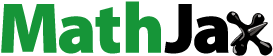
Abstract
Mesoporous silica nanoparticles (MSNs) were synthesized as a promising drug delivery carrier due to the large surface area and porous characteristics. Our previous study successfully recycled wastes from the liquid crystal display (LCD) industry as the silica precursor. In this study, we substantiated the possibility of applying this material as a drug carrier. MSNs synthesized from the extraction of wastes from the manufacture of LCD panels were characterized as having an average diameter of 100 nm, a surface area of 788 m2/g, a uniform pore size distribution of 3.8 nm, and a pore volume of up to 1.04 cm3/g. Methotrexate and camptothecin were entrapped in MSNs at about 33.88% and 75.12%, respectively. The cell viability assay demonstrated that MSNs at 1 μg/mL had no significant influence on human lung fibroblast (WI-38) cells or ovarian cancer (ES-2) cells. A lactate dehydrogenase assay also indicated no inflammation occurred. Moreover, a hemolytic erythrocyte test indicated that the dose range of <100 μg/mL showed that 5% of erythrocytes were affected. After exposure to biofluids, the ordered structure was slightly degraded. The results revealed that MSNs synthesized from extraction of wastes from the manufacture of LCD panels had a good entrapment capacity for hydrophobic drugs and controllable safety conditions; they may be applied as a drug delivery carrier.
Introduction
Because of their great surface area, large pore diameters, and uniform pore structures, mesoporous silica nanoparticles (MSNs) are utilized for adsorption, separation, catalysis, and sensors in various chemical reactions.Citation1 The most common MSNs, such as Mobil Crystalline Material (MCM)-41,Citation2 MCM-48,Citation3 Santa Barbara amorphous silica (SBA)-15,Citation4 Michigan State University silica (MSU)-n,Citation5 and folded sheet-derived mesoporous silica (FSM)-16,Citation6 were studied in the environmental protection field. Silicon alkoxidesCitation7 and sodium silicateCitation8 are common silica sources; however, from the viewpoint of environmental resource protection, the current trend is towards recycling wastes containing silica or silicon as precursors for synthesizing mesoporous SiO2, instead of using commercial agents. For example, fly ashCitation9–Citation11 and rice husksCitation12 can be utilized as precursors to synthesize mesoporous SiO2 characterized by a hexagonal pore arrangement with a high surface area. In addition, the semiconductor and photoelectric industries in Taiwan are world-famous industries; large amounts of waste are also produced with increasing yields. Recently, MSNs have attracted much attention in the biomedical field due to their unique characteristics; Vallet-Regi et al applied MCM-41 as a carrier in a drug delivery system.Citation2 Due to the versatile properties of MSNs, including a high Brunauer–Emmett– Teller (BET) surface area, a large pore volume, and uniform porosity, MSNs are excellent carrier candidates for encapsulation and release of a variety of active pharmaceutical ingredients (APIs).Citation13,Citation14
Nano-enabled drug delivery systems are being applied in the pharmaceutical industry since nanoparticle-based drugs may have improved solubility and altered pharmacokinetics and biodistributions compared to small-molecule drugs.Citation15 The most interesting chemical properties of MSNs are the large specific surface area and large pore volume which have a high capacity for loading APIs in the inner pore system. MSN carriers used in drug delivery applications can provide several advantages including achievement of a sustained or controlled release, improved solubility of poorly soluble drugs, protection of the biological payload from physiological degradation, and a volumetric drug reservoir.Citation16 With clinical chemotherapy, the main problem is the rapid therapeutic drug release or dumping from the vehicle or matrix before reaching the targeted site, which can cause systemic side effects. MSN surfaces suitably decorated with recognition moieties, such as antibodies,Citation17 ligands, and polyethylene glycol (PEG), could achieve specific targeting. The functionalized process avoids nonspecific drug distribution throughout the body and minimizes side effects, while improving the therapeutic efficacy.Citation18
Lin et alCitation19,Citation20 successfully recycled wastes from the liquid crystal display (LCD) industry as a silica precursor, and synthesized an efficient mesoporous adsorbent for CO2 capture. Moreover, the other elements also ensured that no significant residuals were present in the MSNs. Building on previous experience, the current study focused on safety and biocompatibility evaluations of MSNs synthesized from extraction of wastes from the manufacture of LCD panels to substantiate possible applications as drug carriers. Material characterizations of the pore structure, specific surface area, and pore volume were determined by the BET method using nitrogen adsorption–desorption isotherms. The safety assay utilized a cell viability test on a normal human lung fibroblast (WI-38) cell line and a human ovarian cancer (ES-2) cell line, and hemolysis of erythrocytes was also evaluated. The entrapment efficiencies (EE%) of two hydrophobic drugs were measured by a centrifugation method. In order to understand changes in the pore structure of MSNs when administrated by oral or intravenous injection through gastric, intestinal, or plasma routes, the crystalline structure of MSNs was examined by powder x-ray diffraction and transmission electron microscopy (TEM).
