Abstract
A novel injectable bone cement was developed by integration of nano calcium-deficient hydroxyapatite/multi(amino acid) copolymer (n-CDHA/MAC) and calcium sulfate hemihydrate (CSH; CaSO4 · 1/2H2O). The structure, setting time, and compressive strength of the cement were investigated. The results showed that the cement with a liquid to powder ratio of 0.8 mL/g exhibited good injectability and appropriate setting time and mechanical properties. In vitro cell studies indicated that MC3T3-E1 cells cultured on the n-CDHA/MAC/CSH composite spread well and showed a good proliferation state. The alkaline phosphatase activity of the MC3T3-E1 cells cultured on the n-CDHA/MAC/CSH composite was significantly higher than that of the cells on pure CSH at 4 and 7 days of culture. The n-CDHA/MAC/CSH cement was implanted into critical size defects of the femoral condyle in rabbits to evaluate its biocompatibility and osteogenesis in vivo. Radiological and histological results indicated that introduction of the n-CDHA/MAC into CSH enhanced new bone formation, and the n-CDHA/MAC/CSH cement exhibited good biocompatibility and degradability. In conclusion, the injectable n-CDHA/MAC/CSH composite cement has a significant clinical advantage over pure CSH cement, and may be a promising bone graft substitute for the treatment of bone defects.
Introduction
Treatment of large bone defects is currently a significant challenge for orthopedic surgeons. Many efforts have been made to develop new bone graft substitutes in recent years, due to the inherent limitations of autografts and allografts.Citation1,Citation2 Nano hydroxyapatite possesses excellent biocompatibility and bioactivity and is thus widely applied for bone tissue engineering.Citation3 However, its extensive clinical application is still limited by its poor degradability and brittle property.Citation4 Biocompatible polymers are another suitable candidate for bone graft substitutes. However, this material also has several shortcomings, including uncontrollable degradation, induction of local adverse tissue responses, and rapid strength decay.Citation5,Citation6 In order to overcome the above-mentioned disadvantages of bioceramics and biopolymers, ceramic/polymer composite materials that mimic the unique structure of hydroxyapatite/collagen in natural bone have become a focus of current research.Citation7–Citation9
Recently, a degradable biocomposite of nano calcium-deficient hydroxyapatite/multi(amino acid) copolymer (n-CDHA/MAC) has been developed in our laboratory.Citation10 The composite consists of n-CDHA and an amide linkage copolymer. The n-CDHA inorganic component has a lower Ca/P ratio than nano hydroxyapatite (1.50 versus 1.67) and thus shows an improved degradability.Citation11,Citation12 The MAC that is composed of 6-aminocaproic acid and five amino acids exhibits good ductility and biocompatibility.Citation13 Our previous works have suggested that the amide-calcium-amide linkage and hydrogen bond formed between n-CDHA and MAC endow this biocomposite with eligible mechanical strength (compressive strength in the range of 88–129 MPa), degradability, cytocompatibility, and osteoconductivity.Citation10–Citation13 However, the n-CDHA/MAC composite material has relatively poor flexibility, which limits its application in repairing complex and irregular bone defects. Injectable composites are gaining increasing interest in the orthopedic field, as they can be delivered to the target site via a minimally invasive manner. The injectable system has many advantages such as shortening of operation time, minimizing unwanted tissue damage, and reducing hospitalization length and cost.Citation14 Thus, we sought to develop an injectable form of the n-CDHA/MAC composite.
Calcium sulfate hemihydrate (CSH; CaSO4 · 1/2H2O), also known as plaster of Paris, has a long history of clinical utilization in treating bone defects.Citation15–Citation17 The ability of self-setting in situ renders CSH easy to handle and suitable for different types of bone injury. CSH has been successfully introduced to the nano-hydroxyapatite/collagen composite to yield an injectable and self-setting in situ bone graft substitute.Citation18 These findings encouraged us to integrate the n-CDHA/MAC and CSH, aiming to combine the advantages of each compound and produce a novel injectable bone cement. In this study, we evaluated the self-setting time, mechanical properties, biocompatibility, and osteogenesis of the new composite based on different ratios of n-CDHA/MAC and CSH both in vitro and in vivo.
Materials and methods
Synthesis of n-CDHA/MAC/CSH composite
The n-CDHA/MAC composite was synthesized by an in-situ polymerization method as described previously.Citation10 In brief, 105 g of 6-aminocaproic acid, 6 g of alanine, 7 g of phenylalanine, 1 g of glycine, 6 g of proline, 2 g of lysine, and n-CDHA slurry (5 g n-CDHA/100 mL water) were added to a reaction kettle with continuous stirring. The mixture was heated to 200°C for about 2 hours until the water was fully evaporated, and the mixture was melted. The reaction product was then kept at 21°C for 2 hours and at 220°C for 1 hour. After cooling to room temperature, the target composites were obtained. The reaction system was protected by a continuous flow of nitrogen gas to avoid undesirable oxidation reactions. Then, the resultant composite was physically mixed with the powder of CSH at different ratios. The mixture was extruded at 170°C using a twin-screw extruder, air-cooled, and pulverized using a mill into a powder of particle size in the range of 38 to 125 μm. The n-CDHA/MAC/CSH composites with different weight percentages of n-CDHA/MAC (ie, 10%, 20%, 30%, and 40%) were obtained and moistened by distilled water with a liquid to powder (L/P) ratio of 0.8 mL/g. The mixture was further molded into cylinders and disks before use.
