Abstract
Purpose
Photothermal ablation is a minimally invasive approach, which typically involves delivery of photothermal sensitizers to targeted tissues. The purpose of our study was to demonstrate that gold nanoparticles are phagocytosed by pancreatic cancer cells, thus permitting magnetic resonance imaging (MRI) of sensitizer delivery and photothermal ablation.
Patients and methods
Iron-oxide core/gold-shell nanoparticles (GoldMag®, 30 nm diameter; Xi’an GoldMag Biotechnology Co, Xi’an, People’s Republic of China) were used. In a 96-well plate, 3 × 104 PANC-1 (human pancreatic cancer cell line) cells were placed. GoldMag (0, 25, or 50 μg/mL) was added to each well and 24 hours allowed for cellular uptake. Samples were then divided into two groups: one treated with photothermal ablation (7.9 W/cm2) for 5 minutes, the other not treated. Photothermal ablation was performed using laser system (BWF5; B&W Tek, Inc, Newark, DE, USA). Intraprocedural temperature changes were measured using a fiber optic temperature probe (FTP-LN2; Photon Control Inc, Burnaby, BC, Canada). After 24 hours, the remaining number of viable cells was counted using trypan blue staining; cell proliferation percentage was calculated based on the total number of viable cells after treatment compared with control. MRI of GoldMag uptake was performed using a 7.0T ClinScan system (Bruker BioSpin, Ettlingen, Germany).
Results
Temperature curves demonstrated that with increased GoldMag uptake, laser irradiation produced higher temperature elevations in the corresponding samples; temperature elevations of 12.89°C, 35.16°C, and 79.51°C were achieved for 0, 25, and 50 μg/mL GoldMag. Without photothermal ablation, the cell proliferation percentage changed from 100% to 71.3% and 47.0% for cells treated with 25 and 50 μg/mL GoldMag. Photothermal ablation of PANC-1 cells demonstrated an effective treatment response, specifically a reduction to only 61%, 21.9%, and 2.3% cell proliferation for cells treated with 0, 25, and 50 μg/mL GoldMag. MRI was able to visualize GoldMag uptake within PANC-1 cells.
Conclusion
Our findings suggest that photothermal ablation may be effective in the treatment of pancreatic cancer. GoldMag nanoparticles could serve as photothermal sensitizers, and MRI is feasible to quantify delivery.
Introduction
Pancreatic cancer is the fourth leading cause of cancer mortality in the United States with an overall 5-year survival rate less than 5%.Citation1,Citation2 Although surgical resection is the sole curative treatment for pancreatic cancer, only 5%–15% of patients with pancreatic cancer are amenable for surgery at the time of diagnosis. Even with an optimal curative resection, the median survival for pancreatic cancer patients is approximately 2 years, with a 5-year survival of 15%–20%, and for those with metastatic disease, survival is shorter than 6 months.Citation3–Citation5 Disruptive approaches are critically needed to impact this deadly disease, and photothermal ablation therapy is one such emerging treatment modality.
Photothermal ablation is a minimally invasive therapy that typically involves the application of photothermal sensitizers.Citation6 These photothermal sensitizers transform the absorbed light (typically laser irradiation) into heat, thus increasing temperatures within the local vicinity of these sensitizing agents. When tissues are heated above 42°C, irreversible cellular damage can occur, eventually resulting in cell death.Citation7 Long-wavelength (800–1300 nm) laser irradiation is necessary for the penetration of tissues during in vivo photothermal ablation procedures; thus, for these applications, the absorption band for nanoparticle sensitizers must be in the near-infrared (NIR) region.Citation8 NIR resonant nanomaterials such as gold nanorods, carbon tubes, and multifunctional magnetic gold nanoshells have each demonstrated the potential to serve as sensitizers during photothermal ablation.Citation6,Citation9,Citation10 Gold nanoparticles permit targeted heat deposition due to their strong absorption cross section and light-to-heat conversion characteristics.Citation11 To enhance tumor-specific heating and thermal deposition, a number of researchers have explored the potential of multifunctional gold nanomaterials; these have been proven as effective photothermal sensitizers for NIR laser irradiation.Citation6,Citation9,Citation12,Citation13
In vivo approaches for noninvasive quantification of gold nanoparticle delivery may be critically needed to optimize these laser irradiation procedures. Super-paramagnetic iron oxide and/or ultra-small superparamagnetic iron oxide nano-particles have been used to label cells, microcapsules, and embolic particles for in vivo tracking with magnetic resonance imaging (MRI) following systemic administration.Citation14–Citation17 Magnetic susceptibility differences between the iron oxide particles and surrounding tissues leads to local magnetic field gradients with proportional signal losses via R2 and R2* relaxation mechanisms. Thus, the quantification of R2 and R2* can be used to estimate intratumoral concentrations and the biodistribution of particles containing iron-oxide nanoparticles.Citation18
In current study, we investigated the use of gold shell-iron oxide core hybrid nanoparticles (Fe3O4/Au) for both imaging and laser irradiation. The aim of our study was to demonstrate that these hybrid nanoparticles are phagocytosed by pancreatic cancer cells, permit quantitative MRI, and elicit a photothermal response with NIR laser irradiation.
