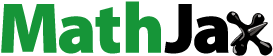
Abstract
To generate a good carrier for gene transfection, O-carboxymethyl chitosan-graft-branched polyethylenimine (OCMPEI) copolymers were synthesized by increasing the weight percentage of branched polyethylenimine conjugated to the carboxyl groups of O-carboxymethyl chitosan. These spherical polyplexes with plasmid deoxyribonucleic acid (pDNA) or small interfering ribonucleic acid (siRNA) had diameters of ∼200–300 nm or ∼10–25 nm, respectively, and displayed significant transfection efficiency in normal and tumor cells. In particular, expression of green fluorescent protein (GFP) following pDNA transfection was effectively suppressed by delivery of GFP-specific siRNA with the same copolymer. The optimized copolymer and polyplexes were nontoxic in vitro and in vivo. The use of endocytosis inhibitors to investigate the mechanisms of transfection of the polyplexes suggested the involvement of macropinocytosis. An in vivo study in mice showed excellent GFP expression in the lung, kidney, and liver. The results demonstrated that the OCMPEI copolymer prepared in this study is a promising carrier for in vitro and in vivo gene delivery applications.
Introduction
Gene therapy has been regarded to show promise for curing serious diseases, such as cancer, immune deficiency disorders such as acquired immune deficiency syndrome, cystic fibrosis, heart disease, diabetes, and hemophilia. Viral gene vectors remain the most popular vehicle for gene therapy in clinical trials owing to their high transfection efficiency, although their high immunogenic and poor circulatory properties restrict their widespread use. In contrast to viral vectors, nonviral gene delivery vectors have attracted great attention because of their biosafety and easy modification.Citation1–Citation5 They have been divided into two types: liposome-based gene carriers (lipoplexes) and polymer-based gene carriers (polyplexes).Citation6 Although lipoplexes often showed high transgene expression, their limited use resulted from their nonspecific membrane activity.Citation7–Citation9 A number of cationic polymers have been developed because they can interact with the anionic phosphate groups of naked DNA or RNA, generating compact complexes.Citation7–Citation10 Among these, polyethyleneimine (PEI) and chitosan have been widely studied, given their attractive properties for drug delivery.Citation11–Citation13
PEI is a promising synthetic vector for gene delivery because its high cationicity allows it to condense and protect nucleic acids (plasmid DNA [pDNA] or small interfering RNA [siRNA]) from nuclease digestion, as well as enabling endosomal escape via the “proton sponge” effect.Citation14,Citation15 The transfection efficiencies and toxicities of PEIs differ in a mass-dependent or structure-dependent manner, and it is difficult to define the relationships between theses parameters.Citation16,Citation17 Therefore, the application of PEI is limited by its high toxicity and proinflammatory effects.Citation18
The natural polysaccharide chitosan has been recognized as a safer carrier, given its biodegradability, lack of toxicity, limited immunogenicity, and biocompatibility.Citation19,Citation20 Above all, the polycationic character of chitosan has attracted many researchers in the field of gene therapy to use chitosan and its derivatives for applications as diverse as drug delivery,Citation19–Citation21 tissue engineering,Citation22–Citation24 antibiotic delivery,Citation25,Citation26 and the preparation of chitosan-based biomaterials.Citation27,Citation28
Several approaches that enable the chemical combination of chitosan and PEI to promote gene delivery were reported recently.Citation29–Citation34 In the present study, O-carboxymethyl chitosan-graft-branched polyethylenimine (OCMPEI) copolymers with various ratios of branched polyethylenimine (bPEI) were synthesized, and their suitability for the delivery of pDNA or siRNA was evaluated. The copolymers were investigated with respect to their biophysical characters, capacity to condense with nucleic acids, cytotoxicity, cellular uptake, and transfection efficiency. Moreover, both the in vitro gene silencing efficacy of OCMPEI-siRNA polyplexes and in vivo gene expression of OCMPEI/pDNA polyplexes were evaluated in cancer cells and mice, respectively. Inhibitors of endocytosis were used to investigate the cellular delivery mechanism of polyplexes.
Materials and methods
Materials
Plasmid DNA (pGL3 vector) that encodes a modified firefly luciferase and a Luciferase Assay System were purchased from Promega (Madison, WI, USA). Plasmid DNA (pEGFP-C1) that encodes green fluorescent protein (GFP) was obtained from BD Biosciences (Palo Alto, CA, USA). The siRNA for targeted silencing of GFP (siGFP) and scrambled siGFP RNA were purchased from Bioneer Co (Daejeon, Republic of Korea). The BCA Protein Assay Kit was from Pierce Chemical (Rockford, IL, USA). Water soluble chitosan (molecular weight, 12,000 Da; deacetylation degree, 97.0%) was provided by Chittolife Co, Ltd (Pyeongtaek, Republic of Korea). Branched PEI (25 kDa), 3-(4,5-dimethylthiazol-2-yl)-2,5-diphenyltetrazolium bromide (MTT), 1-ethyl-3-(30dimethylaminopropyl) carbodiimide hydrochloride (EDC), N-hydroxysuccinimide (NHS), and other chemicals were purchased from Sigma-Aldrich (St Louis, MO, USA).
Preparation of OCMCH
Low molecular weight water soluble chitosan (LMWSC) was prepared as described previously.Citation35 O-carboxymethyl chitosan (OCMCh) was synthesized as described previously, with some modification.Citation36 Briefly, 2 g of LMWSC was immersed in 25 mL of 50% (w/v) NaOH solution and alkalized for 24 hours. The alkalized LMWSC was filtered and then transferred into a flask, after which 5 g of monochloroacetic acid in isopropanol was added in a dropwise manner for 20 minutes. This flask was allowed to react for a further 8 hours at room temperature (RT). The reactants were filtered to remove the solvent, and the filtrates were then dissolved in 100 mL of water, thereafter the pH was adjusted to 7.0 using 2 N HCl. The product was precipitated by ethanol and the precipitates were harvested by filtration. Residual ethanol was removed by vacuum drying.
Phthaloylation of OCMCH
One gram of phthalic anhydride was dissolved in 10 mL of N,N-dimethylformamide containing 5% (v/v) water, followed by the addition of 0.36 g of OCMCh. The mixture was heated under nitrogen at 120°C while gently stirring overnight. After reaction, the phthaloylated chitosan solution was cooled at RT and then left in ice-cold water. The precipitates were harvested by filtration and washed with methanol. Residual methanol was removed by vacuum-drying. This amine protection was analyzed by using 1H nuclear magnetic resonance (1H-NMR; Bruker, Karlsruhe, Germany; 400 MHz) and Fourier transform infrared spectroscopy.
