Abstract
The utilization of self-microemulsifying premicroemulsion systems (SMEPMS) as templates for preparing poorly water-soluble compounds in the nanosized range represents a promising strategy. Fenofibrate was formulated with n-butyl L-lactate, Tween 80, and a number of cosurfactants (ethanol, 1-propanol, and PEG 600), diluted with the water phase (either water or saccharide solution) and then subjected to a freeze-drying (FD) process to obtain SMEPMS nanosized particulates. Results demonstrated that the particle size after resuspension of these FD SMEPMS nanosized particulates in water was too large, so the addition of saccharide solutions (lactose, mannitol, glucose, sucrose, and trehalose) as the solid carrier to prevent particles from aggregating seemed to be necessary and workable due to steric hindrance and repulsion. However, instability of these resuspended FD nanosized particulates after 30–90 minutes still occurred, and the addition of 0.5% sodium lauryl sulfate in the resuspending medium was able to retard the aggregation and maintain the particle size within the nano-range. Evaluation by scanning electron microscopy and X-ray powder diffraction also confirmed the results. It was concluded that using an SMEPMS formulation with PEG 600 as the cosurfactant, and in the presence of a suitable saccharide as an anticaking agent and FD process were able to produce fenofibrate nanoparticles.
Introduction
Many newly discovered or existing drug molecules are poorly water-soluble, and thus, they face challenges to become successful therapies and to be marketedCitation1 due to problems of poor bioavailability after oral administration. Since dissolution is the rate-determining step to make these drugs available to patients, formulation design is a practical approach to improve the absorption and efficacy of such drugs.Citation2 Fenofibrate (FFB) is a neutral lipophilic compound (log P = 5.24), and it is practically insoluble in water (with an aqueous solubility <0.5 mg/L), resulting in incomplete and irregular bioavailability after oral ingestion. Reduction in the particle size of FFB by a micronization process improved its solubility and subsequently increased the bioavailability.Citation3 Therefore, development of a cost-effective process to reduce the particle size of FFB to improve its bioavailability would be beneficial.
Microemulsions (MEs) were first introduced decades ago by Hoar and SchulmanCitation4 and many advantages of MEs were found, especially in enhancing the oral bioavailability of poorly soluble drugs and nutraceuticals.Citation5 Self-microemulsifying premicroemulsion systems (SMEPMS) are mixtures of oils and surfactants, sometimes including cosurfactants, and represent a promising alternative to poorly water-soluble compounds. SMEPMS can be self-emulsified into a single optically isotropic and thermodynamically stable ME when contacting with an aqueous medium under gentle digestive motility in the gastrointestinal tract.Citation6–Citation8 The intrinsic physicochemical properties include nanosize, transparency, low viscosity, and thermodynamic stability.Citation8 Nanosized droplets have very high surface-to-volume ratios that lead to high solubilization capacities, enhanced drug release, and subsequently improved bioavailability,Citation9 and offer release in a more reproducible manner, which is less dependent on the gastrointestinal physiology and the fed/fasted state of patients.Citation10
SMEPMS have been reported to improve the in vivo dissolution, and, therefore, enhance the bioavailability of lipophilic drugs.Citation11–Citation15 Commercially available drugs, including cyclosporin A,Citation16 ritonavir, and saquinavir (HIV protease inhibitors),Citation17 are formulated with SMEPMS, and the usefulness of these systems was also clinically demonstrated. However, SMEPMS usually possess liquids or is encapsulated in gelatin capsules. Such dosage forms might be inconvenient for patients’ use and also tend to leak during storage. Hence, a strategy of incorporating liquid SMEPMS into a solid dosage form would overcome the shortcomings of liquid formulations.Citation18
Furthermore, the concept of diluting ME systems with water (continuous phase) to produce nanoparticles of mitotane and griseofulvin by a diffusion technique was introduced by Trotta et al.Citation19,Citation20 The process is based on the water miscibility of those solvents used as the oil phase to solubilize the drug. Upon transferring a transient oil-in-water emulsion (E) or ME into water, the drug dissolved in the organic phase and instantly solidified due to the almost complete diffusion of the organic solvent from the droplets to the continuous phase. Using optimized formulations of Es or MEs and homogenization parameters, drug particles of <100 nm with very narrow polydispersity and high dissolution were obtained. The feasibility of preparing nanodrugs of griseofulvin and mitotane from solvent-in-water MEs by the diffusion technique was validated using nontoxic solvents, surfactants, and cosurfactants. This indicated that optimized dilutable SMEPMS using these partially water-miscible solvents were able to be used as a template for preparing nanodrugs and nanoparticles.