Material and methods
Hybrid iron oxide core gold-shell nanoparticles
Hybrid iron oxide core gold-shell nanoparticles (GoldMag®, Xi’an GoldMag Biotechnology Co, Xi’an, People’s Republic of China) were purchased and used as the photosensitizing agent for the current studyCitation19,Citation20 Briefly the composite particle was prepared by the reduction of Au3+ with hydroxylamine in the presence of Fe3O4 particles as seeds. First, the seeds were co-precipitated Fe (II) and Fe (III) ions in alkaline medium, followed by rinsing with water several times until the pH reached 6–7. Second Fe3O4 particles were dispersed in 0.1 mol/L HAuCl4 · 4H2O solution in a beaker, and slowly mixed in a shaking incubator to allow adsorption of Au3+ onto the Fe3O4 surface. NH2OH solution of 80 mmol/L was then added to the system. The mixture was incubated with shaking for 1 hour. The core/shell structure of Fe3O4/Au was formed and then washed with 1 M HCl solution. Finally, the particles were washed with water until the pH was 6.7.
Transmission electron microscopy (TEM) images of these GoldMag nanoparticles were digitized with a high-magnification (FEI Tecnai Spirit G2; FEI Company, Hillsboro, OR, USA). Absorption spectra of the GoldMag nanoparticles (Fe3O4/Au core/shell nanoparticles) were measured and compared with bare Fe3O4 nanoparticles.
Cell culture
A human pancreatic cancer cell line (PANC-1) was purchased from American Type Culture Collection (ATCC, Manassas, VA, USA) and cultured using DMEM (Dulbecco’s Modified Eagle’s Medium; Life Technologies, Carlsbad CA, USA) with 10% fetal bovine serum (Gemini Bio-Products, West Sacramento, CA, USA) and 1% Pen-Strep (Sigma-Aldrich, St Louis, MO, USA). Cells were maintained in culture flasks at 37°C in a humidified atmosphere containing 5% CO2. The viability of the cells was evaluated with trypan blue staining (confirming >90% cell viability when plating the cells).
Temperature measurements
Representative samples with 0 μg/mL, 25 μg/mL, and 50 μg/mL of the GoldMag nanoparticles in 200 μL DMEM culture medium were plated in a 96-well cell culture plate (well bottom area = 0.32 cm2). Samples were irradiated using an NIR laser system (BWF5; B&W Tek, Inc, Newark, DE, USA) with diode fiber laser and continuous wave at wavelength of 808 nm; four separate samples for each nanoparticle concentration were irradiated with power densities = 3.2 W/cm2 (1.0 W), 4.7 W/cm2 (1.5 W), 6.3 W/cm2 (2.0 W), and 7.9 W/cm2 (2.5 W), respectively. The intraprocedural temperature changes were recorded using a fiber optic temperature probe (FTP-LN2; Photon Control Inc, Burnaby, BC, Canada) with measurements recorded at 15-second intervals. The fiber probe was vertically placed in the center of each sample, approximately 3–5 mm deep inside the culture media. Baseline temperature was recorded for first 30 seconds before application of the NIR laser irradiation; each sample was then irradiated for 5 minutes and temperature curve plotted.
Cellular uptake of nanoparticles
TEM (FEI Tecnai Spirit G2) was performed to evaluate the cellular uptake of nanoparticles. Three samples of PANC-1 pancreatic cancer cells were treated with nanoparticles at concentrations of 0, 25, and 50 μg/mL, respectively. After allowing 24 hours incubation in complete culture media at 37°C, the cells were washed three times with phosphate-buffered saline to remove free particles in the medium and rapidly placed into glutaraldehyde and fixed for at least 1 hour at room temperature. The cell samples were then placed in osmium tetroxide, embedded in agar, and processed. The samples were dehydrated in graded concentrations of ethanol and propylene oxide, and embedded in Spurr’s plastic. Semi-thin (5 μm) sections were cut from blocks, and the blocks were selected for thinning with thin sections (1.5 μm) cut and placed on copper grids. Each sample was digitized to visualize the subcellular location of the nanoparticles within the PANC-1 cells.