Preparation of phthaloylated OCMPEI
Phthaloylated OCMPEI copolymers with different degrees of grafting of 25 kDa bPEI to carboxyl groups of the phthaloylated OCMCh backbone were generated by chemical synthesis. Briefly, phthaloylated OCMCh solution was activated by the addition of EDC and NHS at 1:2:2 molar ratios in 50 mM 4-(2-hydroxyethyl)-1-piperazineethanesulfonic acid (pH 7.0) buffer containing 40% (v/v) dimethyl sulfoxide for 1 hour at RT, after which a solution of 5%, 10%, 15%, or 20% bPEI (weight %) was added, gently stirred, and then incubated for 24 hours at RT. After reaction, the solution of phthaloylated OCMPEI was dialyzed against distilled water for 2 days; the resulting solution was freeze-dried.
Deprotection of phthaloylated OCMPEI
Phthaloylated OCMPEI (200 mg) was mixed in 50 mL distilled water with 30 mL of hydrazine monohydrate using gentle magnetic stirring overnight at 100°C; the mixture solution was then poured into 500 mL of ice water. Excess hydrazine monohydrate in the mixture solution was removed by evaporating using a rotary evaporator. The final product was lyophilized under a vacuum to obtain the deprotected OCMPEI. Synthesis of copolymers was estimated by measuring 1H-NMR (Bruker, 400 MHz, D2O).
Animals for in vivo gene transfection studies
Six-week-old male slc:ICR mice (25 ± 2 g, SLC Japan Inc, Shizuoka, Japan) were used to investigate GFP gene expression in vivo. Mice were housed separately (2 animals per cage) under conditions of controlled temperature (23°C ± 2°C), humidity (50% ± 15%), and lighting (lights on from 8 am to 8 pm, illuminance of 150∼300 Lux). All animal studies were approved by the Animal Care Committee of Sunchon National University (SCNU_IACUC-2013-6), and all animal care was in accordance with the guidelines of the Korea Council on Animal Care.
pDNA/siRNA gel retardation assay
pEGFP-C1 plasmid was transformed into Escherichia coli JM109, and the cells were propagated in Luria-Bertani (LB) media at 37°C overnight. The plasmid was purified using a plasmid extraction kit (Exprep™ Quick, GeneAll Biotechnology Co, Seoul, Republic of Korea) according to the manufacturer’s protocol. The purity of the pDNA was assessed by determining the OD260/OD280 absorbance ratio. The GFP siRNA (sense: 5′-GCAUCAAGGUGAACUUCAAdTdT-3′; antisense: 5′-UUGAAGUUCACCUUGAUGCdTdT-3′), and scrambled siRNA (sense: 5′-CUACGCCACCA-AUUUCGUdTdT-3′; antisense: 5′-ACGAAAUUGGUG-GCGUAGGdTdT-3′) were obtained from Bioneer Co (Daejeon, Republic of Korea). They were stored at −20°C until use.
Both pDNA and siRNA polyplexes were prepared by mixing ∼400–500 ng of pDNA or 500 ng of siRNA and different amounts of OCMPEIs in PBS (phosphate-buffered saline; pH 7.4) at the appropriate concentrations to yield different weight ratios. The products were further incubated for 30 minutes at 25°C. All of the polyplexes were electrophoresed (100V, 50 minutes) in 0.8% (w/v) agarose gel, stained with ethidium bromide (EtBr) and visualized on an UV transilluminator using a gel documentation system (Bio-Rad Laboratories, Hercules, CA, USA).
DNA protection and release assay
The capacity of OCMPEI to protect DNA from degradation was assessed by adding DNase after the formation of polyplexes. Briefly, 1.5 μL of RNase-Free DNase I (1 U/μL in buffer containing 100 mM Tris, 25 mM MgCl2, 5 mM CaCl2) was incubated with the indicated polyplexes for 1 hour at 37°C. After incubation, 5 μL of EDTA (ethylenediaminetetraacetic acid) solution (100 mM) was added and the mixture was incubated at 75°C for 10 minutes to inactivate DNase. The samples were electrophoresed through a 0.8% agarose gel and stained using EtBr.
The pDNA were released from polyplexes by treatment with heparin. Briefly, OCMPEI/pDNA complexes were prepared at various weight ratios, ranging from 1 to 16 in PBS (pH 7.4) and incubated for 30 minutes at RT before adding heparin (10 U). The samples were incubated for 20 minutes and electrophoresed in 0.8% agarose gel. Gels stained with EtBr were visualized on an UV transilluminator.
Measurement of the sizes of polyplexes
The particle sizes of polyplexes (OCMPEI/pDNA complex) were measured using a dynamic light scattering (DLS) instrument (ELS-8000 electrophoretic LS spectrophotometer, Otsuka Electronics, Osaka, Japan), fitted with a He-Ne laser at a fixed scattering angle of 90°. For each sample, 10 μg of pEGFP-C1 was added to 90 μL of OCMPEIs at a weight ratio of 1:16 in PBS (pH 7.4); the mixtures were incubated for 30 minutes at 25°C, followed by the addition of 300 μL PBS.
Morphologies of polyplexes
Both pDNA and siRNA-containing polyplexes were prepared at an OCMPEI concentration of 0.1 mg/mL. Polyplexes (6 μL) were applied to glow-discharged carbon-coated copper grids for 1 minute. The grids were rinsed three times in distilled water and stained with 2% (w/v) uranyl acetate. Electron microscopic images were then recorded using an FEI Tecnai G2 transmission electron microscope (Hillsboro, OR USA) with an accelerating voltage of 200 kV operated in the low-dose mode.
Cell culture
To examine cell viability and in vitro transfection efficiency, HEK293 (human embryonic kidney 293), HCT119 (colon cancer), U937 (human histiocytic leukemia), LoVo (colon cancer), L929 (mouse connective tissue), and G361 (human Caucasian malignant melanoma) cells obtained from Korean Cell Line Bank (Seoul, Republic of Korea) were cultured in either Dulbecco’s Modified Eagle Medium or RPMI-1640 (Roswell Park Memorial Institute 1640) medium. Both media were supplemented with antibiotics (100 U/mL penicillin, 100 μg/mL streptomycin, and 10 μg/mL plasmocin) and 10% fetal calf serum. All cells were grown at 37°C in a humidified atmosphere containing 5% CO2.
