dithiothreitol (Sigma-Aldrich) at 95°C for 5 minutes and alkylated with 50 mM iodoacetamide (Sigma-Aldrich) in the dark at room temperature for 30 minutes. Trichloroacetic acid (Sigma-Aldrich) was added to a final concentration of 20%. The mixture was incubated for 15 minutes on ice and then centrifuged at 13,000 rpm for 10 minutes. The supernatant was removed. The pellet was washed once with 10% trichloroacetic acid and three times with dd-H2O then it was centrifuged at 13,000 rpm for another 5 minutes. Again the supernatant was removed and then the pellet was digested with trypsin in 25 mM ammonium bicarbonate (Sigma-Aldrich) at 37°C for 18 hours.
Protein digestion
Plasma samples (2.5 μL) were diluted to 1 mL with 50 mM ammonium bicarbonate. Samples were denatured and reduced with 8 M urea/10 mM dithiothreitol at 37°C for 1 hour and alkylated with 50 mM iodoacetamide in the dark at room temperature for 30 minutes. Samples were digested with trypsin in 25 mM ammonium bicarbonate at 37°C for 18 hours and then desalted using C18 columns.
Mass spectrometry and protein identification
The tryptic peptides were analyzed with a Q Exactive mass spectrometer (Thermo Fisher Scientific) coupled with a Dionex UltiMate® 3000 Rapid Separation LC system (Thermo Fisher Scientific). Peptide separation was performed by liquid chromatography with C18 columns (Dionex Acclaim® PepMap™ RSLC, 75 × 150 mm, 2 μm; Thermo Fisher Scientific) with the following conditions: linear gradient from 1% to 40% of Mobile phase B (Mobile phase A: 5.0% acetonitrile/0.1% formic acid Mobile phase B: 95.0% acetonitrile/0.1% formic acid) for 55 minutes and then 40%–90% of Mobile phase B for 5 minutes, with a 90-minute separation time. Full mass spectrometry (MS) scans were performed with ranges of m/z 380–2,000, m/z 380–600, m/z 600–800, m/z 800–1,200, and m/z 1,200–2,000, and the ten most intense ions from MS scans were selected for MS/MS scans. Raw data were processed into peak lists by Proteome Discoverer (v 1.3; Thermo Scientific) for Mascot database search (http://www.matrixscience.com/search form select. html) with the National Centre for Biotechnology Information and UniProt database prediction and literature search. Search parameters included variable modifications for deamidation (NQ), oxidation (M), and fixed modification for carbamidomethyl (C). The maximum mass tolerance was set to 10 ppm for precursor ions and 0.05 Da for fragment ions.
Protein functional analyses
The expressed BALF and plasma proteins (Group 1 vs Group 3 and Group 2 vs Group 4) were analyzed with the Protein ANalysis THrough Evolutionary Relationships (PANTHER) Classification System (http://www.pantherdb.org/) and the Database for Annotation, Visualization and Integrated Discovery (DAVID) gene functional analysis tools (http://david.abcc.ncifcrf.gov/) to better understand the biological context of the identified proteins, their connection to disease pathology, and their participation in physiological pathways.Citation20,Citation21 The UniProt accession database was used to access the 19 overlapping BALF proteins (between Group 1 vs Group 3 and Group 2 vs Group 4) identified in this study. These proteins were uploaded and mapped against the Mus musculus reference dataset to extract and summarize the functional annotation associated with individual genes/proteins or groups of genes/proteins and to identify the gene ontology terms, biological processes, functional classification, and important pathways for each dataset.
Determination of OVA-specific IgE
The plasma OVA-specific IgE levels before (on Day 15) and after (on Day 23) AgNP exposure and OVA challenge were measured using Mouse IgE ELISA Set (BD Biosciences San Jose, CA, USA) in accordance with the manufacturer’s instructions.
Results
Characterization of AgNPs
AgNPs were generated by the evaporation–condensation method and were physicochemically characterized by SEM. Spherical AgNPs with an average size of 33 nm and a total mass of 3.3 ± 0.7 mg/m3 were produced, and the vast majority of these rapidly aggregated to form clusters (). ICP-MS data from filters with and without AgNPs demonstrated the production of Ag-containing NPs. The ICP-MS results also excluded the possibility of impurities or contaminants introduced to the AgNPs during their generation.