One important application of ME systems is the use as templates, allowing one to obtain monodispersed sizes of nanoparticles (inorganic or organic) by varying the size of the ME droplet radius. In this study, the utilization of dilutable SMEPMS to form ME as a template for preparing nanosized FFB was developed using n-butyl L-lactate, Tween 80, and a number of cosurfactants (ethanol, 1-propanol, and polyethylene glycol 600). Pseudo-ternary phase diagrams for dilutable SMEPMS were constructed to characterize and optimize the oil phase, surfactants, and cosurfactants to form FFB nanoparticulates.
Materials and methods
Materials
n-Butyl L-lactate was purchased from Alfa Aesar GmbH and Co KG (Karlsruhe, Germany); the Tween series and 1-pro-panol were supplied by Riedel-de Haën (Seelze, Germany); ethanol was bought from Taiwan Sugar Corporation (Taipei, Taiwan); Cremophor RH-40 was purchased from BASF (Ludwigshafen, Germany); polyethylene glycol (PEG) 600 was supplied by Merck KGaA (Darmstadt, Germany); lactose and mannitol were from DFE Pharma (Borculo, the Netherlands); and D(+)-glucose, sucrose, and D(+)-trehalose were purchased from Sigma-Aldrich (St Louis, MO, USA)
Construction of pseudo-ternary phase diagrams to form FFB SMEPMS
The pseudo-ternary phase diagrams of oil, surfactant, and cosurfactant were developed using a water titration method. For each phase diagram, surfactant/cosurfactant (Tween 80/ethanol, 1-propanol, PEG 600), at a specific ratio of 1/1, 1/4, 4/1 (w/w), and homogenous mixture of n-butyl L-lactate with/without FFB was mixed by magnetic stirring to find the optimal type of cosurfactant and the ratio of surfactant/cosurfactant. Next, FFB was dissolved in n-butyl L-lactate, and surfactant/cosurfactant was mixed at a specific ratio; oil phase and surfactant/cosurfactant were accurately weighed (from 1:9 to 9:1) into glass vials. Then, the components were mixed by gentle stirring and vortex mixing, diluted with 500 times the volume of water in a dropwise manner, and visually observed for phase clarity and flow ability. After the identification of ME region in the phase diagrams, the ME formulations were selected at desired component ratios. The same pseudo-ternary phase diagrams of SMEPMS with the oil phase saturated with 50% or 100% of FFB were also constructed.