Cytotoxicity and hemolysis
The cytotoxicity of OCMPEI and OCMPEI-pDNA complexes were evaluated using an MTT (3-(4,5-dimethylthiazol-2-yl)-2,5-diphenyltetrazolium bromide) assay to measure cell viability. A total of 5 × 103 cells/well of HEK 293 or L929 cells were seeded into a 96-well plate and incubated for 24 hours. The OCMPEI and OCMPEI-pDNA complexes were diluted in serial with Opti-MEM medium, and then added to each plate and incubated for 24 hours at 37°C. After the incubation, 10 μL of MTT solution (5 mg/mL in PBS) was added to each well, and the cells were incubated for another 4 hours at 37°C. Ten microliters of 5 mg/mL MTT was added to each well and incubated for an additional 4 hours. The supernatants were aspirated and 100 μL of dimethyl sulfoxide was added to the wells in order to dissolve any remaining precipitate. Absorbance was then measured at 570 nm (optical density) and 670 nm (subtract background) using a microtiter reader, Finally, the absorbance was measured at wavelength of 570 nm (optical density) and 670 nm (subtract background). The relative cell viability (%) was calculated according to the following equation:
In order to investigate in vitro cytotoxicity of polyplexes in rat red blood cells (rRBCs), hemolysis was assayed with blood collected from rats. Heparinized fresh blood was centrifuged at 800g and the collected rRBCs were washed with PBS (pH 7.4) until the supernatant was clear. Polyplexes in PBS were plated and rRBCs were then added to a final concentration of 8% (v/v) in a 96-well plate. The sample was incubated with mild agitation for 1 hour at 37°C. The samples were then centrifuged at 800g for 10 minutes. The absorbance of the supernatant was measured at 414 nm, each measurement was made in triplicate, and percentage hemolysis was calculated using followed equation:
100% hemolysis is defined as the absorbance of rRBCs containing 0.1% Triton X-100 and zero hemolysis consisted of rRBCs alone in PBS.
In vitro transfection
In vitro transfection of naked DNA (pEGFP-C1, 2 μg) or siRNA (0.3∼1 μg), commercial transfection reagents, and OCMCh, bPEI, and OCMPEI with pDNA or siRNA in the absence of serum was performed on various cell lines. The cells were plated in 24-well culture plates at a density of 5 × 104 cells/well and grown in antibiotic-free medium for 24 hours before the treatment. Before transfection, media were removed and the cells were washed with serum-free media, after which the samples in reduced-serum media (Opti-MEM, Life Technologies, Carlsbad CA, USA) were treated at desired weight ratios into each well. In particular, siRNA was labeled with propidium iodide (PI) dye in order to observe intracellular uptake using a fluorescent microscope. After 4 hours, serum was added to each well of the plate at 10% (v/v) and the cells were further cultivated for 12 hours. Then, media were replaced with media containing antibiotic and FBS and the cells were cultivated for an additional ∼1–2 days. Gene transfection was visualized using fluorescence microscopy.
Luciferase assay
To evaluate the transfection efficiencies of polyplexes with pDNA, pGL3 (1 μg) was complexed with OCMCh, bPEI, and OCMPEIs at various weight ratios, and in vitro transfection was performed as described above. After 2 days, the luciferase assay was performed according to the manufacturer’s protocols. Relative light units were measured at 570 nm. Protein quantification was determined by the bicinchoninic acid method to normalize relative light unit/protein concentration in the cell extracts. Each transfection experiment was carried out in triplicate and transfection efficiency was expressed as relative light units.
Gene silencing with GFP siRNA
Gene silencing assays were performed in previously transfected pEGFP-C1 HCT119 cells using either 300 μg or 600 μg of GFP-specific siRNA or scrambled siRNA. After 1 or 2 days, downregulation of GFP expression via transfection of GFP siRNA was investigated by fluorescence microscopy and Western blotting analysis. The proteins extracted from cells were separated on a 12% SDS-PAGE gel, and Western blot analysis was performed using antibodies specific to GFP (1:1,000, ab290; Abcam, Cambridge, UK) and glyceraldehyde 3-phosphate dehydrogenase (1:1,000, ab9484; Abcam) using the standard method.
In vivo transfection and expression of GFP
After mixing pEGFP (30 μg) with 15% OCMPEI at a weight ratio of 32, the polyplexes were incubated for 30 minutes at 25°C. The final volume was adjusted to 100 μL using PBS, and the polyplexes were injected into the tail veins of ICR mice (6-week-old males, 25 ± 2 g). The experiment was performed independently in triplicate. The mice were fed a normal diet for 5 days, and were then anesthetized to obtain in vivo images. They were then sacrificed and the vital organs were removed and washed with chilled PBS. The organs were also fluorescently imaged using an ImageQuant LAS 4000 instrument (GE Healthcare Bio-Sciences Corp, Piscataway, NJ, USA).
Endocytic mechanism of pDNA/OCMPEI polyplexes
To investigate whether endocytic pathways participate in the uptake of pDNA-containing polyplexes, the effects of endocytosis inhibitors was investigated in HCT 119 cells. The cells were seeded in 6-well plates at 1 × 106 cells/well and incubated overnight prior to assay. The cells were then preincubated separately with 10 μg/mL chlorpromazine, 3 μg/mL sodium azide, 8 μg/mL colchicine, and 6 μg/mL indomethacin for 1 hour, after which time the media was replaced with pEGFP-OCMPEI polyplexes containing the endocytic inhibitors mentioned above. After a 4-hour incubation, the media was replaced with fresh media with FBS and antibiotics. The cells were incubated for an additional 24 hours, harvested and analyzed using a FACSCalibur flow cytometer.
Statistical analysis
All the data are presented as the mean ± standard error. The statistical analysis of the results was analyzed by Student’s t-test. A P-value of <0.05 was considered to represent a significant difference.
Results and discussion
Synthesis and characterization of the OCMPEI copolymer
The choice of the gene carrier used for nonviral gene delivery or gene therapy is a critical consideration to ensure high transfection efficiency and low cytotoxicity. Although chitosan has received attention as a biodegradable and biocompatible polycationic nonviral vector, its low transfection efficiency has been an obstacle for its application as a gene delivery vector.Citation13 Features of chitosan that have limited in vitro or in vivo applications include its ability to bind strongly to nucleic acids, leading to a delay in the release of nucleic acids from endosomes to the cytoplasm.Citation37,Citation38 This gradually leads to degradation of the nucleic acids by cytosolic enzymes,Citation39 and suggests that a good gene delivery system is essential for a rapid release of the gene of interest from the carrier. Although high molecular weight bPEI is also recognized as a good potential gene carrier, its use has also been limited owing to its high cytotoxicityCitation14,Citation15 In order to complement these limitations, we synthesized OCMCh-graft-branched PEI (OCMPEI) copolymers which had an increasing amount of bPEI conjugated to the carboxyl groups of OCMCh.