Experimental procedures
Materials
Waste powder from the manufacture of LCD panels was provided by a thin-film-transistor LCD manufacturer in the Hsinchu Science Industrial Park, Hsinchu, Taiwan. There was no decontaminated treatment of these powders in the factory. Methotrexate anhydrous basis (MTX), camptothecin (CPT), and hexadecyltrimethylammonium bromide (CTAB, 89%) were purchased from Sigma-Aldrich (St Louis, MO, USA). Sulfuric acid (98%, reagent of special grade) was from Shimakyu Co., (Osaka, Japan). Other chemicals used in the study were of reagent grade. Human lung fibroblast (WI-38) cells and human ovarian cancer (ES-2) cells were purchased from the Culture Collection and Research Center of the Food Industry Research and Development Institute (Hsinchu, Taiwan). Cell culture media and supplements were obtained from Life Technologies (Carlsbad, CA, USA).
Preparation of MSNs
Waste silica powder obtained from thin-film-transistor LCD was utilized to synthesize MSNs, and the template in the synthesis process of mesoporous structure was chosen as CTAB. Briefly, the synthesis procedure of MSNs was described as below; 9.35 g of waste silica powder was prepared, added, and stirred in 137 mL of deionized (DI) water. Subsequently, a given amount of hydrofluoric acid was added; in addition, 7.28 g of CTAB was prepared and dissolved in 25 mL of DI water, and then dropped into the solution. Then, 32.06 g of ammonium hydroxide solution was added to enhance the hydrolytic condensation of the silica–surfactant precursor. The molar ratio and the composition of the synthesis precursor were 1 SiO2:0.2 CTAB:10 HF:12 NH4OH:120 H2O. All of the above procedures were performed under continuous stirring. The resulting precursor was aged at 25°C for 8 hours; the resultant solid was recovered by filtration, washed with DI water, and dried in an oven at 110°C for 6 hours. Finally, the resultant solid was put in the furnace at 550°C for 6 hours to remove the organic template, and the MSNs could be obtained after the temperature cooled down to 25°C. The schematic illustration of MSNs produced is shown in .
Material characterization of MSNs
The pore size distribution was calculated from the adsorption branch of the N2 adsorption–desorption isotherm and the Barrett–Joyner–Halenda (BJH) method. The specific surface area of the MSNs was determined by the BET method using nitrogen adsorption–desorption isotherms (ASAP 2020; Micromeritics Instrument Corporation, Norcross, GA, USA), and the pore volume was obtained by the t-plot method. The crystalline structure of the MSNs was observed by low-angle X-ray diffraction (LAXRD; D8 Discover; Bruker Corporation, Billerica, MA, USA), and surface observations were obtained by TEM (JEM-2000Ex; JEOL, Tokyo, Japan).
Cell viability assay
The percent cell survival was determined with a 3,(4,5-dimethylthiazol-2-yl)2,5-diphenyltetrazolium bromide (MTT) assay, which produces formazan crystals in mitochondria within cells. Human lung fibroblast (WI-38) and human ovarian cancer (ES-2) cells were respectively seeded in minimum essential medium α and Roswell Park Memorial Institute 1640 medium on 24-well plates at a cell density of 2 × 104 cells/well until cells were confluent. Medium was replaced by MSN suspensions at different concentrations for 24 hours at 37°C in air containing 5% CO2. Then we added 200 μL of the MTT solution (0.5 mg/mL) to each well and incubated the wells for 2 hours. In order to dissolve the water-insoluble formazan, MTT/medium was removed from each well, and 500 μL of dimethyl sulfoxide was added to each well. The extent of cell survival was determined by an enzyme-linked immunosorbent assay reader at 595 nm.
Lactate dehydrogenase assay
Lactate dehydrogenase (LDH) release was determined by a commercially available method (Promega Corporation, Fitchburg, WI, USA). A cell density of 2 × 104 cells/well was exposed to various concentrations of MSNs for 24 hours. The total LDH released was determined by lysing cells with 0.1% Triton x-100 for 30 minutes at 37°C. Inflammation was represented by LDH release in a cell-free medium as a percentage of the total LDH release. The activity of extracellular LDH was measured at 490 nm.
