Abstract
Glioma is a primary malignant tumor in the central nervous system. In recent years, the treatment of glioma has developed rapidly, but the overall survival of glioma patients has not significantly improved. Due to the presence of the blood–brain barrier and intracranial tumor barrier, many drugs with good effects to cure glioma in vitro cannot be accurately transported to the corresponding lesions. In order to enable anti-tumor drugs to overcome the barriers and target glioma, nanodrug delivery systems have emerged recently. It is gratifying that liposomes, as a multifunctional nanodrug delivery carrier, which can be compatible with hydrophilic and hydrophobic drugs, easily functionalized by various targeted ligands, biodegradable, and hypoimmunogenic in vivo, has become a quality choice to solve the intractable problem of glioma medication. Therefore, we focused on the liposome nanodrug delivery system, and summarized its current research progress in glioma. Hopefully, this review may provide new ideas for the research and development of liposome-based nanomaterials for the clinical treatment of glioma.
Background
Glioma, which originates from glial cells, produces the most common primary malignant tumors in the central nervous system (CNS) and accounts for 40% of all primary brain tumors.Citation1,Citation2 The World Health Organization (WHO) has divided and classified glioma tumors into four grades, where grades 1 and 2 denote low-grade gliomas, and grades 3 and 4 denote high-grade gliomas. More than half of the patients suffer from glioblastoma (GBM), which has the worst prognosis among gliomas and is prone to relapse with the highest degree of malignancy.Citation3 Currently, the standard treatment for GBM is surgical resection and concurrent temozolomide (TMZ) chemotherapy combined with radiotherapy, but the overall therapeutic effect remains unsatisfactory.Citation4 Owing to the invasive growth of glioma cells, it is difficult to completely resect the tumor despite the recent advancements in microsurgical techniques.Citation5 Even after radiotherapy, chemotherapy, tumor-treating fields, target therapy, and other comprehensive treatments, the prognosis of glioma patients remains poor. In addition to tumor characteristics such as heterogeneity, DNA damage repair mechanisms, and glioma cell stemness, the existence of the blood–brain barrier (BBB) is a great obstacle.Citation6
Researchers are constantly trying to develop new agents to treat glioma. However, compared to other therapeutic areas, the progress of agents which can cross the BBB directly to treat glioma is not very optimistic. Therefore, many biological nanomaterials that can deliver therapeutical drugs to the glioma efficiently have become the focus of study.Citation7,Citation8 For biomedical applications, the size of nanomaterials, including natural and synthetic nanoparticles, ranges from a few to thousands of nanometers.Citation9 Ideal nanomaterials for in vivo applications should possess the following advantages: high bioavailability, in vivo degradability, high blood stability, cellular uptake ability, nontoxicity, low cost, ease for mass production, and no inflammatory response, thrombosis, or immunogenicity.Citation10 According to their properties, nanomaterials can be classified as inorganic, polymeric, and lipid nanomaterials.Citation11 Inorganic nanoparticles are mainly made of inorganic materials, such as graphene, carbon nanotubes, gold nanoparticles, silica nanoparticles, and iron oxide nanoparticles.Citation12–18 Many natural and synthetic polymers with good biocompatibility and low toxicity are used to prepare nanocarriers, such as polymer nanoparticles, polymer micelles, and dendrimers, to accurately deliver and improve the efficacy of drugs.Citation19–21
Lipid nanomaterials can be divided into five categories according to their lipid composition: solid lipid nanoparticles (SLNs), nano structured lipid carriers (NLCs), sphingosomes, lipid nanoparticles (LNPs), and liposome.Citation22 All of them can be employed as drug delivery carriers on account of their good biocompatibility. Among these, SLNs have an unexpected gelation tendency, low encapsulation efficiency, and drug leakage.Citation23 Although NLCs have improved drug loss, the toxicities of both are not negligible.Citation24 Compared to the two, LNPs have a high encapsulation rate of drugs, but they are more studied as carriers for RNA (siRNA and mRNA), and they are not easy to make complex modifications.Citation25–27 Therefore, more and more attention has been paid to liposomal nanomaterials in the study of penetrating the BBB to target glioma.
Compared with the above-mentioned lipid nanomaterials, liposomes have more obvious advantages as drug carriers. Liposomal nanocarriers mainly contain drugs in the shell between the hydrophilic and hydrophobic lipid layers, most of which are vacuolar structures. Notably, drugs can be packaged into liposomes to improve their availability regardless of their hydrophilicity.Citation28,Citation29
As carriers, liposomal nanoparticles can deliver drugs to the nidus of the brain tissue without destroying the BBB while maintaining the original drug dose.Citation30 In addition, due to the abnormal tumor microenvironment and different targets of glioma, different ligands have been designed to modify the surfaces of liposomes. These ligands can specifically bind to receptors highly expressed on the BBB or blood–brain tumor barrier (ie, BBTB) to selectively deliver drugs towards the tumor site and achieve active targeting.
To sum up, liposomes are not only compatible with hydrophilic and hydrophobic drugs, but also have low toxicity, high drug encapsulation rate, and can protect and improve drug stability.Citation31,Citation32 Moreover, cationic liposomes can also be used as siRNA carriers.Citation33,Citation34 In particular, liposomes can be modified to better target glioma.Citation35–37 Therefore, liposomal nanoparticles have superior clinical application prospects in the treatment of glioma.Citation38
The BBB is a Tough Obstacle in the Treatment of CNS Diseases
The BBB, with very low permeability, is the barrier between plasma and brain cells formed by the brain capillary walls and glial cells.Citation39 It can be divided into three parts: the barrier between the peripheral blood vessels and the brain tissue formed by the capillary network (); the barrier between the cerebrospinal fluid and the brain tissue in the ventricle area formed by the choroid epithelial cells; and the barrier between the subarachnoid perivascular and cerebrospinal fluid formed by arachnoid epithelial cells.Citation40 The capillary network located on the surface of the brain is the most important part of the BBB and is mainly composed of brain microvascular endothelial cells (BMECs), astrocytes, pericytes, and the basement membrane.Citation41
Figure 1 The blood-brain barrier formed by the capillary network. The capillary network on the outer surface of the brain tissue, which was composed by endothelial cells, pericytes, and peripheral astrocytes. The junction between endothelial cells was tight junction.
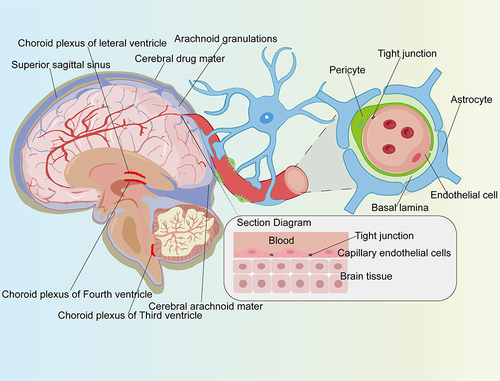
As a particular type of endothelial cells, the structure of BMECs lacks the fenestrations of endothelial cells and contains more mitochondria and fewer plasma membrane vesicles, but also has a very low rate of endocytosis compared with peripheral endothelial cells.Citation42,Citation43 Furthermore, BMECs form a tighter capillary endothelium than peripheral endothelial cells, making the BBB essentially impenetrable to polar molecules.Citation44
Small lipophilic molecules such as oxygen and carbon dioxide can freely diffuse through BMECs. However, special transporters are indispensable for the entry of water-soluble molecules, such as glucose and amino acids into the brain, which are greatly required by the CNS.Citation45 Therefore, the physical barrier, the molecular barrier, and specific transporters for nutrients constitute the main portion of the BBB, which is responsible for regulating the dynamic balance of ion concentrations in the brain, transporting the nutrients needed, preventing potentially harmful molecules from crossing, regulating the cerebral blood volume, exchanging information between the CNS and peripheral tissues, and protecting neuroimmune functions and other normal physiological activities.Citation46
On the one hand, the BBB can protect the brain from many exogenous molecules so that the brain tissue is less or even not damaged by harmful substances circulating in the blood, thus, the basic stability of the brain tissue environment can be preserved, which is of great biological significance for maintaining the normal physiological state of the CNS.Citation47 On the other hand, the BBB restricts or closes the channel by which cytotoxic drugs enter brain tumors.Citation48 Therefore, the BBB not only protects brain tissue but is also a bottleneck in the treatment of intracranial diseases, including glioma.
Current Attempts to Overcome the BBB
To breakthrough this barrier and deliver drugs to the CNS, researchers have explored several approaches. For instance, mechanically, endogenous ligand analogs with high affinity for the CNS and its transporters that also resist clearance by BBB transporter ligands have been developed.Citation49,Citation50 In terms of drug structure, molecular modification of biologics has been attempted to improve BBB permeability, such as PEGylation, esterification, glycosylation, and the addition of fatty acids, amino acids, etc.Citation50–52 Moreover, destruction of the BBB for drug delivery has been exploited, for example, using ultrasound and hypertonic solutions (such as mannitol, urea, and glycerol). Additionally, intranasal drug delivery can bypass the BBB and enter the CNS through four different pathways (): via the olfactory nerve, olfactory mucosal epithelium, blood circulation, and trigeminal nerve.Citation53,Citation54 Studies have shown that intranasal administration of perillyl alcohol can effectively treat recurrent glioma.Citation55 In the development of drug delivery systems, exosomes have been applied to transport small polar molecules and proteins across the BBB.Citation56 Furthermore, nanomaterials, including dendrimers, micelles, liposomes, nanoceramics, and metal and polymer nanoparticles, have been widely developed, allowing drugs to gain access to the site of action through the BBB.Citation57
Figure 2 Several drug delivery methods through blood-brain barrier. Use of ultrasound or hypertonic solution to destroy the blood-brain barrier. (2) Nasal administration (modification of the drug, such as polyethylene glycol esterification, glycosylation or addition of fatty acids, amino acids, etc.). (3) Intracerebral injection. (4) Penetrate the blood-brain barrier with the help of exosomes and nanomaterials.