Characterization of n-CDHA/MAC/CSH composite
The composition and structure of the n-CDHA/MAC/CSH composite containing 30% n-CDHA/MAC were characterized by X-ray diffraction (D/Max-250; Rigaku Corporation, Tokyo, Japan) and Fourier transform infrared spectroscopy (170SX FT-IR Spectrometer; Nicolet, Madison, WI, USA). To observe the morphology of the cement after setting, the cement sample was fractured in liquid nitrogen, and a cross-sectional specimen was prepared for Scanning electron microscopy (SEM).
Measurement of setting time
The setting time of the cements with 0%–40% of n-CDHA/MAC was measured by the Vicat needle test according to the international standard ISO9579-1989E under an environment of 98% humidity and 37°C.Citation19 The initial setting time was defined as the time from the start of mixing to the plunge of the light needle (280 g, Φ 1.13 mm) into the paste and had a span of 5±1 mm to the tube bottom. The final setting time was defined as the time until the heavy needle (350 g, ø2.0 mm) no longer left a visible impression on the surface of the paste. The analysis of each sample was repeated five times, and the results were expressed as mean ± standard deviation (SD).
Compressive strength measurement
The composite samples containing different amounts of n-CDHA/MAC were prepared in a cylindrical form (Φ 10 × 12 mm). The samples were placed in an incubator at 37°C and 100% humidity for 7 days. After incubation, the samples were measured for their compressive strength by using a mechanical testing machine (REGER 30–50; Shenzhen Reger Instrument Co., Ltd, Shenzhen, People’s Republic of China) with 50 kN load cells. The crosshead speed was 5 mm/minute, and the load was applied until the specimens were compressed to about 20% of their original height. The analysis of each sample was repeated five times, and the results were expressed as mean ± SD.
Cell culture and preparation of n-CDHA/MAC/CSH-based disks
MC3T3-E1 cells (a clonal osteogenic cell line derived from newborn mouse calvarial osteoblasts)Citation20 were cultured in alpha-modified Eagle’s Minimum Essential Medium (α-MEM; HyClone; Thermo Fisher Scientific, Waltham, MA, USA) with 10% fetal bovine serum (FBS; HyClone; Thermo Fisher Scientific) plus 1% penicillin/streptomycin (Beyotime; Beyotime Institute of Biotechnology, Shanghai, People’s Republic of China) at 37°C in a humidified 5% CO2 atmosphere. The n-CDHA/MAC/CSH cement and pure CSH cement were individually made into disks (Φ 12 × 2 mm) and sterilized by ethylene oxide gas. The disks were placed into 24-well plates and used for the following cell experiments. Blank culture plates were used as a control.
Cell morphology
The disks (Φ 12 × 2 mm) mentioned above were placed into 24-well plates and prewetted in the culture medium for 24 hours. After incubation, approximately 3 × 104 MC3T3-E1 cells were seeded onto the surface of each disk. After 3 days of culture, the cell/disk constructs were washed to clean away nonattached cells and fixed with 2% glutaraldehyde solution for 2 hours at room temperature, followed by dehydration through a graded series of ethanol. Finally, the specimens were freeze-dried in vacuum, sputter-coated with gold, and examined using a scanning electron microscope (Hitachi S-3000N; Hitachi, Tokyo, Japan).
Measurement of alkaline phosphatase (ALP) activity
As described above, approximately 3 × 104 MC3T3-E1 cells were seeded onto the surface of each disk. The cells were harvested on days 1, 4, and 7 and subjected to assessment of ALP activity, using the Alkaline Phosphatase Colorimetric Assay Kit (ImmunoWay Biotechnology Company; Newark, DE, USA) according to the protocol supplied by the manufacturer. Briefly, the adherent cells were collected and suspended in a cell lysis buffer containing 0.1% Triton-X100. Following three cycles of freezing/thawing, cell lysates were centrifuged at 3,000 rpm for 20 minutes at 4°C and the supernatant was collected for the assays of ALP activity. The absorbance at 405 nm was recorded using a microplate reader, and the ALP activity was calculated from a standard curve. Total protein content in the supernatant was determined by the bicinchoninic acid method using the Beyotime protein assay kit (Beyotime Institute of Biotechnology). ALP levels were normalized to the total protein content.
Animals and surgery
Ethical approval was obtained from the Animal Care and Ethics Committee of Chongqing Medical University of China. Forty-eight skeletally mature New Zealand White rabbits (2.5–3.5 kg) were used in this study for the establishment of critical size defects in the femoral condyle. The animals were anesthetized by intravenous injection of 3% pentobarbital sodium (Gen-View Scientific; El Monte, CA, USA). A longitudinal incision of about 5 cm in the lateral distal femur was performed to expose the femoral condyle. A cavitary defect of 6.5 mm in diameter and 1 cm in depth was created by a micro-burr with a 6.5 mm tip and saline irrigation was used to minimize thermal damage. After washing, the n-CDHA/MAC/CSH cement containing 30% n-CDHA/MAC and medical grade calcium sulfate slurry (Osteoset; Wright Medical Technology Inc, Oakbrook Terrace, IL, USA) was injected into the bone defect. The choice of 30% n-CDHA/MAC was mainly due to an optimal setting time and mechanical strength. The untreated cavitary defect served as a control. At weeks 4, 8, and 16 after surgery, the animals were sacrificed by administering an overdose of pentobarbital sodium and the bone specimens were harvested for further analysis.
