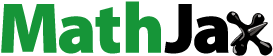
Abstract
Background
The objective of this research was to design an effective gene delivery system composed of cationic solid lipid nanoparticles (SLNs), protamine, and Deoxyribonucleic acid DNA.
Methods
Cationic SLNs were prepared using an aqueous solvent diffusion method with octadecylamine as the cationic lipid material. First, protamine was combined with DNA to form binary protamine/DNA nanoparticles, and the ternary nanoparticle gene delivery system was then obtained by combining binary protamine/DNA nanoparticles with cationic SLNs. The size, zeta potential, and ability of the binary and ternary nanoparticles to compact and protect DNA were characterized. The effect of octadecylamine content in SLNs and the SLNS/DNA ratios on transfection efficiency, cellular uptake and cytotoxicity of the ternary nanoparticles were also assessed using HEK293 cells.
Results
When the weight ratio of protamine to DNA reached 1.5:1, the plasmid DNA could be effectively compacted and protected. The average hydrodynamic diameter of the ternary nanoparticles when combined with protamine increased from 188.50 ± 0.26 nm to 259.33 ± 3.44 nm, and the zeta potential increased from 25.50 ± 3.30 mV to 33.40 ± 2.80 mV when the weight ratio of SLNs to DNA increased from 16/3 to 80/3. The ternary nanoparticles showed high gene transfection efficiency compared with Lipofectamine™ 2000/DNA nanoparticles. Several factors that might affect gene transfection efficiency, such as content and composition of SLNs, post-transfection time, and serum were examined. The ternary nanoparticles composed of SLNs with 15 wt% octadecylamine (50/3 weight ratio of SLNs to DNA) showed the best transfection efficiency (26.13% ± 5.22%) in the presence of serum. It was also found that cellular uptake of the ternary nanoparticles was better than that of the SLN/DNA and binary protamine/DNA nanoparticle systems, and DNA could be transported to the nucleus.
Conclusion
SLNs enhanced entry of binary protamine/DNA nanoparticles into the cell, and protamine protected DNA from enzyme degradation and transported DNA into the nucleus. Compared with Lipofectamine 2000/DNA nanoparticles, these cationic ternary nanoparticles showed relatively durable and stable gene transfection in the presence of serum.
Introduction
Much research effort has been concentrated on gene therapy, because it is a field with great potential for the treatment of congenital and acquired disease,Citation1 such as monogenic hypercholesterolemiaCitation2 and breast cancer.Citation3
Nowadays, the success of gene therapy is principally limited by the low efficiency of vector systems. Historically, two opposing vector systems, ie, viral and nonviral, have been used for gene delivery. Nonviral vectors are mainly composed of cationic polymers and cationic lipids. They are being studied extensively in gene therapy as an attractive alternative to viral-based systems because of the decreased risks, such as oncogenicity or immunogenicity, easier manufacture, and lack of a DNA size limit.Citation4,Citation5 However, the gene transfection efficiency of nonviral vectors is still lower than that of their viral counterparts, so it is necessary to explore further optimal nonviral vectors for gene delivery in order to deliver therapeutic genes to target cells effectively with minimal cell toxicity.
It is known that gene transfection in nonviral systems is conditioned by barriers to intracellular trafficking of the vectors from the exterior of the cell to the nucleus.Citation6 Endocytosis has been considered to be the major cellular entry mechanism for cationic nanoparticles.Citation7,Citation8 Therefore, to achieve efficient expression of the target gene, such nanoparticles must overcome limiting barriers, including internalization into cells,Citation9 gene transfer from the endosome to the cytosol,Citation10 and transport of the target gene to the nucleus.Citation11
Numerous efforts have been made to establish effective and stable gene transfection systems. Several ternary systems have been reported, and were made by compacting Deoxyribonucleic acid (DNA) with cationic condensing molecules first and then adding the cationic liposome. On the one hand, cationic condensing compounds, such as protamineCitation12,Citation13 and tat peptide,Citation14,Citation15 are able to encapsulate a target gene into a compact structure and thereby prevent it against degradation by nuclease. Some of these peptides also function as membrane-disrupting peptides and can destabilize endosomal membranes to increase the efficiency of gene transfection.Citation15 These ternary systems also form small condensed particles that are stable and less prone to the aggregation observed with some lipid-DNA nanoparticles,Citation13 and are suitable for use in vivo. On the other hand, the lipid portion of ternary nanoparticles is essential for efficient transfection, because this permits entry of DNA nanoparticles into the cell via interactions with the cell membraneCitation16 and induces fusion with the endosome membrane to promote gene transfer into the cytosol.Citation17
In this study, protamine, a compound approved by the US Food and Drug Administration,Citation18 was chosen as the condensing peptide because it is a natural polycation existing in most vertebrate sperm cells, and can condense DNA into nanoparticles, with a particular structure in the head of sperm.Citation19,Citation20 Such highly compact nanoparticles are resistant to nuclease and can orientate DNA to the nucleus of the ovum. All these characteristics make protamine attractive for application in transfection. Protamine has been shown by other researchers to enhance cationic lipid-mediated transfection.Citation12,Citation21–Citation23 Addition of protamine, containing nuclear localization signals, improved nuclear entry of DNA.Citation23 However, protamine is a hydrophilic protein, so encounters difficulty crossing the cell membrane.Citation24 Cationic lipid was replaced by solid lipid nanoparticles (SLNs) for investigation of its advantages, such as rapid uptake by cells, protection of the incorporated compound against chemical degradation, and potential for large-scale production.Citation25 SLNs have already been utilized as drug delivery systems,Citation26,Citation27 and their use in gene therapy is attracting increasing attention.Citation12,Citation21 However, their transfection efficiency is low in comparison with that of conventional viral agents. In previously published research,Citation28 we used a nanostructured lipid carrier to form ternary nanoparticles, and the surface charge was negative. Given that cationic SLNs have better ability in terms of endocytosis, in this study we used cationic SLNs to form ternary nanoparticles to test their gene transfection efficiency. Vighi et alCitation29 also used cationic SLNs as a gene vector and the protamine was used as a transfection promoter. It was found that the lower the protamine amount on the surface of the SLN, the lower the efficiency of transfection.