Proteomic profile of BALF
To identify the proteins expressed after AgNP exposure, the overlaps in protein profiles between FA and AgNP inhalation in control () and allergy groups were compared (). There were 169 proteins identified in FA-exposed control mice, whereas 220 proteins were determined in AgNP-exposed control mice. There were 114 common proteins in control mice exposed to FA or AgNP. Therefore, 55 and 106 proteins were unique for FA and AgNP, respectively. We further analyzed the 106 unique AgNP-associated proteins in the control BALF samples (). Similarly, 151 and 211 proteins were identified in allergic mice after FA and AgNP exposure, respectively. Because there were 132 common proteins expressed after FA or AgNP exposure, there were 19 and 79 proteins unique to FA and AgNP exposure, respectively. The 79 unique AgNP-associated proteins in the allergic BALF samples were further analyzed ().
Figure 3 Venn diagrams showing the proteins common or unique to silver nanoparticle (AgNP) treatment. (A) Bronchoalveolar lavage fluid (BALF) proteins in control mice, (B) BALF proteins in allergic mice, (C) plasma proteins in control mice, and (D) plasma proteins in allergic mice. The identified AgNP-unique proteins were further analyzed for commonalities in control and allergic mice after AgNP exposure. (E) Proteins commonly expressed in BALF and (F) proteins commonly expressed in plasma.
Abbreviation: FA, filtered air.
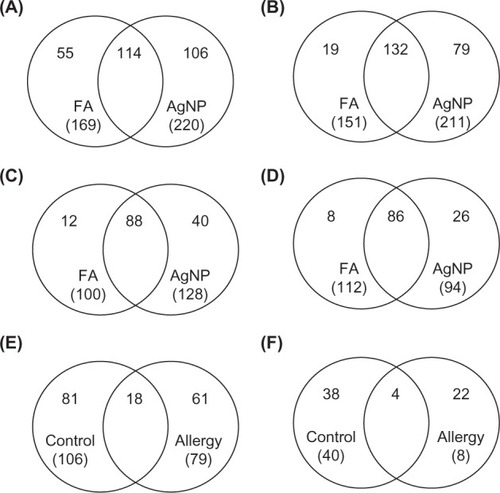
MS-based functional pathway analyses were performed with the BALF proteins in control and allergy groups to understand their biological context. PANTHER analysis was initially used to examine the protein functions in biological processes (). These proteins were associated with 15 biological processes (): cell communication (11.4% for control and 10.2% for allergy), cellular processes (15.7% for control and 14.1% for allergy), transport (5.0%for control and 9.4% for allergy), cellular component organization (4.3% for control and 5.5% for allergy), apoptosis (0.7% for control and 3.1% for allergy), system processes (5.7% for control and 4.7% for allergy), stimulus response (9.3% for control and 7.8% for allergy), developmental processes (8.6% for control and 8.6% for allergy), metabolic processes (18.6% for control and 17.2% for allergy), cell cycle (2.1% for control and 3.9% for allergy), immune system processes (11.4% for control and 10.9% for allergy), cell adhesion (3.6% for control and 4.7% for allergy), reproduction (1.4% for control only), homeostatic processes (0.7% for control only), and the generation of precursor metabolites and energy (1.4% for control only). Metabolic, cellular, and immune system processes and cell communication were the critical biological processes that were modulated in response to AgNP exposure in both control and allergic mice, as determined by BALF analysis.
Figure 4 Biological process in (A) bronchoalveolar lavage fluid (BALF) and (B) plasma obtained using Protein ANalysis THrough Evolutionary Relationships (PANTHER) analysis.
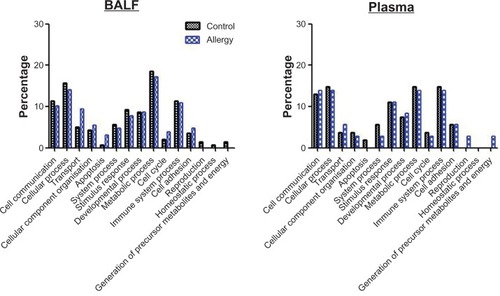
Functional pathways were further investigated using PANTHER analysis (). The AgNP-unique proteins in the control and allergy groups were involved in 12 pathways, including those mediating blood coagulation, integrin signaling, ubiquitin proteasomes, inflammation resulting from chemokine and cytokine signaling, T-cell activation, tricarboxylic acid cycle, dopamine receptor-mediated signaling, plasminogen activating cascade, Fas signaling pathway, cytoskeletal regulation by Rho GTPase, nicotine pharmacodynamics, and nicotinic acetylcholine receptor signaling.