In situ formation and characterizations of FFB nanoparticles
One gram of optimal formulations selected from SMEPMS regions (oil phase saturated with FFB) was added dropwise into 10 mL of various saccharide solutions (lactose, mannitol, glucose, sucrose, and trehalose, either in a concentration of 5% or 10%) at a rate of 3.3 mL/minute. After addition, the mixture was vortexed for 3 minutes and allowed to sit for another 30 minutes, and 1 mL of mixture was withdrawn to measure the size and distribution of particles (N5 Submicron Particle Size Analyzer; Beckman Coulter, Brea, CA, USA) formed in situ (ie, particle sizes before freeze-drying [BFD]). The N5 Submicron Particle Size Analyzer uses Photon Correlation Spectroscopy (PCS), which determines particle size by measuring the rate of fluctuations in laser light intensity scattered by particles as they diffuse through a fluid. Following the same procedure as previously described, particle sizes of the five formulations without/with saturation of the oil phase with FFB after diluting with water were also measured for comparison (designated as the blank and control, respectively). After the measurement, the rest mixture was sonicated for 10 minutes and then freeze-dried (FD) for at least 24 hours. The morphology of the FD powder was observed by scanning electron microscopy (SEM S-2400; Hitachi Ltd, Tokyo, Japan). The specimens were first gold-coated using a sputter coater (Hitachi IB-2; Hitachi) prior to SEM. The crystallinity of the FD powder was characterized by an X-ray powder diffraction method (Powder X-ray diffractometer MiniFlex; Rigaku Corporation, Tokyo, Japan). X-ray diffraction allows one to know if the sample is crystallized. The particle size and distribution of the FD powder were also measured after being reconstituted for 30 and 90 minutes (designated AFD30 m and AFD90 m, respectively) in either an aqueous solution or a 0.5% w/w sodium lauryl sulfate (SLS) solution of 50 times its weight. The transition time (TT) was designated as the time elapsed for the formation of turbidity (appearing when the particle size exceeded 250 nm) after reconstituting the FD powder in an aqueous solution or a 0.5% w/w SLS solution. provides the scheme of SMEPMS preparation and characterizations.
Statistical analysis
All data were presented as mean ± standard deviation. The statistical significance of differences was performed using analysis of variance (ANOVA) test by SPSS statistic software (PASW Statistics 18.0; IBM Corporation, Armonk, NY, USA). Difference was considered significant when the P-value was less than 0.05.
Results and discussion
Construction of pseudo-ternary phase diagrams
The solubility of FFB in n-butyl L-lactate was about 0.2 g/g. The oral 50% lethal dose of n-butyl L-lactate is >5000 mg/kg in rats,Citation21 so it is considered as a safe oil phase in this study. A pseudo-ternary phase diagram of oil (n-butyl L-lactate)/surfactant (Tween 80)/cosurfactant (ethanol, 1-propanol, and PEG 600) with/without saturation of FFB in the oil phase was constructed to determine the SMEPMS region. Surfactant possesses polar and nonpolar portions, and when placed in an oil-water system, the polar groups are attracted to or orient toward the water, and the nonpolar groups are oriented toward the oil. The surfactant molecule lowers the interfacial tension between the oil and water phases so that spontaneous dispersion of water or oil droplets occurs and the system is thermodynamically stable. An effective way to further decrease interfacial tension is to include a second surface-active species (either a surfactant or medium-chain alcohol) that is a cosurfactant. Tween and PEG series, 1-propanol, and ethanol have been previously reported as either effective surfactants or cosurfactants.Citation11,Citation22–Citation25 For example, Wei et alCitation22 found that the use of Tween 80 with 40% content had minimum self-emulsification time. Patel and VaviaCitation23 developed the optimized formulation of fenofibrate for in vitro dissolution and pharmacodynamic studies that was composed of Labrafac® CM10 (Gattefosse, Mumbai, India) (31.5%), Tween 80 (47.3%), and PEG 400 (12.7%). Results are shown in –– without/with FFB saturation in the oil phase, respectively. Without FFB saturation in the oil phase, the SMEPMS (ie, ME; black area) regions for these three cosurfactants were all located at the bottom of the respective ternary diagrams and had similar areas. There was a region in which an E formed close to the corner of the oil phase when using PEG 600 as the cosurfactant. When the oil phase was saturated with FFB, the areas of the SMEPMS significantly decreased compared to that without FFB saturation for all these three cosurfactants, and some of them formed an E (gray area) system leading to an increase in the gray area. This phenomenon might be caused by the decreased interfacial tension between the oil phase and aqueous phase as a result of the solubilization of the hydrophobic FFB in the oil phase. Then the thermodynamic stability of the interface was affected according to Gibbs’ equation. The presence of FFB molecules in the interface affected the structure of the micelles and raised the free energy.