In this research, an SLN/protamine/DNA gene delivery system was designed to improve transfection efficiency. Solid lipid nanoparticles were prepared using an aqueous solvent diffusion method. First, monostearin and octadecylamine were used as solid lipids. Poloxamer 188 (0.1%, w/v) solution was used as the aqueous phase. Monostearin was chosen because it fuses easily with the anionic cell membrane, facilitating uptake by cells.Citation27 The effect of protamine on gene transfection efficiency mediated by SLNs was also investigated. Gene transfection experiments were performed, using human embryonic kidney (HEK) 293 cells as the cell model and pEGFP-N1 and the luciferase reporter gene as reporter genes. The effects of composition of SLNs, post-transfection time, and serum on gene transfection efficiency were examined in detail. The ability of protamine to compact and protect DNA from nuclease and its cellular uptake capacity were then investigated to explore the mechanism responsible for higher transfection efficiency.
Materials and methods
Materials
Monostearin (Shanghai Chemical Reagent Co, Ltd, Shanghai, People’s Republic of China) was used as the solid lipid material for preparation of SLNs. Octadecylamine was purchased from Fluka (Sigma-Aldrich, St Louis, MO, USA), and Poloxamer 188 from Shenyang Jiqi Pharmaceutical Co, Ltd, (Shenyang, People’s Republic of China). Protamine sulfate and DNA (order of DNA, 5′-CCCAGCCTTCCAGCTC-CTTG-3′) labeled with a red dye (ROX) were purchased from Shanghai Sangon Biological Engineering Technology and Services Co, Ltd, (Shanghai, People’s Republic of China). Hoechst 33342 (Sigma-Aldrich) was used as a staining reagent for the nucleus. Fluorescein isothiocyanate (FITC) was purchased from Acros Organic (Fair Lawn, NJ, USA), and 3-(4, 5-dimethylthiazol-2-yl)-2, 5-diphenyl-tetrazolium bromide (MTT) from Sigma-Aldrich. Deoxyribonuclease I (DNase I) was sourced from Takara Biotechnology (Dalian) Co, Ltd, (Mountain View, CA, USA). Dulbecco’s Modified Eagle’s Medium and trypsin-EDTA were purchased from Gibco BRL (Gaithersberg, MD, USA). A bicinchoninic acid protein assay kit was obtained from the Beyotime Institute of Biotechnology (Jiangsu, People’s Republic of China). The luciferase assay agent was provided by Chemi & BioLuminescence (Shanghai, People’s Republic of China). Fetal bovine serum was purchased from Sijiqing Biologic Co, Ltd, (Zhejiang, People’s Republic of China), and pEGFP-N1 (4.7 kb) encoding a green fluorescent protein gene and the luciferase gene were donated by the National Institutes of Health (Bethesda, MD, USA). Ethanol and other chemicals were of analytical reagent grade.
HEK293 cells were provided by the National Institutes of Health. The cells were cultured at 37°C with 5% CO2 under fully humidified conditions. The culture medium was Dulbecco’s Modified Eagle’s Medium supplemented with 10% fetal bovine serum, 100 U/mL penicillin, and 100 μg/mL streptomycin.
Preparation of SLNs by conventional solvent diffusion method
SLNs were prepared using the aqueous solvent diffusion method in an aqueous system, as reported elsewhere.Citation27 Briefly, 50 mg of monostearin and 0–10 mg of octadecylamine were completely dissolved in 5 mL of ethanol in a water bath under 60°C. The resulting organic solution was dispersed rapidly in 50 mL of Poloxamer 188 solution (0.1%, w/v) under mechanical stirring at 400 rpm for 5 minutes. The pre-emulsion (containing melted lipid droplets) obtained was then cooled to room temperature until an SLN dispersion was obtained. The pH of the dispersion was adjusted to 1.2 by adding 0.1 M hydrochloric acid to precipitate the SLNs. After centrifugation (20,000 rpm for 15 minutes), the SLNs obtained were redispersed in 50 mL of Poloxamer 188 solution (0.1%, w/v) and the pH was adjusted to neutral by adding 0.2 M NaOH solution.
Preparation and characterization of protamine/DNA and ternary nanoparticles
Protamine/DNA nanoparticles containing different weight ratios of protamine to DNA were first prepared by vortically mixing DNA solution with appropriate amounts of protamine in distilled water for 30 seconds, and then allowing them to stand for 30 minutes at room temperature. Ternary SLN/protamine/DNA nanoparticles containing different weights of SLNs were prepared by mixing appropriate amounts of SLN nanosuspension with a solution containing binary protamine/DNA nanoparticles for 30 seconds, and then allowing this mixture to stand for 30 minutes at room temperature. The average hydrodynamic diameter, zeta potential, and polydispersity index of the SLN/protamine/DNA nanoparticles were measured by dynamic light scattering using a Zetasizer (3000HS, Malvern Instruments Ltd, Malvern, UK).
Evaluation of surface morphology
Morphologic examination of protamine, binary protamine/DNA nanoparticles, SLNs, and ternary SLN/protamine/DNA nanoparticles was done using atomic force microscopy (SPA 3800N, Seiko, Tokyo, Japan) in tapping mode using a high resonant frequency (F0 = 129 kHz), pyramidal cantilevers, and silicon probes with a force constant of 20 N/m. Scan speeds were set at 2 Hz. The samples were diluted with distilled water and deposited onto freshly cleaved mica plates, followed by vacuum-drying for 24 hours at 25°C.