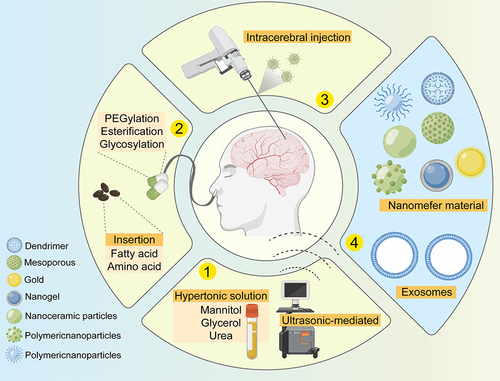
Among these attempts, drugs targeting the CNS across the BBB by means of transporters remained unknown, and endogenous ligand analyses of BBB transporters have not been well studied due to the restrictions posed by enzymes and clearance mechanisms.Citation58 To improve the permeability of the BBB, molecular modification of biological agents has emerged, which often acts through an unexpected mechanism, and is therefore considered an experimental rather than theoretical science approach. For example, the addition of glucose to a peptide greatly enhances the penetration of the peptide through the BBB, but not because the peptide is able to utilize the glucose transporter GLUT1,Citation59 and it is impossible to predict with any accuracy whether such modification would have adverse effects on the brain. When considering drug administration, disruption of the BBB may cause epilepsy, cerebral vasogenic edema, reduced perfusion, tissue ischemia, and other serious consequences.Citation60 Intranasal administration could improve the efficiency of drug delivery, but there are still some problems, such as a small absorption area, an insufficient single dose, the low bioavailability of polar macromolecules (insulin) and macromolecular therapeutic drugs (peptides and proteins), and mucociliary clearance mechanisms in the nasal cavity.Citation53,Citation61 Treatment with viral vectors also faces many difficulties, including production obstacles, high costs, and issues with crossing the BBB. Broadly speaking, direct injection of drugs into the brain has become the most common route of administration, but this causes greater damage.Citation62 At present, however, there is still a long way to go before exosomes can be used in the clinic, and the selection, toxicity, and pharmacokinetics of their donor cells need to be further studied.Citation54
Nanodrug Delivery Systems are Expected to Improve the Plights of Treating Brain Tumors by Drug Therapies
Traditional antitumor drugs have been loaded into nanocarriers by means of physical encapsulation, electrostatic adsorption, and chemical bonding to construct a nanocarrier system by which drug loading and delivery efficiency can be improved through ligand- and receptor-mediated endocytosis.Citation63 Such systems can not only protect drug activity, but also prolong the time of the drug in blood circulation, improve drug targeting, control the drug release speed, and reduce the toxicity and side effects of the drug. The high drug loading capacity, good stability, and degradability that result from these systems could solve the common problem of BBB penetration that many active drug candidates face, or there may be a decrease in the passage of the drug across the BBB to reach the site of action and an inability to maintain an effective blood concentration.Citation64
There are two main advantages of nanoparticle drug delivery systems. First, they can be directly absorbed by endothelial cells on the BBB (which is currently thought to occur via receptor- or adsorption-mediated endocytosis). For example, lipoprotein receptor-related protein (LRP) mediated the introduction of angiopep-2-modified calcium arsenic liposomes (A2-PEG-LP@CAAS) into brain tissue.Citation65 Li and Lu et al reported that a tripeptide RGD-modified liposome (3RGD-Lip) composed of arginyl-glycyl-aspartic acid-coated paclitaxel (PTX-3RGD-Lip) could bind to the integrin αvβ3 receptor and cross the BBB. Among the six different liposomes in this study, the internalization ability of liposomes into BBB were as follows: 3RGD-Lip > 2RGD-Lip > 1RGD-Lip > Lip, while 2×1RGD-Lip ≈ 2RGD-Lip, 3RGD-Lip > 3×1RGD-Lip. The results suggested that 3RGD-Lip showed the best uptake effect in both C6 cells and bEnd.3 cells.Citation36 In another study, a multitargeted redox-sensitive liposome (Lip-SPG) combined with glucose and triphenylphosphonium (TPP) could target GLUT1 (a receptor that is overexpressed on the surface of brain capillary endothelial cells) to enter the brain via endocytosis.Citation66
Second, nanomaterials could reduce drug loss during transport when administered throughout the body. For example, the cyclic RGD (cRGD) peptide-modified liposome gossypol AT-101 showed more than 90% delivery efficiency compared to the control group.Citation67 Another peptide, RGERPPR (RGE), which is a specific ligand of neuropilin-1 (NPR-1), also has the ability to penetrate tumor tissue, significantly enhancing the antitumor effects of modified drugs.Citation68 In Yao Peng’s study, the relative uptake efficiency (Re) by the brain after treatment with Lip-PG liposomes carrying doxorubicin (DOX) was 5.38 times that of free DOX.Citation66
In recent years, researchers have extensively studied the applications of nanoparticle drug delivery systems and have made some breakthroughs. To diagnose glioma, folic acid, and N-(trimethoxysilyl) ethylenediamine triacetic acid (TETT) co-MnO nanoparticles (MnO-TETT-FA) have been developed as a specific MRI contrast agent for imaging.Citation69 For drug transport, drug-carrying gold nanoparticles bound to transferrin ligands targeted transferrin receptors highly expressed on the BBB, resulting in the effective transport of drugs while avoiding the degradation mechanism of the body.Citation70 In other words, the application of nanomaterials to treat glioma has received increasing attention and has shown broad prospects.
Inorganic nanoparticles, including but not limited to metal nanomaterials gold (Au), silver (Ag), copper (Cu), zinc (Zn), titanium (Ti), molybdenum (Mo), gadolinium (Gd), superparamagnetic iron oxide nanomaterials, graphene nanomaterials, mesoporous silica nanoparticles (MSNs), carbon nanomaterials, etc., have been applied in the treatment of glioma.Citation71–82 Nowadays, there have been many studies on inorganic nano-drug delivery platforms (iNDDPs), which are designed to efficiently carry chemotherapeutic drugs to target tumor and have become a potential chemotherapy system in oncology.Citation83 Although metal nanoparticles loaded with chemical drugs could target cancer cells and reduce the side effects of traditional chemotherapy, they are cytotoxic to normal cells in the brain. In addition, chemical modification on the surface of gold nanomaterials may produce varying degrees of immune stimulation.Citation84 In a study by Wang et al, gold nanoparticles (AuNPs) combined with X-ray irradiation significantly reduced the survival rate as well as the migration and invasion ability of glioma cells. However, because of the short observation time, it was still unable to evaluate the long-term antitumor effects and chronic toxicity of AuNPs treatment or combination therapy strategies.Citation85 Many types of polymer nanomaterials, such as polymer nanoparticles, polymer micelles, and dendritic polymers, can be adjusted to obtain the characteristics of passively or actively targeting tumor sites.Citation86 Poly lactic-co-glycolic acid (PLGA) nanoparticles have been utilized to deliver temozolomide (TMZ), which has good encapsulation efficiency and can maintain controllable and continuous drug release for up to 20 days.Citation87 It has been reported that dendritic molecule cationized albumin (dCatAlb) was synthesized by carboxyl activation technology, and the prepared dCatAlb was wrapped on the core of PLGA nanoparticles loaded with doxorubicin (DOX), therefore, a novel hybrid DOX nanoformulation (dCatAlb-pDNP) was developed to release DOX in a controlled manner.Citation88
Compared to iNDDPs and polymer, liposomes have been closely studied because of their similar structure to cell membrane components, non-immunogenic properties, great biocompatibility, and biodegradability in vivo.
Nanomaterial Liposomes and Their Modification
Liposomes are one among the most studied and widely used nanodrug delivery systems. Liposomes are spherical vesicles consisting of single or multiple layers of lipids surrounding a water-based core, usually ranging in size from 20 nm to 1 μm;Citation89 thus, they can be classified as monolayer or multilayer phospholipid bilayer materials. Liposomes have the advantages of protecting drugs from enzyme degradation, nonimmunogenicity, low toxicity, high biocompatibility, flexibility, and biodegradability.Citation90 Most liposomes have a hydrophilic core with hydrophobic phospholipid bilayer structure, which can wrap hydrophilic or hydrophobic drugs, or both. Therefore, liposomal drug delivery systems can increase the solubility of hydrophobic drugs and protect them from metabolism in body fluids.Citation91
Liposomes are small vesicles made of the same substances as cell membranes, namely, phospholipids and cholesterol.Citation92 Based on their lipid bilayer structure, liposomes can be divided into several types, including multivesicular vesicles (MVVs), multilamellar vesicles (MLVs), and unilamellar vesicles (ULVs).Citation93 The size, composition, and charge of liposomes are important factors in drug delivery. Specifically, the size of liposomes plays a crucial role in increasing the targeting efficiency of tumor sites, and in designing effective liposomal antitumor drugs. The appropriate liposome size may be at 100 nm or less, and studies have shown that liposomes with a diameter of ≤ 100 nm may be more conducive to optimizing drug release in vivo, which is mainly reflected in the antitumor activity and toxicity of liposomal antitumor drugs.Citation94
In composition, phospholipids and cholesterol are important lipid carrier materials during the preparation of liposomes and the main components of biological cell membranes and endogenous substances in the body.Citation95 The formation and stability of liposomes are mainly influenced by their phospholipid composition. For instance, 1.2-distearoyl-sn-glycero-3-phosphocholine (DSPC) lipids maintain a perfect spherical structure due to their cylindrical geometry and small-size head groups. However, egg sphingomyelin (DPSM) lipids exhibit conical geometry with larger head groups, which allows liposomes to form micelle structures. As shown by energy analysis of total energy, van der Waals interaction energy, electrostatic interaction energy, etc., DPSM liposomes are more stable than DSPC liposomes.Citation96,Citation97 The phospholipid bilayer has high stability and fluidity, maintains the normal structure of cell membranes, and prevents the accumulation of free cholesterol in the blood vessels.Citation98 Cholesterol can regulate the fluidity of the phospholipid bilayer, reduce membrane permeability, and maintain a certain membrane flexibility to increase the ability of liposomes to cope with changes in external conditions.Citation99 Meanwhile, cholesterol protects phospholipids from oxidation and increases the stability of lipid membranes.Citation100
The fatty acid chains in natural phospholipids contain unsaturated bonds, causing them to be easily oxidized and hydrolyzed in air, which reduces the microviscosity of the liposomal lipid films and increases their fluidity. Therefore, drugs loaded in natural phospholipids leak with ease, and lipid pellets readily accumulate and precipitate.Citation101 Compared with natural phospholipids, synthesized phospholipids are not easily oxidized and hydrolyzed, so liposomes prepared with synthesized phospholipids are more stable. As an important component of liposomes, cholesterol can improve the stability and fluidity of the phospholipid bilayer and affect the particle size and thermodynamic parameters of the liposomes.Citation102 However, cholesterol can induce hypersensitivity and cardiopulmonary side effects associated with the activation of complement effects by regulating serum lipoprotein content, such as pulmonary hypertension.Citation103 In addition, increasing the cholesterol content in cell membranes was shown to significantly decrease the activity of Na+-K+-ATPase and 5’ nucleotide enzymes (approximately 40%), suggesting that cholesterol-rich liposomes may hinder receptor-mediated signal transduction by inhibiting the activity of sodium and potassium pumps on endothelial cells.Citation104 This weakens the binding of liposomes to receptors on BBB endothelial cells to some extent; therefore, it is necessary to modify liposomes by replacing cholesterol with cholesterol analogs.