Proteomic profile of plasma
The protein profiles in plasma after FA and AgNP inhalation by control () or allergy groups were compared (). We identified 100 proteins expressed in FA-exposed control mice and 128 in AgNP-exposed control mice; there were 88 proteins common to both control exposures. There were 12 and 40 proteins unique to FA and AgNP, respectively. We used the 40 unique AgNP-associated proteins in the control BALF samples for further analyses (). Additionally, there were 112 and 94 proteins identified in allergic mice after FA or AgNP exposure, respectively, 86 of which were shared by both groups. There were eight and 26 proteins uniquely expressed after FA and AgNP exposure, respectively. The 26 unique AgNP-associated proteins in the allergic BALF samples were further analyzed ().
PANTHER analysis was used to examine the protein functions in biological processes (). These proteins were associated with 14 biological processes (): cell communication (13.0% for control and 13.9% for allergy), cellular processes (14.8% for control and 13.9% for allergy), transport (3.7% for control and 5.6% for allergy), cellular component organization (3.7% for control and 2.8% for allergy), apoptosis (1.9% for control only), system processes (5.6% for control and 2.8% for allergy), stimulus response (11.1% for control and 11.1% for allergy), developmental processes (7.4% for control and 8.3% for allergy), metabolic processes (14.8% for control and 13.9% for allergy), cell cycle (3.7% for control and 2.8% for allergy), immune system processes (14.8% for control and 13.9% for allergy), cell adhesion (5.6% for control and 5.6% for allergy), reproduction (2.8% for allergy only) and the generation of precursor metabolites and energy (2.8% for allergy only). Cellular, metabolic, and immune system processes and stimulus response were important biological processes that were modulated in response to AgNP exposure in both control and allergic mice, as determined by plasma analysis.
Pathway analysis was performed using PANTHER (). Three pathways – blood coagulation, T-cell activation, and plasminogen activating cascade – were determined based on the AgNP-unique proteins in control groups. Notably, blood coagulation was only found in the allergy group.
Functional pathway analyses of BALF and plasma proteins
To determine the common proteins induced by AgNP exposure in control and allergy groups, the unique AgNP-associated proteins in BALF () and serum () were compared. There were 18 overlapping proteins in BALF (): apolipoprotein E, myosin light polypeptide 6, heterogeneous nuclear ribonucleoproteins A2/B1, transitional endoplasmic reticulum ATPase, Ig alpha chain C region, Ig heavy chain V region MOPC 47A, Ig heavy chain V region 441, histone H4, glyoxalase domain-containing protein 4, extracellular superoxide dismutase (Cu-Zn), Ig lambda-1 chain C region, annexin A2, inter-alpha-trypsin inhibitor heavy chain H2, myosin regulatory light polypeptide 9, histone H2A type 1-F, heat shock 70 kDa protein 4, vimentin, and proteasome subunit alpha type-1. Further, there were four overlapping proteins in plasma (): carboxypeptidase N subunit 2, zinc-alpha-2-glycoprotein, vasohibin-1, and interleukin-1 receptor accessory protein.
Table 1 Silver nanoparticle-unique proteins expressed in mice sensitized with phosphate-buffered saline (control) or ovalbumin (allergy)
DAVID analysis revealed functional classifications, including heterotetramer (P = 3.9 × 10−5; 27%), immunoglobulin v region (P = 7.7 × 10−4; 20%), acetylation (P = 9.4 × 10−4; 53%), immunoglobulin (P = 9.5 × 10−4;20%), immunoglobulin domain (P = 4.5 × 10−3; 27%), immunoglobulin c region (P = 8.6 × 10−3; 13%), phosphoprotein (P = 7.9 × 10−2; 60%), duplication (P = 9 × 10−2; 13%) and secretion (P = 9.4 × 10−2; 27%). Further, the overlapping unique AgNP-associated proteins were associated with systemic lupus erythematosus (P = 6.8 × 10−3; 13%). For the plasma samples, analysis of functional classification showed that the overlapping unique AgNP-associated proteins were secreted peptides (P = 5 × 10−4; 100%) and signaling molecules (P = 7.2 × 10−2; 75%). However, there was no pathway identified by these overlapping unique AgNP-associated proteins in plasma.