The morphology of the ternary nanoparticles was examined further by transmission electron microscopy (JEM-1230EX, JOEL, Tokyo, Japan). Samples were prepared by placing a drop of the ternary nanoparticle suspension onto a copper grid, followed by negative staining with one drop of a 2% aqueous solution of sodium phosphotungstate for contrast enhancement. The air-dried samples were then observed directly by transmission electron microscopy.
Gel retardation assay
The potential of the binary nanoparticles (containing different weight ratios of protamine to DNA) and ternary nanoparticles (containing different amounts of SLNs) to condense DNA (1 μg/well) was investigated on 0.8% agarose gel with a current voltage of 120 V for 30 minutes in Tris-acetate-EDTA buffer solution (40 mM Tris-HCl, 1% (v/v) acetic acid, 1 mM EDTA). Images were obtained using an ultraviolet transilluminator and a digital imaging system (GL 200, Kodak, Windsor, CO, USA).
DNase I protection assay
For the DNase I protection assay, the binary and ternary nanoparticles (DNA, 1 μg/well) were incubated in a buffer solution (10 mM Tris-HCl, 150 mM NaCl, 1 mM MgCl2, pH 7.4) containing 20 units of DNase I. The change in ultraviolet absorbance at 260 nm was measured at different time intervals using an ultraviolet spectrophotometer (Ultrospec 5300pro, ImageQuant, Piscataway, NJ, USA).
In vitro gene transfection test
HEK293 cells were seeded in 24-well plates at a density of 1 × 105 cells/well in 1 mL of complete medium. After incubation for 24 hours, the medium was replaced by 0.5 mL of serum-free or 10% fetal bovine serum containing binary or ternary nanoparticles (pDNA, 2 μg/well), and then incubated for a further 6 hours. The medium was then replaced with 1 mL of fresh complete medium, and incubation was continued for a further 72 hours at 37°C. A gene transfection test using Lipofectamine™ 2000/DNA nanoparticles was performed as a positive control following the manufacturer’s protocol.
Luciferase gene expression was assessed by measuring luciferase activity with a chemiluminescence detector (FB12, Sirius, Berthold Detection Systems, Hannover, Germany). Relative light units were normalized to protein concentration in the cell extracts, and measured using the bicinchoninic acid method with an enzyme-linked immunosorbent assay plate reader (Model 680, Bio-Rad Laboratories, Hercules, CA, USA).
Transfection efficiency was also evaluated by FACScan analysis. After 24, 48, and 72 hours of incubation, the cells were washed with cold Dulbecco’s phosphate-buffered saline and harvested by addition of trypsin-EDTA solution. Next, 1 mL of phosphate-buffered saline was added and the cells were centrifuged at 1,000 × g for 4 minutes. The pellet was then suspended in phosphate-buffered saline containing 2% formalin and analyzed on a FACScan flow cytometer (FACSCalibur, Becton Dickinson, BD Biosciences, Franklin Lakes, NJ, USA). Determination of green fluorescent protein- positive events was performed using a standard gating technique. The cells were also viewed directly under a fluorescent microscope (Olympus America, Melville, NY, USA).
Cell growth inhibition assay
The effects of the SLNs and ternary nanoparticles on inhibition of cell growth were determined by MTT assay, with HEK293 cells used as the cell model. The cells were seeded in 24-well plates at a density of 1 × 105 cells/well and incubated for 24 hours prior to addition of SLNs and ternary nanoparticles. The medium containing SLNs and ternary nanoparticles was replaced by fresh complete medium after 6 hours of incubation. After further incubation for 72 hours, the medium was replaced with complete medium containing 200 μg/mL MTT, and incubated for a further 4 hours. Absorbance was then measured at 570 nm using the enzyme-linked immunosorbent assay plate reader. Untreated cells were used as controls. Growth assays were performed in triplicate. Cell viability was calculated using the following equation:
Cellular uptake of DNA and location of ternary nanoparticles
ROX-labeled DNA was used to investigate trafficking of DNA inside the cells. SLNs loaded with octadecylamine-FITC were synthesized using a previously reported method.Citation30 The cells were seeded in 24-well plates at a density of 1 × 105 cells/well in 1 mL of complete medium. After incubation for 24 hours, the medium was replaced with 0.5 mL of medium containing DNA, binary nanoparticles, or ternary nanoparticles, and incubated for a further 6 hours. Prior to observation of the samples under a microscope, the cell nuclei were labeled with Hoechst 33342 for 15 minutes and then washed three times with phosphate-buffered saline. Images were captured using a fluorescent microscope (Olympus America). Observations and image capturing were done using a 20× objective.
Statistical analysis
The results are reported as the mean ± standard deviation. Statistical analysis between different groups was done using the non-paired t-test. Differences were considered to be statistically significant if P was <0.05.