As shown in , the C6-OH position on LMWSC was first modified to a carboxymethyl group by monochloroacetic acid which generated OCMCh. The free amine group in OCMCh was protected by phthalic anhydride and the phthaloylated-OCMPEI copolymers were synthesized by forming an amide bond between activated carboxyl groups in phthaloylated-OCMCh and bPEI amine groups. OCMPEI with free amine groups was prepared by a deprotecting procedure (). The synthesized OCMCh, 10% OCMPEI copolymer, and bPEI were analyzed by 1H-NMR (), and characterized as follows: OCMCh 1H-NMR (D2O, ppm, TMS); δ = 4.9 ppm (1, CH of C1 position in OCMCh), δ = 3.9 ppm (7, CH of C7 position OCMCh), δ = 3.0∼3.8 ppm (2, 3, 4, 5, and 6, CH, CH2 in C2, C3, C4, C5, and C6 position of OCMCh) and δ = 2.3∼2.8 ppm (a, b, c, and d CH in bPEI). In addition, copolymers with different bPEI weight ratio percentage values increased the characteristic chemical shifts of bPEI according to the amount of bPEI grafted (). This suggests that synthesis of the OCMPEI copolymers was successful. The OCMPEI copolymers significantly enhanced the transfection efficiencies of pDNA and siRNA without problematic levels of cytotoxicity.
Figure 1 Proposed reaction scheme for synthesis of OCMPEI copolymer.
Notes: LMWSC was modified to OCMCh (A) by monochloroacetic acid. OCMPEI copolymers (B) were synthesized by a chemically coupling reaction between EDC/NHS-activated carboxyl groups of OCMCh and amine groups of bPEI.
Abbreviations: bPEI, branched polyethylenimine; DMF, dimethylformamide; EDC, 1-ethyl-3-(30dimethylaminopropyl) carbodiimide hydrochloride; LMWSC, low molecular weight water soluble chitosan; NHS, N-hydroxysuccinimide; OCMCh, O-carboxymethyl chitosan; OCMPEI, O-carboxymethyl chitosan-graft-branched polyethylenimine.
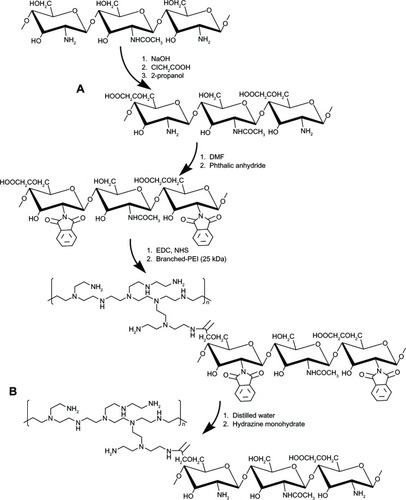
Figure 2 1H-NMR of OCMCh (A), bPEI (B), and OCMPEI (C).
Note: Numbers 1–7 and letters a–f indicate peaks of OCMCh and PEI, respectively.
Abbreviations: bPEI, branched polyethylenimine; NMR, nuclear magnetic resonance; OCMCh, O-carboxymethyl chitosan; OCMPEI, O-carboxymethyl chitosan-graft-branched polyethylenimine.
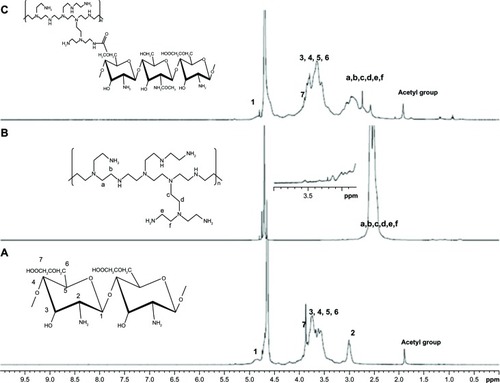
Characterization of pDNA/OCMPEI polyplexes
The ability of the OCMPEI copolymers to bind pDNA was determined using an electrophoretic mobility shift assay. shows the condensation of DNA with 5%, 10%, 15%, and 20% OCMPEI copolymers in various weight ratios. Whereas free pDNA (, control) showed the distinct bands, which corresponded with the supercoiled circular, and nicked forms of the plasmid electrophoretic migration of pDNA complexed with OCMPEI copolymers was dose-dependently retarded in grafting ratios ranging from 5% to 15% due to enhanced positive charge in OCMPEI copolymers. Interestingly, the retardation pattern of 20% pDNA/OCMPEI polyplexes was different from the others, in that the highly and lowly condensed bands appeared together. This result was consistent with size distributions of 20% OCMPEI, shown in . We suggest that DNA binding pattern of 20% OCMPEI is due to its stronger positive charge and DNA forms. For example, because its binding to DNA is very fast upon strong electrostatic interaction, the size of the polyplexes is small and excess polymers are unable to bind with the polyplexes due to repulsive force.
Figure 3 Gel retardation assay of the pDNA/OCMPEI polyplexes.
Notes: (A) Electrophoretic mobility shift assay of pDNA/OCMPEI polyplexes at weight ratios. (B) DNase protection assay of pDNA/OCMPEI polyplexes (w/w: 1:1 and 1:2) when treated with DNase I. (C) DNA-releasing assay from pDNA/OCMPEI polyplexes when treated with heparin.
Abbreviations: DNA, deoxyribonucleic acid; OCMPEI, O-carboxymethyl chitosan-graft-branched polyethylenimine; pDNA, plasmid DNA.
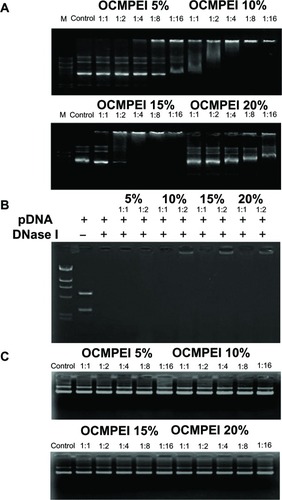
Figure 4 Particle size and morphological observation of pDNA/OCMPEI polyplexes.
