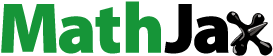
Abstract
The first C60(OH)24-DNA complex and its fluorescence enhancement is reported. The enhanced fluorescence intensity of fullerenol C60(OH)24 is in proportion to the concentration of DNA in the range of 1 × 10−9 to 8 × 10−5 molL−1 and the detection limit was 1.3 ng mL−1. Fullerenol C60(OH)24 binds significantly to the phosphate backbone of native dsDNA and to base-pairs within the major groove of sodium salt of dsDNA.
Introduction
Nanoscale materials seems to offer great opportunities for biomedical applications such as therapeutic and diagnostic tools.Citation1–Citation9 Biomedical applications under development include drug delivery systems targeted to the brain and cancer tissues, gene transfection, and intravascular nanosensor and nanorobotic devices for imaging and diagnosis.Citation3,Citation9
In this context, the biological activities of fullerene derivatives have attracted much attention in the past 25 years.Citation10–Citation17 As potent free-radical scavengers and antioxidantsCitation18–Citation23 the water-soluble polyhydroxylated [C60]fullerenes, fullerenols, exhibit an exciting range of biological activities as glutamate receptor antagonists,Citation24 antiproliferative,Citation25–Citation27 neuroprotective,Citation28–Citation31 and anticancer agents.Citation32–Citation37
Knowing the ways fullerenols interact with proteins and nucleotides is a prerequisite for understanding their biological effects at membrane penetration and the intracellular level, only two studies deal with their binding to proteinsCitation38,Citation39 and their interaction with DNA has not been reported to date.
On the other hand, the solution-based assays and quantitative analysis of nucleic acids are critical in current biochemistry and biomedical science. Throughout the years, a number of fluorimetric methods for the determination of nucleic acids have been developed with ethidium bromide,Citation40–Citation42 lanthanide cations,Citation43–Citation45 ruthenium complexes,Citation46–Citation48 and asymmetric cyanine dyes as fluorescence probes.Citation49–Citation51
Despite the prominence of fullerenes in bionanotechnology, the exploration of their fluorescent properties in solution remains still at a very early age. Several studies have been devoted to dsDNA/single-walled carbon nanotube hybrid systems,Citation52,Citation53 but only very few deal with their fluorescent proprietiesCitation54,Citation55 when dispersed in aqueous solution.
Herein, we are happy to report the first complexation of dsDNA with C60(OH)24 in aqueous media in the absence of a buffer in physiological pH-range.
Materials and methods
C60 (99.5+%) was purchased from MER Corp (Tuscon, AZ, USA). KOH (99.99%, semiconductor grade) was purchased from Sigma-Aldrich (St. Louis, MO, USA). DNA (low molecular weight, salmon sperm) was purchased from Fluka (St. Louis, MO, USA). All other reagents were purchased from Sigma-Aldrich.
Fluorescence spectroscopy was performed with a Perkin Elmer LS55 spectrometer Perkin Elmer, Wellesley, MA, USA). To prepare fluorescence samples, the only operation was the mixing of two solutions before fluorescence measurements. X-ray photoelectron spectroscopy (XPS) measurements were carried out using a Leybold LHS 10 spectrometer (Leybold, Cologne, Germany).
To the best of our knowledge, most of these fullerenols are not pure C60(OH)n, but a complex mixture of products. For instance, those synthesized through sulfuric/nitric acid,Citation56 hydroboration,Citation57 or nitronium chemistryCitation58 afforded products with average composition of C60Ox(OH)y. The so-called fullerenols prepared by alkaline polyhydroxylation of C60 under phase transfer conditionsCitation59 are not simply C60(OH)x, but stable radical anions with the molecular formula, Na+n[C60Ox(OH)y]n−,Citation60 and the fullerenol obtained by alkaline hydrolysis of C60Br24 is not C60(OH)24 as claimed by Bogdanovi and Dvordjevic,Citation37,Citation61 but C60(ONa)8(OH)16.Citation62 In the light of this, many biomedical studies involving fullerenols species in the literature may need to be reconsidered. The pure fullerenol C60(OH)24 used in this study was prepared by a modified method of alkaline hydrolysis of C60Br24,Citation62 followed by demetallation of the obtained C60(OK)8(OH)16 with a cation exchange resin and exhaustive purification by dialysis.