MRI after nanoparticle uptake
To evaluate the MRI properties of these hybrid nanoparticles macroscopically after cellular uptake, first we constructed three phantoms, each containing 10 × 106 PANC-1 pancreatic cancer cells. These cells were exposed to 0, 25, and 50 μg/mL dose of nanoparticles for 24 hours, respectively. After washing the cells three times using phosphate-buffered saline, the pancreatic cancer cells were gently mixed with 10 mL of 0.8% agarose and placed within 15 mL centrifuge tubes. MRI studies were performed using a 7.0T ClinScan MRI scanner (Bruker BioSpin, Ettlingen, Germany) with Siemens gradient system/pulse sequences and a surface coil. Quantitative R2 measurements were performed using a Carr-Purcell-Meiboom-Gill sequence with the following parameters: repetition time (TR) = 800 ms, echo time (TE) = 11.5, 23, 34.5, and 46 ms, slice thickness = 0.9 mm, 10 slices, field of view (FOV) = 20 mm × 35 mm, matrix = 114 × 192, and bandwidth = 150 Hz/pixel. R2* was measured using a multiple gradient-recalled echo sequence with the following parameters: TR = 700 ms, TE = 2.47, 6.66, 9.65, and 13.24 ms, slice thickness = 0.9 mm, 10 slices, FOV = 20 mm × 35 mm, matrix = 114 × 192, and bandwidth = 500 Hz/pixel. MRI post-processing was performed offline using Matlab software (The Math Works Inc, Natick, MA, USA). R2 and R2* maps were calculated on a voxel-wise basis employing the nonlinear Levenberg-Marquardt algorithm to fit a mono-exponential function S(TEi) = S(0) · exp(−R2* · TEi). A region of interest (ROI) was manually drawn within each resulting R2 and R2* map to record the mean R2 and R2* values within these ROI for each slice within each phantom.
To demonstrate the potential to visualize single cells after nanoparticle uptake, high-resolution three-dimensional multiple gradient-recalled echo scans were performed with the following parameters: TR = 80 ms, TE = 3.22, 6.81, 10.4, and 13.99 ms, slice thickness = 0.15 mm, FOV = 29 mm × 29 mm, matrix = 384 × 384, and bandwidth = 305 Hz/pixel. PANC-1 pancreatic cancer cells treated with 25 μg/mL nanoparticles for 24 hours were prepared in an MRI compatible dish (diameter = 1 inch). After placing these PANC-1 cells at the bottom of the dish, a thin film of agarose was added atop the cells. MRI images were collected using a surface coil with the diameter of 3 cm.
Photothermal ablation
In a 96-well plate, 3 × 104 PANC-1 cells were placed in each well and exposed to a 0, 25, or 50 μg/mL dose of nanoparticles. After allowing 24 hours for cellular uptake, the samples exposed to these three different nanoparticle concentrations were divided into two groups, one exposed to laser irradiation at a power density of 7.9 W/cm2 (2.5 W/well) for 5 minutes, the other group left untreated thus serving as controls. After exposure, the cells were returned to a 37°C incubator for another 24 hours before cell viability tests. These tests were performed in triplicate.
Next, we investigated the efficacy of this photothermal approach when using different power densities during irradiation. Twenty-four hours after PANC-1 cells were exposed to a 25 μg/mL solution of nanoparticles, the cells were irradiated with power densities of 3.2 W/cm2 (1.0 W), 4.7 W/cm2 (1.5 W), 6.3 W/cm2 (2.0 W), or 7.9 W/cm2 (2.5 W), respectively, for 5 minutes. After these NIR laser procedures, the PANC-1 cells were incubated for another 24 hours before cell viability tests.
To evaluate the cell proliferation percentage for each of the aforementioned samples, cells were stained using trypan blue, and the total viable cells were counted three times using an automated cell counter (Countess; Invitrogen, San Diego, CA, USA). The cell proliferation percentage was calculated based on the total viable PANC-1 cells after treatment compared with the control group. Each sample was counted in triplicate.
