Abstract
Cellular internalization and trans-barrier transport of nanoparticles can be manipulated on the basis of the physicochemical and mechanical characteristics of nanoparticles. Research has shown that these factors significantly influence the uptake of nanoparticles. Dictating these characteristics allows for the control of the rate and extent of cellular uptake, as well as delivering the drug-loaded nanosystem intra-cellularly, which is imperative for drugs that require a specific cellular level to exert their effects. Additionally, physicochemical characteristics of the nanoparticles should be optimal for the nanosystem to bypass the natural restricting phenomena of the body and act therapeutically at the targeted site. The factors at the focal point of emerging smart nanomedicines include nanoparticle size, surface charge, shape, hydrophobicity, surface chemistry, and even protein and ligand conjugates. Hence, this review discusses the mechanism of internalization of nanoparticles and ideal nanoparticle characteristics that allow them to evade the biological barriers in order to achieve optimal cellular uptake in different organ systems. Identifying these parameters assists with the progression of nanomedicine as an outstanding vector of pharmaceuticals.
Introduction
The emergence of nanomedicine provides a strategic, therapeutic tool that aims to increase drug targeting to site-specific areas within the body. Nanoparticle (NP) research has identified the crossing of mucosal barriers and cellular uptake to support NP utilization, as well as NP surface properties that affect these phenomena.Citation1 In the design of NPs for biological use, significant factors to overcome limitations associated with insufficient drug delivery to targeted sites include NP size, surface charge, shape, chemical composition, and stability.Citation2,Citation3 Manipulating these pertinent NP characteristics may facilitate various applications and enhanced cellular and trans-barrier internalization of NPs into the target sites. These sites innately have a biological barrier to prevent the entry of foreign objects, thus resulting in decreased drug concentrations at the intended site. Ideally, nanomedicine should circumvent the biological barriers and enhance drug targeting and NP uptake.Citation4
illustrates different transport mechanisms across and into the biological membrane for the internalization of NPs; key terms related to NP internalization and trans-barriers are provided in . According to Kumari et alCitation5 NP internalization occurs mainly through intracellular, paracellular, and transcellular pathways. However, endocytosis pathways are poorly understood regardless of their clinical significance and continued research.Citation3 Continued research in this paradigm, coupled with nanoparticulate internalization and characterization, will provide immense insight into an ideal pharmaceutical formulation design.
Table 1 Key terms
Figure 1 The transport mechanisms of a typical biological barrier.
Notes: (A) Cellular internalization of nanoparticle into cell via endocytosis; (B) transcellular transport of nanoparticles through cell; (C) paracellular transport of nanoparticle between cells through the tight junction; and (D) receptor-mediated transcytosis.
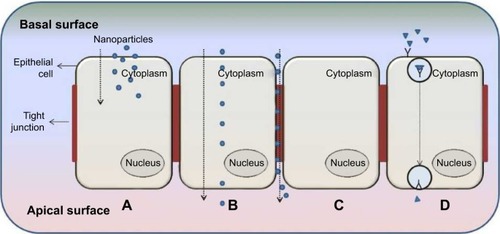
Current studies on nanomedicine are encouraged in order to structure a framework that enables efficient, safer drug delivery and to eliminate many of the disadvantages posed by conventionally delivered drugs. Studies to specifically determine the effect of NP internalization are limited yet necessary in order to enhance biomedical technology and inform toxicity studies. Elucidating the parameters of NPs that enable them to target cells in response to disease-specific signals could significantly improve the therapeutic care of complex diseases. The current review therefore discusses NP properties and characteristics such as size, shape, charge, hydrophobicity, and ligand attachments that influence their uptake into target cells and through biological barriers. Intracellular pathways and current mechanisms employed to augment NP uptake and biological barrier transport were also discussed in detail.