Representative procedure for synthesis of C60(OH)24
All experiments were performed with Schlenk techniques under argon and protected from light. According to the literature, before the synthesis of the polyhydroxylated fullerene, bromofullerene C60Br24 was synthesized first.Citation62 In the synthesis of the C60(OH)24, to a sonicated (40 W, 15 min) suspension of C60Br24 (200 mg, 0.075 mmol) in de-aerated water (100 mL), fresh KOH (200 mg, 3.57 mmol) was added under argon protection and stirred for 10 days at room temperature. After the reaction was completed, the resulting dark-brown solution was passed to a centrifuge at 4000 rpm for 30 min and the supernatant was brought to dryness in a rotavapor apparatus at 40 °C. The dark-brown residue was dissolved in 50 mL of deionized water, stirred with ion exchange resin AMBERJET™ 1200[H] (Rohm and Haas Company, Philadelphia, PA, USA) (20 mL) for 8 h and subjected to dialysis (Spectra/Por® 1000 D; Spectrum Laboratories, Rancho Dominguez, CA, USA) for four days. Finally, the dialyzed solution was brought to dryness in a rotavapor apparatus at 60 °C and dried at 80 °C and 10−4 Torr for 24 h. The fullerenol thus obtained contained 24 hydroxyl groups as characterized by elemental analysis, Fourier transform infrared (FT-IR) spectroscopy, and XPS spectroscopic measurements.
Elemental analysis
Calculated for C60H24O24: C, 63.82; H, 2.12. Found: C, 63.66; H, 1.98. FTIR (KBr): ν max, 3436 (−OH), 1605 (C = C), 1430 (δ −OH), 1095, 1046 (ν C-OH), 1018, 994, 825, 877, 570, 530 cm−1.
XPS analysis
C1s components: % C = C (284.6 eV) 59.77 (clcd. 60); % C-OH: (285.8 eV) 39,76 (clcd. 40); O/C = 0.42 (clcd. 0.40).
Results and discussion
The fullerenol water solution, exhibited different maxima depending on the concentration (). In the range of 1.6 × 10−5 to 4.4 × 10−5 molL−1 one fluorescent maximum was observed at 469 nm, while two fluorescence maxima where found for lower concentrations located at 469 nm and 492 nm at λex = 420 nm.
Figure 1 Fluorescence data of C60(OH)24 in aqueous media. A) Fluorescence emission spectra of C60(OH)24 after 5 min incubation in water, with excitation at 420 nm. B) Plot of fluorescence intensity versus [C60(OH)24], with excitation at 420 nm; average standard error, 3.76%.
![Figure 1 Fluorescence data of C60(OH)24 in aqueous media. A) Fluorescence emission spectra of C60(OH)24 after 5 min incubation in water, with excitation at 420 nm. B) Plot of fluorescence intensity versus [C60(OH)24], with excitation at 420 nm; average standard error, 3.76%.](/cms/asset/3bd3afb7-9d24-42df-b31f-35c16a1a5169/dijn_a_6630_f0001_b.jpg)
These emission profiles of fullerenol at different concentrations provided the baseline for understanding perturbation upon interaction with dsDNA. As shown in , the most appropriate concentrations of fullerenol in water for fluorescence measurements at λex = 420 nm are within the range of 1.6 × 10−5 to 4.4 × 10−5 molL−1 (λem = 469 nm).