Figure 2 Phase diagrams of butyl lactate/surfactant (Tween 80)/cosurfactants.
Notes: Phase diagrams of butyl lactate/surfactant (Tween 80)/cosurfactants (including propanol [A and D], ethanol [B and E], and PEG 600 [C and F]) without (A–C) and with the addition of fenofibrate (D–F). Black area indicates the microemulsion region, while gray indicates the emulsion region.
![Figure 2 Phase diagrams of butyl lactate/surfactant (Tween 80)/cosurfactants.Notes: Phase diagrams of butyl lactate/surfactant (Tween 80)/cosurfactants (including propanol [A and D], ethanol [B and E], and PEG 600 [C and F]) without (A–C) and with the addition of fenofibrate (D–F). Black area indicates the microemulsion region, while gray indicates the emulsion region.](/cms/asset/a70b1a70-adcb-4d8b-a13e-f1bc45cc315d/dijn_a_48465_f0002_b.jpg)
SMEPMS using PEG 600 as the cosurfactant revealed a larger ME region area in the diagrams compared to other cosurfactants. Therefore, five SMEPMS formulations with cosurfactant of PEG 600 were selected as a template for preparing FFB nanoparticulates (the exact locations of these five SMEPMS formulations in the phase diagram are illustrated in ) and we chose five formulation points (ratio of butyl lactate, Tween 80, and PEG 600). The composition and dilution status with water (ME or E) for these five SMEPMS formulations are listed in . For comparison, all three cosurfactants, PEG 600 (Ape-Epe), propanol (Ap-Ep), and ethanol (Ae-Ee), were included in the following study of preparing nanoparticulates of FFB, and their compositions and dilution status with water are also listed in .
Table 1 Compositions of various formulations for SMEPMS
Preparation and size analysis of nanoparticles using SMEPMS as templates
It was preliminarily found that, when simply using water as the dilution phase, the reconstitution of FD powder was unable to form the desired nanoparticle size regardless of composition. Therefore, the effects of various saccharide solutions (L, lactose; M, mannitol; G, glucose; S, sucrose; or T, trehalose) at 5% (50) or 10% concentration (100) as anticaking agents present in the dilution aqueous phase during FD were compared. Results of five SMEPMS formulations as templates using PEG 600 (Ape-Epe), propanol (Ap-Ep), or ethanol (Ae-Ee) as the cosurfactant are illustrated in –, respectively (exemplifying those reconstituted in the 0.5% SLS solution). shows that particle sizes of the blank and control were within the nanosize range when PEG 600 was the cosurfactant. Hence, these five SMEPMS formulations selected as templates were capable of forming ME after being diluted with water; however, the particle sizes increased after diluting with all saccharide solutions (under the “BFD” column) compared to those diluting with water (as the control). Particle sizes also tended to increase with higher concentrations of saccharide in the dilution, especially for dilution of 10% lactose solution (L100). Nevertheless, most of those still maintained their particle sizes within the nanosize range, except when diluted with the 10% lactose solution (excluding Dpe) and with Ape diluted with the 10% glucose solution.