Results and discussion
Preparation of ternary SLN/protamine/DNA nanoparticles
SLNs containing different amounts of octadecylamine were prepared using the solvent diffusion method. Ternary SLN/protamine/DNA nanoparticles were then prepared by mixing appropriate amounts of SLNs, protamine, and DNA. The size and zeta potential of the nanoparticles was measured using a dynamic light scattering technique. SLNs with 15 wt% octadecylamine had an average size of 112.17 ± 4.65 nm and a zeta potential of 42.7 ± 0.8 m V. Binary protamine/DNA nanoparticles (1.5:1, w/w) had an average size of 125.43 ± 3.44 nm and a zeta potential of 6.6 ± 1.0 mV. shows the relationship of average hydrodynamic diameter, zeta potential, and weight ratio of SLNs (15 wt% octadecylamine) with DNA in the ternary nanoparticles. When the weight ratio of SLNs to DNA increased from 16/3 to 80/3, the size increased from 188.50 ± 0.26 nm to 259.33 ± 3.44 nm and the zeta potential increased from 25.50 ± 3.30 mV to 33.40 ± 2.80 mV. The ternary nanoparticles also showed a decrease in size distribution with increasing weight ratio of SLNs to DNA (). Nanoparticles have an affinity for positively and negatively charged molecules because of their large surface energy.Citation31,Citation32 Although the binary protamine/DNA nanoparticles and SLNs were positively charged, the zeta potential of the binary protamine/DNA nanoparticles was relatively low, and ternary nanoparticles could be formed by nonspecific absorption between biomacromolecules (ie, binary nanoparticles) and SLNs.
Figure 1 Average hydrodynamic diameter, zeta potential, and polydispersity index of SLN/protamine/DNA nanoparticles containing different amounts of SLNs to DNA (protamine/DNA, 1.5:1, w/w).
Abbreviations: SLN, solid lipid nanoparticles; DNA, deoxyribonucleic acid; ODA, octadecylamine.
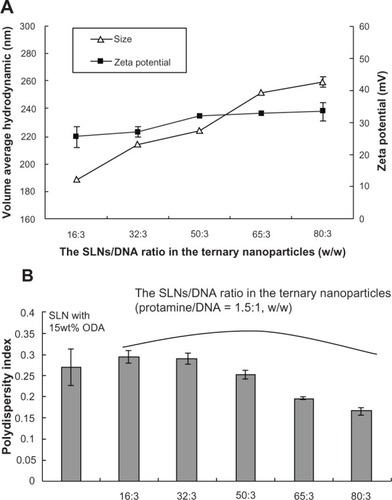
Atomic force microscopy was used to investigate the morphology of the binary and ternary nanoparticles. – shows atomic force microscopic images of the binary protamine/DNA (1.5:1, w/w) nanoparticles, blank SLNs (15 wt% octadecylamine), and ternary SLN/protamine/DNA nanoparticles (weight ratio of SLNs to protamine and DNA, 50/4.5/3), respectively. SLNs prepared using the solvent diffusion method were less than 100 nm in diameter, with a smooth surface morphology and a spherical shape (). The aqueous solution of protamine was seen to cover the mica plates entirely and to form a lamellar structure less than 0.5 nm in size (data not shown), whereas the binary protamine/DNA nanoparticles formed spherical, round-shaped compounds () with an average size of about 100 nm. The size of the ternary SLN/protamine/DNA nanoparticles was slightly greater than that of the binary ones and the blank SLNs ().
Figure 2 Atomic force microscopic images of (A) binary protamine/DNA nanoparticles (1.5:1, w/w), (B) SLN with 15 wt% ODA, (C) SLN/protamine/DNA nanoparticles (SLNs with 15 wt% ODA/protamine/DNA, 50:4.5:3, w/w/w), transmission electron microscopic images of (D) binary protamine/DNA nanoparticles, (E) SLNs with 15 wt% ODA (1.5:1, w/w), and (F) SLN/protamine/DNA nanoparticles (SLNs with 15 wt% ODA/protamine/DNA, 50:4.5:3, w/w/w).
Abbreviations: ODA, octadecylamine; SLNs, solid lipid particles; DNA, deoxyribonucleic acid.
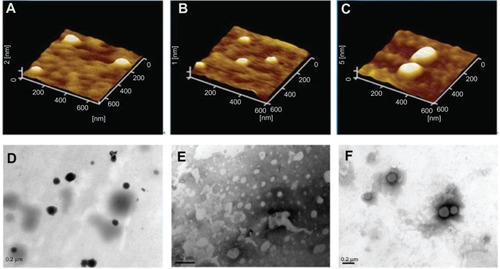
Topographic imaging using transmission electron microscopy also showed the structure of the binary protamine/DNA (1.5:1, w/w) nanoparticles, blank SLNs (15 wt% octadecylamine), and ternary SLN/protamine/DNA nanoparticles (–). They were observed to form small spherical particles, and the size of the tested nanoparticles corresponded to the results determined by atomic force microscopy.
Gel retardation assay
The ability of the binary, SLN/DNA, and ternary nanoparticles to condense DNA was analyzed by agarose gel electrophoresis. Complete retardation of the binary protamine/DNA nanoparticles was observed when the weight ratio was above 1.0 (). In our previous research, we investigated the DNA condensation ability of SLNs, and showed that SLNs with 10 wt% or 15 wt% octadecylamine could completely retard DNA migration when the weight ratio of SLNs to DNA was above 3 (data not shown). Moreover, when the weight ratio of protamine to DNA was reduced to 0.5:1 and the weight ratio of SLNs to DNA in SLN/protamine/DNA nanoparticles was fixed at 3, stable nanoparticles could also be formed, and the DNA could be condensed completely ().
Figure 3 Gel retarding (A and B) and DNase I degradation assay (C) of protamine/DNA nanoparticles and SLN/protamine/DNA nanoparticles. In (B), lanes 3, 4, and 5 represent SLN/protamine/DNA nanoparticles prepared with SLNs containing 0 wt%, 10 wt%, or 15 wt% ODA (w/w/w, 3:0.5:1). (C) line A represents naked DNA as a control, line B represents SLN/DNA nanoparticles (SLNs with 15 wt% ODA/DNA, 50:3, w/w), line C represents binary protamine/DNA nanoparticles at a weight ratio of 1.5:1, line D represents SLN/protamine/DNA nanoparticles (SLNs with 15 wt% ODA/protamine/DNA, 50:4.5:3, w/w/w), and line E represents SLN/protamine/DNA nanoparticles (SLNs with 0 wt% ODA/protamine/DNA, 50:4.5:3, w/w/w).