As can be seen from , the fluorescence intensity of fullerenol alone is dependent on its concentration in the range of 4.4 × 10−5 to 1.6 × 10−5 molL−1 according to a very significant linear relationship.
shows the fullerenol and DNA emission spectra recorded at different excitation wavelengths. Inspection of how fluorescence emission spectra of fullerenol and DNA change as a function of excitation wavelength yield additional supporting information on the appropriate fullerenol excitation wavelength suitable for the fluorescence investigation of C60(OH)24 – DNA complex in aqueous media. One can observe that for a concentration higher than 1.6 × 10−5 molL−1, the emission spectra of fullerenol do not overlap with emission spectra of DNA when excited at 340, 360, 380, and 420 nm, respectively. Apparently, all these fluorescence excitations should be suitable for a fluorescence study of DNA-fullerenol interaction. However, the emission maxima of fullerenol at concentrations <1.6 × 10−5 molL−1 () and DNA () overlap at 492 nm when recorded at λex = 420 nm. This is the reason why, to cover a large concentration range (1−9−4.5−5 molL−1) of fullerenol, the fluorescence excitation at 420 nm and emission at 469 nm were used for fluorescence intensity measurements in this work.
Figure 2 Fluorescence emission spectra of fullerenol (- - -) and dsDNA (——) for different excitation wavelength. A) λ = 420 nm. B) λ = 380 nm. C) λ = 360 nm. D) λ = 340 nm. [C60(OH)24] = 1 × 10−4 molL−1; [DNA] = 1 × 10−4 molL−1.
![Figure 2 Fluorescence emission spectra of fullerenol (- - -) and dsDNA (——) for different excitation wavelength. A) λ = 420 nm. B) λ = 380 nm. C) λ = 360 nm. D) λ = 340 nm. [C60(OH)24] = 1 × 10−4 molL−1; [DNA] = 1 × 10−4 molL−1.](/cms/asset/8f8cbed8-6cdb-4133-9c2c-cbb10f01fed8/dijn_a_6630_f0002_b.jpg)
In , the emission spectra of DNA-fullerenol complexes, with constant DNA concentration and increasing fullerenol content are shown. It can been seen that increasing the concentration of the fullerenol results in a strong increase in fluorescence intensity of fullerenol from 50 to 500 nm, without causing any perceptible shifts of the fluorescence maximum at λ = 469 nm. In order to establish the DNA binding affinity of fullerenol, these fluorescent-enhancing data were plotted () according to the Equationequation (6)(6) derived from the equilibrium Equationequation (1)
(1) :
Figure 3 Fluorescence data of C60(OH)14 in the presence od dsDNA. A) Fluorescence emission spectra of fullerenol (——) in the presence of dsDNA (- - -) with excitation at 420 nm, as a function of fullerenol concentration; [dsDNA] = 6.31 × 10−5 molL−1. B) Plot for DNA-C60(OH)24 system as a function of fullerenol concentration in the range of 0.94 × 10−7 to 4.5 × 10−5 molL−1; [dsDNA] = 6.31 × 10−5 molL−1; average standard error, 0.17%.
![Figure 3 Fluorescence data of C60(OH)14 in the presence od dsDNA. A) Fluorescence emission spectra of fullerenol (——) in the presence of dsDNA (- - -) with excitation at 420 nm, as a function of fullerenol concentration; [dsDNA] = 6.31 × 10−5 molL−1. B) Plot for DNA-C60(OH)24 system as a function of fullerenol concentration in the range of 0.94 × 10−7 to 4.5 × 10−5 molL−1; [dsDNA] = 6.31 × 10−5 molL−1; average standard error, 0.17%.](/cms/asset/5e917492-340c-44a4-8e79-c074e8a11078/dijn_a_6630_f0003_b.jpg)
In order to evaluate the range of [DNA] determination, the binding of fullerenol to DNA was characterized through fluorescence emission titration of fullerenol. The enhancement of the fluorescence intensity of fullerenol with DNA at increasing concentrations is shown in . One can observe that even for nanoscale concentration of DNA the fluorescence intensity of fullerenol increases from 25 to 100 (). The plot in is broken down into two regimes corresponding to ranges from 1.3 × 10−9 to 3.1 × 10−6 gL−1 and 2.5 × 10−5 to 5 × 10−5 gL−1. The low [DNA] range in the plots of (detection limit = 1.3 ng/mL) are close to what can be accomplished with current available fluorescence probes, ie, Hoechst 33258 (20 ng/mL) and YO-PRO-1/YOYO-1 (0.5–2.5 ng/mL). In addition, from the shape and intensity of emission spectrum recorded for [DNA] = 2.1 × 10−9 molL−1 (), it is useful to point out that the sensitivity can be extended into lower regions.