Erythrocyte hemolysis
Blood samples, obtained from healthy human volunteers, were collected into VACUTAINER™ tubes (BD Co., Ltd Franklin Lakes, NJ, USA). Erythrocytes were separated from blood samples by centrifugation at 2,000 rpm for 5 minutes and washed four times with phosphate-buffered saline (PBS). Following the last wash, red blood cells (RBCs) were dispersed in PBS (v/v, 1/9) as the erythrocyte stock. The RBC stock (100 μL) was mixed with 50 μL of sample and 2.45 mL of PBS, and the mixture was incubated at 37°C for 1 hour, and then centrifuged at 2,000 rpm for 5 minutes to separate hemoglobin from complete and incomplete erythrocytes. The absorbance of the supernatant (hemoglobin) was measured with a spectrophotometer at 415 nm. Erythrocytes were dissolved in double-distilled water as a positive control (100% hemolysis) and in PBS as a negative control (0% hemolysis). The percentage of hemolysis was calculated as follows:
TEM
The morphology of original MSNs was examined by TEM to characterize the microstructure. MSNs were incubated at 37°C and shaken at 50 rpm in an incubator in various fluids, including 0.1 N HCl for 1 hour to simulate gastric fluid and at pH 6.8 for 4 hours to simulate intestinal fluid. Original MSNs were suspended in double-distilled water, and those cells were applied to a carbon film-covered copper grid. After drying, the sample was examined by TEM (JEM-2000Ex; JEOL).
LAXRD
In order to measure the crystal structure of MSNs, LAXRD was performed. After the same pretreatment process as for TEM, the sample was washed with double-distilled water in a centrifuge at 5,000 rpm for 10 minutes, and then the supernatant was removed. The centrifugation process was repeated three or four times. MSN pellets were dried at 50°C for 8 hours. The sample was examined by LAXRD (D8 Discover; Bruker) with CuKα radiation (λ = 0.154 nm) at a 2θ angle range of 0°–10° to measure the crystal structure.
Loading drugs into MSNs and determining the EE%
MTX and CPT were separately used as model drugs, and effects of different concentrations of drugs of 0.01, 0.05, and 0.1 mg/mL loaded into MSNs were examined. The model drug molecules were loaded into pores of MSNs by soaking in a solution containing MTX or CPT for 12 hours. MTX or CPT was pre-dissolved in absolute ethanol or 30% ethanol, respectively. The EE% utilized a centrifugation method to separate the incorporated drug from the free form. Vesicle suspensions were centrifuged at 15,000 rpm for 30 minutes. Following centrifugation, non-incorporated free drug was in the supernatant, and the pellet was washed three or four more times. Amounts of free drug and entrapped drug were analyzed by high-performance liquid chromatography (HPLC) to determine the EE%, which was calculated by the following equation:
Quantification of drugs by HPLC
Model drug contents of the various samples were analyzed with an HPLC system consisting of an L-7110 pump, an L-7200 sample processor, an L-7485 ultraviolet detector, and an L-7485 fluorescence detector (all from Hitachi Ltd Tokyo, Japan). For MTX determination, a 25 cm long, 4 mm inner diameter stainless steel RP-18 column (Merck KGaA, Darmstadt, Germany) was used. The mobile phase consisted of acetonitrile-water-tetrahydrofuran (65: 30: 5 v/v/v) at pH 3.0 adjusted with phosphoric acid at a flow rate of 0.8 mL/minute. The ultraviolet detector was set to a wavelength of 313 nm. For CPT determination, a 25 cm long, 4 mm inner diameter stainless steel RP-18 column (Merck) was used. The mobile phase was an acetonitrile: pH 4 aqueous solution adjusted with acetic acid (35:65) at a flow rate of 1.0 mL/minute. Wavelengths of excitation and emission were set to 360 and 440 nm.
Statistical analysis
All data are expressed as the mean ± standard derivation. Statistical analyses were performed using an unpaired Student’s t-test with WINKS SDA 6.0 software (TexaSoft, Duncanville, TX, USA). A 0.05 level of probability was used as the level of significance. An analysis of variance test was also used.
Results and discussion
MSNs are well-known inorganic materials, which provide large spaces as drug delivery carriers. Our past research explored MSNs synthesized from the extraction of waste from the manufacture of LCD panels as an excellent CO2 adsorbent.Citation19,Citation20 Synthesized MSNs possessed mesoporous properties of about 100 nm in average diameter, a surface area of 788 m2/g, a uniform pore size distribution of 3.8 nm, and a pore volume of up to 1.04 cm3/g. MSNs were capable of encapsulating hydrophobic chemotherapeutic drugs including MTX and CPT, and after exposure to simulated biofluids, the ordered structure was degradable. These results demonstrate that MSNs synthesized by extraction from LCD panel manufacture wastes are biocompatible for application as drug carrier candidates.