Notes: (A) pDNA was complexed with OCMPEI 5% (1), 10% (2), 15% (3), and 20% (4) at a w/w ratio of 16 and analyzed by DLS. (B) spherical forms of pDNA/OCMPEI polyplexes were observed by using transmission electron microscope; (1–4) are the same percentages as in (A).
Abbreviations: DLS, dynamic light scattering; OCMPEI, O-carboxymethyl chitosan-graft-branched polyethylenimine; pDNA, plasmid deoxyribonucleic acid.
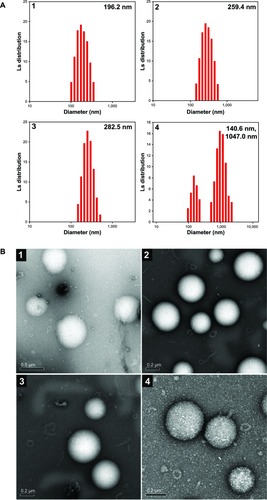
To protect the bound pDNA from digestion with nucleases, polyplexes that were complexed at w/w ratios of 1 and 2 were treated with DNase I for 1 hour. As shown in , the complete degradation of naked pDNA and pDNA with 5% OCMPEI by the enzyme indicated the resistance of pDNA to digestion by nucleases. However, other polyplexes presented the distinguishable protecting ability of bound pDNA from DNase I at a w/w ratio of 2. If the surface of DNA is surrounded by copolymers, or DNase cleavable sites of DNA are occupied at low concentrations, polymers can protect from DNase attack sufficiently. These results proposed that OCMPEI copolymers, even in low concentration, have the ability to protect the bound pDNA from degradation by nucleases present in blood and other body fluids when pDNA-carrying polyplexes are used in vivo.
Given the importance of effective release of pDNA from polyplexes for efficient transfection, to deliver genes to be expressed within cells,Citation31 we confirmed the release of pDNA after incubation with the anionic polysaccharide heparin (). Interestingly, pDNA at all w/w ratios was in the same position with free pDNA, indicating complete release of pDNA from copolymers. This may indicate an easy endosomal escape of pDNA when polyplexes are delivered into cells.
Sizes and morphologies of pDNA-containing polyplexes
To investigate the size distributions and morphologies of pDNA-containing polyplexes, pDNA was complexed with OCMPEI copolymers at a w/w ratio of 1:16, and the polyplexes were analyzed using DLS and transmission electron microscopy (TEM). As shown in , the unimodal sizes of polyplexes with 5%, 10%, and 15% OCMPEI at a w/w ratio of 16 ranged from ∼200–300 nm, whereas the average dimodal size of polyplexes with 20% OCMPEI was 408 nm, which is consistent with the DNA migration pattern of . The morphologies and sizes of polyplexes were also visualized using TEM (), which revealed them to have a regular spherical shape. The sizes that were estimated using TEM are similar to those estimated using DLS.
Cytotoxicity of the OCMPEI copolymer and pDNA/OCMPEI polyplexes
An MTT assay was performed on HEK293 cells to assess the in vitro toxicity of OCMPEI copolymers. Assessment of the viability of cells after treatment with various levels of OCMPEIs indicated that free bPEI was more cytotoxic than either OCMCh or OCMPEI copolymers (). Furthermore, we investigated the hemolytic effect of pDNA-bPEI, pDNA-OCMCh, and pDNA-OCMPEI complexes on hRBCs (). Whereas the pDNA-PEI complex induced high levels of hemoglobin release from rRBCs, pDNA-OCMCh and pDNA-OCMPEI complexes displayed less than 5% hemolysis even at higher w/w ratios. Both the luciferase assay and fluorescence microscopy showed that the transfection efficiency of the OCMPEI-gene polyplexes depended on the amount of bPEI grafted, with 20% OCMPEI being more cytotoxic than other copolymers tested. The ability of polymers to bind nucleic acids via electrostatic interaction is generally enhanced by increased cationicity. However, cationic polymers are likely to increase cytotoxicity by impairing membrane functions or damaging membrane structures. This is consistent with the aggregation of polymers and disrupted cell membrane on the cell surface. We found that 10% and 15% OCMPEI have an optimum cationicity with good gene transfection and minimum cytotoxicity.
In vitro cell transfection of pDNA/OCMPEI polyplexes
The nucleic-acid-carrying ability of OCMPEI copolymers was evaluated by in vitro transfection of pEGFP into HEK293, HCT119, LoVo, L929, and G361 cells (). Observation of the pEGFP-transfected HCT119 cells by fluorescence microscopy () revealed that polyplexes of 10% and 15% OCMPEI showed significant transfection efficiency at a w/w ratio of 16, although lower levels of GFP expression were observed for 5% and 20% OCMPEI polyplexes than for either 10% or 15% OCMPEI polyplexes. This might be attributable to the low condensation activity of 5% OCMPEI and the relatively high cytotoxicity of 20% OCMPEI. In addition, the transfection efficiency of free OCMCh was poor, as was that of free bPEI, notwithstanding its very high capacity to bind DNA at a low w/w ratio. In particular, almost all cells transfected with free bPEI polyplexes were dead. Investigation of the efficiency of gene transfections with the luciferase gene in HEK293, HCT119, and Lovo cells at various weight ratios () generated results similar to those seen with GFP expression. Many reports suggest that the higher transfection efficiency of polyplexes that include nucleic acids might be attributed to the higher buffering capacity of these complexes and rapid unpackaging of nucleic acids from polymers when they are transfected into the cells.Citation29,Citation39,Citation40 As described in the above results, OCMPEI copolymers permitted easy unpackaging ability, which might account for their capacity for high rates of transfection.
Figure 6 Gene transfection efficiency of pDNA/OCMPEI polyplexes in various cells.
Notes: (A) pEGFP gene transfection of polyplexes at a w/w ratio of 16, free OCMCh, free bPEI, and lipofectamine in HCT119 cells. (B) luciferase expression of pGL3 DNA complexes with OCMPEI copolymers, free OCMCh, free bPEI, and lipofectamine in HEK293, HCT119, and lovo cells.
Abbreviations: bPEI, branched polyethylenimine; DNA, deoxyribonucleic acid; OCMCh, O-carboxymethyl chitosan; OCMPEI, O-carboxymethyl chitosan-graft-branched polyethylenimine; pEGFP, plasmid DNA encoding green fluorescent protein.