To solve these problems, some molecules, such as polymers, monosaccharides, polysaccharides, and polypeptides, have been used for modification to construct synthetic liposomes (). The multibranched RGD ligand has a high affinity for the integrin αvβ3 receptor, and this branched structure could increase receptor aggregation, giving 3RGD-Lips a strong ability to target the BBB and glioma cells.Citation36 Another form of RGD peptide (cRGDyK) significantly promoted the transport of procaine in liposomes across the BBB and improved procaine uptake by rat C6 glioma cells.Citation90 Mannose sulfonate liposomes have also been developed for the targeted delivery of chlorogenic acid (CHA) to tumor-associated macrophages (TAMs). The prepared CHA-mannose sulfonate liposomes had an ideal particle size, good stability, and preferential accumulation in tumors through mannose receptor-mediated targeting.Citation105
Figure 3 Simplified Liposome Modification Model. Membranes of liposomes could be added with ligands or other modifiers, such as charges or polypeptides, etc. The modified liposomes protected their carried drugs from degradation and exerted the therapeutic effect better.
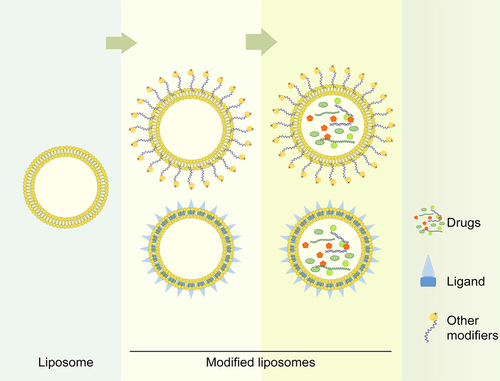
Based on the charged state of the liposome surface, liposomes are classified into cationic, anionic, and neutral types. Compared with neutral liposome, anionic liposomes internalized much less and induce higher cholesterol efflux, while cationic liposomes are the opposite. Therefore, anionic liposome is used to guide the treatment of atherosclerosis.Citation106 As the type more easily ingested by cells, cationic liposomes are commonly used for tumor drug targeting therapy. It seems that researchers prefer to choose cationic liposomes to reach glioma when the carrier is siRNA.Citation107,Citation108 Cationic liposomes more easily cross the BBB via non-specific endocytosis due to their interaction with negative charges on the BBB.Citation109 Cationic liposomes composed of 1.2-dipalmitoyl-sn-glycero-3-phosphocholine (DPPC), cholesterol, and a gemini amphiphile (diastereomeric SS or MESO) are significantly more permeable to the BBB than neutral liposomes (DPPC/cholesterol).Citation110 In addition, many studies have focused on charge- or ionic-based interactions, where the complementary charge between lipids and active pharmaceutical ingredients contributes to their efficient binding, and some “ionizable cationic lipids” are non-toxic and non-immunogenic at doses of 1 mg/kg.Citation111,Citation112,Citation113 These ionizable cationic lipids are also conducive to the efficient release of drugs within tumor cells through the “endosomal escape” mechanism.Citation114,Citation115
The Way of Crossing the BBB and Targeting Glioma
Since SLNs, NLCs, sphingosomes, LNPs, and liposome are all vesicular structures composed of lipids, they have similar abilities to cross the BBB theoretically.Citation116–119 However, there are no reports on the study of sphingosomes to cross the BBB and treat glioma up to now. This may be because they are inefficient and uneconomical to deliver drugs.Citation120 According to the researches, lipid nanoparticles, mainly the other four categories, cross the BBB mainly via passive diffusion and active transportCitation121(). Whereas it seems that no studies have reported the BBB-crossing capabilities between these four lipid-based nanoparticles together.
Figure 4 Liposome loaded drug targeting glioma process. The modified liposomes are transported with the peripheral blood into the brain, and target gliomas through the blood-brain barrier through three ways: (a) With the help of transporters; (b) Transcellular lipophilic; (c) Receptor-mediated transcytosis.
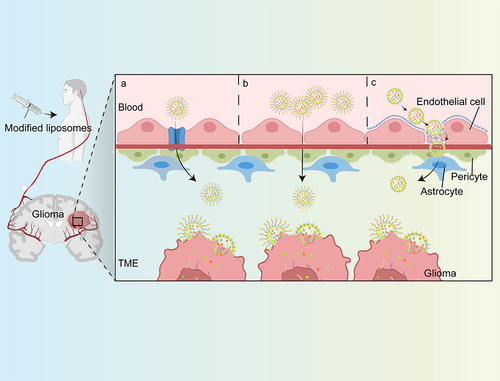
The approach in which liposomes cross the BBB through passive diffusion is also known as a non-specific endocytosis, a process that is mainly due to the size and surface properties of liposomes, such as electrical charge.Citation122,Citation123 Based on the electrostatic interaction between the positive charge on the surface of the liposomes and the negative charge on the surface of the BBB cells, the internalization of BBB endothelial cells is triggered, so that liposomes are taken into the cell. In addition, lipid nanoparticles can also enter cells via active transport. Studies have found that up to 11% of the proteins on the BBB are specific solute carriers (transporters), including different specific protein transporters that transport glucose or amino acids.Citation124 For carrier-mediated transport (CMT), liposomes bind to protein carriers on one side of the endothelial cell, leading to changes in the structure of the carrier protein, and the transfer of liposomes to the other side of the membrane. Researchers also performed various modifications (proteins, peptides, antibodies, etc.) as bioactive ligands on the surface of the liposomes, which can target receptors on endothelial cells of the BBB, including transferrin receptors, folate receptors, etc., so that the liposomes can enter cells via receptor-mediated endocytosis.Citation121 It increases the bioavailability and concentration of drugs in the brain in a controlled manner, providing a novel and effective strategy for targeted drug delivery.Citation125,Citation126
Liu C proved that a dual-modified liposome, named transferrin-cell penetrating peptide-sterically stabilized liposome (TF-CPP-SSL), was initially endocytosed to form clathrin-coated vesicles in tumor cells and could still maintain the integrity of liposomes after crossing the BBB. In the cytoplasm, CPP adsorbs a large amount of H+, and then promotes lipid fusion between cationic CPP-H+ liposome and anionic components in the lysosomal membrane through electron attraction. Fusion pores were subsequently formed, and the liposomes released liposomal substances into cytosol, which provided the opportunity for the drug to enter the nucleus to play pharmacological roles.Citation127
Applications of Liposomes-Drug Delivery System in the Treatment of Glioma
A variety of receptors are highly expressed in the BBB (glioma vessels and/or glioma cells), and liposomes modified with ligands can specifically bind to these receptors to accurately target and penetrate the BBB for anticancer drug deliveryCitation128 (). Specifically, the selective binding modified liposomes could target glioma and improved drug uptake by glioma cells.Citation129 It has been reported that liposomes modified in multiple ways could be employed in combination, where one type of liposome was bound to different ligands, or the same ligand was bound to different liposomes, and these liposomes could carry drugs or encapsulate siRNA.Citation130
Table 1 Application of Modified-Liposomes in Drug Delivery System on Glioma Treatment
Liposomes have long been studied for the treatment of brain tumors. Owing to improvements in liposomal structures, these materials show little or no toxicity in vivo because of their biocompatibility and biodegradability.Citation133 Liposomal system improves stability by interacting with molecules such as PEG or polyethyleneimine (PEI) and promoted intracellular targeting by coupling with peptides, ligands, or specific antibodies.Citation148 Liposomes are very effective carrier systems that can deliver many glioma-targeting drugs when modified with specific ligands.Citation149 The receptors of some natural ligands, such as folic acid and TF, are overexpressed in glioma tissues. A folic acid (FA)-modified lidocaine-carrying liposome (Lid-FA-Lip) significantly enhances the ability of lidocaine to cross the BBB and suppresses the growth of glioma xenografts in mice by targeting the PI3K/Akt pathway.Citation132 The study evaluated the penetration rate of three TfR targeting peptides: T12, B6, and T7. Among all TfR-targeting or non-targeting groups, T7-modified liposomes (T7-LS) showed the highest BBB penetration capacity and brain distribution. When loaded with vincristine (VCR), T7-LS/VCR achieved the best anti-glioma outcomes.Citation135 The anti-proliferative activity against C6s confirmed the strong inhibitory effect of liposomes modified with doxorubicin-loaded TAT.Citation150 Therefore, coupling these natural ligands to the surface of liposomal vesicles will facilitate selective binding between liposomes and gliomas.Citation151
Cen Juan et al designed a new DOX-loaded liposome modified by a small peptide SS31 (LS-DOX), the penetration ratio of LS-DOX through the BBB was at a time-dependent increase, and the cumulative penetration ratio of LS-DOX reached about 17% at 24 h, while that of L-DOX and free DOX group was only 6% and 9%, respectively.Citation152 Similarly, the encapsulation rate and DOX-loading rate of liposome modified by glial fibrous acidic protein (GFAP-DOX-LPs) were 91.84 ± 0.41% and 9.27 ± 0.55%, and the anti-tumor effect of GFAP-DOX-LPs was significantly enhanced compared with the free DOX group.Citation153 In another study, compared to non-PEGylated liposomes (Lip) or DOX combined with carboplatin (DOX + CB), PEGylated liposomes (PEG-Lip) were more efficient in increasing therapeutic effects and decreasing side effects of the drugs, and the survival time of glioblastoma-bearing rats were 39, 35, and 30 days in the PEG-Lip with DOX + CB, Lip with DOX + CB, and free DOX+CB groups, respectively. Meanwhile, the weight loss of mice was 8.7%, 10.5%, and 13%, respectively. The results demonstrated that PEG-Lip with DOX + CB was a promising approach to improve the therapeutic effects and alleviate the side effects in glioma treatment.Citation154 Zhang et al produced a theranostic liposome (QSC-Lip) integrated with superparamagnetic iron oxide nanoparticles (SPIONs) and quantum dots (QDs) and cilengitide (CGT) to target glioma under magnetic targeting (MT) for guiding surgical resection of glioma, besides, CGT was also specifically scattered among glioma after administration of QSC-Lip under MT, leading to an effective inhibition of glioma.Citation155 As indicated above, liposomal nanoparticles modified by ligands and loaded with agents showed more therapeutic benefits in glioma therapy than the drugs alone.
Furthermore, compared with single ligand-modified liposomes or free drugs, dual ligand-modified liposomes produce better targeting and anti-glioma effects in vivo.Citation2,Citation156,Citation157 The specific liposomes (Lip-CTPP), co-modified with p-nopentaacetic acidhydroxybenzoic acid (p-HA) and triphenylphosphonium (TPP), loaded with doxorubicin (DOX) and lonidamine (LND), can greatly restrained glioma cell proliferation, migration, and invasion.Citation158 As reported by Li et al, a special liposome carrying PTX dual-modified by cell penetrating peptide dNP2 and tumor microenvironment-cleavable folic acid (FA) (cFd-Lip/PTX) was produced, which exhibited high BBB permeation because of the overexpression of folate receptor (FR) on BBB and glioma cells, and also the cell penetration ability of dNP2. When cFd-Lip/PTX was exposed to the tumor microenvironment, the acid-sensitive FA on the cFd-Lip/PTX was cleaved at pH 6.8, releasing more dNP2, and then the endocytosis of cFd-Lip/PTX into glioma cells was furtherly accelerated.Citation159 In addition, T7 and DA7R dual peptides-modified liposomes (T7/DA7R-LS) were designed to efficiently co-deliver DOX and VCR to the glioma. And the survival (%) of C6 cells after T7/DA7R-LS crossing the bEnd.3 cells, a type of cerebral microvascular endothelial cells, was 40.05 ± 2.12%, while free DOX + VCR group was 97.88 ± 2.53%.Citation160 In a word, they can target glioma cells with low cytotoxicity and inhibit glioma cell proliferation with high efficiency.