Physicochemical characterization of MSNs
An illustration of the N2 adsorption-desorption isotherms of MSNs is shown in . It is clear that MSNs exhibited type IV isotherms which was characteristic of mesoporous materials, featuring a narrow step due to capillary condensation of N2 within the primary mesopores.Citation21,Citation22 The isotherms of the MSNs also indicated the type H1 hysteresis loop associated with well-arranged cylindrical channels with uniform shape and pore size.Citation23 also showed the BJH pore size distributions of MSNs. It is clear that the MSNs also showed a narrow pore size distribution with the peak size at around 3.5 nm, and the mesostructure of MSNs was very uniform. The physicochemical properties of BET specific surface area, specific pore volume, and average pore diameter derived from the N2 adsorption–desorption measurements are summarized in . lists an average particle diameter of MSNs of 100 nm, a specific surface area of 788 m2/g, an average pore diameter of 3.8 nm by the BJH analysis, and a pore volume of 1.04 cm3/g. To observe the microstructure, a TEM analysis was also performed. shows the original silica source of MSNs which have a nanometer-sized spherical outline and uniform shapes. The images also revealed that MSNs possess a worm-like structural order with a mean diameter of 100 nm at magnifications of 40,000× and 80,000×.
Table 1 Structural parameters of mesoporous silica nanoparticles
Figure 2 The N2 adsorption–desorption isotherm and BJH pore diameter distribution of the MSNs.
Abbreviations: BJH, Barrett–Joyner–Halenda; MSNs, mesoporous silica nanoparticles.
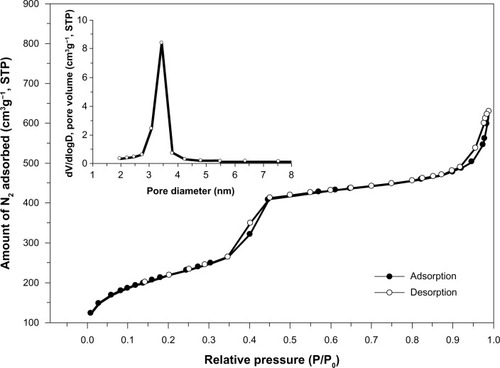
Figure 3 TEM images.
Notes: TEM images of (A) original MSNs, (B) MSNs incubated in simulated gastric fluid for 1 hour, and (C) MSNs incubated in simulated intestinal fluid for 4 hours. All of the upper panels were magnified 40,000×; lower panels were magnified 80,000×.
Abbreviations: MSNs, mesoporous silica nanoparticles; TEM, transmission electron microscopy.
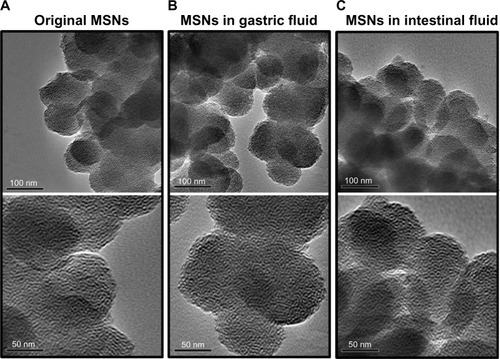
Cell viability and LDH release
We screened both the WI-38 and ES-2 cell lines to understand the cytotoxicity of MSNs, as shown in . When 1 μg/mL of MSNs was added to WI-38 cells, there was no significant change compared to the control group (P > 0.05). The addition of 5 μg/mL and 10 μg/mL of MSNs reduced the viability of the WI-38 cells to 82% and 84%, respectively, whereas at 50 μg/mL, the viability of the WI-38 cells significantly decreased to 34% (P < 0.001). On the other hand, 1 μg/mL and 5 μg/mL of MSNs did not produce significant changes in ES-2 cells. When concentrations were increased to 10 μg/mL and 50 μg/mL, cell viabilities were respectively reduced to 84% and 46%.
Figure 4 Cellular viability of mesoporous silica nanoparticles suspended in cell media at concentrations of 1, 5, 10, and 50 μg/ml in (A) a human lung fibroblast (WI-38) cell line and (B) a human ovarian cancer (ES-2) cell line.