Statistical analysis
All statistics were performed using the SPSS statistical software package (SPSS, Chicago, IL, USA), version 17. R2 and R2* values for three groups (control, 25 μg/mL, and 50 μg/mL) were compared by one-way analysis of variance test followed by post hoc tests. Paired t-test was performed to compare the cell proliferation between non-ablated cells (GoldMag treated without laser irradiation) and ablated cells (GoldMag treated with laser irradiation) for each GoldMag concentration. Test was considered statistically significant with a P-value < 0.05.
Results
Heating properties of hybrid nanoparticles
Representative TEM image for GoldMag nanoparticles is shown in , and absorption spectra of GoldMag nanoparticles (Fe3O4/Au core/shell nanoparticles) and bare Fe3O4 nanoparticles is shown in . A surface plasmon resonance band was observed at 590 nm for the GoldMag nanoparticles. The surface plasmon resonance band for the Fe3O4/Au core/shell bimetallic nanoparticles showed a red-shift and broadening of the peak, which is commonly observed in other Au bimetallic systems.Citation21,Citation22 When we utilized an 808 nm laser, a corresponding absorption value at 808 nm for the Fe3O4/Au core/shell nanoparticles was directly converted to heat energy that resulted in our heating profiles (). However, bare Fe3O4 nanoparticles did not show absorption peaks around 808 nm, and no heating resulted following 808 nm laser irradiation.
Figure 1 Transmission electron microscopy image of GoldMag nanoparticles (A), and ultraviolet-visible spectra of GoldMag (Fe3O4/Au core/shell nanoparticles) and Fe3O4 in water (arrow) a wavelength of the laser (B).
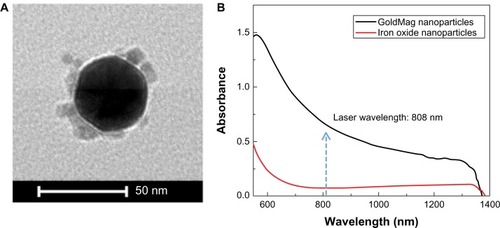
Figure 2 Photothermal response of 0 (A), 25 (B), and 50 (C) μg/mL nanoparticle samples at 3.2, 4.7, 6.3, and 7.9 W/cm2 laser irradiation power densities.
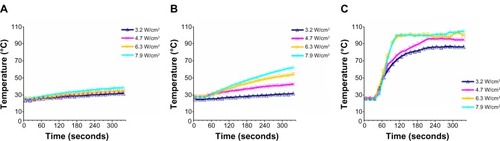
For identical laser irradiation power densities of 7.9 W/cm2, samples containing nanoparticles achieved significantly higher temperatures compared with those samples without the nanoparticles. With an increased concentration of nanoparticles, laser irradiation produced increasingly higher temperature elevations to 13°C, 35°C, and 80°C, respectively, at concentrations of 0, 25, and 50 μg/mL nanoparticles. For an identical nanoparticle concentration of 25 μg/mL, increasing irradiation power density increased the magnitude of the resulting temperature elevations from 7°C at a power density of 3.2 W/cm2 to 14°C, 26°C, and 35°C for power densities of 4.7, 6.3, and 7.9 W/cm2, respectively. Dynamic temperature curves during laser irradiation for each concentration and power density are shown in .
Cellular uptake
TEM images showing cellular uptake of nanoparticles after a 24-hour exposure period are shown in . These TEM images demonstrated that the particles were phagocytosed into the pancreatic cancer cells (arrow). Nanoparticle uptake increased within the 50 μg/mL exposure group (more nanoparticles observed within 50 μg/mL exposure group sample compared with both 25 μg/mL exposure group and controls).
MRI measurements
Voxel-wise R2 and R2* maps for cell suspension phantoms are shown in . Mean R2 and R2* for these cell suspension phantoms increased significantly for those samples exposed to a higher concentration of nanoparticles (P = 0.000 for R2, and P = 0.000 for R2*). A difference in mean R2 and R2* values were found between each of the three phantoms. For control sample, mean R2 ± standard deviation (SD) = 4.77 ± 1.02 (ms−1), mean R2* ± SD = 9.71 ± 0.06 (ms−1); for those cells exposed to 25 μg/mL nanoparticle dose, mean R2 ± SD = 7.68 ± 1.75 (ms−1), mean R2* ± SD = 104.25 ± 1.83 (ms−1); and for cells exposed to 50 μg/mL nanoparticle dose, mean R2 ± SD = 13.03 ± 0.64 (ms−1), mean R2* ± SD = 200.86 ± 4.75 (ms−1) ().