Glioma cells proliferate rapidly with high oxygen consumption and glycolysis rates, consistently maintaining high ROS levels.Citation161 In view of the particularity of the tumor microenvironment, site-dependent drug release triggered by hypoxia, acidity, and redox properties was essential to obtain and maintain a higher level of drug concentration in tumor cells.Citation162 Taking advantage of this difference, stimulus-responsive lipid nanodrug delivery systems have been developed to increase the aggregation of anticancer drugs in glioma lesions, accelerate the drug release rate in tumor cells, improve efficacy, and reduce the side effects of drugs on normal brain tissues and other organs (). Using a hypoxia-responsive linker - nitroimidazoles, the hypoxia-responsive ionizable liposomes could be more likely to be taken up by tumor cells, as nitroimidazoles could convert to aminoimidazole (containing positive charge) under hypoxic conditions.Citation163 In another study, researchers designed a drug delivery liposome named as Lip-TPGS which was modified by glucose and triphenylphosphonium (TPP) as targeting moieties. By adding a linker acid-sensitive amide bond formed by 1-cyclohexene-1,2-dicarboxylic anhydride, redox-sensitive doxorubicin prodrugs (SDOX), and chemotherapeutic sensitizer lonidamine (LND) were released at the acid environment when the Lip-TPGS acrossed the BBB, which significantly inhibited glioma proliferation.Citation164 All these studies demonstrated that once exploiting the properties of glioma microenvironment to design liposomes, the therapeutic effects of tumor would be strongly amplified.
Table 2 Application of Responsive Liposomes in Drug Delivery System on Glioma Treatment
By introducing chemical groups and bonds into the liposome, the controlled release of drugs from the liposomes could be achieved. Liu et al synthesized a malate dehydrogenase lipid molecule known as MLP using a hypoxia-sensitive nitroimidazole group to deliver siPLK1 to glioma cells, which effectively enhanced the uptake of MLP/siPLK1 by cells, and vastly inhibited the growth of glioma cells in vitro and in vivo.Citation171 Amphiphilic diblock copolymers have also been incorporated into phospholipid L-α-phosphatidylcholine (Egg, Chicken) EPC membranes and liposomes to obtain pH-responsive chimeric nanoparticles, which were activated in a low pH environment and promoted the release of drugs in cells, significantly improving the therapeutic effect.Citation166 Since the concentration of GSH in tumor cells is much higher than that of normal tissues, experts have also designed liposomal nanodrug delivery systems that could respond to the redox microenvironment, among which disulfide bonds have been widely used to reduce reaction bonds.Citation174,Citation175 For example, Zhao et al linked the chemotherapy drug Doxorubicin (DOX) to cholesterol with redox-sensitive disulfide bonds, and the disulfide bonds were destroyed in a high-concentration GSH environment, and then DOX was released, based on which liposomes were designed with targeting and glioma-specific stimulation response drug release ability.Citation164 Studies have also been conducted to deliver heat-sensitive liposomes to tumors, using brain heating systems to release contents when the tumor core is heated up to 40°C to treat malignant gliomas.Citation173
In addition to delivering drugs to tumors in response to stimuli, there are also studies using hydrogen peroxide produced by tumor cells for responsive chromogenic analysis to facilitate imaging.Citation176 Chen et al loaded HRP and its substrate 2.2’-azido-bis (3-ethylbenzothiazolin-6-sulfonic acid) into liposomal nanoparticles to obtain an optical nanoprobe named as Lipo@HRP&ABTS. It performs sensitive photoacoustic imaging of gliomas in situ by reacting with endogenous hydrogen peroxide produced by glioma cells.Citation169 In summary, researchers have made full use of the natural structure of liposomes and the inherent characteristics of glioma microenvironment in the process of designing lipid nanomaterials. Some application limitations caused by the physiological characteristics of liposomes and the special location of gliomas are also constantly being optimized.
Limitations and Strategies
However, synthetic liposomes have some limitations. First, they are quickly cleared in blood circulation by macrophages in the reticuloendothelial system.Citation177 Of note, coupling cow serum albumin (cBSA) with liposomes appears to be a promising approach to deliver drugs to the CNS.Citation178 Liposomes bound to cationized bovine serum albumin (cBSA) can be absorbed by the brain via porcine brain capillary endothelial cells (PBCECs) after administration of a lower concentration, avoiding surveillance by the reticuloendothelial system. In addition, when the size of the liposomal vesicle is less than 100 nm, the retention time in circulation can be extended.Citation123
Second, cholesterol-rich animal-derived liposomes may impede receptor-mediated signal transduction by inhibiting the activity of Na+ and K+ pumps on endothelial cells, which weakens the binding of liposomes to BBB endothelial cell receptors to some extent. Moreover, liposomes with immunogenic modifications can cause hypersensitivity in the body.Citation103,Citation104 To solve these problems, researchers modify liposomes by extracting some cholesterol analogues to replace cholesterol, and many researchers reduce cholesterol content by optimizing the ratio of cholesterol and phospholipid, so as to increase the passage rate of liposomes to the blood–brain barrier and improve the safety of liposomes in vivo. For example, Zhu et al reported that Rg3-PTX-LPs could effectively cross the BBB for targeted glioma treatment.Citation141 Phytosterols such as carotenoids and soy-derived sterols have also been shown to improve the physicochemical properties of the phospholipid bilayer.Citation179,Citation180
Third, the drug loading capacity of liposome is limited, while the liposome can be optimized multi-functionally. Objectively speaking, the drugs may be consumed with liposome loss before reaching tumor cells. But some elements responsive to tumor microenvironment such as low pH and hypoxia etc., can be added to liposomes, so as to ensure that drugs release from liposome in glioma cells slowly and constantly, which maintains the tumor killing effects for a longer period of time. For another, to improve the permeation of liposome, the ligand corresponding to the receptor overexpressed on the BBB and glioma cells can be combined with cell penetration peptide. Besides, combined with exogenous targeted therapy such as photodynamic therapy (PDT) or sonodynamic (SDT) would greatly raise therapeutic effects.
Conclusions
Compared with other tumors, glioma tumors are located in the CNS, and their treatment options are limited by the BBB. To overcome this barrier for chemotherapy drug delivery, nanomaterials can be used as carriers to increase drug accumulation at the glioma site by hiding the undesirable physical and chemical properties of the drug and ensuring the integrity of the BBB. Some of the accumulated damage in normal brain tissue after radiotherapy and chemotherapy will also be alleviated by this efficient method of administration.
Liposomes have been widely explored as drug delivery vehicles and are preferred for their unique properties, such as their abilities to hold hydrophilic, lipophilic, and hydrophobic drugs simultaneously and control the release of their contents in a sustained and slow manner. Modifying liposomes with surface ligands can enhance glioma cell targeting by binding to various receptors present or overexpressed on the BBB surface.
There is still much work to be done to make an optimized liposome nanoparticle widely used in clinical practice. First of all, the current standard treatment plan for glioma is still mainly surgery (resection of tumor tissue within the maximum safety range), and adjuvant radiotherapy and chemotherapy therapy. As a drug delivery system, liposomal nanoparticles cannot completely replace surgery to kill tumor cells, but it is not ruled out that liposome nanoparticles may be used as the main treatment to reduce the trauma and complications brought by surgery to patients in the early stage of glioma. For adjuvant surgical treatment, liposomal nanoparticles are more likely to be used before and after surgery according to the current research and the matching scheme of liposomal nanoparticles. Before surgery, liposomal nanoparticles can be loaded with chemotherapy drugs to reduce the tumor as much as possible, so as to reduce the impact of large tumor compression on patients, and then receive surgical treatment in better physical conditions. After surgery, optimized liposomal nanoparticles for precision killing of residual tumor tissue, which may be a better choice than chemotherapy drugs or radiotherapy alone. Second, a rigorous assessment of the toxic effects of the lipid nanoparticles is inevitable Although it is theoretically capable of crossing the blood–brain barrier in maximum concentration, targeting glioma sites to kill tumor cells at specific effective concentrations, with no or minimal damage to normal brain tissue and nerve function. Furthermore, lipid nanodrug delivery system involves multidisciplinary expertise in liposome nanotechnology, brain surgery, neuroscience, pharmacology, imaging, oncology, etc. In order to successfully apply liposomal nanoparticles to the clinical treatment of glioma, it is necessary to form a research team and formulate specific treatment plans for different patients by experts from various disciplines.
Combination therapy is an important therapeutic strategy in glioma treatment. Liposomes with their easy functionalization could not only selectively load chemotherapy drugs to kill glioma cells but also deliver immunomodulator such as CHA to TAMs for immunotherapy of GBM.Citation105 Immunosuppression is a major feature of tumor microenvironment (TME).Citation181,Citation182 Hence, researchers have focused on the liposomal targeting to the major components in tumor immune environment, and tried to make it possible to transform the immunosuppressive TME to an immunosupportive state. To co-deliver adjuvant monophosphoryl lipid A (MPLA) and melanoma antigen peptide TRP2180-188 (SVYDFFVWL) derived from tyrosinase-related protein 2, a nanoplatform of liposomes-coated AuNCs modified by CD11c antibody was fabricated. Obvious increase of DCs maturation and CD8+ T lymphocytes activation in the TME of melanoma were observed after injection, which boosted the anti-tumor immune response.Citation183 Such a strategy could also be use for reference in the future for glioma treatment. In addition, it is known that glioma is a radioresistant tumor due to its good DNA repair activity.Citation184,Citation185 Liu et al produced a hypoxic radiosensitizer-prodrug liposome to deliver the DNA repair inhibitor - Dbait, which increased the sensitivity of glioma cells to X-ray radiotherapy.Citation186 Such studies indicated that the combination between liposome drug delivery system and immunotherapy or radiotherapy is a promising approach to improve glioma efficacy.Citation187–189
In conclusion, liposomes have high clinical application potential as a hot spot in nanomaterial research. In the future, in-depth studies on the characteristics of liposomes and their continuous optimization will provide further possibilities for their application with anti-glioma drugs.