Determination of OVA-specific IgE
AgNP inhalation induced significant allergic responses in both control and allergy groups, as determined by the plasma levels of OVA-specific IgE in samples collected 2 days after OVA challenge (P< 0.05, ). There was no significant difference in the plasma levels of OVA-specific IgE in control and allergy groups before and after FA exposure. OVA-specific IgE levels were 1.3 times higher in control mice and 14.3 times higher in allergic mice after AgNP exposure.
Discussion
BALF and plasma are important biological fluids containing diverse proteins released by dysfunctional cells and injured tissues. BALF and plasma proteomics are of considerable interest for toxicological and clinical studies, particularly in disease biomarker discovery.Citation22,Citation23 A “protein biomarker” is a protein or peptide that can be objectively and quantitatively measured and evaluated as an indicator of normal or pathological biological processes or exposure to environmental factors. This study used proteomics to determine the allergenicity of AgNPs, generated using the evaporation-condensation method, in allergen provoked murine models. We found 106 and 79 unique AgNP-associated proteins in BALF from control and allergic mice, respectively, after AgNP inhalation. Additionally, 40 and 26 unique AgNP-associated proteins were found in the plasma of control and allergic mice, respectively. The BALF and plasma protein profiles suggested that metabolic, cellular, and immune system processes were modulated in response to AgNP pulmonary exposure. The inhaled AgNPs may regulate immune responses in the lungs of control and allergic mice, as the overlapping unique AgNP-associated proteins in BALF (18 proteins) have also been associated with systemic lupus erythematosus. Further, significant allergy responses were observed after AgNP exposure in control and allergic mice, as determined by OVA-specific IgE.
We investigated the allergenicity of AgNPs in the lungs of mice. The pulmonary toxicity of airborne materials is commonly evaluated by inhalation exposure in animals. These models mimic the natural route of entry into the host and, as such, are the preferred method for the introduction of toxicants into the lungs.Citation24 Toxicokinetics and inhalation studies are important methods used to measure the hazards associated with AgNP exposure. In this study, AgNPs were produced by the evaporation–condensation method, and the allergic responses in mice after AgNP inhalation were determined. The advantage of this procedure is that AgNPs can be generated continuously and stably for long-term inhalation at a range of levels, reflecting the natural exposure route for respirable NPs rather than exposure via intratracheal instillation or pulmonary aspiration. Pure silver powder was evaporated at 1,100°C and the vapor was quenched immediately, resulting in spherical AgNPs. Using SEM and ICP-MS, uncontaminated spherical AgNPs were generated by this method. Our results are in line with those of a previous study,Citation25 suggesting that the AgNPs could associate with physically bonded (ie, agglomerate) and chemically or sinter-bonded (aggregate) structures. The AgNP aggregate, with a size of 33 nm, was easily inhaled deep into the lungs and interacted with the epithelial cells.
Proteomic analyses of biological fluids are very effective for studying pathogen- or other stimulus-induced alterations in hosts and to host responses.Citation26 We investigated the protein profiles in BALF and plasma after AgNP inhalation by healthy (control) and diseased (OVA-sensitized allergy) mouse models. The gas-phase fractionation method was used to increase proteome coverage and the reproducibility of peptide ion selection by direct MS analysis of the peptides produced by proteolytic digestion of unfractionated proteins from the BALF and plasma samples.Citation8,Citation10 Using the gasphase fractionation method, we demonstrated that AgNPs induced expression of 106 unique proteins in healthy mice and 79 unique proteins in OVA-sensitized mice. Protein identifications have been published previously.Citation8,Citation27 Inhaled NPs first interact with the lung epithelial lining fluid, which induces a rapid biological response. PANTHER analysis identified four major biological processes associated with AgNP exposure in the BALF of control and allergic mice: metabolic, cellular, and immune system processes and cell communication. A previous study exposed Sprague Dawley rats in whole-body exposure chambers to environmental cigarette smoke for 28 days. Environmental cigarette smoke stimulated 56 proteins in lung tissues, including those involved in the stress response, protein removal, cell replication, apoptosis, phagocytosis, and the immune response.Citation28 A recent study observed that AgNPs have significant immunological adjuvant effects in BALB/c mice.Citation5 Additionally, Kang et alCitation29 demonstrated that ultrafine ambient particles are adjuvants that enhance allergic inflammation in BALB/c mice. Expression of polymeric