Table 2 Particle size analysis of self-microemulsifying premicroemulsion systems using PEG 600 as the cosurfactant
Table 3 Particle size analysis of self-microemulsifying premicroemulsion systems using propanol as the cosurfactant
Table 4 Particle size analysis of self-microemulsifying premicroemulsion systems using ethanol as the cosurfactant
After the process of freeze-drying, these FD FFB samples were reconstituted in either deionized (DI) water or a 0.5% SLS solution, and their particle sizes were measured at 30 and 90 minutes after reconstitution. The transition time (TT) elapsed before the appearance of turbidity after reconstitution was recorded and designated TT. TT means that, after reconstitution, the time when the nanosized FD FFB (particle size <250 nm) precipitated or recrystallized is long enough to turn the solution turbid. All these results are respectively given under the columns of AFD30 m, AFD90 m, and TT in . TT of >0.0 indicated the formation of FFB nanoparticles after FD, and a longer TT implied a more stable FFB nanoparticle after dispersion. TT data in demonstrate that SMEPMS using PEG 600 as the cosurfactant could be utilized as templates to form FFB nanoparticles in the five saccharide solutions, and nanoparticles were recovered after FD. Furthermore, reconstitution of the FD FFB nanoparticles produced in these five saccharide solutions in the 0.5% SLS solution led to longer TT compared to that in DI water, which indicates that the addition of SLS may have had a solubilization effect on these FFB nanoparticles. Among the five saccharide solutions, the enhanced stability of the resultant FFB nanoparticles in the 5% glucose solution was the most profound. An increasing concentration of saccharide did not improve the stability of FFB nanoparticles when dispersed in the corresponding solution. Furthermore, it turned out that 0.5% SLS also maintained FFB nanoparticles within the nanosize range in the reconstitution solution for as long as 90 minutes (marked with deep-colored shading). This stabilization effect was most effective for FFB FD nanoparticles from the 5% glucose solution using Ape, C pe, D pe, and Epe SMEPMS as templates (but not Bpe) and from the 5% sucrose solution using Cpe, Dpe, and Epe SMEPMS as templates (but not Ape or Bpe). This phenomenon might be explained by the Cpe, Dpe, and Epe SMEPMS formulations with PEG 600 as the cosurfactant forming MEs after dilution with the aqueous phase as indicated in , where Ape and Bpe SMEPMS formulations were only able to form Es. It was concluded that using SMEPMS formulations with PEG 600 as the cosurfactant as templates was able to produce FFB nanoparticles in the presence of a suitable saccharide as an anticaking agent and FD process.
and further list the particle sizes of the blank and control using five SMEPMS formulations with propanol or ethanol as the cosurfactant, respectively. After diluting these five SMEPMS formulations with either propanol or ethanol as the cosurfactant in all saccharide solutions, TT values of the FD FFB samples reconstituted in either DI water or the 0.5% SLS solution and their particle sizes, measured at 30 and 90 minutes after reconstitution, are also shown in and . gives the results of using three SMEPMS formulations (F versus A, G versus B, and H versus C) with Tween 20 as the surfactant and PEG 600 as the cosurfactant as templates to produce FFB nanoparticles. Similar to the five SMEPMS formulations with PEG 600 as the cosurfactant, nanoemulsions formed from the blank and control group, and the particle sizes still fell within the nanosize range after dilution with most of the saccharide solutions before FD. However, TT values in and indicate that there were quite a few cases of FD FFB particles remaining as nanoparticles after FD. Particle sizes of most of those FD FFB particles after reconstitution in either DI water or the 0.5% surfactant did not form nanoparticles. It was concluded that SMEPMS formulations with Tween 80 as the surfactant and PEG 600 as the cosurfactant were optimal templates to produce FFB nanoparticles.
Characterization of FD nanosized FFB
shows SEM images of Dpe with four saccharide solutions as the solid carrier. Dpe with 5% mannitol and lactose (data not shown) had fewer amorphous forms, whereas that with 10% mannitol had fewer crystalline needles and better particle dispersion. The presence of saccharide might inhibit the formation of drug crystals, and the extent of inhibition of the lattice arrangement of drug crystals with various saccharides differed. The formulation with trehalose caused columnar crystals and poor redispersibility. Results of powder X-ray diffractometry were consistent with those of the particle size analysis. The peak intensity of FD nanosized FFB was much lower than that of the pure saccharide solution as shown in , and a rearrangement of the lattice structure during the process of dilution might have contributed. Compared to the peak of FFB, the lower peak intensity of FD nanosized FFB indicates that the FFB formed amorphous nanoparticles through the use of surfactants, cosurfactants, and the added saccharides.