Notes: Cell viability was normalized by setting the viability of untreated cell to 100%. Data are presented as the mean ± standard deviation (n = 3). *P<0.05, **P<0.01, ***P<0.001.
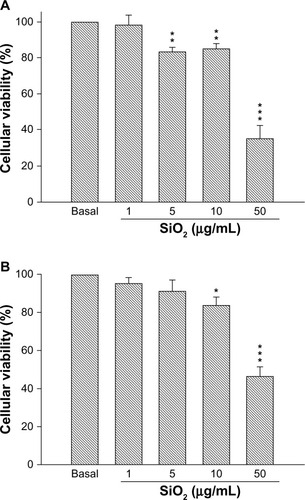
Since inflammation may elevate LDH release, we studied the influence of the MSNs concentration in both normal WI-38 cells and ES-2 cancer cells. The percentage of LDH was measured after exposure of WI-38 cells to various concentrations of MSNs for 24 hours. The LDH results also presented the same trend as the MTT assays, as shown in . After exposure to MSNs at 1 μg/mL to 10 μg/mL, there were no significant differences compared to the blank (P > 0.05); however, with 50 μg/mL of MSNs, LDH release significantly increased to 62.59% ± 5.98% of the total value (P < 0.001). In ES-2 cells, LDH release had the same tendency. There were no significant differences when incubated with MSNs at 1–10 μg/mL; however, when the MSNs concentration was increased to 50 μg/mL, LDH significantly increased to 54.83% ± 1.62% of the total value (P < 0.001).
Figure 5 Inflammation assay by measuring LDH release from (A) human lung fibroblast (WI-38) cells and (B) human ovarian cancer (ES-2) cells treated with different concentrations of mesoporous SiO2 nanoparticles.
Note: ***P < 0.001.
Abbreviation: LDH, lactate dehydrogenase.
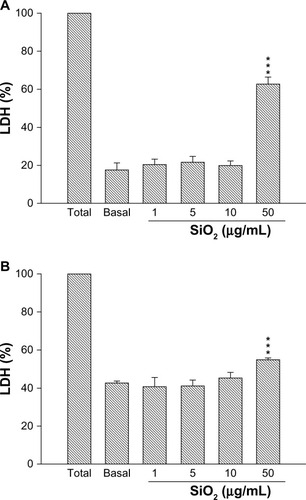
Cell viability and LDH assays were designed to understand the cytotoxicity and inflammation, and exposure of WI-38 and ES-2 cells to SiO2 MSNs. At 1 μg/mL of SiO2 MSNs, there was no cytotoxicity after 24 hours of exposure. In contrast, exposure to 5–50 μg/mL significantly increased cell death, causing the viability of WI-38 and ES-2 cells to remain at about 30%–50%. LDH results were consistent for both WI-38 and ES-2 cells, which indicated that SiO2 MSNs induced inflammation at the 50 μg/mL dose. On the other hand, exposure to 1–10 μg/mL showed no inflammatory phenomena.
Silica MSN materials are extensively used in a wide variety of applications including catalytic support, photonic crystals, gene delivery, photodynamic therapy, and the biomedical field, being particularly attractive as a drug delivery carrier.Citation24 If employing SiO2 MSNs as an injectable drug delivery carrier, these nanoparticles must be <100 nm in diameter to avoid immediate uptake by the reticuloendothelial system.Citation25 However, much research indicated that amorphous mesoporous/nanoporous silica nanoparticles of <50 nm in diameter reduced cell viability in an in vitro model. In addition, a previous study pointed out that ultrafine particles (,15 nm) had a greater ability to enhance pulmonary injury than fine particles (>200 nm) at the same chemical composition in an in vivo model.Citation26 Past research proved that exposure to silica is associated with progressive pulmonary inflammation and fibrosis. We need to confirm a limiting dose for usage.
Hemolysis
The hemolysis assay was used to evaluate a safe dose when human erythrocytes are exposed to MSNs via intravenous administration. As shown in , MSNs at 5 μg/mL produced 3% hemolytic activity, at 1,000 μg/mL produced 30% activity, and at 5,000 μg/mL produced 86% activity. The hemolysis percentage of erythrocytes increased in a dose-dependent manner. These data revealed a good linear correlation (r = 0.954) in the range of 5–5,000 μg/mL of MSNs.
Figure 6 (A) Percentage of hemolytic human erythrocytes induced by different concentrations of MSNs at 5–5,000 μg/ml. (B) Images of erythrocyte samples which had been centrifuged to detect free hemoglobin in the supernatants.
Note: Data are presented as the mean ± SD (n = 3).