immunoglobulin receptor, complement C3, neutrophil gelatinase-associated lipocalin, chitinase 3-like protein 3, chitinase 3-like protein 4, and acidic mammalian chitinase was altered in BALF after particle exposure. To compare the differences in biological processes after pulmonary exposure to AgNPs and ambient particles, in the current study, these six BALF proteins were further analyzed with PANTHER. We found that ambient particle exposure induced proteins involved in reproduction (7.7%), stimulus response (15.4%), immune system processes (15.4%), cellular processes (15.4%), metabolic processes (15.4%), cell communication (7.7%), system processes (7.7%), and transport (15.4%). A similarity was observed in the biological processes induced by AgNP exposure and ambient particle exposure. Taken together, these data suggest that pulmonary exposure to particulate substances such as AgNPs induced immune responses. The particle’s physicochemistry may be a pivotal parameter that determines the severity of immunotoxicology. The physicochemical characteristics of nanoscaled particles contribute to oxidative-inflammatory reactions.Citation30,Citation31 We also elucidated the functions of proteins modulated in response to AgNPs in the lung environment; these functional categories included blood coagulation, activation of the integrin signaling pathway and ubiquitin proteasome pathway; inflammation mediation by chemokines; activation of the cytokine signaling pathway; T-cell activation; activation of the tricarboxylic acid cycle, dopamine receptor-mediated signaling pathway, plasminogen activating cascade, and Fas signaling pathway; cytoskeletal regulation by Rho GTPase; and activation of the nicotine pharmacodynamics pathway and nicotinic acetylcholine receptor signaling pathway. AgNP exposure may alter blood coagulation via platelet activation and thrombus formation,Citation32 oxidative-inflammatory responses,Citation2,Citation3 and innate immune responses.Citation33 The mechanisms underlying AgNP-induced pathologies should be investigated.
Additionally, we identified 40 and 26 AgNP-associated proteins in plasma from healthy and allergen-sensitized mice, respectively. PANTHER analysis indicated that the biological processes involved were similar to those in BALF samples, which were categorized as cellular, metabolic, and immune system processes and stimulus responses. Stimulus responses were the major difference between the BALF and plasma samples. Internal and external stimuli can induce biological responses. Previous studies revealed that the inhalation of AgNPs induced oxidative stress and inflammation.Citation3,Citation7 AgNPs initiate oxidative imbalance in the form of inflammatory responses. Oxidative stress promotes the influx of inflammatory cells (eg, macrophages and neutrophils) to the lungs, leading to a second wave of systemic oxidative stress and inflammation due to the large quantities of free radicals released from activated inflammatory cells.Citation34 Blood coagulation, T-cell activation, and the plasminogen-activating cascade were associated with AgNP inhalation. Comparisons between BALF and plasma proteins suggest that because the lung environment interacts directly with inhaled AgNPs, it is important for initiating several biological pathways. In contrast, plasma samples may represent downstream biological responses to lung stimulation. The association between pulmonary and systemic responses to AgNP exposure requires further investigation.
Alterations in overlapping AgNP-associated proteins in BALF and plasma were also examined. The identified proteins were able to elucidate the common biological processes and pathways in control and allergy subjects. Further, these proteins may be candidates for AgNP-related biomarkers. Our proteomic analysis revealed 18 overlapping proteins in the BALF and four overlapping proteins in plasma. DAVID functional classification software was used to determine the pathway of the proteins identified in BALF and plasma. The proteins found in BALF samples suggest that AgNP exposure is associated with “systemic lupus erythematosus,” which is an autoimmune disease characterized by intense polyclonal production of autoantibodies and circulating immune complexes. Environmental pollutants are risk factors for systemic lupus erythematosus. Cigarette smoke, for example, is positively associated with increased risk for systemic lupus erythematosus.Citation35 Air pollution has been shown to be associated with autoimmune rheumatic disease in humans.Citation36 Systemic lupus erythematosus is characterized by a Th2 immune responseCitation37 and increased IgE production and is also associated with allergy.Citation37 In this study, we observed that OVA-specific IgE levels were significantly increased following AgNP inhalation in control and allergy groups. While these results confirm that immune responses are induced by AgNP exposure, they might also suggest that exposure to AgNPs increases the risk of systemic lupus erythematosus, but this hypothesis requires further investigation.