Figure 4 Scanning electron microscopic images of freeze-dried formulations of DpeNotes: (A) 5% mannitol, (B) 10% mannitol, (C) 10% lactose, and (D) 10% trehalose solutions (Zoom ratio: 1000).
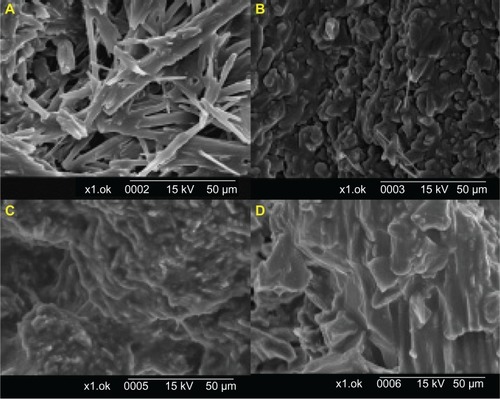
Figure 5 Powder X-ray diffractometry.
Notes: (A) fenofibrate only; (B) lactose only; Dpe freeze-dried with a 5% (B1) or 10% (B2) lactose solution; (C) mannitol only; Dpe freeze-dried with a 5% (C1) or 10% (C2) mannitol solution; (D) sucrose only; Dpe freeze-dried with a 5% (D1) or 10% (D2) sucrose solution; (E) trehalose only; Dpe freeze-dried with a 5% (E1) or 10% (E2) trehalose solution.
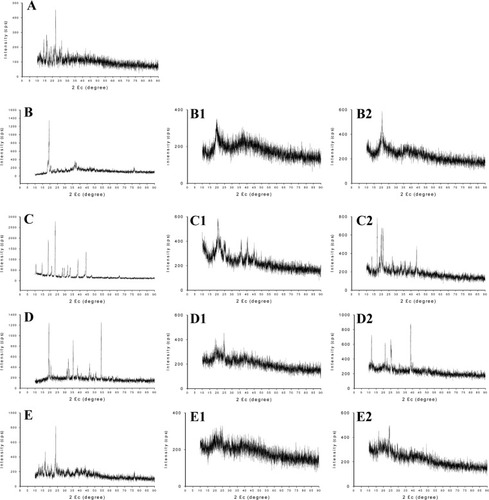
Conclusion
FFB saturated in n-butyl L-lactate, Tween 80, and a number of cosurfactants (ethanol, 1-propanol, and PEG 600) and then diluted with saccharide solutions prior to the FD process was developed. It was concluded that, by using SMEPMS formulations with PEG 600 as the cosurfactant, FFB nanoparticles were able to be produced, and FD in the presence of a suitable saccharide as an anticaking agent was necessary to maintain the particle size stability of the reconstituted powder. Our studies indicate that the potential utilization of SMEPMS for the delivery of poorly soluble drugs, such as FFB, improve its bioavailability; furthermore, the use of lyophilization and the addition of saccharide made an FFB oral solid nanoparticle dosage form with easy administration. The SMEPMS templates establish a database about monodispersed sizes of nanoparticles (inorganic or organic) by varying the size of the ME droplet radius, allowing one to use the information to design orally active dosage form.
Disclosure
The authors report no conflicts of interest in this work.