Abbreviations: MSNs, mesoporous silica nanoparticles; SD, standard deviation.
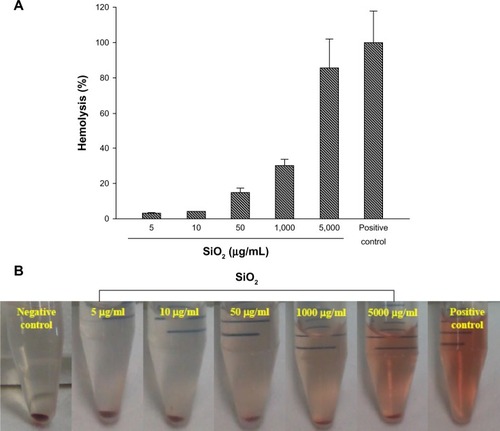
Generally, a drug delivery carrier is administrated by an intravenous route, and the hemolysis of erythrocyte is a serious biosafety concern.Citation27 To determine the concentration leading to lysis of erythrocytes by MSNs, human erythrocytes were incubated at a range of concentrations of 50–5,000 μg/mL for 1 hour. The in vitro results showed that the highest dose of 5,000 μg/mL of MSNs used in this study produced an 86% hemolytic effect. In contrast, at the lower doses of 5 μg/mL and 10 μg/mL of MSNs, hemolysis of RBCs was detected at about 3%–4%. We discuss the relationship between hemolysis and MSNs from three aspects. (1) In terms of the amorphous structure, silica materials with amorphous particle morphology will cause hemolysis of mammalian RBCs.Citation28 This kind of hemolytic behavior raises serious biosafety concerns regarding the application of amorphous silica for drug delivery involving intravenous administration and transport. (2) In terms of functional groups on MSNs surfaces, most researchers agree that the hemolytic activity of silica is related to surface silanol groups.Citation29 In the present study, our MSNs were pure silica, and no hydroxyl groups were connected to the Si. (3) In terms of particle size, previous studies indicated that larger particle sizes of about 250 nm may be preferable for MSNs hemocompatibility,Citation24 and at about 600 nm, strong local membrane deformation occurs leading to spiculation of the RBCs.Citation30 According to the above points, SiO2 MSNs in the present work did not have an amorphous structure, there were no surface modifications on SiO2 MSNs with hydroxyl groups, and particle sizes were smaller than 100 nm. Hence, SiO2 MSNs should not cause serious hemolytic effects when within controlled ranges.
EE% of anticancer drugs
MTX and CPT are used as potent chemotherapeutic agents in the clinic. However, because of the poor water solubility of these drugs, they do not dissolve in most biocompatible media, which obstructs delivery into tumor cells. Although synthesizing analogs by modifying the chemical structure is an alternative strategy, the irinotecan analog presented lower cytotoxicity toward cancer cells.Citation31 In addition, an alternative strategy is to make hydrophobic drugs in a lyophilized powder form, a well-known method to resolve delivery problems. Unfortunately, a narrow therapeutic index and toxicity cause serve adverse reactions. For example, CPT needs to be resuspended in an alkaline solution before being intravenously injected, which significantly alters the toxicological profile of the drug.Citation32 A clinical study clearly demonstrated that criteria of therapeutic drug monitoring need to be selected for MTX and CPT to optimize cancer chemotherapy.Citation33 Hence, changes in the physicochemical properties of hydrophobic drugs are necessarily required.
In this work, we employed a novel calcination method to extract a silica precursor as MSNs, and investigated the EE% of the hydrophobic drugs, as shown in . The results showed that the respective EE% values of MTX and CPT adsorbed in the MSNs were about 34% and 75%, respectively. Some physicochemical-dependent manners exist between hydrophobic drugs and MSNs, such as the molecular weight, log P, polar surface area, and pKa. Basically, MSNs in this study presented a wormhole-like porous structure which produced a large specific surface area measured via BET method of 788 m2/g. However, MTX presented a low entrapment efficiency compared to CPT. That may be attributed to MTX’s structure with amino and carboxylic groups which interact with SiO2 through weak ionic interactions. On the other hand, CPT was highly entrapped in the MSNs, and the possibility of interactions between CPT and SiO2 molecules was considerable. The hydrocarbon moiety of the CPT structure may interact with SiO2 by hydrogen bonding forces. Typically, electronegative oxygen exists in SiO2, and oxygen has a partial negative charge, which may exhibit an affinity to interact with hydrogen atoms of CPT. Many hydrocarbon moieties exist in the CPT structure which can provide protons to interact with oxygens of SiO2.