Figure 4 Dependence of fluorescence intensity of C60(OH)24 on dsDNA concentration. A) Fluorescence spectra of fullerenol (– · –) with increasing concentration of DNA (—) with excitation at 420 nm ; B) Plot of fluorescence intensity of fullerenol as a function of [dsDNA] in the concentration range of 1.3 × 10−9 to 4.4 × 10−6 molL−1; [C60(OH)24] = 4.5 × 10−5 molL−1; average standard errors: 2.69% for 2.5E−5−5E−5 region and 10.80% for 1.3E−9−3.1E−6 region.
![Figure 4 Dependence of fluorescence intensity of C60(OH)24 on dsDNA concentration. A) Fluorescence spectra of fullerenol (– · –) with increasing concentration of DNA (—) with excitation at 420 nm ; B) Plot of fluorescence intensity of fullerenol as a function of [dsDNA] in the concentration range of 1.3 × 10−9 to 4.4 × 10−6 molL−1; [C60(OH)24] = 4.5 × 10−5 molL−1; average standard errors: 2.69% for 2.5E−5−5E−5 region and 10.80% for 1.3E−9−3.1E−6 region.](/cms/asset/b610f832-14bc-4e7b-a015-06b2cc27eb97/dijn_a_6630_f0004_b.jpg)
Figure 5 Emission spectra of C60(OH)24 (red line), DNA-sodium salt (blue line) and C60(OH)24 − DNA-sodium salt complex (green) line.
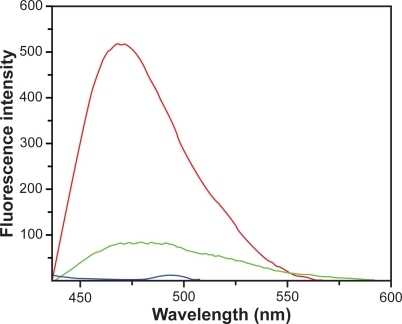
As regards the chemical interactions between fullerenol and the DNA target, the electrostatic and intercalative binding are ruled out and hydrogen-bonding interaction can only be taken under consideration. Earlier studiesCitation38,Citation39 have pointed out that hydrogen-bonding plays the main role in the interaction between fullerenols and proteins. Thus, the major groove binding of fullerenol through the hydrogen-bonding between its hydroxyl groups and free or bridged –NH2 in base-pairs of DNA is predictable.
Taking into account that phenols can interact with phosphates,Citation63 the hydrogen bonding between fullerenol and phosphate backbone of DNA can be also suggested as a possibility for the binding of fullerenols with DNA.
From the linear plot in according to Equationequation (1)(1) the value of n was estimated to be 0.8 ± 0.2. This value accounts for a single fullerenol molecule associated with a base pair of DNA. An important question is whether the fullerenol binds significantly to the phosphate backbone of DNA in addition to nonintercalative groove binding to base-pairs of DNA.
Because of its diameter (9.8 Å) the globular three-dimensional C60(OH)24 molecule does not fit the minor groove of DNA (6 Å). The width of the major groove (12 Å) is larger than 9.8 Å and thus the fullerenol molecule can fit snugly according to a nonintercalation model as shown in .
Scheme 1 Binding fullerenol C60(OH)24 to dsDNA. A) Binding fullerenol to the major groove of sodium salt of dsDNA. B) Binding fullerenol to the outside of the dsDNA.
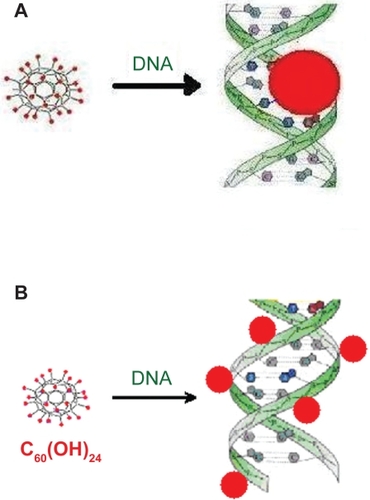
Indeed, upon binding to sodium salt of DNA (), the fluorescence intensity of fullerenol does not increase, but decreases, which suggests that only in the absence of hydrogen bond-forming P-OH moieties of DNA, fullerenol binds to base-pairs into the major groove of DNA, according to a nonintercalative model, which strongly change its average local environment. The perceptible shift of the emission maximum of fullerenol also support a strong change of its average local environment. We can thus conjecture that, under the present experimental conditions with native dsDNA, hydrogen binding to phosphate backbone to the outside of dsDNA helix is the main binding mode of fullerenol C60(OH)24 to DNA, as shown in .