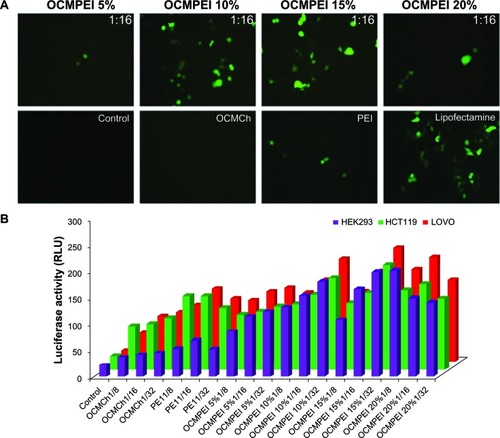
siRNA condensation of OCMPEI copolymers
We anticipated that OCMPEI copolymers might be well suited to delivery of siRNA, given that the chitosan and PEI used have a low molecular weight and assume a branched structure, respectively. Therefore, to investigate the capacity of synthetic copolymers to bind siRNA, copolymers were mixed with GFP-siRNA to allow then to assemble polyplexes at various weight ratios via electrostatic interaction. shows the gel retardation results of siRNA/OCMPEI polyplexes with increasing weight ratios. All polyplexes migrated at a siRNA-OCMPEI weight ratio of 1:2. The 15% and 20% siRNA/OCMPEI showed complete retardation upon electrophoresis, and their particle sizes varied from ∼10–25 nm at a w/w of 1:16 (). Polyplexes with siRNA were remarkably smaller than those with pDNA, given the difference in the molecular weights of siRNA and pDNA.
Figure 7 Gel retardation assay and morphological observation of siRNA/OCMPEI polyplexes.
Notes: (A) Electrophoretic mobility of siRNA complexed by (1) OCMPEI 5%, (2) 10%, (3) 15%, and (4) 20% at w/w ratios of 1:1∼1:16. (B) morphological observation of siRNA/OCMPEI 10% and 15% at a w/w ratio of 1:8 using TEM.
Abbreviations: OCMPEI, O-carboxymethyl chitosan-graft-branched polyethylenimine; siRNA, small interfering ribonucleic acid; TEM, transmission electron microscopy.
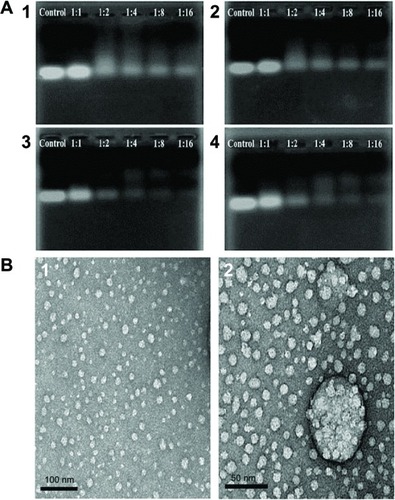
In vitro siRNA delivery
Fluorescence microscopy analysis was performed using PI-labeled siRNA-OCMPEIs to investigate the cellular uptake and distribution of siRNA/OCMPEI polyplexes. HCT 119 cells were treated with siRNA/bPEI or siRNA/OCMPEIs for up to 1 hour (). A failure to observe cellular uptake of free PI-labeled siRNA suggested that siRNA was successfully labeled by PI dye (, PI dye). Almost all cells transfected with free bPEI were dead, and the apparent distribution of the free bPEI throughout the cell suggested that it had a harmful effect. However, both siRNA/OCMPEIs were effectively delivered to the cytoplasm without cellular damage.
In order to investigate the gene silencing efficiency of siRNA/OCMPEI polyplexes, HCT119 cells that were previously transfected with pEGFP/OCMPEI and stably expressed GFP were transfected with the 10% and 15% GFP-siRNA/OCMPEI complexes. The transfection efficiency of the polyplexes was analyzed by fluorescence microscopy and Western blot analysis using anti-GFP antibody. As shown in , control cells without siRNA expressed high levels of GFP, indicating successful and stable GFP expression through pretransfection with pEGFP. Scrambled siRNA had no effect on silencing GFP expression following transfection under the same conditions, with similar levels of GFP to those found in control cells. Interestingly, cells transfected with GFP-siRNA/OCMPEI polyplexes showed a significant downregulation of GFP expression compared with control cells. Moreover, siRNA reduced GFP expression in a dose-dependent manner. These results are consistent with immunoblot data for cellular GFP (). Gene silencing by 15% GFP-siRNA-OCMPEI was more effective than that by 10% GFP-siRNA-OCMPEI.
Figure 8 Gene silencing efficiency of siRNA/OCMPEI 10% and 15% polyplexes in HCT 119 cells.
Notes: (A) HCT 119 cells pretreated with pEGFP/OCMPEI were transfected with scrambled siRNA (600 ng) or GFP-siRNA (300 ng or 600 ng)/OCMPEI 10% and 15%. After 2 days, GFP gene silencing was observed by fluorescence microscope. (B) Western blotting analysis of GFP downregulation. Lane 1: control without pEGFP; lane 2: pretreated with pEGFP/OCMPEI 10%; lane 3: scrambled siRNA/OCMPEI 10%; lane 4: GFP-siRNA/OCMPEI 10% (300 ng); lane 5: GFP-siRNA/OCMPEI 10% (600 ng); lane 6: pretreated with pEGFP/OCMPEI 15%; lane 7: scrambled siRNA/OCMPEI 15%; lane 8: GFP-siRNA/OCMPEI 15% (600 ng), lane 9: GFP-siRNA/OCMPEI 15% (600 ng).
Abbreviations: OCMPEI, O-carboxymethyl chitosan-graft-branched polyethylenimine; siRNA, small interfering ribonucleic acid; GAPDH, glyceraldehyde 3-phosphate dehydrogenase; pEGFP, plasmid DNA encoding green fluorescent protein; GFP, green fluorescent protein.
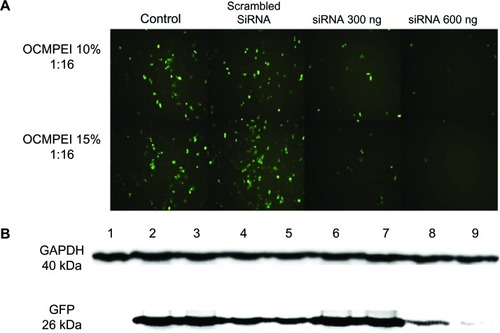
In vivo analysis of transfection efficiency
Gene expression was analyzed in vivo to investigate the possibility of the use of OCMPEI as a carrier for gene delivery. Analysis of GFP expression in a mouse that was intravenously injected with free pEGFP did not show any biodistribution to any of the organs (data not shown), but a mouse injected with pEGFP/OCMPEI showed excellent GFP expression in the lung, kidney, and liver (). We propose that an optimized OCMPEI copolymer in this study can be used for in vivo applications. Moreover, gene delivery into specific diseased tissues or cells might be possible if an appropriate targeting moiety is added to surface of the polyplexes.