Micro computed tomography (micro-CT) analysis
High-resolution images of all specimens were obtained using a micro-CT scanner (Viva CT40; SCANCO Medical AG, Brüttisellen, Switzerland) running at 70 kV and 114 μA. The sagittal and coronal images were reconstructed in a three-dimensional format. The quality of new formed bone was analyzed by calculating the percent bone volume (bone volume/total volume [BV/TV]) and trabecular number (Tb.N) in the central region of circular bone defects using the manufacturer’s software (SCANCO Medical AG).
Histological analysis
The bone specimens were fixed in 10% buffered formaldehyde, dehydrated by increasing concentrations of ethanol, and embedded in poly(methyl methacrylate) without decalcification. The specimens were cut longitudinally into approximately 10 μm sections and stained with the Van Gieson stain. The stained slides were observed under a light microscope (BX51; Olympus Corporation, Tokyo, Japan).
Statistical analysis
All data are presented as mean ± SD. Statistical difference was analyzed using one-way analysis of variance and Tukey’s multiple comparison tests. A value of P<0.05 was considered to be statistically significant.
Results
X-ray diffraction analysis
The X-ray diffraction patterns of the composite with 30 wt% n-CDHA/MAC, MAC, CSH, and n-CDHA are shown in . The peaks at 2θ =20.0° and 23.8° corresponded to the MAC () and those at 2θ =14.8°, 25.8°, 29.8°, 31.9°, 42.3°, 49.4°, and 55.1° corresponded to the CSH (). The peak positions at 2θ =11.5°, 25.7°, 31.8°, 32.7°, 34°, 39.7°, 47.1°, 49.6°, and 53° shown in indicated an apatite structure (). All of the aforementioned peaks appeared in the X-ray diffraction profile of the n-CDHA/MAC/CSH composite (). Of note, the peaks at 2θ =25.7°, 31.9°, and 49.3° for n-CDHA and CSH were overlapped. Taken together, these results indicate that the n-CDHA/MAC/CSH composite is composed of n-CDHA, MAC, and CSH, and each component in the basic crystal structure does not change.
Figure 1 X-ray diffraction patterns of the composite cement with 30% n-CDHA/MAC (A), MAC (B), CSH (C), and n-CDHA (D).
Abbreviations: CSH, calcium sulfate hemihydrate; n-CDHA, nano calcium-deficient hydroxyapatite; n-CDHA/MAC, nano calcium-deficient hydroxyapatite/multi(amino acid) copolymer; MAC, multi(amino acid) copolymer.
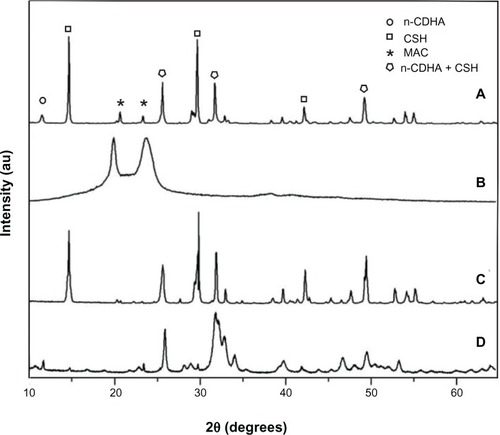
Infrared spectroscopy analysis
shows infrared spectra of the composite with 30 wt% n-CDHA/MAC, MAC, CSH, and n-CDHA. The peak of MAC at 3,415 cm−1 represented the stretching vibration of nitrogen–hydrogen (NH), and the bands between 2,935 cm−1 and 2,860 cm−1 were attributed to carbon–hydrogen (CH2) vibration (). The band at 1,538 cm−1 belonged to the stretching vibration of carbon–nitrogen (CN). The band at 1,638 cm−1 was assigned to carbonyl vibration (C=O). These peaks indicate an amide copolymer structure. The peaks of CSH at 1,619, 1,117, 669, 600, and 463 cm−1 were assigned to SO42−. The stretching mode of CSH crystalline water was also observed at 3,601 and 3,556 cm−1 (). In terms of the infrared spectrum of n-CDHA (), the peaks at 3,570 and 605 cm−1 reflected the vibration of the hydroxyl (OH) group, and the peaks at 566, 1,034, and 1,094 cm−1 corresponded to PO43−. These typical peaks of n-CDHA, MAC, and CSH were detected in the infrared spectrum of the n-CDHA/MAC/CSH composite (), indicating that this composite is composed of the three components.
Figure 2 Infrared spectra of the composite cement with 30% n-CDHA/MAC (A), MAC (B), CSH (C), and n-CDHA (D).
Abbreviations: CSH, calcium sulfate hemihydrate; n-CDHA, nano calcium-deficient hydroxyapatite; n-CDHA/MAC, nano calcium-deficient hydroxyapatite/multi(amino acid) copolymer; MAC, multi(amino acid) copolymer.