Abbreviations: M, marker; DNA, deoxyribonucleic acid; ODA, octadecylamine; SLNs, solid lipid particles.
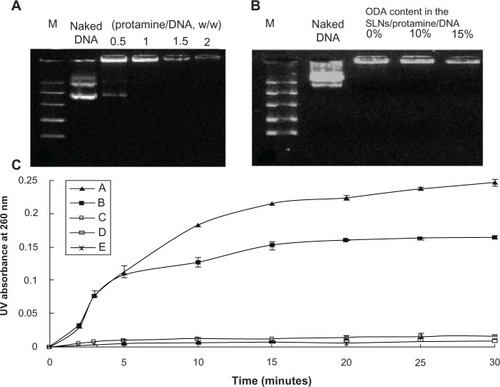
DNase I protection assay
The effect of protection to pDNA from nuclease degradation was examined using DNase I as a model enzyme. The results are shown in , which clearly demonstrates that naked plasmid DNA was degraded after 15 minutes. SLN/DNA nanoparticles (with 15 wt% octadecylamine) could not protect the plasmid DNA from enzyme degradation efficiently, and the weight ratio of SLNs to DNA was increased to 50/3. Combined with the gel retardation results for SLN/DNA nanoparticles, it was found that although the cationic SLNs could compact plasmid DNA, they could not protect the DNA from degradation. However, binary protamine/DNA nanoparticles (1.5:1, w/w) and ternary SLN/protamine/DNA nanoparticles (50:4.5:3, w/w/w) provided powerful protection after 30 minutes. This indicates that protection of plasmid DNA against nucleases could be achieved after incorporating protamine, which is a requisite for efficient gene delivery in vivo and in vitro.Citation33
In vitro gene transfection
Intracellular expression of plasmid DNA (pEGFP-N1) was examined using fluorescent microscopy and flow cytometry. Plasmid pGL-3 was used as the luciferase reporter gene, and a luciferase expression assay was carried out. Moreover, the effects of serum, ratio of SLNs to DNA, content of octadecylamine in SLNs, and post-transfection time on gene transfection efficiency were investigated.
Effect of SLN content in ternary nanoparticles
Fixing the weight ratio of protamine to DNA at 1.5:1, the gene transfection efficiency of the ternary nanoparticles containing different weight ratios of SLNs with 15 wt% octadecylamine to DNA were examined. The results are shown in . It is clear that the best transfection was obtained when the SLN/protamine/DNA content was optimal, ie, containing concentrations of 50 μg/mL, 4.5 μg/mL, and 3.0 μg/mL for SLNs, protamine, and DNA, respectively. From the results of flow cytometry, it was calculated that the percentage of cells transfected was 26.13% ± 5.22% and was nearly half that of Lipofectamine 2000/DNA (55.02% ± 10.93%) without serum. Transfection efficiency decreased when the SLN content in the ternary nanoparticles was increased further, which may result from the toxicity at high doses and larger size ().
Figure 4 Effect of amounts of SLNs on gene transfection of SLN/protamine/DNA nanoparticles when the weight ratio of SLNs with 15 wt% ODA to DNA increases from 16:3 to 80:3 with a weight ratio of protamine to DNA of 1.5:1.
Abbreviations: ODA, octadecylamine; SLNs, solid lipid particles; DNA, deoxyribonucleic acid; RLU, relative luminescence units.
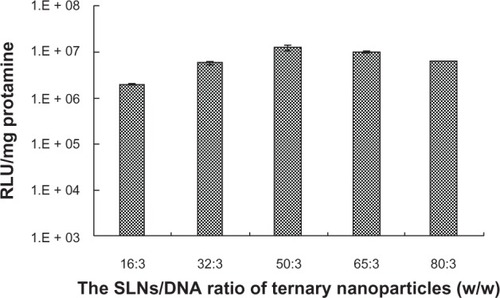
Effect of octadecylamine content in SLNs
SLNs with the same monostearin content but different percentages of octadecylamine were prepared, and gene transfection experiments were carried out using SLN/protamine/DNA nanoparticles with SLNs containing 0 wt%, 5 wt%, 10 wt%, 15 wt%, and 20 wt% octadecylamine. Each assay was done using the same content of binary protamine/DNA nanoparticles (4.5 μg/mL protamine and 3.0 μg/mL DNA) and 50 μg/mL SLNs (monostearin content 50 μg/mL). The results are shown in . The transfection efficiency of the ternary nanoparticles improved with increasing octadecylamine content in the SLNs. However, the efficiency of ternary nanoparticles containing SLNs with 20 wt% octadecylamine was lower than that of SLNs with 15 wt% octadecylamine, which might result from higher cytotoxicity of SLNs with 20 wt% octadecylamine. SLN/protamine/DNA nanoparticles without octadecylamine in the SLNs showed the lowest transfection efficiency, which may be due to lack of effective interaction between their low positive surface charge (8.7 ± 0.3 mV) and the negatively charged cell membrane.
Figure 5 Fluorescent microscopy of heK293 cells transfected by pEGFP-N1. (A) Negative control (naked DNA only), (B) transfection with protamine/DNA nanoparticles for 72 hours, (C, E and G) cells transfected with Lipofectamine™ 2000/DNA for 24, 48, and 72 hours, respectively. (D, F and H) cells transfected with SLN/protamine/DNA nanoparticles (50 μg/mL SLNs containing 15 wt% ODA, 4.5 μg/mL protamine, 3.0 μg/ml DNA) for 24, 48, and 72 hours, respectively, (I) negative control, and (J–N) cells transfected with SLN/protamine/DNA nanoparticles containing 0 wt%, 5 wt%, 10 wt%, 15 wt%, and 20 wt% ODA, respectively (concentrations of all types of SLNs, 50 μg/mL with 4.5 μg/mL protamine and 3.0 μg/mL DNA).