Transport mechanisms of nanocarriers
Intracellular endocytic delivery pathways
Various receptor-mediated pathways exist for cellular internalization of biological substances such as hormones and enzymes that require internalization to exert an effect at a cellular level (). By adopting these mechanisms, drugs and NPs can be delivered to the necessary cell type. Cellular uptake mechanisms need to be understood in order to enhance internalization and identify NP characteristics that promote specific mechanisms.Citation1 The mechanisms of different endocytic pathways as illustrated in are thoroughly described in the subsequent discussions.
Pinocytosis
Included in the pinocytosis classification are clathrin- and caveolae-mediated endocytosis and macropinocytosis. Clathrin-mediated endocytosis involves clathrin-coated vesicle formation in the presence of adaptor and accessory proteins. Endocytic event cascade is activated by the signaling of the NP on the cell surface,Citation6 which aligns surface proteins to prompt clathrin recruitment from the cytosol to begin clathrin-coating on the inner membrane of the cell. An adaptor protein, Epsin, is involved in the initial stages of membrane curvature and pit formation and accessory proteins such as dynamin (GTPase) affect vesicle formation from shallow to deep invagination by inducing deformation of the membrane.Citation7 With the aid of dynamin, a clathrin-coated vesicle with a size of 100–150 nm is formed due to polymerization of the coat complex and the NP-containing clathrin-coated vesicle then internally detaches from the donor membrane.Citation6 Once within the cell, clathrin and adaptor proteins uncoat to allow fusing of the vesicle within the cell to release the endocytosed NPs ().
Caveolae-mediated endocytosis is a pathway dependent on membrane cholesterol, dynamin, and cell receptor mediation.Citation8 Caveolae are formed by a cluster of caveolin proteins (caveolin-1, -2, -3) that bind directly to membrane cholesterol,Citation9 as shown in . Complex signaling is driven by the cell membrane–bound NPs to be endocytosed, which induces actin reorganization and dynamin recruitment from the cytosolCitation10 to stimulate membrane invagination and further on, vesicle budding. The uncoated invagination initially assumes a flask shape with a body diameter of 60–80 nm and a neck diameter of 10–15 nm.Citation11 Similar to clathrin-mediated endocytosis, dynamin regulates vesicle budding of the caveolae to internalize cell membrane segments that contain the cargo and the caveolae membrane fuses into the acceptor compartment to release its contents.
Macropinocytosis
Macropinocytosis is a clathrin-, caveolin-, and dynamin-independent process that involves uptake of a larger volume of the membrane, also allowing for larger sized particles of sizes .1 μm to be internalized.Citation12 The pathway proceeds by forming protrusions due to actin polymerization from the cell membrane, which then encapsulates the substance to be internalized and once again fuses back with the cell membrane.Citation9 The process is initiated by activation of tyrosine kinases that signal a cascade of changes in the actin cytoskeleton and the formation of membrane protrusions. The random macropinocytic extensions enclose the material in the extracellular environment for cellular uptake () and collapse to fuse with the cell membrane, generating an internalized macrosome vesicle of an average of ~10 μm.Citation12,Citation13 The uncoated membrane of the macrosome either acidifies and shrinks or fuses with the lysosomal compartment, consequently releasing the NPs into the intracellular compartment.Citation14
Phagocytosis
Similar to macropinocytosis, phagocytosis is also a clathrin-, caveolin-, and dynamin-independent process and is proficient in internalizing larger particles such as drug nanocarriers and pathogens.Citation14 It involves sequential instigation of receptors as a result of cell surface recognition of cargo, leading to internalizations by encircling it into triggered cup-shaped cell membrane deformations, forming a phagosome.Citation9 The pathway begins with opsonization of the NPs, which then attach to the cell surface via Fc receptors and complement receptors,Citation15 marking the beginning of actin polymerization and rearrangement and membrane extension to form surface extensions that encapsulate the opsonized NPs for internalization ().Citation14,Citation16 A reduction and contraction of actin at the base of the cup allows for closure of the phagosome and the entire actin-lined phagosome is then internalized with its contents.Citation15 In a macropinocytotic manner, the vesicle undergoes degradation after transporting the opsonized particle into the cell.