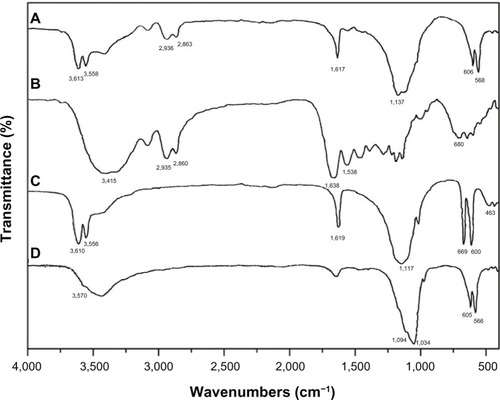
Morphological characterization of n-CDHA/MAC/CSH
The surface morphology of the n-CDHA/MAC/CSH composite containing 30% n-CDHA/MAC and pure CSH observed by SEM is shown in . As shown in , the pure CSH showed rod-like crystal morphology. In contrast, the n-CDHA/MAC/CSH composite exhibited a rough surface with some micropores of 100–200 μm in diameter (). Higher magnification images further revealed that CSH had been integrated into the n-CDHA/MAC matrix ().
Figure 3 The surface morphology of the CSH (A and B) and composite cement with 30% n-CDHA/MAC (C and D) determined by scanning electron microscopy.
Notes: Black arrows indicate the CSH crystal, white arrows indicate the n-CDHA/MAC.
Abbreviatons: CSH, calcium sulfate hemihydrate; n-CDHA/MAC, nano calcium-deficient hydroxyapatite/multi(amino acid) copolymer.
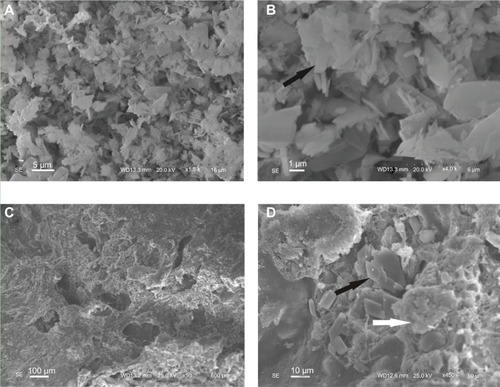
Self-setting properties
We checked whether the ratio of n-CDHA/MAC to CSH affected the injectability of the n-CDHA/MAC/CSH composite. As shown in , the setting time of the n-CDHA/MAC/CSH composite increased with the elevation in weight percentages of n-CDHA/MAC. The composite with 40% n-CDHA/MAC had a final setting time of over 60 minutes. In addition, the composite did not show any demixing or filter pressing during extrusion from the syringe. These results suggest that the proportion of n-CDHA/MAC has a retarding effect on the setting time of the n-CDHA/MAC/CSH cement.
Compressive strength measurement
We also assessed the effects of the ratio of n-CDHA/MAC to CSH on the compressive strength of the n-CDHA/MAC/CSH composite. As shown in , elevated percentages (up to 30%) of the n-CDHA/MAC composition markedly increased the compressive strength of the n-CDHA/MAC/CSH composite after setting. However, when the weight percentage of the n-CDHA/MAC component reached 40%, the compressive strength of the composite was significantly (P<0.05) decreased as compared with that of 30% n-CDHA/MAC.
Cell morphology
SEM analysis revealed that after 3 days of culture, MC3T3-E1 cells spread well on the surface of both the n-CDHA/MAC/CSH composite with 30% n-CDHA/MAC and pure CSH (). At day 7, the cultured MC3T3-E1 cells in each group aggregated with each other to form clusters ().
Figure 6 SEM micrographs of MC3T3-E1 cells adhesion on the surface of n-CDHA/MAC/CSH and CSH after 3 days (A and B) and 7 days (C and D) of culture.
Notes: (A and C) n-CDHA/MAC/CSH; (B and D) CSH. Arrows indicate the cells grown on the biocomposites.
Abbreviations: CSH, calcium sulfate hemihydrate; n-CDHA/MAC/CSH, nano calcium-deficient hydroxyapatite/multi(amino acid) copolymer/calcium sulfate hemihydrate; SEM, scanning electron microscopy.
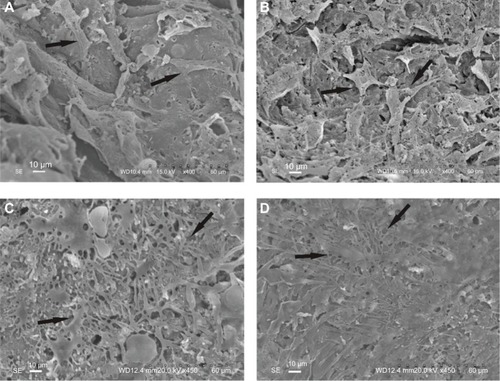
ALP activity
The ALP activities of MC3T3-E1 cells cultured on the n-CDHA/MAC/CSH composite with 30% n-CDHA/MAC and CSH (as a control) were assessed at days 1, 4, and 7, and the results are shown in . The ALP activity increased over time in all groups. No significant difference in the ALP activity was noted between the n-CDHA/MAC/CSH composite with 30% n-CDHA/MAC and CSH at day 1. However, at days 4 and 7, the ALP activity in the n-CDHA/MAC/CSH group was significantly (P<0.05) greater than in the control group.