Conclusions
Fullerenol C60(OH)24 binds to phosphate backbone to the outside of native dsNNA and to base-pairs within major groove of sodium salt of dsDNA. The fluorescence of fullerenol C60(OH)24 is highly enhanced by dsDNA due to the binding of the probe to DNA in a nonintercalative way. Because of its high binding affinity (K = 105 M−1) and sensitivity (1.2 × 10−9 g/mL) towards DNA, there are good prospects that C60(OH)24 will be used as versatile fluorescent probe for DNA quantification. In addition to its high sensitivity, other advantages of this fullerenol-based method include its simplicity, nontoxicity, and rapidity.
Acknowledgements
We are grateful to the Romanian Ministry of Education and Research for funding through the CNCSIS-GRANT Nr.306. The authors report no conflicts of interest in this work.
References
- WilkinsonJMNanotechnology applications in medicineMed Device Technol200314293112852120
- RocoMCNanotechnology: convergence with modern biology and medicineCurr Opin Biotechnol20031433734612849790
- TongRChengJAnticancer polymeric nanomedicinesPolymer Review200747345381
- MoghimiSMTheme editorKissel TTParticulate nanomedicinesAdv Drug Deliv Rev2006581451145517081650
- TorchilinVPTargeted pharmaceutical nanocarriers for cancer therapy and imagingAAPS J20079E128E14717614355
- ChanWCWBio-applications of NanoparticlesNew York, NYSpringer Science2007
- LammersTHenninkWEStormGTumor-targeted nanomedicines: principles and practiceBr J Cancer20089939239718648371
- MuthuMSSinghSTargeted nanomedicines: effective treatment modalities for cancer, AIDS and brain disordersNanomed2009410511819093899
- KreuterJNanoparticulate systems for brain delivery of drugsAdv Drug Deliv Rev200147658111251246
- Da RosTTwenty years of promises: Fullerenes in medicinal chemistryCataldoFDa RosTMedicinal Chemistry and Pharmacological Potential of Fullerenes and Carbon NanotubesNew York, NYSpringer-Verlag2008
- InjacRRadicNGovedaricaBDvordjevicABorutSBioapplication and activity of fullerenol C60(OH)24Afr J Biotech2008749404950
- YamawakiHIwaiNCytotoicity of water-soluble fullerene in vascular endothelial cellsAm J Physiol Cell Physiol200629014951502
- CuiDMedicinal chemistry and pharmacological potential of fullerenes and carbon nanotubesCataldoFDa RosTFunctionalized Carbon Nanotubes and their ApplicationsNew York, NYSpringer-Verlag2008
- BeuerleFLebovutzRHirschAAntioxidant properties of water-soluble fullerene derivativesCataldoFDa RosTMedicinal Chemistry and Pharmacological Potential of Fullerenes and Carbon NanotubesNew York, NYSpringer-Verlag2008
- BosiSDa RosTSpallutoGBalzariniJPratoMSynthesis and ant-HIV propreties of new water-soluble bis-functionalized [C60]fullerene derivativesBioorg Med Chem Lett2003134437444014643341
- NakamuraEIsobeMFunctionalized fullerenes in water. The first 10 years of their chemistry, biology, and nanoscienceACC Chem Res20033680781514622027
- BosiSDa RosTSpallutoGPratoMFullerene derivatives: an attractive tool for biological applicationsEur J Med Chem20033891392314642323
- SatohMTakayanagiIPharmacological studies on fullerene C60, a novel carbon allotrope, and its derivativesJ Pharmacol Sci200610051351816682790