Figure 4 R2 and R2* measurements within PANC-1 cell suspensions after 24-hour exposures to nanoparticles at concentrations of 0, 25, and 50 μ g/mL (each cell suspension consisting of 10 × 106 PANC-1 cells in 10 mL 0.8% agarose) (A). Mean R2 (B) and R2* values (C) ± standard deviation for each corresponding cell suspension.
Note: R2* = R2 + R2.
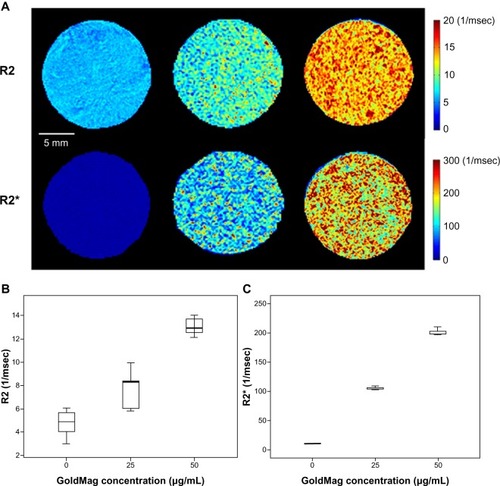
High-resolution T2*-weighted images permitted depiction of individual PANC-1 cell positions after uptake of the iron oxide core gold-shell nanoparticles (after 24 hour exposure period). A representative T2*-weighted image is shown in along with corresponding microscopy confirmation image.
Photothermal response
Representative microscopy images showing the PANC-1 cells exposed to different concentrations of nanoparticles are shown in . The top row shows the PANC-1 cells exposed to nanoparticles at concentrations of 0, 25, and 50 μg/mL without laser irradiation; without laser irradiation, cell proliferation percentages changed from 100% to 71.3% and 47.0% with exposures to 0, 25, and 50 μg/mL nanoparticle doses, respectively. The bottom row of images shows the pancreatic cancer cell samples treated with gold nanoparticles (0, 25, and 50 μg/mL, respectively) and laser irradiation at a power density of 7.9 W/cm2; with laser irradiation, the PANC-1 cells showed significantly decreased cell proliferation (compared with corresponding samples without irradiation) to 61%, 21.9%, and 2.3%, respectively. The latter results for nanoparticle-exposed PANC-1 cells both with and without laser irradiation are summarized in . Difference was found between non-ablated samples and ablated samples in cell proliferation (P = 0.000 for each GoldMag concentration).
Figure 6 PANC-1 cells exposed to 0, 25, and 50 μg/mL dose of nanoparticles, respectively, without laser irradiation (A–C) and with laser irradiation at 7.9 W/cm2 power density (D–F).
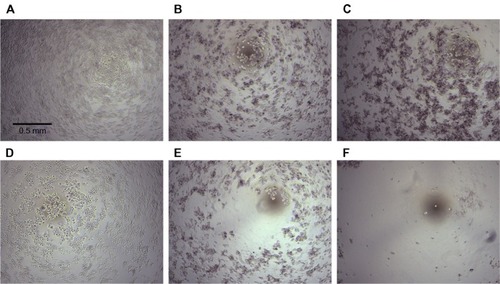
Figure 7 Cell proliferation percentage for PANC-1 cells exposed to different concentrations of nanoparticles with (red) and without (grid) laser irradiation (A); cell proliferation percentage for PANC-1 cells exposed to 25 μg/mL nanoparticle dose and increasing power densities during NIR laser irradiation (B).
Abbreviation: NIR, near-infrared.
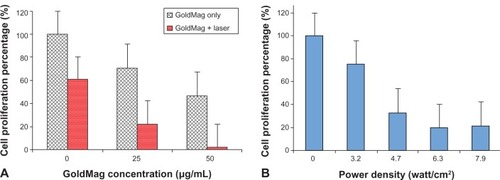
For PANC-1 cells treated with 25 μg/mL nanoparticles, the photothermal response increased with increasing power density. PANC-1 cell proliferation percentages decreased from 100% to 75.6%, 32.9%, 20%, and 21.9% as power density increased from 3.2 to 4.7, 6.3, and 7.9 W/cm2, respectively ().