Abbreviations: ODA, octadecylamine; SLNs, solid lipid particles; DNA, deoxyribonucleic acid.
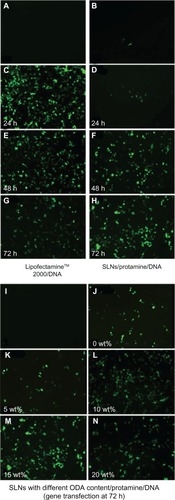
Effect of post-transfection time
Gene transfection was also observed using a fluorescent microscope at 24, 48, and 72 hours post-transfection. shows a sharp increase in Lipofectamine 2000/DNA expression in the initial 24 hours post-transfection. Green fluorescent protein expression decreased rapidly after 24 hours. When ternary nanoparticles was used as the transfection system (culture medium containing 10% fetal bovine serum, 50 μg/mL 15% octadecylamine-loaded monostearin SLNs, 4.5 μg/mL protamine, 3.0 μg/mL DNA), the intensity of fluorescence increased during the 72 hours post-transfection. Successful clinical gene therapy has been limited by lack of sustained gene expression. This system may be advantageous in the treatment of genetic diseases such as cystic fibrosis in which there is a need for long-acting therapeutic effects.Citation35
Effect of serum
The gene transfection efficiency of the ternary nanoparticles and Lipofectamine 2000/DNA nanoparticles was investigated using both luciferase gene expression and flow analysis. shows the relative light units of luciferase gene expression for the ternary SLN/protamine/DNA nanoparticles and Lipofectamine 2000/DNA nanoparticles with and without serum. The transfection efficiency of the ternary nanoparticles increased significantly (P < 0.05, t-test) in the presence of 10% serum, may be due to desired cell viability. However, Lipofectamine 2000/DNA nanoparticles showed less transfection activity in the presence of 10% serum (P < 0.05, t-test) than Lipofectamine 2000/DNA nanoparticles without serum, which might be attributable to interaction between Lipofectamine 2000 and proteins in serum. Flow analysis was also used to quantify gene transfection efficiency. For the ternary nanoparticles, the percentage of transfected cells was highest (26.13% ± 5.22%) in the presence of serum, indicating that the ternary nanoparticles were more suitable than Lipofectamine 2000 for gene transfection in vivo. Several other studies have also demonstrated that addition of polycations to cationic lipids enhances transfection activity in the presence of serum.Citation36 It was confirmed that the gene transfection efficiency of ternary SLN/protamine/DNA nanoparticles (50:4.5:3, w/w/w) in the presence of serum was greater than that of Lipofectamine 2000/DNA nanoparticles. This result may favor future in vivo application of ternary SLN/protamine/DNA nanoparticles as a gene delivery system.
Figure 6 Effects of serum on transfection of lipofectamine™2000/DNA and SLN/protamine/DNA nanoparticles (SLNs with 15 wt% ODA/protamine/DNA, 50:4.5:3, w/w/w) using a luminescence assay. *P < 0.05 by t-test. Transfection time for Lipofectamine 2000/DNA and SLN/protamine/DNA nanoparticles was 24 and 72 hours, respectively.
Abbreviations: ODA, octadecylamine; RLU, relative light units; SLNs, solid lipid particles; DNA, deoxyribonucleic acid.
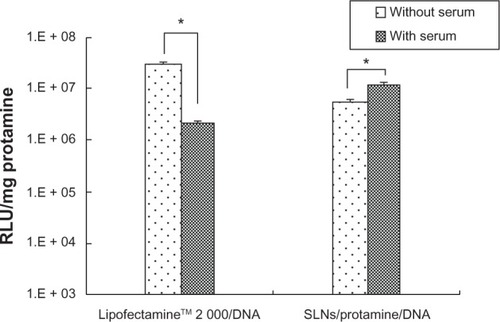
Cell growth inhibition assay
The inhibitory effects of the different nanoparticles on cell growth were determined by MTT assay. shows survival rates after HEK293 cells were incubated for 72 hours with ternary SLN/protamine/DNA nanoparticles in which SLNs contained 15 wt% octadecylamine. Cell viability using all the gene delivery systems was greater than 90% after 72 hours of incubation. An octadecylamine content of 15 wt% in SLNs did not significantly increase the cytotoxicity of the ternary nanoparticles.
Figure 7 Cell viability by MTT test. (1) Negative control, (2) SLN/protamine/DNA nanoparticles (50 μg/mL SLNs containing 15 wt% ODA, 4.5 μg/mL protamine, 3.0 μg/mL DNA), (3) SLNs (50 μg/mL SLNs containing 0 wt% ODA, 4.5 μg/mL protamine, 3.0 μg/mL DNA), (4) Lipofectamine™ 2000/DNA nanoparticles (6 μg/mL Lipofectamine 2000, 3.0 μg/mL DNA), and (5) protamine/DNA binary nanoparticles (4.5 μg/mL protamine, 3.0 μg/mL DNA). *P < 0.05 comparing it with Lipofectamine.
Abbreviations: ODA, octadecylamine; SLNs, solid lipid particles; DNA, deoxyribonucleic acid.