Table 2 EE% of the anticancer drugs, MTX, and CPT
Biocompatibility
In order to understand the effects of MSNs after oral administration or intravenous injection into the circulation, the structural order of MSNs will be changed by various biofluids. Original MSNs synthesized from wastes from LCD panel manufacture are shown in , which present an ordered worm-like structure with a mean diameter of 100 nm. On the other hand, the mean diameter and pore order of MSNs did not significantly change in simulated gastric or intestinal fluid on the microscopic scale.
Figure 7 Low-angle X-ray diffraction patterns of original mesoporous silica nanoparticles which had been incubated in simulated gastric fluid for 1 hour, in intestinal fluids for 4 hours, and in plasma-like conditions for 24 hours.
Abbreviation: MSNs, mesoporous silica nanoparticles.
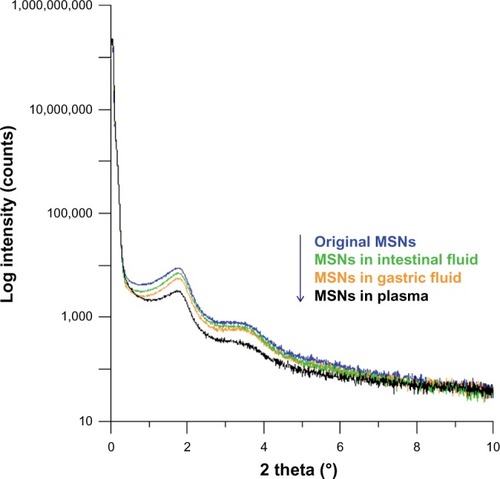
Moreover, LAXRD was performed to confirm changes in the pore order in microscopic observations, since impurities exist in the plasma medium which can be observed by TEM. Although shows that MSNs incubated in gastric and intestinal fluids exhibited negligible changes, under LAXRD determination as shown in , MSNs incubated in gastric fluid for 1 hour and intestinal fluids for 4 hours showed a slight decrease, particularly at the 1.9° peak. LAXRD patterns of MSNs exposed to plasma for 24 hours were particularly distinctive at the 1.9° peak and in the range of 2.5°–4°. The results suggest that MSNs present slight biodegradable properties, particularly in gastric conditions and plasma circulation. This property is more important in relation to the design concept as to what kind of drug is suitable to be carried.
Conclusion
In summary, we utilized MSNs synthesized from extraction of wastes from the manufacture of LCD panels as a silica source for drug delivery carriers. This is pioneering work which successfully entrapped hydrophobic dugs in MSNs to overcome their water-insoluble properties. Moreover, exposure of MSNs to bio-conditions caused the ordered structure to become more degradable, especially in plasma conditions. This indicates that MSNs have good biodegradable properties. We also established dose limitations when applied as delivery carriers. The present study revealed that MSNs synthesized from extraction of wastes from the manufacture of LCD panels had good entrapment capacity for hydrophobic drugs and controlled safety conditions; they can be applied as a silica source for drug delivery carriers. There is a need for more in vivo assessments in a rat model with administration of MSNs orally or by injection to understand the changes in biochemical indicators and to investigate the pharmacokinetics. Also, the release behavior needs to be clarified.
Acknowledgments
We are grateful to Professor Tsong-Long Hwang, Laboratory of Cellular Pharmacology, Graduate Institute of Natural Products, College of Medicine, Chang Gung University, Taiwan, for assistance with the cellular cytotoxicity and inflammation assays.
Disclosure
The authors report no conflicts of interest in this work.