Discussion
These studies demonstrated the efficacy of using iron oxide core gold-shell nanoparticles as potent MRI-visible photosensitizers during laser irradiation of human pancreatic cancer cells. Previous studies have also shown that other types of nanoparticles can also perform well for these types of applications.Citation23 The salient combination of iron oxide core gold-shell nanoparticles with the local application of NIR laser irradiation offers a promising approach for photothermal ablation therapy in the setting of pancreatic cancer. PANC-1 cell proliferation significantly decreased with exposure to increasing concentrations of the nanoparticles and increasing applied power densities. MRI studies demonstrated quantitative increases in both R2 and R2* with increasing nanoparticle uptake along with the ability to depict individual PANC-1 pancreatic cancer cells after particle phagocytosis.
Heating curves collected during NIR irradiation demonstrated that temperature increased relatively slowly from 8°C to 13°C for those PANC-1 cells that were not exposed to the iron oxide core gold-shell nanoparticles. Upon 24-hour exposure to these particles, temperature changes during irradiation increased dramatically: 7°C, 14°C, 26°C, and 35°C increases for power densities of 3.2, 4.7, 6.3, and 7.9 W/cm2, respectively, in 25 μg/mL exposure group; 60°C, 68°C, 75°C, and 80°C increases for the same power densities in 50 μg/mL exposure group. These hybrid nanoparticles significantly enhanced photothermal response during these NIR laser irradiation procedures. Similar observations have been reported for a number of prior studies using gold nanomaterials as photothermal sensitizers for ablation of human prostate cancer cells, murine renal carcinoma cells, and MDA-MB-435 cells.Citation10,Citation24
Current clinically used thermal ablation technologies include radiofrequency ablation,Citation25–Citation27 microwave coagulation therapy,Citation28 and cryoablation therapy.Citation29 These thermal ablation approaches have a common limitation, nonspecific heating of targeted tissues, and potential injury to surrounding healthy tissues Photothermal ablation approaches alternatively may permit improved tumor-specific heating with nanomaterials serving to photosensitize the targeted tissues. During the current study, iron oxide core gold-shell nanoparticles were readily phagocytosed into human pancreatic cancer cells. These nanoparticles permitted photothermal ablation in a controllable manner with resulting therapeutic reductions to pancreatic cell proliferation dependent upon the photosensitizer dose and power deposition during irradiation. Given the promising results of these initial in-vitro studies, additional investigations are now warranted to rigorously examine the potential therapeutic efficacy of these approaches with in vivo preclinical animal model studies. These approaches may ultimately overcome some of the limitations of current thermal ablation therapies by limiting unwanted damage to adjacent normal tissues.
Intra-procedural imaging-guidance has been widely advocated for most thermal ablation procedures with current modalities including ultrasound, computed tomography, and MRI.Citation24,Citation30,Citation31 X-ray computed tomography, MRI, fluorescence, and optical coherence tomography techniques have each been used to quantitatively visualize gold nanoparticle distributions.Citation6,Citation32–Citation34 However, fluorescence and optical imaging typically require cell fixation and staining; while noninvasive in vivo imaging may be feasible for rodent models, depth penetration issues limit application to the examination of only superficial tissues in large mammal and human studies. Given that lack of ionizing radiation, MRI may offer the greatest potential for clinical translation.
Cellular MRI techniques have been widely developed for in vivo visualization and quantification of single cells labeled with iron oxide nanoparticles.Citation35,Citation36 Quantitative R2 and R2* measurements can be used to map the biodistribution of iron oxide nanoparticles.Citation35,Citation36 The nanoparticles used for the current studies included an iron oxide component as a core of the particles, and subsequent MRI studies demonstrated that increased PANC-1 cell uptake yielded significant changes in both R2 and R2* relaxation. Given the noninvasive nature of these measurements, these can already be readily performed in clinical settings. Such measurements may ultimately be critical for treatment planning, allowing the physicians to potentially adjust laser irradiation parameters (power density and duration) based upon MRI assessments of nanoparticle deposition within the targeted pancreatic tumor tissues. Given the superior soft tissue contrast over competing modalities, MRI may also be ideal for real-time placement of the diode fiber laser probe (akin to current clinical procedures using MRI for the placement of radiofrequency ablation or cryo-probes as well as biopsy needles).Citation37
The primary limitation of this current in-vitro feasibility study was the lack of subsequent in vivo validation. This will be a critical next step and will allow important investigation of physiologic influences that may impact therapeutic outcomes. These influences include the resultant nanoparticle biodistribution (following intravenous systemic administration or direction percutaneous injection) as well as potential local heat losses due to perfusion of the tissue targeted for ablation (heat-sink effects).Citation38 The circulation times of nanoparticles after intravenous injection has been a challenge for targeted therapy. Particle size and surface charge are critical factors for avoiding uptake by macrophages prior to reaching the targeted tumors. Polyethylene glycol can extend circulation times but was not used for surface modification of our nanoparticles for the current study. For future studies, the in vivo bioavailablity of Goldmag nanoparticles should be further investigated. In addition, targeting moieties can be readily added to these nanoparticles to facilitate selective delivery to the targeted tumor tissues. Active targeting such as antibody targeting enhances targeting efficiency and should result in better therapeutic response than passive targeting approaches. Prostate stem cell antigen antibodies have been added to these hybrid iron oxide core gold-shell nanoparticles for selective delivery to prostate tumors.Citation39 Anti-epidermal growth factor receptor antibodies are also overexpressed in most human pancreatic cancer cell lines, offering additional potential targets to facilitate selective delivery.Citation6,Citation9 The decrease in cell proliferation after nanoparticle administration observed in our study may have resulted from the high surface charge of these nanoparticles. Future modification and improvement of these gold nanoparticles is warranted.