- LaiHSChenYChenWJFree radical scavenging activity of fullerenol on grafts after small bowel transplantation in dogsTransplant Proc20003261272127410995943
- LaiHSChenWJChiangLYFree radical scavenging activity of fullerenol on the ischemia-reperfusion intestine in dogsWorld J Surg200024445045410706918
- WangHJosephJAQuantifying cellular oxidative stress by dichlorofluorescein assay using microplate readerFree Radic Biol Med19992761261610490282
- TsaiMCChenYHChangLYPolyhydroxylated C60, Fullerenol, a novel free-radical trapper, prevented hydrogen peroxide – and cumene hydroperoxyde – elicited changes in rat hippocampus in-vitroJ Pharm Pharmacol1997494384549232545
- ChiangLYLuFJLinJWFree radical scavenging activity of water-soluble fullerenolsJ Chem Soc Chem Commun19951212831286
- JinHChenWQTanhXWPolyhydroxylated C60, fullerenols, as glutamate receptor antagonists and neuroprotective agentsJ Neurosci Res20006260060711070504
- GeldermanMPSimakovaOClogstonJDAdverse effects of fullerenes on endothelial cells: Fullerenol C60(OH)24 induced tissue factor and ICAM-1 membrane expression and apoptosis in vitroInt J Nanomedicine20083596818488416
- HuangHCLuLHChiangLYAntiprofilative effect of polyhydroxylated C60 on vascular smooth muscle cellsProc Electrochem Soc199640396100
- ZhaoQFZhuYRanTCCytotoxicity of fullerenols on Tetrahymena pyriformisNucl Sci Techniq200617280284
- SilvaGANeuroscience nanotechnology: progress, opportunities and challengesNat Rev Neurosci20067657416371951
- SilvaGANanotechnology approaches for the regeneration and neuroprotection of the central nervous systemSurg Neurol20056330130615808703
- DuganLLLovettEGQuickKLLathariusJLinTTO’MalleyKLFullerene-based antioxidants and neurodegenerative disordersParkinsonism Relat Disord2001724324611331193
- DuganLLGabrielsenJKYuSPBuckminsterfullerenol free radical scavengers reduce excitotoxic and apoptotic death of cultured cortical neuronsNeurobiol Dis199631291359173920
- InjacRRadicNGovedaricaBAcute doxorubicin pulmotoxicity in rats with malignant neoplasm is effectively treated with fullerenol C60(OH)24 through inhibition of oxidative stressPharmacol Rep20096133534219443948
- InjacRPerseMCerneMProtective effects of fullerenol C 60(OH) 24 against doxor ubicin-induced cardiotoxicity and hepatotoxicity in rats with colorectal cancerBiomaterials2009301184119619046599
- InjacRBoskovicMPerseMAcute doxorubicin nephrotoxicity in rats with malignant neoplasm can be successfully treated with fullerenol C60(OH)24 via suppression of oxidative stressPharmacol Rep200860574274919066422
- YinJJLaoFMengJInhibition of tumor growth by endohedral metallofullerenol nanoparticles optimized as reactive oxygen species scavengerMol Pharmacol2008741132114018635669
- ZhuJJiZWangJTumor-inhibitory effect and immunomodulatory activity of fullerol C60(OH)xSmall2008481168117518574800
- BogdanoviGKojicVDvordjevicAVojinovicMBaltivVVModulating activity of fullerol C60(OH)24 on doxorubicin-induced cytotoxicityToxicol in Vitro20041862963715251181
- BingsheXXuguangLXiaoqinYJinliQWeijunJStudies on the interaction of water-soluble fullerols with BSA and effects of metallic ionsMat Res Soc Symp Proc2001675W7.4.1W7.4.5.