Abbreviations
ABTS, 2.2′-azino-bis(3-ethylbenzothiazoline-6-sulfonic acid); AT, Arginine-conjugated tocopherol lipid; ATO, Arsenic trioxide; BBB, Blood‒brain barrier; BBTB, Blood‒brain tumor barrier; BMECs, Brain microvascular endothelial cells; cBSA, Cow serum albumin; CBZ, Cabazitaxel; CGT, cilengitide; CHA, Chlorogenic acid; CNS, Central nervous system; CTX/ELE, Cabazitaxel/Elemene; DOX, Doxorubicin; DTX, Docetaxel; FA, Folic acid; FTH1, Ferritin heavy chain 1; GBM, Glioblastoma; Gd-DTPA, Gd-diethylendiami-no pentaacetic acid; GRP78, Glucose regulated protein 78; LFR, Lactoferrin receptor; LNPs, lipid nanoparticles; LRP, Lipoprotein receptor-related protein; MLVs, Multilamellar vesicles; MTI-31, mTORC1/mTORC2 inhibitor; MVVs, Multivesicular vesicles; NLCsnAChR, nano structured lipid carriers nicotinic Acetylcholine Receptor; NPR-1, Neuropilin-1; PA, Photoacoustic; PBCECs, Porcine brain capillary endothelial cells; PDMAEMA-b-PLMA, pH-responsive amphiphilic diblock copolymers poly(2-(dimethylamino)ethyl methacrylate)-b-poly(lauryl methacrylate); PEG, Polyethylene glycol; PEI, Polyethyleneimine; pHA, p-Hydroxybenzoic acid; pTRAIL, Plasmid encoding tumor necrosis factor (TNF)-related apoptosis-inducing ligand; PTX, Paclitaxel; PTX-CHO, Paclitaxel-Cholesterol; 3RGD-Lip, Tripeptide RGD-modified liposome; RGD-LP, RGD modified liposomes; RGE, RGERPPR; S1PRs, Sphingosine-1-phosphate receptors; siPLK1, polo-like kinase 1 siRNA; SLNs, solid lipid nanoparticles; SPIONs, Superparamagnetic iron oxide nanoparticles; TAMs, Tumor-associated macrophages; TF, Transferrin; TFR, Transferrin receptor; TME, tumor microenvironment; TMZ, Temozolomide; TPP, Triphenylphosphonium; ULVs, Unilamellar vesicles; VAP, DSDNDTDRDVDADP; VCR, Vincristine.
Consent for Publication
All authors agree to be published.
Author Contributions
All authors made a significant contribution to the work reported, whether that is in the conception, study design, execution, acquisition of data, analysis and interpretation, or in all these areas; took part in drafting, revising, or critically reviewing the article; gave final approval of the version to be published; have agreed on the journal to which the article has been submitted; and agree to be accountable for all aspects of the work.
Disclosure
The authors declare that there are no conflicts of interest in this work.
Acknowledgments
The authors would like to thank Professor Zhanyou Wang and Professor Shenglong Li for kind guidance in the writing of this review.
Additional information
Funding
References
- Young RM, Jamshidi A, Davis G, Sherman JH. Current trends in the surgical management and treatment of adult glioblastoma. Ann Transl Med. 2015;3(9):121.
- Gao J, Lv Q, Li L, et al. Glioma targeting and blood-brain barrier penetration by dual-targeting doxorubincin liposomes. Biomaterials. 2013;34(22): 5628–5639.
- Gritsch S, Batchelor TT, Gonzalez Castro LN. Diagnostic, therapeutic, and prognostic implications of the 2021 World Health Organization classification of tumors of the central nervous system. Cancer. 2021;128(1):47–58.
- Chen R, Smith-Cohn M, Cohen AL, Colman H. Glioma subclassifications and their clinical significance. Neurotherapeutics. 2017;14(2):284–297.
- Dong X. Current Strategies for Brain Drug Delivery. Theranostics. 2018;8(6):1481–1493.
- Wu W, Klockow JL, Zhang M, et al. Glioblastoma multiforme (GBM): an overview of current therapies and mechanisms of resistance. Pharmacol Res. 2021;2021:171.
- Guo Q, Yin M, Fan J, et al. Peroxidase-mimicking TA-VOx nanobranches for enhanced photothermal/chemodynamic therapy of glioma by inhibiting the expression of HSP60. Mater Design. 2022;2022:224.
- Liu D, Dai X, Ye L, et al. Nanotechnology meets glioblastoma multiforme: emerging therapeutic strategies. Wiley Interdisc Rev Nanomed Nanobiotechnol. 2023;15(1):1.
- Wong HL, Wu XY, Bendayan R. Nanotechnological advances for the delivery of CNS therapeutics. Advan Drug Deliv Rev. 2012;64(7):686–700.
- Karathanasis E, Ghaghada KB. Crossing the barrier: treatment of brain tumors using nanochain particles. WIREs Nanomed Nanobiotechnol. 2016;8(5):678–695.
- Liu Z, Che R, Elzatahry AA, Zhao D. Direct imaging Au nanoparticle migration inside mesoporous silica channels. ACS Nano. 2014;8(10):10455–10460.
- Zhao L-X, Gong Z-Q, Zhang Q, et al. Graphdiyne nanoplatforms for photothermal-ferroptosis combination therapy against glioblastoma. J Cont Rel. 2023;359:12–25.
- Nam J, Won N, Bang J, et al. Surface engineering of inorganic nanoparticles for imaging and therapy. Advan Drug Deliv Rev. 2013;65(5):622–648.
- Chung HT, Won JH, Zelenay P. Active and stable carbon nanotube/nanoparticle composite electrocatalyst for oxygen reduction. Nat Commun. 2013;4(1):1.
- Jeong S-Y, Park S-J, Yoon SM, et al. Systemic delivery and preclinical evaluation of Au nanoparticle containing β-lapachone for radiosensitization. J Cont Rel. 2009;139(3):239–245.
- Wang D, Li Z-C, Chen L. Templated synthesis of single-walled carbon nanotube and metal nanoparticle assemblies in solution. J Am Chem Soc. 2006;128(47):15078–15079.
- Liu D, Dai X, Zhang W, et al. Liquid exfoliation of ultrasmall zirconium carbide nanodots as a noninflammatory photothermal agent in the treatment of glioma. Biomaterials. 2023;292:121917.
- Yin M, Chen X, Guo Q, et al. Ultrasmall zirconium carbide nanodots for synergistic photothermal-radiotherapy of glioma. Nanoscale. 2022;14(40):14935–14949.
- Lu L, Chen H, Wang L, et al. A dual receptor targeting- and BBB penetrating- peptide functionalized polyethyleneimine nanocomplex for secretory endostatin gene delivery to malignant glioma. Intern J Nanomed. 2020;15:8875–8892.
- Shi J, Kantoff PW, Wooster R, Farokhzad OC. Cancer nanomedicine: progress, challenges and opportunities. Nat Rev Can. 2016;17(1):20–37.
- Dai X, Liu D, Pan P, Liang G, Wang X, Chen W. Multifunctional two-dimensional Bi2Se3 nanodisks as a non-inflammatory photothermal agent for glioma treatment. J Coll Interf Sci. 2024;661:930–942.
- Khare P, Edgecomb SX, Hamadani CM, Tanner EEL, Manickam D S. Lipid nanoparticle-mediated drug delivery to the brain. Advan Drug Deliv Rev. 2023;197:1.
- Jnaidi R, Almeida AJ, Gonçalves LM. Solid lipid nanoparticles and nanostructured lipid carriers as smart drug delivery systems in the treatment of glioblastoma multiforme. Pharmaceutics. 2020;12(9):1.
- Gaba B, Fazil M, Ali A, Baboota S, Sahni JK, Ali J. Nanostructured lipid (NLCs) carriers as a bioavailability enhancement tool for oral administration. Drug Delivery. 2015;22(6):691–700.
- Strilchuk AW, Hur WS, Batty P, et al. Lipid nanoparticles and siRNA targeting plasminogen provide lasting inhibition of fibrinolysis in mouse and dog models of hemophilia A. Sci Transl Med. 2024;16:735.
- Kang DD, Hou X, Wang L, et al. Engineering LNPs with polysarcosine lipids for mRNA delivery. Bioactive Materials. 2024;37:86–93.
- Khare P, Edgecomb SX, Hamadani CM, Conway JF, Tanner EEL, Manickam D S. Ionic liquid-coated lipid nanoparticles increase siRNA uptake into CNS targets. Nanoscale Adv. 2024;6(7):1853–1873.
- Liu X, Situ A, Kang Y, et al. Irinotecan delivery by lipid-coated mesoporous silica nanoparticles shows improved efficacy and safety over liposomes for pancreatic cancer. ACS Nano. 2016;10(2):2702–2715.
- Chiang Y-T, C-L L. pH-Responsive polymer-liposomes for intracellular drug delivery and tumor extracellular matrix switched-on targeted cancer therapy. Biomaterials. 2014;35(20):5414–5424.
- Shaw TK, Paul P. Recent approaches and success of liposome-based nano drug carriers for the treatment of brain tumor. Current Drug Delivery. 2022;19(8):815–829.
- Fan Y, Marioli M, Zhang K. Analytical characterization of liposomes and other lipid nanoparticles for drug delivery. J Pharm Biomed Anal. 2021;192:113642.
- Peddinti V, Rout B, Agnihotri TG, Gomte SS, Jain A. Functionalized liposomes: an enticing nanocarrier for management of glioma. J Liposome Res. 2024;34(2):349–367.
- Liu X, Madhankumar AB, Slagle-Webb B, Sheehan JM, Surguladze N, Connor JR. Heavy chain ferritin siRNA delivered by cationic liposomes increases sensitivity of cancer cells to chemotherapeutic agents. Cancer Res. 2011;71(6):2240–2249.
- Constantinescu CA, Fuior EV, Rebleanu D, et al. Targeted transfection using pegylated cationic liposomes directed towards P-selectin increases siRNA delivery into activated endothelial cells. Pharmaceutics. 2019;11(1):1.
- Alves A, Correia-da-Silva M, Nunes C, et al. Discovery of a new xanthone against glioma: synthesis and development of (Pro)liposome formulations. Molecules. 2019;24(3):1.
- Li Z, Lu J, Tang B, et al. Triple branched RGD modification on liposomes: a prospective strategy to enhance the glioma targeting efficiency. Bioorg Med Chem. 2022;60:116704.
- X-T L, Tang W, Xie H-J, et al. The efficacy of RGD modified liposomes loaded with vinorelbine plus tetrandrine in treating resistant brain glioma. J Lipos Res. 2019;29(1):21–34.
- Niu W, Xiao Q, Wang X, et al. A biomimetic drug delivery system by integrating grapefruit extracellular vesicles and doxorubicin-loaded heparin-based nanoparticles for glioma therapy. Nano Letters. 2021;21(3):1484–1492.