Conclusion
Our knowledge of the mechanisms underlying asthma is still limited. As far as we are aware, this study is the first to investigate BALF and plasma protein profiles after pulmonary exposure to AgNPs. Comprehensive proteomic analysis using the gas-phase fractionation method and bioinformatics analyses revealed that several proteins were induced in the BALF and plasma of healthy and allergic mice. Our results suggest that these proteins are associated with various essential physiological processes and biological functions. Further, the BALF proteomic results suggest that immunotoxicology is a pivotal response to AgNPs. One limitation of this study is that the levels of specific proteins were not determined, and these data should be generated in future studies. We suggest that BALF proteomic profiling could be used as an effective approach to screen AgNP adjuvant effects. Our findings may fill the gap between epidemiological and clinical research, but the safety of AgNPs in airway therapeutic strategies should be examined carefully before commercial applications are implemented.
Supplementary table
The following tables present the protein profiles in BALF and plasma that do not appear in the main part of the article.
Table S1 Silver nanoparticle-unique proteins expressed in bronchoalveolar lavage fluid in the control mice
Table S2 Silver nanoparticle-unique proteins expressed in bronchoalveolar lavage fluid in the allergy mice
Table S3 Silver nanoparticle-unique proteins expressed in plasma in the control mice
Table S4 Silver nanoparticle-unique proteins expressed in plasma in the allergy mice
Acknowledgments
The authors wish to thank Drs Yuan-Horng Yan, Kuo-Liang Huang, Hui-Hsien Chang, and Ta-Chih Hsiao for their technical assistance with this research.
Disclosure
The authors declare no conflicts of interest in this work.
References
- BennTMWesterhoffPNanoparticle silver released into water from commercially available sock fabricsEnviron Sci Technol200842114133413918589977
- ParkHSKimKHJangSAttenuation of allergic airway inflammation and hyperresponsiveness in a murine model of asthma by silver nanoparticlesInt J Nanomedicine2010550551520957173
- JangSParkJ WChaHRSilver nanoparticles modify VEGF signaling pathway and mucus hypersecretion in allergic airway inflammationInt J Nanomedicine201271329134322457593
- FoldbjergROlesenPHougaardMDangDAHoffmannHJAutrupHPVP-coated silver nanoparticles and silver ions induce reactive oxygen species, apoptosis and necrosis in THP-1 monocytesToxicol Lett2009190215616219607894
- XuYTangHLiuJHWangHLiuYEvaluation of the adjuvant effect of silver nanoparticles both in vitro and in vivoToxicol Lett20132191424823454833
- GhoshMJMSinhaSIn vitro and in vivo genotoxicity of silver nanoparticlesMutat Res20127491–2606922960309
- MeiNZhangYChenYSilver nanoparticle-induced mutations and oxidative stress in mouse lymphoma cellsEnviron Mol Mutagen201253640941922576574
- YuCJWangCLWangCIComprehensive proteome analysis of malignant pleural effusion for lung cancer biomarker discovery by using multidimensional protein identification technologyJ Proteome Res201110104671468221806062
- SchrohlASWürtzSKohnEBanking of biological fluids for studies of disease-associated protein biomarkersMol Cell Proteomics20087102061106618676364
- YiECMarelliMLeeHApproaching complete peroxisome characterization by gas-phase fractionationElectrophoresis200223183205321612298092
- KennedyJYiECUse of gas-phase fractionation to increase protein identifications: application to the peroxisomeMethods Mol Biol200843221722818370021
- BrookRDRajagopalanSPopeCAIIIParticulate matter air pollution and cardiovascular disease: An update to the scientific statement from the American Heart AssociationCirculation2010121212331237820458016
- HoMWuKYCheinHMChenLCChengTJPulmonary toxicity of inhaled nanoscale and fine zinc oxide particles: mass and surface area as an exposure metricInhal Toxicol2011231494795622122307
- SinghYJavierRNEhrmanSHMagnussonMDeppertKApproaches to increasing yield in evaporation/condensation nanoparticle generationJ Aerosol Sci200233913091325