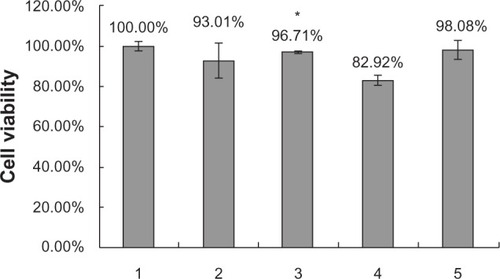
Detection of intracellular ROX-labeled DNA by fluorescent microscopy
A DNA trafficking study was performed to detect uptake of the nanoparticles into HEK293 cells. show fluorescent images of these cells after incubation with the nanoparticles for 6 hours. It can be seen that cellular uptake of DNA in the SLN/DNA nanoparticles was relatively less, which may be due to the low affinity between SLNs and DNA, and the low DNase I protection ability of SLNs (data shown in ). It seemed that protamine could condense the DNA molecules into a form that was compatible with endocytosis, and the ternary nanoparticles with SLNs containing 15 wt% octadecylamine could further enhance DNA uptake by cells. However, the SLN/protamine/DNA nanoparticles without octadecylamine in the SLNs were less internalized into cells, which may result from the relative low positive surface charge of the ternary nanoparticles (8.7 ± 0.3 mV). indicates the location of ROX-labeled DNA and FITC-labeled SLNs in cells after incubation with ternary SLN/protamine/DNA nanoparticles for 6 hours. It is clear that the SLNs were distributed only in the cytoplasm, while most of the DNA was conveyed to the nucleus, as seen in . This is possibly due to enhanced nuclear importing of the DNA released, that promoted the transfection efficiency of this SLN-based gene delivery system.
Figure 8 Fluorescent images after cells were incubated with fluorescent nanoparticles for 6 hours. The cells were treated with Hoechst 33342 (blue) to detect the nucleus and DNA was labeled by ROX (red).
Notes: (A) is naked DNA. (C) is the DNA of SLNs with 15% ODA/DNA. (E) is the DNA of Protamine/DNA. (G) is the DNA of SLNs with 0 wt ODA/protamine/DNA. (B, D, F, H, J) are the nucleus corresponding to the above group. (I) is the DNA of SLNs with 15% Wt DA/protamine/DNA. (K–O) are the merged images of DNA with nucleus.
Abbreviations: ODA, octadecylamine; SLNs, solid lipid particles; DNA, deoxyribonucleic acid.
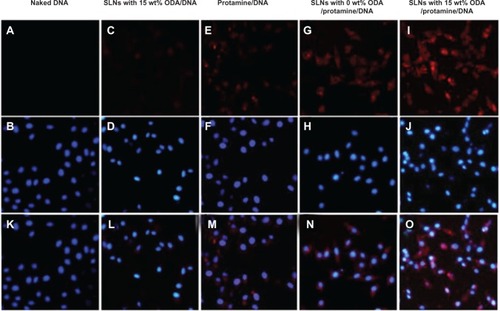
Figure 9 Fluorescent images of cells incubated with the fluorescent ternary nanoparticles for 6 hours. The cells were treated with Hoechst 33342 to detect the nucleus, SLNs were labeled with ODA-FITC (green), and DNA was labeled with ROX (red). (A) ROX-DNA, (B) ODA-FITC-loaded SLNs, (C) nucleus, (D) observed under transmitted light, (E) merged image of ROX-DNA and nucleus, and (F) merged image of ODA-FITC and nucleus.
Abbreviations: ODA, octadecylamine; FITC, fluorescein isothiocyanate; SLNs, solid lipid particles; DNA, deoxyribonucleic acid.
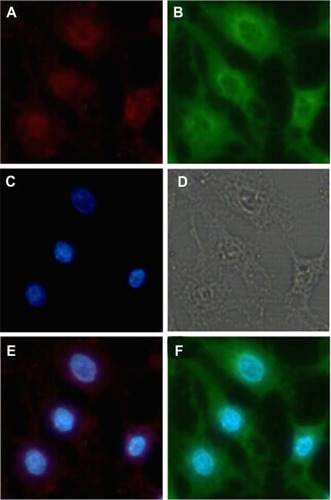
Conclusion
In this paper, we have reported on a cationic ternary gene delivery system prepared by mixing cationic SLNs and binary protamine/DNA nanoparticles. Ternary nanoparticles containing 50 μg/mL SLNs with 15 wt% octadecylamine, 4.5 μg/mL protamine, and 3.0 μg/mL DNA had a small size (around 200 nm) and showed optimal transfection efficiency in the presence of 10% serum at 72 hours post-transfection. The lipid portion appeared to be essential for efficient transfection because it enhanced entry of binary protamine/DNA nanoparticles into the cell, with protamine acting as a DNA condenser to avoid enzyme degradation and also acting as the nucleus transporter. These cationic ternary nanoparticles can express gene more sustained in the presence of serum than Lipofectamine 2000/DNA nanoparticles. Our results suggest that these cationic ternary nanoparticles have potential as an effective and long-acting nonviral vector for gene delivery.
Acknowledgments
This work was sponsored by the Zhejiang Provincial Program for Cultivation of High-Level Innovative Health talents.
Disclosure
The authors report no conflicts of interest in this work.