Figure 7 ALP activity of MC3T3-E1 cells cultured on n-CDHA/MAC/CSH cement, CSH cement, and TCP as controls.
Note: *P<0.05.
Abbreviations: ALP, alkaline phosphatase; CSH, calcium sulfate hemihydrate; n-CDHA/MAC/CSH, nano calcium-deficient hydroxyapatite/multi(amino acid) copolymer/calcium sulfate hemihydrate; TCP, tissue culture plate.
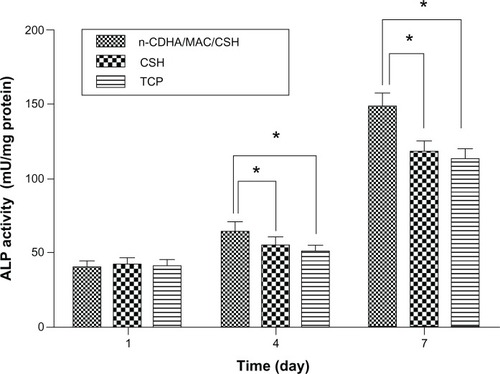
Micro-CT results
The micro-CT scanning images of the repaired femoral condyles are shown in . It can be seen in the specimens at week 4 post-implantation; the bone defects were filled with mineralized implants and new bone started to form in the n-CDHA/MAC/CSH and CSH groups (); we found no signs of healing in the untreated group (). At week 8, cortical parts of the n-CDHA/MAC/CSH- and CSH-treated bone defects were markedly restored with new bone. However, the bone cavity in the untreated group was visible at week 8 (). In the central area of the defect, the boundary between the implants and regenerated bone was indistinguishable (). In the n-CDHA/MAC/CSH- and CSH-treated groups, the defects were completely repaired at week 16. Moreover, the trabecular density as determined by visual inspection was higher in the n-CDHA/MAC/CSH group than in the CSH group (). As expected, the untreated group still showed an evident defect at the end of the experiment ().
Figure 8 Representative coronal micro-CT images revealed the repair of bone defects in each group at postoperative 4, 8, and 16 weeks: (A, D and G) n-CDHA/MAC/CSH cement group, (B, E and H) CSH cement group, and (C, F and I) untreated control group.
Note: The arrow indicates the defect site.
Abbreviations: CSH, calcium sulfate hemihydrate; n-CDHA/MAC/CSH, nano calcium-deficient hydroxyapatite/multi(amino acid) copolymer/calcium sulfate hemihydrate; micro-CT, micro computed tomography
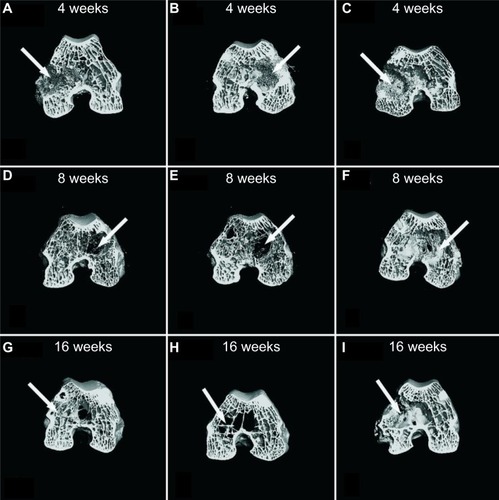
To measure the amount and quantity of new bone formation, percent bone volume (BV/TV%) and Tb.N in each group were determined by micro-CT analysis. As shown in , BV/TV% and Tb.N values increased from 4 to 16 weeks in all groups. The values of BV/TV% and Tb.N in the n-CDHA/MAC/CSH- and CSH-treated groups were significantly higher than the untreated group at each time point after implantation (P<0.05). Although there were comparable values between the n-CDHA/MAC/CSH- and CSH-treated groups at week 4, a significant difference in BV/TV% was found at weeks 8 and 16. Additionally, the n-CDHA/MAC/CSH-treated group had a significantly higher value of Tb.N at 4, 8, and 16 weeks after implantation, as compared to the CSH-treated group (P<0.05).
Figure 9 Quantitative analysis of bone defect repair at 4, 8, and 16 weeks after implantation with n-CDHA/MAC/CSH cement, CSH cement, or no cement filling (blank).
Notes: (A) BV/TV%; (B) Tb.N; *P<0.05.
Abbreviations: BV/TV%, percent bone volume; CSH, calcium sulfate hemihydrate; n-CDHA/MAC/CSH, nano calcium-deficient hydroxyapatite/multi(amino acid) copolymer/calcium sulfate hemihydrate; Tb.N, trabecular number.
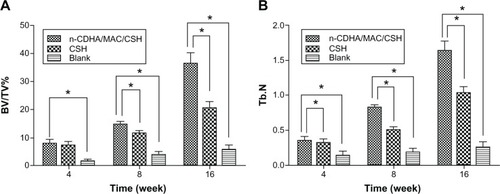
Histological findings
Representative micrographs of undecalcified bones from indicated groups at 4, 8, and 16 weeks after implantation are shown in . In the n-CDHA/MAC/CSH-treated group, a small amount of newly formed bone grew into the inner site of the n-CDHA/MAC/CSH cement at week 4 post-implantation, coupled with generation of immature bone among it. More newly formed bone tissues were found at week 8, while the volume of cement was decreased due to degradation. At week 16, the defects were completely repaired by mature trabecular bone, and there was only a small amount of residual nondegradable cement. The CSH cement displayed a rapid degradation compared to the n-CDHA/MAC/CSH cement. The implanted CSH cement was divided into several small pieces at week 4 and almost completely degraded at week 8. At week 16, the number and density of trabecular bone were markedly lower in the CSH-treated group than in the n-CDHA/MAC/CSH-treated group.