- MaciejczykPZhongMLiQXiongJNadziejkoCChenLCEffects of subchronic exposures to concentrated ambient particles (CAPs) in mice. II. The design of a CAPs exposure system for biometric telemetry monitoringInhal Toxicol2005174–518919715804936
- MorenoTGibbonsWJonesTRichardsRThe geology of ambient aerosols: characterising urban and rural/coastal silicate PM10−2.5 and PM2.5 using high-volume cascade collection and scanning electron microscopyAtmos Environ2003373042654276
- JonesTMorenoTBéruBéKRichardsRThe physicochemical characterisation of microscopic airborne particles in south Wales: a review of the locations and methodologiesSci Total Environ20063601–3435916257436
- PichavantMGoyaSHamelmannEGelfandEWUmetsuDTAnimal models of airway sensitizationCurr Protoc Immunol2007 Chapter 15: Unit 15.18
- LiNWangMBrambleLAThe adjuvant effect of ambient particulate matter is closely reflected by the particulate oxidant potentialEnviron Health Perspect200911771116112319654922
- Huang daWShermanBTTanQDAVID Bioinformatics resources: expanded annotation database and novel algorithms to better extract biology from large gene listsNucleic Acids Res200735Web Server issueW169W17517576678
- SrivastavaRRaySVaibhavVSerum profiling of leptospirosis patients to investigate proteomic alterationsJ Proteomics201276Spec No566822554907
- SulDEvaluation of toxicological monitoring markers using proteomic analysisJ Proteome Res20065102525252617022623
- KennedySThe role of proteomics in toxicology: identification of biomarkers of toxicity by protein expression analysisBiomarkers20027426929012171755
- DriscollKECostaDLHatchGIntratracheal instillation as an exposure technique for the evaluation of respiratory tract toxicity: uses and limitationsToxicol Sci2000551243510788556
- EggersdorferMLKadauDHerrmannHJPratsinisSEAggregate morphology evolution by sintering: number and diameter of primary particlesJ Aerosol Sci20124671923658467
- GharibSANguyenEVLaiYPlampinJDGoodlettDRHallstrandTSInduced sputum proteome in healthy subjects and asthmatic patientsJ Allergy Clin Immunol2011128611761184. e621906793
- TyanYCWuHYLaiWWSuWCLiaoPCProteomic profiling of human pleural effusion using two-dimensional nano liquid chromatography tandem mass spectrometryJ Proteome Res200541274128616083277
- IzzottiABagnascoMCartigliaCChemoprevention of genome, transcriptome, and proteome alterations induced by cigarette smoke in rat lungEur J Cancer200541131864187415953715
- KangXLiNWangMAdjuvant effects of ambient particulate matter monitored by proteomics of bronchoalveolar lavage fluidProteomics20101052053120029843
- ChuangHCChengYLLeiYCChangHHChengTJProtective effects of pulmonary epithelial lining fluid on oxidative stress and DNA single-strand breaks caused by ultrafine carbon black, ferrous sulphate and organic extract of diesel exhaust particlesToxicol Appl Pharmacol2013266332933423261976
- ChuangHCFanCWChenKYChang-ChienGPChanCCVasoactive alteration and inflammation induced by polycyclic aromatic hydrocarbons and trace metals of vehicle exhaust particlesToxicol Lett201221413113622940192
- JunEALimKMKimKSilver nanoparticles enhance thrombus formation through increased platelet aggregation and procoagulant activityNanotoxicology20115215716720822370
- BezemerGFBauerSMOberdörsterGActivation of pulmonary dendritic cells and Th2-type inflammatory responses on instillation of engineered, environmental diesel emission source or ambient air pollutant particles in vivoJ Innate Immun20113215016621099199
- KellyFJOxidative stress: its role in air pollution and adverse health effectsOccup Environ Med200360861261612883027
- KiyoharaCWashioMHoriuchiTCigarette smoking, alcohol consumption, and risk of systemic lupus erythematosus: a case-control study in a Japanese populationJ Rheumatol20123971363137022589266
- BernatskySFournierMPineauCAClarkeAEVinetESmargiassiAAssociations between ambient fine particulate levels and disease activity in patients with systemic lupus erythematosus (SLE)Environ Health Perspect20111191454920870568
- AttaAMSousaC PCarvalhoEMSousa-AttaMLImmunoglobulin E and systemic lupus erythematosusBraz J Med Biol Res200437101497150115448870