Figure 9 In vivo gene delivery of intravenous injected pEGFP/OCMPEI polyplexes to an ICR mouse.
Notes: OCMPEI 15% complexed with 30 μg of pEGFP at a w/w of 32:1 was injected through the mouse tail vein; after 5 days, the whole body (A) and vital organs (B) were fluorescently imaged.
Abbreviations: OCMPEI, O-carboxymethyl chitosan-graft-branched polyethylenimine; pEGFP, plasmid DNA encoding green fluorescent protein.
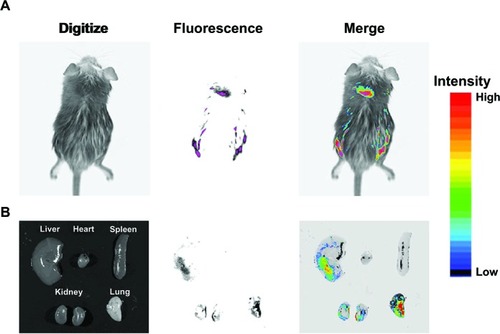
Mechanism of cellular uptake of pDNA/OCMPEI polyplexes
Given that polymeric gene delivery was accomplished by endocytic pathways, the mechanism of internalization of the pDNA-OCMPEI polyplexes was investigated using specific endocytic inhibitors. Sodium azide, indomethacin, colchicine, and chlorpromazine inhibit energy-dependent endocytosis, caveolae-mediated endocytosis, microtubule-dependent macropinocytosis, and clathrin-mediated endocytosis, respectively. As shown in , gene delivery was significantly inhibited by colchicine, suggesting that pDNA uptake via OCMPEI copolymers was achieved by macropinocytosis.
Figure 10 Effect of endocytic inhibitors on cellular uptake in HCT119 cells treated with pDNA/OCMPEI polyplexes.
Notes: (A) After 1-hour preincubation of endocytic inhibitors (3 μg/mL sodium azide, 10 μg/mL chlorpromazine, 8 μg/mL colchicine, and 6 μg/mL indomethacin) with HCT119 cells, pEGFP/OCMPEI 10% was transfected, after which the cell was analyzed by FACSCalibur flow cytometer. (B) The results were visualized using fluorescence microscopy.
Abbreviations: FITC-A, fluorescein isothiocyanate A; OCMPEI, O-carboxymethyl chitosan-graft-branched polyethylenimine; pDNA, plasmid deoxyribonucleic acid.
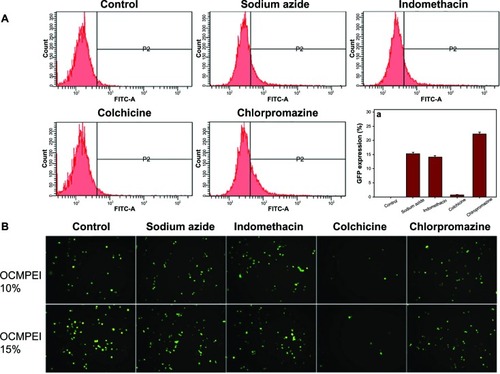
Conclusion
In the present study, bPEI was conjugated to the carboxyl groups of OCMCh, which was derived from the LMWSC (OCMPEI). This conjugation, which proceeded without the loss of amine groups or excess carboxyl groups from chitosan, improved the solubility and binding capacity of OCMCh, as well as its capacity to release a pDNA or siRNA cargo. The use of OCMPEI copolymers not only enhanced transfection efficiency but also reduced cytotoxicity. Moreover, OCMPEI copolymers provide a delivery system that enables the transfection of pDNA and siRNA into cells either separately or simultaneously. The option to deliver pDNA and siRNA either separately or together might have important therapeutic implications. Our in vivo transfection analysis showed that this copolymer might emerge as a valuable polymeric vector for gene delivery.
Supplementary Figures
Figure S1 1H NMR of OCMPEI 5%, 10%, 15%, and 15% in D2O solvent.
Note: The red arrow indicates the increasing intensity peak of PEI.
Abbreviations: D2O, deuterium oxide; 1H NMR, 1H nuclear magnetic resonance; OCMPEI, O-carboxymethyl chitosan-graft-branched polyethylenimine.
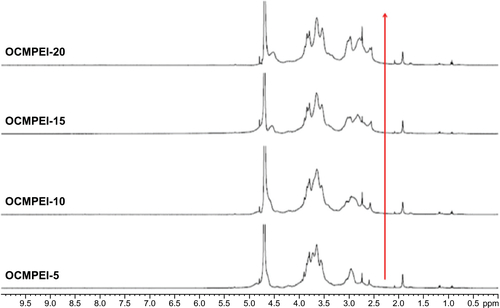
Figure S2 Cellular uptake of PI-labeled siRNA/OCMPEI polyplexes in HCT 119 cells.
Note: PI-labeled siRNA was complexed with OCMPEI 10% and 15% at a w/w ratio of 1:16 and cell nuclei were stained by Hoechst 33342.
Abbreviations: BF, bright field; OCMPEI, O-carboxymethyl chitosan-graft-branched polyethylenimine; PI, propidium iodide; siRNA, small interfering ribonucleic acid; bPEI, branched polyethylenimine.
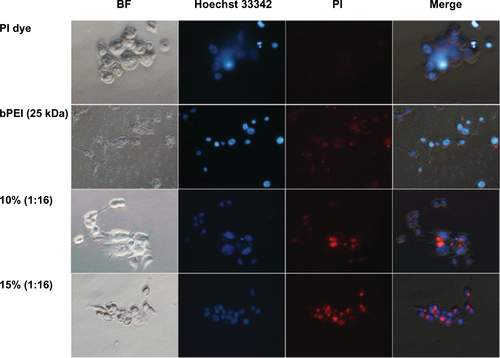
Acknowledgments
This research was supported by the Basic Science Research Program through the National Research Foundation of Korea (NRF) funded by the Ministry of Education, Science and Technology (2011-0012039).
Disclosure
The authors report no conflicts of interest in this work.