Transcellular delivery pathway
As described by clathrin-dependent and clathrin-independent internalization, postuptake, the vesicle undergoes degradation. However, through other pathways, the vesicle can be transported to the other end of the cell surface, where its contents can be expelled into the extracellular environment as shown in .Citation17 This pathway allows for lipophilic NPs and molecules to move efficiently through the transcellular route by partitioning into and out of lipid bilayers via vesicular carriers due to its lipidic nature.Citation18 The cargo to be transported binds to cell membrane receptors to form a complex. Membrane invagination is then initiated and the deep pit is internalized as a vesicle containing NPs.Citation19,Citation20 Following internalization, the endocytosed vesicle is sorted into a transcytotic vesicle to prevent typical endosome degradation. The transcytotic vesicle is then transported to the other end of the cell, where the vesicle membrane fuses with the cell membrane and the content of the vesicle is secreted externally.Citation19,Citation21
Paracellular delivery pathway
Paracellular delivery of drugs is a passive process that occurs between adjacent cells via tight junctions (TJs) through the intercellular space and not through the cell as described by transcellular delivery ().Citation22 This route is specific for hydrophilic NPs and molecules, since hydrophilic molecules cannot cross biological membranes and as aqueous pathways that normally absorb nutrients, vitamins, or cofactors.Citation18 Hydrophilic molecules are unable to cross biological membranes in the manner of lipophilic molecules due to the lipid properties of the cell bilayer membrane; therefore, targeting the paracellular pathway can significantly affect their transepithelial transport. Transit through this route is regulated by TJs that have heterogeneous pores with a variety of diameters of up to 15 Å;Citation22 the overall charge of the TJ is negative and is therefore more responsive to positively charged NPs for paracellular permeation.Citation23
Parameters and characteristics of nanostructures governing cellular internalization
The need for understanding the fundamental physicochemical characteristics of NPs and their pivotal role in cellular uptake is essential when designing smart nanosystems (Graphical Abstract). Ascertaining NP functionality provides basic and necessary information to influence the rational design of optimal nanocarriers by selectively delivering drug to targeted tissue to increase the rate of cellular uptake and effectively reduce drug-induced side effects.Citation24–Citation26 Other determining factors relating to cellular uptake are: 1) variation in the uptake pathways,Citation27 2) cell specificity,Citation28 3) NP interaction with cell surface receptors,Citation25 and 4) NP interaction with plasma proteins leading to NP–protein complex formation.Citation29
Particle size
An important consideration regarding particle size is the 4 μm diameter of the smallest blood vessels, as a micrometer-sized particle may cause an embolism. Acknowledging size should therefore not be limited to its internalization ability but also for its physical effects once inside the body.Citation30,Citation31 Cells within the human body vary from 1–100 μm, and therefore, have a low probability of internalizing particles of ~100 μm. This particle size range for intracellular delivery is limited to the cells with a large enough capacity to host the particle. In addition to the higher rates of endocytosis of smaller sized NPs (<100 nm),Citation12,Citation32,Citation33 experimental data also promote higher bioavailability of the endocytosed drug carrier at 100–1,000 nm.Citation34,Citation35 Various studies revealed that within a 1–100 nm range, 50 nm NPs show maximum cellular uptake, with 14–20 nm NPs having a higher endocytotic rate than the 100 nm NPs.Citation36–Citation38 No significant difference in cellular uptake has been shown by NPs between 25–130 nm,Citation39 while some reports postulate that NPs have higher internalization between 50–100 nm.Citation40 On the contrary, from a cellular uptake research experiment using thio-organosilica NPs of 50–500 nm, Awaad et alCitation38 concluded that 95–200 nm is the ideal size for increased cellular uptake.