- Daneman R, Prat A. The blood–brain barrier. Cold Spring Harbor Perspec Biol. 2015;7(1):1.
- Abbott NJ, Rönnbäck L, Hansson E. Astrocyte–endothelial interactions at the blood–brain barrier. Nat Rev Neurosci. 2006;7(1):41–53.
- O’Brown NM, Pfau SJ, Gu C. Bridging barriers: a comparative look at the blood–brain barrier across organisms. Gen Develop. 2018;32:7–8.
- Obermeier B, Daneman R, Ransohoff RM. Development, maintenance and disruption of the blood-brain barrier. Nature Medicine. 2013;19(12):1584–1596.
- Profaci CP, Munji RN, Pulido RS, Daneman R. The blood–brain barrier in health and disease: important unanswered questions. J Experim Med. 2020;217(4):1.
- Chow BW, Gu C. The molecular constituents of the blood–brain barrier. Trend Neurosci. 2015;38(10):598–608.
- Serlin Y, Shelef I, Knyazer B, Friedman A. Anatomy and physiology of the blood–brain barrier. Sem Cell Developm Biol. 2015;38:2–6.
- Andreone BJ, Lacoste B, Gu C. Neuronal and vascular interactions. Annual Rev Neurosci. 2015;38(1):25–46.
- Ogawa K, Kato N, Yoshida M, et al. Focused ultrasound/microbubbles-assisted BBB opening enhances LNP-mediated mRNA delivery to brain. Journal of Controlled Release. 2022;348:34–41.
- Mo F, Pellerino AA-O, Soffietti RA-O, Rudà R. Blood-brain barrier in brain tumors: biology and clinical relevance. Internat J Molec Sci. 2021;22(23):12654.
- Pan W, Banks WA, Fasold MB, Bluth J, Fau - Kastin AJ, Kastin AJ. Transport of brain-derived neurotrophic factor across the blood-brain barrier. Neuropharmacology. 1998;37(12):1553–1561.
- Kim T, Kim HJ, Choi W, et al. Deep brain stimulation by blood-brain-barrier-crossing piezoelectric nanoparticles generating current and nitric oxide under focused ultrasound. Nat Biomed Eng. 2023;7(2):149–163.
- Han D, Wang M, Dong N, et al. Selective homing of brain-derived reconstituted lipid nanoparticles to cerebral ischemic area enables improved ischemic stroke treatment. J Cont Rel. 2024;365:957–968.
- Wu X, Yuan R, Xu Y, et al. Functionalized lipid nanoparticles modulate the blood-brain barrier and eliminate α-synuclein to repair dopamine neurons. Asian J Pharm Sci. 2024;19(2):100904.
- Saaber D, Wollenhaupt S, Fau - Baumann K, Baumann K, Fau - Reichl S, Reichl S. Recent progress in tight junction modulation for improving bioavailability. Expert Opinion on Drug Discovery. 2014;9(4):367–381.
- Kroll RA, Neuwelt EA. Outwitting the blood-brain barrier for therapeutic purposes: osmotic opening and other means. Neurosurgery. 1998;42(5):1083–1099.
- Chen T, da Fonseca C, Schönthal A. Intranasal Perillyl Alcohol for Glioma Therapy: molecular Mechanisms and Clinical Development. Internat J Molec Sci. 2018;19(12):1.
- Ha D, Yang N, Nadithe V. Exosomes as therapeutic drug carriers and delivery vehicles across biological membranes: current perspectives and future challenges. Acta Pharmaceutica Sinica B. 2016;6(4):287–296.
- Cheng Z, Li M, Dey R, Chen Y. Nanomaterials for cancer therapy: current progress and perspectives. J Hematol Oncol. 2021;14(1):1.
- Banks WA. From blood–brain barrier to blood–brain interface: new opportunities for CNS drug delivery. Nature Reviews Drug Discovery. 2016;15(4):275–292.
- Polt R, Dhanasekaran M, Keyari CM. Glycosylated neuropeptides: a new Vista for neuropsychopharmacology? Medicinal Research Reviews. 2005;25(5):557–585.
- Pandit R, Chen L, Götz J. The blood-brain barrier: physiology and strategies for drug delivery. Advan Drug Deliv Rev. 2020;165-166:1–14.
- Dholakia J, Prabhakar B, Shende P. Strategies for the delivery of antidiabetic drugs via intranasal route. Internat J Pharmac. 2021;2021:608.
- Mingozzi F, High KA. Immune responses to AAV vectors: overcoming barriers to successful gene therapy. Blood. 2013;122(1):23–36.
- Di Filippo LD, de Carvalho SG, Duarte JL, et al. A receptor-mediated landscape of druggable and targeted nanomaterials for gliomas. Materials Today Bio. 2023;2023:20.
- Yang J, Shi Z, Liu R, Wu Y, Zhang X. Combined-therapeutic strategies synergistically potentiate glioblastoma multiforme treatment via nanotechnology. Theranostics. 2020;10(7):3223–3239.
- Xu H, Li C, Wei Y, et al. Angiopep-2-modified calcium arsenite-loaded liposomes for targeted and pH-responsive delivery for anti-glioma therapy. Biochem Biophys Res Communic. 2021;551:14–20.
- Peng Y, Lu J, Li R, et al. Glucose and triphenylphosphonium co-modified redox-sensitive liposomes to synergistically treat glioma with doxorubicin and lonidamine. ACS Appl Mater Interf. 2021;13(23):26682–26693.
- Liu H, Zhang R, Zhang D, et al. Cyclic RGD-decorated liposomal gossypol AT-101 targeting for enhanced antitumor effect. Internat J Nanomed. 2022;17:227–244.
- Jia G, Han Y, An Y, et al. NRP-1 targeted and cargo-loaded exosomes facilitate simultaneous imaging and therapy of glioma in vitro and in vivo. Biomaterials. 2018;178:302–316.
- Chen N, Shao C, Qu Y, et al. Folic acid-conjugated MnO Nanoparticles as aT1Contrast agent for magnetic resonance imaging of tiny brain gliomas. ACS Appl Mater Interf. 2014;6(22):19850–19857.
- Clark AJ, Davis ME. Increased brain uptake of targeted nanoparticles by adding an acid-cleavable linkage between transferrin and the nanoparticle core. Proceed Nat Acad Sci. 2015;112(40):12486–12491.
- Sivasoorian SS, Urade R, Chiu -C-C, Wang L-F. Neuropeptide-functionalized gold nanorod enhanced cellular uptake and improved in vitro photothermal killing in LRP1-positive glioma cells. Pharmaceutics. 2022;14(9):1.
- Hatipoğlu A, Baran A, Keskin C, et al. Green synthesis of silver nanoparticles based on the Raphanus sativus leaf aqueous extract and their toxicological/microbiological activities. Environm Sci Pollut Res. 2023;2023:1.
- Tagami T, Imao Y, Ito S, Nakada A, Ozeki T. Simple and effective preparation of nano-pulverized curcumin by femtosecond laser ablation and the cytotoxic effect on C6 rat glioma cells in vitro. Internat J Pharmac. 2014;468(1–2):1.
- Yang K, Han W, Jiang X, et al. Zinc cyclic di-AMP nanoparticles target and suppress tumours via endothelial STING activation and tumour-associated macrophage reinvigoration. Nature Nanotechnol. 2022;17(12):1322–1331.
- Thirumurugan S, Dash P, Liu X, et al. Angiopep-2-decorated titanium-alloy core-shell magnetic nanoparticles for nanotheranostics and medical imaging. Nanoscale. 2022;14(39):14789–14800.
- Masjedi-Arani M, Amiri M, Amiri O, Ahmadi M, Salavati-Niasari M. Glioma cells eradication by photoexcitation of bioengineered molybdenum trioxide nanoparticles synthesized by wet chemical and microwave route: dose dependent photosensitizer bioactivity. Internat J Pharmac. 2020;591:120021.
- Zhang L, Chen D, Zhang J, et al. A novel cholchicine/gadolinium-loading tubulin self-assembly nanocarrier for MR imaging and chemotherapy of glioma. Nanotechnology. 2020;31(25):255601.
- Fleige G, Nolte C, Synowitz M, Seeberger F, Kettenmann H, Zimmer C. Magnetic labeling of activated microglia in experimental gliomas. Neoplasia. 2001;3(6):489–499.
- Yang S, Baeg E, Kim K, et al. Neurodiagnostic and neurotherapeutic potential of graphene nanomaterials. Biosens Bioelect. 2023;2023:115906.
- Wierzbicki M, Sawosz E, Strojny B, Jaworski S, Grodzik M, Chwalibog A. NF-κB-related decrease of glioma angiogenic potential by graphite nanoparticles and graphene oxide nanoplatelets. Scientific Reports. 2018;8(1):1.
- Zhang H, Zhang W, Zhou Y, Jiang Y, Li S. Dual functional mesoporous silicon nanoparticles enhance the radiosensitivity of VPA in glioblastoma. Translat Oncol. 2017;10(2):229–240.
- Golubewa L, Kulahava T, Timoshchenko I, Shuba M, Svirko Y, Kuzhir P. Rapid and delayed effects of single-walled carbon nanotubes in glioma cells. Nanotechnology. 2021;32(50):1.
- Persano F, Batasheva S, Fakhrullina G, Gigli G, Leporatti S, Fakhrullin R. Recent advances in the design of inorganic and nano-clay particles for the treatment of brain disorders. J Mater Chem B. 2021;9(12):2756–2784.
- Hu D, Xia M, Wu L, et al. Challenges and advances for glioma therapy based on inorganic nanoparticles. Materials Today Bio. 2023;2023:20.
- Wang H, Xu Q, Dong X, et al. Gold nanoparticles enhances radiosensitivity in glioma cells by inhibiting TRAF6/NF-κB induced CCL2 expression. Heliyon. 2023;9(3):1.
- Qian C-G, Chen Y-L, Feng P-J, et al. Conjugated polymer nanomaterials for theranostics. Acta Pharmacol Sin. 2017;38(6):764–781.
- Ramalho MJ, Sevin E, Gosselet F, et al. Receptor-mediated PLGA nanoparticles for glioblastoma multiforme treatment. Internat J Pharmac. 2018;545(1–2):1.
- Muniswamy VJ, Raval N, Gondaliya P, Tambe V, Kalia K, Tekade RK. ‘Dendrimer-Cationized-Albumin’ encrusted polymeric nanoparticle improves BBB penetration and anticancer activity of doxorubicin. Internat J Pharmac. 2019;555:77–99.
- Large DE, Abdelmessih RG, Fink EA, Auguste DT. Liposome composition in drug delivery design, synthesis, characterization, and clinical application. Advanc Drug Deliv Rev. 2021;2021:176.
- Li D, Gao J, Yang C, et al. cRGDyK‑modified procaine liposome inhibits the proliferation and motility of glioma cells via the ERK/p38MAPK pathway. Experimental and Therapeutic Medicine. 2021;22(2):1.