In conclusion, these studies demonstrated the potential to use iron oxide gold-shell nanoparticles as MRI-visible photothermal sensitizers during laser irradiation of pancreatic cancer cells. Exposure to these nanoparticles and subsequent laser irradiation led to significant reductions in pancreatic cancer cell proliferation; MRI R2 and R2* measurements correlated to nanoparticle cellular uptake levels. Future in vivo translational studies are now warranted to validate these approaches in animal models of pancreatic cancer.
Acknowledgments
This publication was made possible by: Grant number CA141047 and CA159178 from the National Institutes of Health; and Grant 81220108011 from the National Natural Science Foundation of China. Its contents are solely the responsibility of the authors and do not necessarily represent the official view of the National Institutes of Health.
Disclosure
The authors report no conflicts of interest in this work.
References
- HidalgoMPancreatic cancerN Engl J Med2010362171605161720427809
- JemalASiegelRXuJWardECancer statistics, 2010CA Cancer J Clin201060527730020610543
- PhilipPAMooneyMJaffeDConsensus report of the national cancer institute clinical trials planning meeting on pancreas cancer treatmentJ Clin Oncol200927335660566919858397
- WillettCGCzitoBGBendellJCRyanDPLocally advanced pancreatic cancerJ Clin Oncol200523204538454416002845
- LebedevaIVSarkarDSuZZMolecular target-based therapy of pancreatic cancerCancer Res20066642403241316489047
- ParkJHvon MaltzahnGXuMJCooperative nanomaterial system to sensitize, target, and treat tumorsProc Natl Acad Sci U S A2010107398198620080556
- CobleyCMAuLChenJXiaYTargeting gold nanocages to cancer cells for photothermal destruction and drug deliveryExpert Opin Drug Deliv20107557758720345327
- LiuHChenDTangFPhotothermal therapy of Lewis lung carcinoma in mice using gold nanoshells on carboxylated polystyrene spheresNanotechnology2008194545510121832760
- HuangXEl-SayedIHQianWEl-SayedMACancer cell imaging and photothermal therapy in the near-infrared region by using gold nanorodsJ Am Chem Soc200612862115212016464114
- FisherJWSarkarSBuchananCFPhotothermal response of human and murine cancer cells to multiwalled carbon nanotubes after laser irradiationCancer Res201070239855986421098701
- HuangXJainPKEl-SayedIHEl-SayedMAGold nanoparticles: interesting optical properties and recent applications in cancer diagnostics and therapyNanomedicine (Lond)20072568169317976030
- LuWSinghAKKhanSASenapatiDYuHRayPCGold nanopopcorn-based targeted diagnosis, nanotherapy treatment, and in situ monitoring of photothermal therapy response of prostate cancer cells using surface-enhanced Raman spectroscopyJ Am Chem Soc201013251181031811421128627
- MortonJGDayESHalasNJWestJLNanoshells for photothermal cancer therapyMethods Mol Biol201062410111720217591
- LeungKUltrasmall superparamagnetic iron oxide nanoparticles conjugated with Ile-Pro-Leu-Pro-Phe-Tyr-AsnMolecular Imaging and Contrast Agent Database (MICAD) [Internet]Bethesda (MD)National Center for Biotechnology Information (US)2004–2013 February 23, 2010 [updated March 25, 2010]
- LiuGWangZLuJLow molecular weight alkyl-polycation wrapped magnetite nanoparticle clusters as MRI probes for stem cell labeling and in vivo imagingBiomaterials201132252853720869767
- KinoshitaMYoshiokaYOkitaYHashimotoNYoshimineTMR molecular imaging of HER-2 in a murine tumor xenograft by SPIO labeling of anti-HER-2 affibodyContrast Media Mol Imaging201051182220140973
- IslamTJosephsonLCurrent state and future applications of active targeting in malignancies using superparamagnetic iron oxide nanoparticlesCancer Biomark2009529910719414927