Figure 10 Van Gieson stained sections of n-CDHA/MAC/CSH cement (A, C and E) and CSH cement (B, D and F) harvested at 4 (A and B), 8 (C and D), and 16 (E and F) weeks post-implantation (magnification 40×).
Notes: Black arrows denote newly formed bone tissue, white arrows denote n-CDHA/MAC/CSH cement, and red arrows denote CSH cement.
Abbreviations: CSH, calcium sulfate hemihydrate; n-CDHA/MAC, nano calcium-deficient hydroxyapatite/multi(amino acid) copolymer.
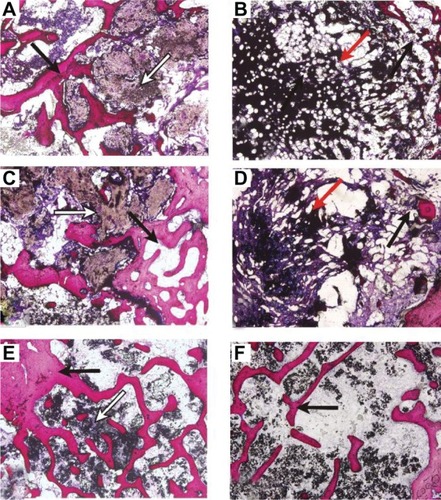
Discussion
An ideal artificial bone graft substitute should have good biocompatibility, osteoinductivity, osteoconductivity, and biodegradability.Citation21 Ceramic/polymer composite materials are considered to play an important role in bone regeneration.Citation22 Recent studies have shown that n-CDHA at a lower Ca/P ratio (1.5–1.67) is more biologically active than the nano hydroxyapatite because the former has similar compositions and crystal structures to the mineral of nature bone.Citation23,Citation24 The addition of MAC to n-CDHA results in an improvement in the mechanical strength and ductility. However, the granular form of n-CDHA-MAC renders it difficult to be applied in irregular, complex bone injuries. In this study, we developed and characterized an injectable system based on n-CDHA-MAC and evaluated its application in treatment of bone defects.
The injectability and setting time greatly influence the applicability of an injectable self-setting biomaterial. Our present data revealed that compared with pure CSH, the n-CDHA/MAC/CSH composite shows a retarded setting time. The initial setting duration for the n-CDHA/MAC/CSH composites containing different proportions of n-CDHA/MAC varies from 10 to 30 minutes, which is consistent with previous studies.Citation19,Citation25 In contrast, the initial setting time for pure CSH is as short as 5 minutes. Clinically, the initial setting time of bone cements should be ideally controlled between 15–25 minutes. Taking into account that injectable cements must be delivered to bone defects before initial setting, the prolonged duration for self-setting confers an apparent advantage in clinical applications. However, when the weight percentage of n-CDHA/MAC in the n-CDHA/MAC/CSH composite is increased to 40% or more, the length of setting time is too long to meet the demands of surgery. Therefore, establishment of an optimal proportion of n-CDHA/MAC is critical for developing the injectable n-CDHA/MAC/CSH system.
Mechanical strength is another important parameter for bone graft substitutes. Generally, the adult’s compressive strength varies between 100 and 200 MPa for cortical bone, and between 2 and 20 MPa for cancellous bone.Citation26 We found that after complete solidification, the compressive strength for the n-CDHA/MAC/CSH composite with 30% n-CDHA/MAC increases to about 45 MPa, indicating that this material is suitable for clinical use. It is noteworthy that when the n-CDHA/MAC concentration in the n-CDHA/MAC/CSH composite reached 40%, the compressive strength of this composite decreased instead of further increasing. This may be explained by the mechanical strength of this composite being largely influenced by the self-transformation of CSH (CaSO4 · 1/2H2O) to calcium sulfate dihydrate (CSD; CaSO4 · 2H2O). Such transformation involves three main steps, ie, hydration, setting, and solidification. CSD crystals form an intertwined structure, which is regarded as a major source of mechanical strength.Citation27 Inclusion of too much (eg, 40% or more) of the n-CDHA/MAC compound may diminish the production of CSD and form a loose complex structure, consequently leading to reduced compressive strength.
The ability to bond to osseous tissue is a vital index to evaluate the bioactivity of bioactive materials. Attachment, adhesion, and spreading in bone implants are required for osteogenic cells to proliferate and differentiate.Citation28 The surface property of bioactive materials is thought to have an important influence on these cellular functions. As determined by the SEM analysis, we found that MC3T3-E1 cells spread well on both the n-CDHA/MAC/CSH composite and pure CSH, suggesting an excellent bioactive property. It has been documented that the MAC containing –COOH, –NH2, and –NH–C(O) groups can promote adsorption of adhesive proteins such as fibronectin and vitronectin, which help cells attach to the material.Citation13,Citation29 Therefore, the incorporation of n-CDHA into the MAC matrix may improve the biological properties of the composite material and has positive effects on cell proliferation and viability.Citation24 ALP is an early marker for osteoblast differentiation and participates in the process of mineralization.Citation30 Our data showed that the ALP activity in MC3T3-E1 cells cultured on the n-CDHA/MAC/CSH composite is significantly higher than in the cells cultured on pure CSH or control plates at 4 and 7 days of culture, indicating a promotion of osteogenesis by the n-CDHA/MAC/CSH composite. Taken together, the results demonstrate that the n-CDHA/MAC/CSH composite has good biocompatibility.