- Dos Santos Rodrigues B, Lakkadwala S, Kanekiyo T, Singh J. Development and screening of brain-targeted lipid-based nanoparticles with enhanced cell penetration and gene delivery properties. Int J Nanomedicine. 2019;14:6497–6517.
- Daraee H, Etemadi A, Kouhi M, Alimirzalu S, Akbarzadeh A. Application of liposomes in medicine and drug delivery. Artificial Cells Nanomed Biotechnol. 2014;44(1):381–391.
- Lai W-F, Wong W-T, Rogach AL. Molecular design of layer-by-layer functionalized liposomes for oral drug delivery. ACS Appl Mater Interf. 2020;12(39):43341–43351.
- Nagayasu A, Uchiyama K, Fau - Kiwada H, Kiwada H. The size of liposomes: a factor which affects their targeting efficiency to tumors and therapeutic activity of liposomal antitumor drugs. Advan Drug Deliv Rev. 1999;40(1–2):75–87.
- Sebti T, Amighi K. Preparation and in vitro evaluation of lipidic carriers and fillers for inhalation. Eur J Pharm Biopharm. 2006;63(1):51–58.
- Hashemzadeh H, Javadi H, Darvishi MH. Study of Structural stability and formation mechanisms in DSPC and DPSM liposomes: a coarse-grained molecular dynamics simulation. Scientif Rep. 2020;10(1):1.
- Wang L, Xing H, Guo S, et al. Negatively charged phospholipids doped liposome delivery system for mRNA with high transfection efficiency and low cytotoxicity. Drug Delivery. 2023;30(1):1.
- Nsairat H, Khater D, Sayed U, Odeh F, Al Bawab A, Alshaer W. Liposomes: structure, composition, types, and clinical applications. Heliyon. 2022;8(5):1.
- Boughter CT, Monje-Galvan V, Im W, Klauda JB. Influence of cholesterol on phospholipid bilayer structure and dynamics. J Phys Chem B. 2016;120(45):11761–11772.
- Martinez-Seara H, Róg T, Pasenkiewicz-Gierula M, Vattulainen I, Karttunen M, Reigada R. Interplay of unsaturated phospholipids and cholesterol in membranes: effect of the double-bond position. Biophysical Journal. 2008;95(7):3295–3305.
- Yi X, Gao X, Zhang X, Xia G, Shen X. Preparation of liposomes by glycolipids/phospholipids as wall materials: studies on stability and digestibility. Food Chemistry. 2023;402:134328.
- Canepa E, Bochicchio D, Brosio G, et al. Cholesterol-containing liposomes decorated with au nanoparticles as minimal tunable fusion machinery. Small. 2023;19(23):1.
- Szebeni J, Baranyi L, Fau - Savay S, et al. Role of complement activation in hypersensitivity reactions to doxil and hynic PEG liposomes: experimental and clinical studies. Journal of Liposome Research. 2002;12(1–2):165–172.
- Mayol V, Duran MJ, Gerbi A, et al. Cholesterol and omega-3 fatty acids inhibit Na, K-ATPase activity in human endothelial cells. Atherosclerosis. 1999;142(2):327–333.
- Ye J, Yang Y, Jin J, et al. Targeted delivery of chlorogenic acid by mannosylated liposomes to effectively promote the polarization of TAMs for the treatment of glioblastoma. Bioactive Materials. 2020;5(3):694–708.
- Tang J, Rakshit M, Chua HM, et al. Liposome interaction with macrophages and foam cells for atherosclerosis treatment: effects of size, surface charge and lipid composition. Nanotechnology. 2021;32:50.
- Sun X, Chen Y, Zhao H, et al. Dual-modified cationic liposomes loaded with paclitaxel and survivin siRNA for targeted imaging and therapy of cancer stem cells in brain glioma. Drug Delivery. 2018;25(1):1718–1727.
- Huang J, Dai M, He M, et al. Treatment of ulcerative colitis by cationic liposome delivered NLRP3 siRNA. International Journal of Nanomedicine. 2023;18:4647–4662.
- Wu S, Fu J, Liu D, Chen D, Hu H. The blood-brain barrier cell-targeted gene delivery system to enhance nerve growth factor protein secretion in the brain. ACS Biomater Sci Eng. 2020;6(11):6207–6216.
- Simonis B, Vignone D, Gonzalez Paz O, et al. Transport of cationic liposomes in a human blood brain barrier model: role of the stereochemistry of the gemini amphiphile on liposome biological features. J Coll Interf Sci. 2022;627:283–298.
- Basha G, Novobrantseva TI, Rosin N, et al. Influence of cationic lipid composition on gene silencing properties of lipid nanoparticle formulations of siRNA in antigen-presenting cells. Molecular Therapy. 2011;19(12):2186–2200.
- Semple SC, Akinc A, Chen J, et al. Rational design of cationic lipids for siRNA delivery. Nat Biotechnol. 2010;28(2):172–176.
- Walsh CL, Nguyen J, Tiffany MR, Szoka FC. Synthesis, characterization, and evaluation of ionizable lysine-based lipids for siRNA delivery. Bioconjug Chem. 2013;24(1):36–43.
- Yanez Arteta M, Kjellman T, Bartesaghi S, et al. Successful reprogramming of cellular protein production through mRNA delivered by functionalized lipid nanoparticles. Proceed Nation Acad Sci Unit Stat Am. 2018;115(15):E3351–E3360.
- Zuhorn IS, Bakowsky U, Polushkin E, et al. Nonbilayer phase of lipoplex-membrane mixture determines endosomal escape of genetic cargo and transfection efficiency. Molecular Therapy. 2005;11(5):801–810.
- Satapathy MK, Yen T-L, Jan J-S, et al. Solid Lipid Nanoparticles (SLNs): an advanced drug delivery system targeting brain through BBB. Pharmaceutics. 2021;13(8):1.
- Goli VVN, Tatineni S, Hani U, et al. Pharmacokinetics and pharmacodynamics of a nanostructured lipid carrier co-encapsulating artemether and miRNA for mitigating cerebral malaria. Pharmaceuticals (Basel). 2024;17(4):1.
- Han EL, Padilla MS, Palanki R, et al. Predictive high-throughput platform for dual screening of mRNA lipid nanoparticle blood-brain barrier transfection and crossing. Nano Letters. 2024;24(5):1477–1486.
- Du Q, Liu Y, Fan M, Wei S, Ismail M, Zheng M. PEG length effect of peptide-functional liposome for blood brain barrier (BBB) penetration and brain targeting. J Cont Rel. 2024;2004:1.
- Erdogan S, Ozer AY, Volkan B, Caner B, Bilgili H. Thrombus localization by using streptokinase containing vesicular systems. Drug Deliv. 2006;13(4):303–309.
- Agrawal M, Tripathi DK, Saraf S, et al. Recent advancements in liposomes targeting strategies to cross blood-brain barrier (BBB) for the treatment of Alzheimer’s disease. J Contr Rel. 2017;260:61–77.
- Torchilin VP. Recent advances with liposomes as pharmaceutical carriers. Nature Rev Drug Discov. 2005;4(2):145–160.
- Joshi S, Cooke JRN, Chan DKW, et al. Liposome size and charge optimization for intraarterial delivery to gliomas. Drug Deliv Translat Res. 2016;6(3):225–233.
- Dahlin A, Royall J, Hohmann JG, Wang J. Expression profiling of the solute carrier gene family in the mouse brain. J Pharmacol Experim Therap. 2009;329(2):558–570.
- X-Y L, Zhao Y, Sun M-G, et al. Multifunctional liposomes loaded with paclitaxel and artemether for treatment of invasive brain glioma. Biomaterials. 2014;35(21):5591–5604.
- Liu P, Chen G, Zhang J. A review of liposomes as a drug delivery system: current status of approved products, regulatory environments, and future perspectives. Molecules. 2022;27(4):1.
- Liu C, Liu X-N, Wang G-L, et al. A dual-mediated liposomal drug delivery system targeting the brain: rational construction, integrity evaluation across the blood–brain barrier, and the transporting mechanism to glioma cells. Internat J Nanomed. 2017;12:2407–2425.
- Mojarad-Jabali S, Farshbaf M, Walker PR, et al. An update on actively targeted liposomes in advanced drug delivery to glioma. Internat J Pharmac. 2021;2021:602.
- Ferraris C, Cavalli R, Panciani PP, Battaglia L. Overcoming the blood-brain barrier: successes and challenges in developing nanoparticle-mediated drug delivery systems for the treatment of brain tumours. Int J Nanomedicine. 2020;15:2999–3022.
- Yang ZZ, Li JQ, Wang ZZ, Dong DW, Qi XR. Tumor-targeting dual peptides-modified cationic liposomes for delivery of siRNA and docetaxel to gliomas. Biomaterials. 2014;35(19):5226–5239.
- Li J, Zeng H, You Y, et al. Xie T: active targeting of orthotopic glioma using biomimetic liposomes co-loaded elemene and cabazitaxel modified by transferritin. J Nanobiotechnol. 2021;19(1):1.
- Li D, Yang X, Li B, et al. Lidocaine liposome modified with folic acid suppresses the proliferation and motility of glioma cells via targeting the PI3K/AKT pathway. Experim Therap Med. 2021;22(3):1.
- Wang X, Meng N, Wang S, et al. Non-immunogenic, low-toxicity and effective glioma targeting MTI-31 liposomes. Journal of Controlled Release. 2019;316:381–392.
- Wu S, Lu L, Zhou J, et al. All-stage targeted therapy for glioblastoma based on lipid membrane coated cabazitaxel nanocrystals. J Controll Rel. 2022;345:685–695.
- Mojarad-Jabali S, Farshbaf M, Hemmati S, et al. Comparison of three synthetic transferrin mimetic small peptides to promote the blood–brain barrier penetration of vincristine liposomes for improved glioma targeted therapy. Internat J Pharmac. 2022;613.
- Xin XA-O, Liu W, Zhang ZA, et al. Efficient anti-glioma therapy through the brain-targeted RVG15-modified liposomes loading paclitaxel-cholesterol complex. Internat J Nanomed. 2021;2021:5755–5776.
- Hu Y, Jiang K, Wang D, et al. Core-shell lipoplexes inducing active macropinocytosis promote intranasal delivery of c-Myc siRNA for treatment of glioblastoma. Acta Biomaterialia. 2022;138:478–490.
- Shi D, Mi G, Shen Y, Webster TJ. Glioma-targeted dual functionalized thermosensitive Ferri-liposomes for drug delivery through an in vitro blood–brain barrier. Nanoscale. 2019;11(32):15057–15071.
- Ravula V, Lo Y-L, Wu Y-T, Chang C-W, Patri SV, Wang L-F. Arginine-tocopherol bioconjugated lipid vesicles for selective pTRAIL delivery and subsequent apoptosis induction in glioblastoma cells. Mater Sci Enginee. 2021;2021:126.