- PersigehlTBiekerRMatuszewskiLAntiangiogenic tumor treatment: early noninvasive monitoring with USPIO-enhanced MR imaging in miceRadiology2007244244945617562810
- ZhangSZouLZhangDPangXYangHXuYGoldMag nanoparticles with core/shell structure: characterization and application in MR molecular imagingJ Nanopart Res20111338673876
- CuiYWangYHuiWZhangZXinXChenCThe synthesis of GoldMag nano-particles and their application for antibody immobilizationBiomed Microdevices20057215315615940431
- LyonJLFlemingDAStoneMBSchifferPWilliamsMESynthesis of Fe oxide core/Au shell nanoparticles by iterative hydroxylamine seedingNano Letters200444719723
- ChengGWalkerARHSynthesis and characterization of cobalt/gold bimetallic nanoparticlesJ Magn Magn Mater20073113135
- MaLLFeldmanMDTamJMSmall multifunctional nanoclusters (nanoroses) for targeted cellular imaging and therapyACS Nano2009392686269619711944
- von MaltzahnGParkJHAgrawalAComputationally guided photothermal tumor therapy using long-circulating gold nanorod antennasCancer Res20096993892390019366797
- EdreiYGrossECorchiaNVascular profile characterization of liver tumors by magnetic resonance imaging using hemodynamic response imaging in miceNeoplasia201113324425321390187
- LencioniRCioniDCrocettiLEarly-stage hepatocellular carcinoma in patients with cirrhosis: long-term results of percutaneous image-guided radiofrequency ablationRadiology2005234396196715665226
- LauWYLaiECThe current role of radiofrequency ablation in the management of hepatocellular carcinoma: a systematic reviewAnn Surg20092491202519106671
- LiangPDongBYuXPrognostic factors for survival in patients with hepatocellular carcinoma after percutaneous microwave ablationRadiology2005235129930715731369
- HinshawJLLeeFTJrCryoablation for liver cancerTech Vasc Interv Radiol2007101475717980318
- HongKGeorgiadesCSGeschwindJFTechnology insight: Image-guided therapies for hepatocellular carcinoma – intra-arterial and ablative techniquesNat Clin Pract Oncol20063631532416757969
- GuoYZhangYNijmGMIrreversible electroporation in the liver: contrast-enhanced inversion-recovery MR imaging approaches to differentiate reversibly electroporated penumbra from irreversibly electroporated ablation zonesRadiology2011258246146821131581
- KimDParkSLeeJHJeongYYJonSAntibiofouling polymer-coated gold nanoparticles as a contrast agent for in vivo X-ray computed tomography imagingJ Am Chem Soc2007129247661766517530850
- YangLMaoHCaoZMolecular imaging of pancreatic cancer in an animal model using targeted multifunctional nanoparticlesGastroenterology2009136515141525e219208341
- ZagaynovaEVShirmanovaMVKirillinMYContrasting properties of gold nanoparticles for optical coherence tomography: phantom, in vivo studies and Monte Carlo simulationPhys Med Biol200853184995500918711247
- ArbabASYocumGTKalishHEfficient magnetic cell labeling with protamine sulfate complexed to ferumoxides for cellular MRIBlood200410441217122315100158
- WuYLYeQFoleyLMIn situ labeling of immune cells with iron oxide particles: an approach to detect organ rejection by cellular MRIProc Natl Acad Sci U S A200610361852185716443687
- MahnkenAHBueckerASpuentrupEMR-guided radiofrequency ablation of hepatic malignancies at 1.5 T: initial resultsJ Magn Reson Imaging200419334234814994303
- KimSKRhimHKimYSRadiofrequency thermal ablation of hepatic tumors: pitfalls and challengesAbdom Imaging200530672773316252153
- RenJZhangZWangFMRI of prostate stem cell antigen expression in prostate tumorsNanomedicine (Lond)20127569170322630152