A critical size defect is defined as the smallest tissue defect that would not completely heal during the lifetime of an animal. It has been suggested that critical size defects are suitable for testing bone repair materials or methods.Citation31 In this study, a cancellous bone site was chosen for generation of bone defects due to the equilibrium between osteogenesis and biodegradation activities.Citation32 Based on the above considerations, a critical size defect in the rabbit femoral condyle was used to evaluate the biocompatibility and osteogenesis of n-CDHA/MAC/CSH composite in vivo. In rabbits, a 6 mm bone defect, which was established in this study, is generally regarded as the critical size defect.Citation33 During the experiment, all the n-CDHA/MAC/CSH-treated rabbits remained in good health and did not show any fracture and wound complications. After sampling, no chronic inflammation and adverse tissue reactions were observed. These findings suggest that this composite exhibits excellent biocompatibility in vivo.
It has been documented that the addition of mineralized collagen (nano hydroxyapatite/collagen) improves the performance of CSH in bone regeneration.Citation34 In agreement with this study, we also found that the n-CDHA/MAC/CSH implants accelerated new bone formation in the treatment of critical size defects, compared to the pure CSH implants. Moreover, the n-CDHA/MAC/CSH implant contacted with the host bone intimately, without causing any evident adverse effect on surrounding soft tissues. In contrast, no evident bone formation was detected in the untreated control group at the end of the experiment and, instead, the fibrous tissue grew into the defects. These findings suggest that the n-CDHA/MAC/CSH composite may prevent fibrous tissue in-growth into bone defects and guide bone repair effectively.
Biodegradability is another crucial factor for bone graft substitutes in bone tissue regeneration. An ideal bone graft substitute should be able to degrade with time in vivo, preferably at a controlled resorption rate and eventually creating space for the new bone tissue to grow.Citation35 Previous studies reported that calcium sulfate resorption is nearly completed by 6 weeks, suggesting that it is not feasible for long-term clinical use.Citation36,Citation37 Our data revealed that in the n-CDHA/MAC/CSH-treated group, new bone regenerated and gradually penetrated into the cement, which was accompanied by the resorption of the cement. This cement was almost completely degraded until 16 weeks, when the bone defect was repaired. This suggests that the n-CDHA/MAC/CSH composite cement not only has good biocompatibility, but also appropriate biodegradability and osteoinductivity.
Conclusion
In summary, we developed a novel injectable composite cement by incorporating n-CDHA/MAC into the CSH matrix. The setting time and injectability of this composite material are significantly influenced by the weight ratio between the CSH and n-CDHA/MAC components. In vitro cell studies showed that the composite cement is more effective in promoting cell proliferation and adhesion of MC3T3-E1 osteogenic cells compared with pure CSH. Macroscopic observation of the composite cement implanted into the bone defects of rabbits did not cause undesirable tissue reactions such as inflammation and necrosis. Radiological and histological studies confirmed that the n-CDHA/MAC/CSH composite cement facilitates new bone formation in vivo. These findings indicate that the injectable n-CDHA/MAC/CSH composite is a promising candidate for bone regeneration as a bone graft substitute, which shows good biocompatibility, bioactivity, and bioresorbability. Further studies are needed to investigate the feasibility of n-CDHA/MAC/CSH in other animal models and clinical settings and compare this novel composite with other clinically used bone cements.
Acknowledgments
This work is supported by the National Natural Science Foundation of China (81171685).
Disclosure
The authors report no conflicts of interest in this work.