References
- RekhaMRSharmaCPPolymers for gene delivery: current status and future perspectivesRecent Pat DNA Gene Seq2012629810722670610
- TieraMJShiQWinnikFMFernandesJCPolycation-based gene therapy: current knowledge and new perspectivesCurr Gene Ther201111428830621453278
- SunXZhangNCationic polymer optimization for efficient gene deliveryMini Rev Med Chem201010210812520408796
- SunshineJCBishopCJGreenJJAdvances in polymeric and inorganic vectors for nonviral nucleic acid deliveryTher Deliv20112449352122826857
- GuoXHuangLRecent advances in nonviral vectors for gene deliveryAcc Chem Res201245797197921870813
- Tros de llarduyaCSunYDüzgünes¸NGene delivery by lipoplexes and polyplexesEur J Pharm Sci201040315917020359532
- WasunguLHoekstraDCationic lipids, lipoplexes and intracellular delivery of genesJ Control Release2006116225526416914222
- ZhangJSLiuFHuangLImplications of pharmacokinetic behavior of lipoplex for its inflammatory toxicityAdv Drug Deliv Rev200557568969815757755
- DassCRCytotoxicity issues pertinent to lipoplex-mediated gene therapy in-vivoJ Pharm Pharmacol200254559360112005353
- MidouxPBreuzardGGomezJPPichonCPolymer-based gene delivery: a current review on the uptake and intracellular trafficking of polyplexesCurr Gene Ther20088533535218855631
- JereDJiangHLAroteRDegradable polyethylenimines as DNA and small interfering RNA carriersExpert Opin Drug Deliv20096882783419558333
- RudzinskiWEAminabhaviTMChitosan as a carrier for targeted delivery of small interfering RNAInt J Pharm20103991–211120732398
- MaoSSunWKisselTChitosan-based formulations for delivery of DNA and siRNAAdv Drug Deliv Rev2010621122719796660
- CreusatGRinaldiASWeissEProton sponge trick for pH-sensitive disassembly of polyethylenimine-based siRNA delivery systemsBioconjug Chem2010215994100220481503
- VicennatiPGiulianoAOrtaggiGMasottiAPolyethylenimine in medical chemistryCurr Med Chem200815272826283918991638
- KwokAHartSLComparative structural and functional studies of nanoparticle formulations for DNA and siRNA deliveryNanomedicine20117221021920709624
- HanzlíkováMSoininenPLampelaPMännistöPTRaasmajaAThe role of PEI structure and size in the PEI/liposome-mediated synergism of gene transfectionPlasmid2009611152118804489
- KawakamiSItoYCharoensitPYamashitaFHashidaMEvaluation of proinflammatory cytokine production induced by linear and branched polyethylenimine/plasmid DNA complexes in miceJ Pharmacol Exp Ther200631731382139016522808
- Garcia-FuentesMAlonsoMJChitosan-based drug nanocarriers: where do we stand?J Control Release2012161249650422480607
- Bernkop-SchnürchADünnhauptSChitosan-based drug delivery systemsEur J Pharm Biopharm201281346346922561955
- WangJJZengZWXiaoRZRecent advances of chitosan nanoparticles as drug carriersInt J Nanomedicine2011676577421589644
- CasettariLVllasaliuDLamJKSolimanMIllumLBiomedical applications of amino acid-modified chitosans: a reviewBiomaterials201233307565758322818987
- KimIYSeoSJMoonHSChitosan and its derivatives for tissue engineering applicationsBiotechnol Adv200826112117884325
- ZakhemERaghavanSGilmontRRBitarKNChitosan-based scaffolds for the support of smooth muscle constructs in intestinal tissue engineeringBiomaterials201233194810481722483012
- JarmilaVVavríkováEChitosan derivatives with antimicrobial, antitumour and antioxidant activities – a reviewCurr Pharm Des201117323596360722074429
- ParkSCNahJWParkYpH-dependent mode of antibacterial actions of low molecular weight water-soluble chitosan (LMWSC) against various pathogensMacromol Res2011198853860
- GaoJLiuRWuJThe use of chitosan based hydrogel for enhancing the therapeutic benefits of adipose-derived MSCs for acute kidney injuryBiomaterials201233143673368122361096
- MaloySFMartinGLAtanassovPCooneyMJControlled deposition of structured polymer films: chemical and rheological factors in chitosan film formationLangmuir20122852589259522220999
- TripathiSKGoyalRKashyapMPDepolymerized chitosans functionalized with bPEI as carriers of nucleic acids and tuftsin-tethered conjugate for macrophage targetingBiomaterials201233164204421922417621
- TripathiSKGoyalRKumarPGuptaKCLinear polyethylenimine-graft-chitosan copolymers as efficient DNA/siRNA delivery vectors in vitro and in vivoNanomedicine20128333734521756861
- HuhMSLeeSYParkSTumor-homing glycol chitosan/polyethylenimine nanoparticles for the systemic delivery of siRNA in tumor-bearing miceJ Control Release2010144213414320184928
- JiangHLKimYKAroteRMannosylated chitosan-graft-polyethylenimine as a gene carrier for Raw 264.7 cell targetingInt J Pharm20093751–213313919481699
- JiangHLKwonJTKimEMGalactosylated poly(ethylene glycol)-chitosan-graft-polyethylenimine as a gene carrier for hepatocyte-targetingJ Control Release2008131215015718706946
- LouYLPengYSChenBHWangLFLeongKWPoly(ethylene imine)-g-chitosan using EX-810 as a spacer for nonviral gene delivery vectorsJ Biomed Mater Res A20098841058106818404706
- NahJWJangMKSpectroscopic characterization and preparation of low molecular, water-soluble chitosan with free-amine group by novel methodJ Polym Sci Part A: Polym Chem2002402137963803
- XuTXinMLiMHuangHZhouSLiuJSynthesis, characterization, and antibacterial activity of N,O-quaternary ammonium chitosanCarbohydr Res2011346152445245021920510
- LavertuMMéthotSTran-KhanhNBuschmannMDHigh efficiency gene transfer using chitosan/DNA nanoparticles with specific combinations of molecular weight and degree of deacetylationBiomaterials200627274815482416725196
- MacLaughlinFCMumperRJWangJChitosan and depolymerized chitosan oligomers as condensing carriers for in vivo plasmid deliveryJ Control Release1998561–32592729801449
- GoyalRTripathiSKTyagiSLinear PEI nanoparticles: efficient pDNA/siRNA carriers in vitro and in vivoNanomedicine20128216717521703995
- RenYJiangXPanDMaoHQCharge density and molecular weight of polyphosphoramidate gene carrier are key parameters infuencing its DNA compaction ability and transfection efficiencyBiomacromolecules201011123432343921067136