References
- LipinskiCALombardoFDominyBWFeeneyPJExperimental and computational approaches to estimate solubility and permeability in drug discovery and development settingsAdv Drug Deliv Rev2000461–332611259830
- PoutonCWFormulation of poorly water-soluble drugs for oral administration: physicochemical and physiological issues and the lipid formulation classification systemEur J Pharm Sci2006293–427828716815001
- MunozAGuichardJPReginaultPMicronised fenofibrateAtherosclerosis1994110SupplS45S487857384
- HoarTPSchulmanJHTransparent water-in-oil dispersions: The oleopathic hydro-micelleNature19431523847102103
- SpernathAAserinAMicroemulsions as carriers for drugs and nutraceuticalsAdv Colloid Interface Sci20061281304764
- StuchlíkMZákSLipid-based vehicle for oral drug deliveryBiomed Pap Med Fac Univ Palacky Olomouc Czech Repub20011452172612426768
- PoutonCWLipid formulations for oral administration of drugs: non-emulsifying, self-emulsifying and ‘self-microemulsifying’ drug delivery systemsEur J Pharm Sci200011Suppl 2S93S9811033431
- ConstantinidesPPLipid microemulsions for improving drug dissolution and oral absorption: physical and biopharmaceutical aspectsPharm Res19951211156115728592652
- KhooSMHumberstoneAJPorterCJHEdwardsGACharmanWNFormulation design and bioavailability assessment of lipidic self-emulsifying formulations of halofantrineInt J Pharm19981671–2155164
- FrimanSBäckmanLA new microemulsion formulation of cyclosporin: pharmacokinetic and clinical featuresClin Pharmacokinet19963031811938882300
- KimJYKuYSEnhanced absorption of indomethacin after oral or rectal administration of a self-emulsifying system containing indomethacin to ratsInt J Pharm20001941818910601687
- KangBKLeeJSChonSKDevelopment of self-microemulsifying drug delivery systems (SMEDDS) for oral bioavailability enhancement of simvastatin in beagle dogsInt J Pharm20042741–2657315072783
- ChenYLiGWuXSelf-microemulsifying drug delivery system (SMEDDS) of vinpocetine: formulation development and in vivo assessmentBiol Pharm Bull200831111812518175953
- WooJSSongYKHongJYLimSJKimCKReduced food-effect and enhanced bioavailability of a self-microemulsifying formulation of itraconazole in healthy volunteersEur J Pharm Sci200833215916518178070
- FatourosDGNielsenFSDouroumisDHadjileontiadisLJMullertzAIn vitro-in vivo correlations of self-emulsifying drug delivery systems combining the dynamic lipolysis model and neuro-fuzzy networksEur J Pharm Biopharm200869388789818367386
- CooneyGFJeevanandamVChoudhurySFeutrenGMuellerEAEisenHJComparative bioavailability of neoral and sandimmune in cardiac transplant recipients over 1 yearTransplant Proc1998305189218949723323
- VenkateshGMajidMIAMansorSMNairNKCroftSLNavaratnamVIn vitro and in vivo evaluation of self-microemulsifying drug delivery system of buparvaquoneDrug Dev Ind Pharm201036673574520136493
- NazzalSKhanMAControlled release of a self-emulsifying formulation from a tablet dosage form: stability assessment and optimization of some processing parametersInt J Pharm20063151–211012116563673
- TrottaMGallarateMPattarinoFMorelSEmulsions containing partially water-miscible solvents for the preparation of drug nanosuspensionsJ Control Release2001761–211912811532318
- TrottaMGallarateMCarlottiMEMorelSPreparation of griseofulvin nanoparticles from water-dilutable microemulsionsInt J Pharm2003254223524212623199
- ClaryJJFeronVJvan VelthuijsenJASafety assessment of lactate estersRegul Toxicol Pharmacol199827288979671563
- WeiLSunPNieSPanWPreparation and evaluation of SEDDS and SMEDDS containing carvedilolDrug Dev Ind Pharm200531878579416221613
- PatelARVaviaPRPreparation and in vivo evaluation of SMEDDS (self-microemulsifying drug delivery system) containing fenofibrateAAPS J200793E344E35218170981
- ShenHZhongMPreparation and evaluation of self-microemulsifying drug delivery systems (SMEDDS) containing atorvastatinJ Pharm Pharmacol20065891183119116945176
- KoganAAserinAGartiNImproved solubilization of carbamazepine and structural transitions in nonionic microemulsions upon aqueous phase dilutionJ Colloid Interface Sci2007315263764717825310