References
- SummersBNEisensteinSMDonor site pain from the ilium. A complication of lumbar spine fusionJ Bone Joint Surg Br19897146776802768321
- WheelerDLEnnekingWFAllograft bone decreases in strength in vivo over timeClin Orthop Relat Res2005435364215930919
- WangHLiYZuoYLiJMaSChengLBiocompatibility and osteogenesis of biomimetic nano-hydroxyapatite/polyamide composite scaffolds for bone tissue engineeringBiomaterials200728223338334817481726
- LeGerosRZLinSRohanizadehRMijaresDLeGerosJPBiphasic calcium phosphate bioceramics: preparation, properties and applicationsJ Mater Sci Mater Med200314320120915348465
- LacknerJMJaccardJBlanchardEBTesting the sequential model of pain processing in irritable bowel syndrome: a structural equation modeling analysisEur J Pain20059220721815737813
- WitteFCalliessTWindhagenHBiodegradable synthetic implant materials: clinical applications and immunological aspectsOrthopade2008372125130 German18214423
- WeiGMaPXStructure and properties of nano-hydroxyapatite/polymer composite scaffolds for bone tissue engineeringBiomaterials200425194749475715120521
- CuiYLiuYCuiYJingXZhangPChenXThe nanocomposite scaffold of poly(lactide-co-glycolide) and hydroxyapatite surface-grafted with L-lactic acid oligomer for bone repairActa Biomater2009572680269219376759
- WangZLiMYuBCaoLYangQSuJNanocalcium-deficient hydroxyapatite-poly (e-caprolactone)-polyethylene glycol-poly (e-caprolactone) composite scaffoldsInt J Nanomedicine201273123313122848159
- LiHGongMYangAMaJLiXYanYDegradable biocomposite of nano calcium-deficient hydroxyapatite-multi(amino acid) copolymerInt J Nanomedicine201271287129522457591
- GuoHSuJWeiJKongHLiuCBiocompatibility and osteogenicity of degradable Ca-deficient hydroxyapatite scaffolds from calcium phosphate cement for bone tissue engineeringActa Biomater20095126827818722167
- FuchsSJiangXGotmanIInfluence of polymer content in Ca-deficient hydroxyapatite-polycaprolactone nanocomposites on the formation of microvessel-like structuresActa Biomater2010683169317720144913
- LiHYanYWeiJBone substitute biomedical material of multi(amino acid) copolymer: in vitro degradation and biocompatibilityJ Mater Sci Mater Med201122112555256321898161
- ConstantzBRIsonICFulmerMTSkeletal repair by in situ formation of the mineral phase of boneScience19952675205179617997892603
- BorrelliJJrPrickettWDRicciWMTreatment of nonunions and osseous defects with bone graft and calcium sulfateClin Orthop Relat Res200341124525412782881
- UrbanRMTurnerTMHallDJInfangerSCheemaNLimTHHealing of large defects treated with calcium sulfate pellets containing demineralized bone matrix particlesOrthopedics200326Suppl 5s581s58512755230
- ThomasMVPuleoDACalcium sulfate: Properties and clinical applicationsJ Biomed Mater Res B Appl Biomater200988259761019025981
- HuNMChenZLiuXMechanical properties and in vitro bioactivity of injectable and self-setting calcium sulfate/nano-HA/collagen bone graft substituteJ Mech Behav Biomed Mater20121211912822705910
- HuanZChangJSelf-setting properties and in vitro bioactivity of calcium sulfate hemihydrate-tricalcium silicate composite bone cementsActa Biomater20073695296017588507
- SudoHKodamaHAAmagaiYYamamotoSKasaiSIn vitro differentiation and calcification in a new clonal osteogenic cell line derived from newborn mouse calvariaJ Cell Biol19839611911986826647
- KhanYYaszemskiMJMikosAGLaurencinCTTissue engineering of bone: material and matrix considerationsJ Bone Joint Surg Am200890Suppl 1364218292355
- RezwanKChenQZBlakerJJBoccacciniARBiodegradable and bioactive porous polymer/inorganic composite scaffolds for bone tissue engineeringBiomaterials200627183413343116504284
- LinJHKuoKHDingSJJuCPSurface reaction of stoichiometric and calcium-deficient hydroxyapatite in simulated body fluidJ Mater Sci Mater Med200112873174115348246
- SponerPStrnadováMUrbanKIn vivo behaviour of low-temperature calcium-deficient hydroxyapatite: comparison with deproteinised bovine boneInt Orthop201135101553156020721552
- LiuXWangXMChenZInjectable bone cement based on mineralized collagenJ Biomed Mater Res B Appl Biomater2010941727920336741
- BoseSRoyMBandyopadhyayARecent advances in bone tissue engineering scaffoldsTrends Biotechnol2012301054655422939815
- NilssonMWielanekLWangJSTannerKELidgrenLFactors influencing the compressive strength of an injectable calcium sulfate-hydroxyapatite cementJ Mater Sci Mater Med200314539940415348442
- AnselmeKOsteoblast adhesion on biomaterialsBiomaterials200021766768110711964
- PompeTKellerKMothesGSurface modification of poly(hydroxybutyrate) films to control cell-matrix adhesionBiomaterials2007281283716963116
- MaromRShurISolomonRBenayahuDCharacterization of adhesion and differentiation markers of osteogenic marrow stromal cellsJ Cell Physiol20052021414815389528
- MaJLPanJLTanBSCuiFZDetermination of critical size defect of minipig mandibleJ Tissue Eng Regen Med20093861562219731258
- LuJXGallurAFlautreBComparative study of tissue reactions to calcium phosphate ceramics among cancellous, cortical, and medullar bone sites in rabbitsJ Biomed Mater Res19984233573679788497
- HollingerJOKleinschmidtJCThe critical size defect as an experimental model to test bone repair materialsJ Craniofac Surg19901160681965154
- LiuHYLiuXZhangLPAiHJCuiFZImprovement on the performance of bone regeneration of calcium sulfate hemihydrate by adding mineralized collagenTissue Eng Part A20101662075208420136401
- WilliamsDFOn the mechanisms of biocompatibilityBiomaterials200829202941295318440630
- TurnerTMUrbanRMGitelisSHaggardWORichelsophKResorption evaluation of a large bolus of calcium sulfate in a canine medullary defectOrthopedics200326Suppl 5s577s57912755229
- StubbsDDeakinMChapman-SheathPIn vivo evaluation of resorbable bone graft substitutes in a rabbit tibial defect modelBiomaterials200425205037504415109866