References
- WagnerAMSchoeberleinASurbekDFetal gene therapy: opportunities and risksAdv Drug Deliv Rev20096181382119426772
- KassimSHLiHVandenbergheLHGene therapy in a humanized mouse model of familial hypercholesterolemia leads to marked regression of atherosclerosisPLoS One20105e1342420976059
- YangJLamDHGohSSTumor tropism of intravenously injected human-induced pluripotent stem cell-derived neural stem cells and their gene therapy application in a metastatic breast cancer modelStem Cells2012301021102922311724
- MorilleMPassiraniCVonarbourgAClavreulABenoitJPProgress in developing cationic vectors for non-viral systemic gene therapy against cancerBiomaterials2008293477349618499247
- SmithAEViral vectors in gene therapyAnnu Rev Microbiol1995498078388561480
- PathakAPatnaikSGuptaKCRecent trends in non-viral vector-mediated gene deliveryBiotechnol J200941559157219844918
- GodbeyWTWuKKMikosAGTracking the intracellular path of poly(ethylenimine)/DNA nanoparticles for gene deliveryProc Natl Acad Sci U S A1999965177518110220439
- YamanoSDaiJYuviencoCKhapliSMoursiAMMontclareJKModified Tat peptide with cationic lipids enhances gene transfection efficiency via temperature-dependent and caveolae-mediated endocytosisJ Control Release201115227828521315780
- IslamMAYunCHChoiYJAccelerated gene transfer through a polysorbitol-based transporter mechanismBiomaterials2011329908992421959011
- El-SayedAMasudaTKhalilIAkitaHHarashimaHEnhanced gene expression by a novel stearylated INF7 peptide derivative through fusion independent endosomal escapeJ Control Release200913816016719465073
- PanyamJLabhasetwarVBiodegradable nanoparticles for drug and gene delivery to cells and tissueAdv Drug Deliv Rev20035532934712628320
- VighiEMontanariMRuoziBTosiGMagliALeoENuclear localization of cationic solid lipid nanoparticles containing protamine as transfection promoterEur J Pharm Biopharm20107638439320691262
- JunghansMLoitschSMSteinigerSCKreuterJZimmerACationic lipid-protamine-DNA (LPD) nanoparticles for delivery of antisense c-myc oligonucleotidesEur J Pharm Biopharm20056028729415939239
- LoSLWangSAn endosomolytic Tat peptide produced by incorporation of histidine and cysteine residues as a nonviral vector for DNA transfectionBiomaterials2008292408241418295328
- ArthanariYPluenARajendranRAojulaHDemonacosCDelivery of therapeutic shRNA and siRNA by Tat fusion peptide targeting BCR-ABL fusion gene in chronic myeloid leukemia cellsJ Control Release201014527228020403398
- TrosDICSunYDuzgunesNGene delivery by lipoplexes and polyplexesEur J Pharm Sci20104015917020359532
- ChanCLMajzoubRNShiraziRSEndosomal escape and transfection efficiency of PEGylated cationic liposome-DNA nanoparticles prepared with an acid-labile PEG-lipidBiomaterials2012334928493522469293
- MartinezGJCsabaNFischerSSurface coating of PLGA microparticles with protamine enhances their immunological performance through facilitated phagocytosisJ Control Release200813016116718588928
- BraunREPackaging paternal chromosomes with protamineNat Genet200128101211326265
- ThuMSBryantLHCoppolaTSelf-assembling nanoparticles by combining ferumoxytol, heparin and protamine for cell tracking by magnetic resonance imagingNat Med20121846346722366951
- VighiEMontanariMRuoziBIannuccelliVLeoEThe role of protamine amount in the transfection performance of cationic SLN designed as a gene nanocarrierDrug Deliv20121911022070724
- SorgiFLBhattacharyaSHuangLProtamine sulfate enhances lipid-mediated gene transferGene Ther199749619689349433
- ChenJYuZChenHGaoJLiangWTransfection efficiency and intracellular fate of polycation liposomes combined with protamineBiomaterials2011321412141821047681
- LiuJGuoSLiZLiuLGuJSynthesis and characterization of stearyl protamine and investigation of their nanoparticles with DNA for gene deliveryColloids Surf B Biointerfaces200973364119481426
- MehnertWMaderKSolid lipid nanoparticles: production, characterization and applicationsAdv Drug Deliv Rev20014716519611311991
- NassimiMSchlehCLauensteinHDA toxicological evaluation of inhaled solid lipid nanoparticles used as a potential drug delivery system for the lungEur J Pharm Biopharm20107510711620206256
- YuanHMiaoJDuYZYouJHuFQZengSCellular uptake of solid lipid nanoparticles and cytotoxicity of encapsulated paclitaxel in A549 cancer cellsInt J Pharm200834813714517714896
- YuanHZhangWDuYZYouJHuFQTernary nanoparticles composed of anionic lipid nanoparticles/protamine/DNA for gene deliveryInt J Pharm201039222423120230883
- VighiEMontanariMHanuskovaMIannuccelliVCoppiGLeoEDesign flexibility influencing the in vitro behavior of cationic SLN as a nonviral gene vectorInt J Pharm201344016116922982257
- YuanHChenJDuYZHuFQZengSZhaoHLStudies on oral absorption of stearic acid SLN by a novel fluorometric methodColloids Surf B Biointerfaces20075815716417446050
- HeinzHFarmerBLPandeyRBNature of molecular interactions of peptides with gold, palladium, and Pd-Au bimetal surfaces in aqueous solutionJ Am Chem Soc20091319704971419552440
- FischerFDWaitzTVollathDSimhaNKOn the role of surface energy and surface stress in phase-transforming nanoparticlesProg Mater Sci200853481527
- Harada-ShibaMTakamisawaIMiyataKIntratracheal gene transfer of adrenomedullin using polyplex nanomicelles attenuates monocrotaline-induced pulmonary hypertension in ratsMol Ther2009171180118619337232
- RorkeSKeeneyMPanditANon-viral polyplexes: scaffold mediated delivery for gene therapyProg Polym Sci201035441458
- AitkenMLBellonGDe BoeckKLong-term inhaled dry powder mannitol in cystic fibrosis: an international randomized studyAm J Respir Crit Care Med201218564565222198974
- ObataYSuzukiDTakeokaSEvaluation of cationic assemblies constructed with amino acid-based lipids for plasmid DNA deliveryBioconjug Chem2008191055106318429628