- Yuan J, Zeng C, Cao W, et al. Bufalin-Loaded PEGylated liposomes: antitumor efficacy, acute toxicity, and tissue distribution. Nanoscale Research Letters. 2019;14(1):1.
- Zhu Y, Liang J, Gao C, et al. Multifunctional ginsenoside Rg3-based liposomes for glioma targeting therapy. J Cont Rel. 2021;330:641–657.
- Liu Q, Zhou L, Lu R, et al. Biotin and glucose co-modified multi-targeting liposomes for efficient delivery of chemotherapeutics for the treatment of glioma. Bioorg Medic Chem. 2021;2021:29.
- Qi N, Zhang S, Zhou X, et al. Combined integrin αvβ3 and lactoferrin receptor targeted docetaxel liposomes enhance the brain targeting effect and anti-glioma effect. J Nanobiotechnol. 2021;19(1):1.
- Wang X, Zhao Y, Dong S, et al. Cell-penetrating peptide and transferrin co-modified liposomes for targeted therapy of glioma. Molecules. 2019;24(19):1.
- Kang S, Duan W, Zhang S, Chen D, Feng J, Qi N. Muscone/RI7217 co-modified upward messenger DTX liposomes enhanced permeability of blood-brain barrier and targeting glioma. Theranostics. 2020;10(10):4308–4322.
- Fu Q, Zhao Y, Yang Z, et al. Liposomes actively recognizing the glucose transporter GLUT(1) and integrin alpha(v) beta(3) for dual-targeting of glioma. Arch Pharm. 2019;352(2):e1800219.
- Qi N, Duan W, Gao D, et al. “Guide” of muscone modification enhanced brain‐targeting efficacy and anti‐glioma effect of lactoferrin modified DTX liposomes. Bioengin Translat Med. 2022;8(2):1.
- Iwasaki M, Yoshimoto M. Confinement of metalloenzymes in pegylated liposomes to formulate colloidal catalysts for antioxidant cascade. Langmuir. 2021;37(35):10624–10635.
- Renault-Mahieux M, Vieillard V, Seguin J, et al. Co-encapsulation of fisetin and cisplatin into liposomes for glioma therapy: from formulation to cell evaluation. Pharmaceutics. 2021;13(7).1.
- Qin Y, Chen H, Zhang Q, et al. Liposome formulated with TAT-modified cholesterol for improving brain delivery and therapeutic efficacy on brain glioma in animals. Internat J Pharmac. 2011;420(2):304–312.
- Fiandaca MS, Berger MS, Bankiewicz KS. The use of convection-enhanced delivery with liposomal toxins in neurooncology. Toxins. 2011;3(4):369–397.
- Cen J, Dai X, Zhao H, et al. Doxorubicin-loaded liposome with the function of “killing two birds with one stone” against glioma. ACS Appl Mater Interf. 2023;15(40):46697–46709.
- Li Q, Xu J. Research on the inhibitory effect of doxorubicin-loaded liposomes targeting GFAP for glioma cells. Anti-Cancer Agents in Medicinal Chemistry. 2024;24(3):177–184.
- Ghaferi M, Raza A, Koohi M, et al. Impact of PEGylated liposomal doxorubicin and carboplatin combination on glioblastoma. Pharmaceutics. 2022;14(10):1.
- H-L X, Yang -J-J, ZhuGe D-L, et al. Glioma-targeted delivery of a theranostic liposome integrated with quantum dots, superparamagnetic iron oxide, and cilengitide for dual-imaging guiding cancer surgery. Advan Healthcare Mater. 2018;7(9):1.
- Zong T, Mei L, Gao H, et al. Enhanced glioma targeting and penetration by dual-targeting liposome co-modified with T7 and TAT. Journal of Pharmaceutical Sciences. 2014;103(12):3891–3901.
- Qin LI, Wang C-Z, Fan H-J, et al. A dual-targeting liposome conjugated with transferrin and arginine-glycine-aspartic acid peptide for glioma-targeting therapy. Oncology Letters. 2014;8(5):2000–2006.
- Lu J, Li R, Mu B, et al. Multiple targeted doxorubicin-lonidamine liposomes modified with p-hydroxybenzoic acid and triphenylphosphonium to synergistically treat glioma. Europ J Med Chem. 2022;2022:230.
- Li M, Shi K, Tang X, et al. pH-sensitive folic acid and dNP2 peptide dual-modified liposome for enhanced targeted chemotherapy of glioma. Europ J Pharmac Sci. 2018;124:240–248.
- Zhang Y, Zhai M, Chen Z, et al. Dual-modified liposome codelivery of doxorubicin and vincristine improve targeting and therapeutic efficacy of glioma. Drug Delivery. 2017;24(1):1045–1055.
- Garofano L, Migliozzi S, Oh YT, et al. Pathway-based classification of glioblastoma uncovers a mitochondrial subtype with therapeutic vulnerabilities. Nat Cancer. 2021;2(2):141–156.
- Xiao Y, Yu D. Tumor microenvironment as a therapeutic target in cancer. Pharmacol Ther. 2021;221:107753.
- Zhang L, Cao Y, Guo X, et al. Hypoxia-induced ROS aggravate tumor progression through HIF-1α-SERPINE1 signaling in glioblastoma. Journal of Zhejiang University-SCIENCE B. 2023;24(1):32–49.
- Zhao Y, Peng Y, Yang Z, et al. pH-redox responsive cascade-targeted liposomes to intelligently deliver doxorubicin prodrugs and lonidamine for glioma. Europ J Med Chemist. 2022;235:114281.
- Yang C, Yang Z, Wang S, et al. Berberine and folic acid co-modified pH-sensitive cascade-targeted PTX-liposomes coated with Tween 80 for treating glioma. Bioorg Med Chem. 2022;69:116893.
- Naziris N, Pippa N, Sereti E, et al. Chimeric Stimuli-responsive liposomes as nanocarriers for the delivery of the Anti-glioma agent TRAM-34. Internat J Molec Sci. 2021;22(12):1.
- Zhao Y, Ren W, Zhong T, et al. Tumor-specific pH-responsive peptide-modified pH-sensitive liposomes containing doxorubicin for enhancing glioma targeting and anti-tumor activity. J Cont Rel. 2016;222:56–66.
- Pacheco-Torres J, Mukherjee N, Walko M, et al. Image guided drug release from pH-sensitive Ion channel-functionalized stealth liposomes into an in vivo glioblastoma model. Nanomedicine. 2015;11(6):1345–1354.
- Chen Q, Liang C, Sun X, et al. H(2)O(2)-responsive liposomal nanoprobe for photoacoustic inflammation imaging and tumor theranostics via in vivo chromogenic assay. Proc Natl Acad Sci U S A. 2017;114(21):5343–5348.
- Hua L, Wang Z, Zhao L, et al. Hypoxia-responsive lipid-poly-(hypoxic radiosensitized polyprodrug) nanoparticles for glioma chemo- and radiotherapy. Theranostics. 2018;8(18):5088–5105.
- Liu H-M, Zhang Y-F, Xie Y-D, et al. Hypoxia-responsive ionizable liposome delivery siRNA for glioma therapy. Internat J Nanomed. 2017;12:1065–1083.
- Kim C, Guo Y, Velalopoulou A, et al. Closed-loop trans-skull ultrasound hyperthermia leads to improved drug delivery from thermosensitive drugs and promotes changes in vascular transport dynamics in brain tumors. Theranostics. 2021;11(15):7276–7293.
- Aoki H, Kakinuma K, Morita K, et al. Therapeutic efficacy of targeting chemotherapy using local hyperthermia and thermosensitive liposome: evaluation of drug distribution in a rat glioma model. Int J Hyperthermia. 2004;20(6):595–605.
- Torchilin V. Multifunctional and stimuli-sensitive pharmaceutical nanocarriers. Eur J Pharm Biopharm. 2009;71(3):431–444.
- Yang Y, Sun B, Zuo S, et al. Trisulfide bond-mediated doxorubicin dimeric prodrug nanoassemblies with high drug loading, high self-assembly stability, and high tumor selectivity. Sci Adv. 2020;6(45):1.
- Deng Z, Qian Y, Yu Y, et al. Engineering intracellular delivery nanocarriers and nanoreactors from oxidation-responsive polymersomes via synchronized bilayer cross-linking and permeabilizing inside live cells. J Am Chem Soc. 2016;138(33):10452–10466.
- Vieira D, Gamarra L. Getting into the brain: liposome-based strategies for effective drug delivery across the blood–brain barrier. Internat J Nanomed. 2016;11:5381–5414.
- Helm F, Fricker G. Liposomal conjugates for drug delivery to the central nervous system. Pharmaceutics. 2015;7(2):27–42.
- Gallová J, Uhríková D, Kučerka N, et al. Influence of cholesterol and β-sitosterol on the structure of EYPC bilayers. J Memb Biol. 2011;243(1–3):1.
- Sujak A, Strzałka K, Gruszecki WI. Thermotropic phase behaviour of lipid bilayers containing carotenoid pigment canthaxanthin: a differential scanning calorimetry study. Chem Phys Lip. 2007;145(1):1–12.
- Bilotta MT, Antignani A, Fitzgerald DJ. Managing the TME to improve the efficacy of cancer therapy. Front Immunol. 2022;13:954992.
- Zhang F, Liu H, Duan M, et al. Crosstalk among m6A RNA methylation, hypoxia and metabolic reprogramming in TME: from immunosuppressive microenvironment to clinical application. J Hematol Oncol. 2022;15(1):84.
- Liang R, Xie J, Li J, et al. Liposomes-coated gold nanocages with antigens and adjuvants targeted delivery to dendritic cells for enhancing antitumor immune response. Biomaterials. 2017;149:41–50.
- Dutreix M, Cosset JM, Sun JS. Molecular therapy in support to radiotherapy. Mutat Res. 2010;704:1–3.
- Chalmers AJ. Radioresistant glioma stem cells--therapeutic obstacle or promising target? DNA Repair (Amst). 2007;6(9):1391–1394.
- Liu H, Cai Y, Zhang Y, et al. Development of a hypoxic radiosensitizer-prodrug liposome delivery DNA repair inhibitor dbait combination with radiotherapy for glioma therapy. Advanced Healthcare Materials. 2017;6(12):1.
- Zhu X, Li S. Nanomaterials in tumor immunotherapy: new strategies and challenges. Molecular Cancer. 2023;22(1):94.
- Chen Z, Yue Z, Yang K, Li S. Nanomaterials: small particles show huge possibilities for cancer immunotherapy. J Nanobiotechnol. 2022;20(1):484.
- Liu H, Xie Y, Zhang Y, et al. Development of a hypoxia-triggered and hypoxic radiosensitized liposome as a doxorubicin carrier to promote synergetic chemo-/radio-therapy for glioma. Biomaterials. 2017;121:130–143.