References
- TangFLiLChenDMesoporous silica nanoparticles: synthesis, biocompatibility and drug deliveryAdv Mater201224121504153422378538
- Vallet-RegiMRámilaAdel RealRPPérez-ParienteJA new property of MCM-41: drug delivery systemChem Mater2001132308311
- HuoQLeonRPetroffPMStuckyGDMesostructure design with gemini surfactants: supercage formation in a three-dimensional hexagonal arrayScience199526852151324132717778977
- ZhaoDFengJHuoQTriblock copolymer syntheses of mesoporous silica with periodic 50 to 300 angstrom poresScience199827953505485529438845
- BagshawSAProuzetEPinnavaiaTJTemplating of mesoporous molecular sieves by nonionic polyethylene oxide surfactantsScience199526952281242124417732109
- InagakiSFukushimaYKurodaKSynthesis of highly ordered mesoporous materials from a layered polysilicateJ Chem Soc Chem Commun19938680682
- BeckJSVartuliJCRothWJA new family of mesoporous molecular sieves prepared with liquid crystal templatesJ Am Chem Soc1992114271083410843
- LinHPChengSMouCYMesoporous molecular sieves MCM-41 with a hollow tubular morphologyChem Mater1998102581589
- ChoiCLParkMLeeDHKimIEParkBYChoiJSalt-thermal zeolitization of fly ashEnviron Sci Technol200135132812281611452614
- ChangHLChunCMAksayIAShihWHConversion of fly ash into mesoporous aluminosilicateInd Eng Chem Res1999383973977
- HuiKSChaoCYSynthesis of MCM-41 from coal fly ash by a green approach: influence of synthesis pHJ Hazard Mater200613721135114816647813
- BhagiyalakshmiMYunLJAnuradhaRJangHTUtilization of rice husk ash as silica source for the synthesis of mesoporous silicas and their application to CO2 adsorption through TREN/TEPA graftingJ Hazard Mater20101751–392893819939554
- ChoYShiRBorgensRBIvanisevicAFunctionalized mesoporous silica nanoparticle-based drug delivery system to rescue acrolein-mediated cell deathNanomedicine (Lond)20083450751918694313
- PopatAHartonoSBStahrFLiuJQiaoSZQing Max LuGMesoporous silica nanoparticles for bioadsorption, enzyme immobilisation, and delivery carriersNanoscale2011372801281821547299
- ShiJVotrubaARFarokhzadOCLangerRNanotechnology in drug delivery and tissue engineering: from discovery to applicationsNano Lett20101093223323020726522
- ArrueboMDrug delivery from structured porous inorganic materialsWiley Interdiscip Rev Nanomed Nanobiotechnol201241163021374827
- ClimentEBernardosAMartínez-MáñezRControlled delivery systems using antibody-capped mesoporous nanocontainersJ Am Chem Soc200913139140751408019739626
- AznarEMarcosMDMartínez-MáñezRpH- and photo-switched release of guest molecules from mesoporous silica supportsJ Am Chem Soc2009131196833684319402643
- LinLYKuoJTBaiHSilica materials recovered from photonic industrial waste powder: its extraction, modification, characterization and applicationJ Hazard Mater2011192125526221641110
- LinLYBaiHEfficient method for recycling silica materials from waste powder of the photonic industryEnviron Sci Technol20134794636464323521136
- Al-AttarLDyerAHarjulaRUptake of radionuclides on microporous and layered ion exchange materialsJ Mater Chem20031329632968
- BoreMTRathodSBWardTLDatyeAKHexagonal mesostructure in powders produced by evaporation-induced self-assembly of aerosols from aqueous tetraethoxysilane solutionsLangmuir2003192256264
- ChandrasekarGYouKAhnJAhnWSynthesis of hexagonal and cubic mesoporous silica using power plant bottom ashMicroporous Mesoporous Mater20081111–3455462
- LinYSHaynesCLImpacts of mesoporous silica nanoparticle size, pore ordering, and pore integrity on hemolytic activityJ Am Chem Soc2010132134834484220230032
- HeXNieHWangKTanWWuXZhangPIn vivo study of biodistribution and urinary excretion of surface-modified silica nanoparticlesAnal Chem200880249597960319007246
- NapierskaDThomassenLCRabolliVSize-dependent cytotoxicity of monodisperse silica nanoparticles in human endothelial cellsSmall20095784685319288475
- KosterVSKuksPFMLangeRTalsmaHParticle size in parenteral fat emulsions, what are the true limitations?Int J Pharm19961341–2235238
- ZhaoYSunXZhangGTrewynBGSlowingIILinVSInteraction of mesoporous silica nanoparticles with human red blood cell membranes: size and surface effectsACS Nano2011521366137521294526
- MurashovVHarperMDemchukEImpact of silanol surface density on the toxicity of silica aerosols measured by erythrocyte haemolysisJ Occup Environ Hyg200631271872317133693
- LinYSHaynesCLSynthesis and characterization of biocompatible and size-tunable multifunctional porous silica nanoparticlesChem Mater2009211739793986
- CunhaKSRegulyMLGrafURodrigues de AndradeHHComparison of camptothecin derivatives presently in clinical trials: genotoxic potency and mitotic recombinationMutagenesis200217214114711880543
- LuJLiongMZinkJITamanoiFMesoporous silica nanoparticles as a delivery system for hydrophobic anticancer drugsSmall2007381341134617566138
- HonYYEvansWEMaking TDM work to optimize cancer chemotherapy: a multidisciplinary team approachClin Chem19984423884009474050