- ZhaoGCZhangPWeiXWYangZSDetermination of proteins with fullerol by a resonance light scattering techniqueAnal Biochem200433429730215494137
- NafisiSSabouryAAKeramatNNeaultJ-FTajmir-RiahiHAStability and structural features of DNA intercalation with ethidium bromide, acridine orange and methyleneblueJ Molec Struct20078273543
- HellerDPGreenstockCLFluorescence lifetime analysis of DNA intercalated ethidium bromide and quenching by free dyeBiophys Chem1994503053128011950
- Le PecqJBPaolettiCA new fluorometric method for RNA and DNA determinationAnal Biochem1966171001076008008
- NishiokaTYuanJMatsumotaKFluorescent lanthanide labels with time-resolved fluorometry in DNA analysisFerrariMMicro/Nano Technology for Genomic and ProteomicsNew York, NYSpringer-Verlag2007
- KubátPLangKZelingerZKrálVFormation of lanthanide(III) texaphyrin complexes with DNA controlled by the size of the central metal cationJ Inorg Biochem2005991670167515993945
- SelvinPRPrinciples and biophysical applications of lanthanide-based probesAnnu Rev Biophys Biomol Struct20023127530211988471
- LakowiczJRPrinciples of Fluorescence SpectroscopyNew York, NYSpringer-Verlag2006681697
- GaoFChaoHZhouFYuanYXPengBJinLNDNA interactions of a functionalized ruthenium(II) mixed-polypyridyl complex [Ru(bpy)2ppd]2+J Inorg Biochem20061001487149416766033
- SongGLiLLiuLFluorometric determination of DNA using a new ruthenium complex Ru(bpy)2PIP(V) as a nucleic acid probeAnal Chem200218757759
- ZipperHBrunnerHBernhagenJVitzhumFInvestigations of DNA interaction and surface binding by SYBR Green I, its structure determination and methodological implicationsNucleic Acids Res200432e10315249599
- RengarajanKCristolSMMehtaMNickersonJMQuantifying DNA concentration using fluorometry: a comparison of phluorophoresMol Vis2002841642112432341
- VitzhumGGeigerHBisswangerHBrunneJBernhagenJA quantitative fluorescence based microplate assay for the determination of double-straned DNA using SYBR Green I a standard ultravioled transluminator gel imaging systemAnal Biochem1999276596410585744
- CuiDAdvances and prospects on biomolecular functionalized carbon nanotubesJ Nanosci Nanotechnol2007171298131417450892
- WeiWSethuramanAJinACMonteiro-RiviereNANarayanRJBiological properties of carbon nanotubesJ Nanosci Nanotechnol200771284129717450891
- StarATuENiemannJGabrielJJoinerCSValckeCLabel-free detection of DNA hybridization using carbon nanotube network field-effect transitionsProc Nat Acad Sci U S A2006103921926
- HobbieEKBauerBJStephensJBeckerMLMcGuigganPHudsonSDColloidal particles coated and stabilized by DNA-wrapped carbon nanotubesLangmuir200521102841028716262277
- ChiangLYUpasaniRBSwirczewskiSSEvidence of hemiketals incorporated in the structure of fullerols derived from aqueous acid chemistryJ Am Chem Soc199311554535457
- SchneiderNSDarwishADKrotoHWTaylorRWaltonDRMJ Chem Soc Chem Commun19944463464
- ChiangLYUpasaniRBSwirczewskiSSVersatil nitronium chemistry for C60 fullerene functionalizationJ Am Chem Soc19921141015410157
- AlvesGCLadeiraLORighiASynthesis of C60(OH)18–20 in aqueous alkaline solution under O2-atmosphereJ Braz Chem Soc20061711861190
- HuseboLOSitharamanBFurukawaKKatoTJWilsonLJFullerenols revisited as stable radical anionsJ Am Chem Soc2004126120551206415382940
- DjordjevicAVojinovic-MiloradivMPetranovicNDevecerskiABogdanovicGJAdamovJSynthesis and characterization of water-soluble biologically active C60(OH)24Arch Oncol19975139145
- TroshinPAAstakovaASLyubovskayaRNSynthesis of fullerenols from halofullerenesFullerenes Nanotubes Carbon Nanostruct200513113
- De VenteJBruynPJZaagsmaJFluorescence spectroscopic analysis of the hydrogen bonding properties of catecholamines, resorcinolamines, and related compounds with phosphate and other anionic species in aqueous solutionJ Pharm Pharmacol1981332902966116776