In an in vitro study undertaken by Nicolete et alCitation41 after 4 hours of being culture incubated, 6.5±3.9 μm poly(lactic-co-glycolic acid) (PLGA) microparticles were still attached to the cell surface and required more time for endocytosis to occur. Simultaneously, PLGA NPs of size 389 nm (polydispersity index =0.2) had already, within the same time period, been encapsulated in vesicles and endocytosed into the intracellular compartment (). These results were consistent with the study conducted by Loh et alCitation42 which demonstrated poor internalization capability of chitosan particles that were >1 μm, much unlike the extensive uptake of NPs of sizes 110–390 nm. Interestingly, Sahay et alCitation12 stated that microparticles also enter cells but not as rapidly as NPs and concluded that poly(ethylene glycol) (PEG) particles <5 μm can gain entry into cells via pinocytosis. Likewise, considering the possibility of the aggregation of NPs, the size of an aggregated nanocluster is larger than a single NP and will affect the internalization rate accordingly. In vitro, the stability of the NPs should be controlled to promote a consistent nanosystem.
Figure 5 Representation of the internalization potential dependent on particle size.
Note: The larger surface area of the nanoparticle allows for increased surface contact with the cell membrane for higher internalization rates as described in the investigation of Nicolete et al.Citation41
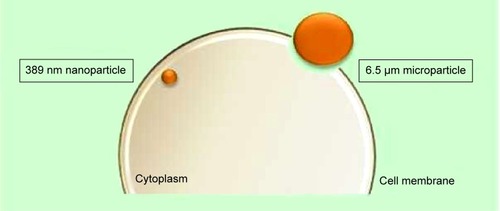
A research review compiled by AcostaCitation34 predominantly concluded that NPs with a size of ≤500 nm produce higher cellular uptake than NPs of a larger size. These results were also coherent with the enhanced penetration abilities of specialized nanocarrier systems that housed the NPs. The uptake of NPs with a size of 500 nm has a lower viability but may be promoted with the use of a constructive, complementary delivery system. Essentially, an assisting delivery system would have lipophilic properties or a suitable surface charge for enhanced internalization.
Despite several studies promoting the use of smaller NPs (<100 nm), disparities exist in other studies providing evidence that particle size affects internalization as significantly as its complementary system. The variation in internalization profiles and NP size demonstrates that the influence of NP size is also dependent on the type of cells and the chemical composition of the nanomaterial.Citation12,Citation42
Surface charge
Charge parameters are vital in the characterization of NPs, as they determine aggregation in the blood or interaction with oppositely- and like-charged cell membrane surfaces. A plethora of studies concluded that cationic and neutral particles show the highest transport efficiency compared to negatively charged particles due to the charge attraction between the positive NPs and negative cell membrane surface, thereby increasing the rate and extent of internalization.Citation33,Citation43–Citation45 These electrostatic forces are long-range forces and can act across intervening aqueous space.Citation46
Cationic and neutral nanoparticles
In the study by Jallouli et alCitation47 the uptake of 60 nm neutral and cationic maltodextrin porous NPs with a phospholipid core was investigated in brain capillary endothelial cells. Neutral NPs were endocytosed in the caveolae-mediated pathway, while the cationic NPs were found to utilize the paracellular pathway due to the rich anionic sites found on the luminal surface of the endothelial cells. The cationic NPs had a stronger charge affinity to the collagen fibers on the surface of the endothelial cells, thus impeding drug delivery, while the neutral NP sample showed better transcytosis into the targeted cells.
Cationic nanoparticles
Karlsson et alCitation48 showed that cationic particles had a 2.5-fold higher uptake than neutral particles and a 25-fold higher uptake than anionic particles, concluding that cationic particles are transported using the paracellular pathway to a greater degree than neutral or anionic particles. These results contradict the study by Lin et alCitation49 who concluded from their experimental study that cationic and neutral gold-coated NPs were internalized via endocytosis while anionic NPs showed lesser permeability and were trafficked through the paracellular pathway.
Anionic nanoparticles
Contrary to the abovementioned research, many studies have shown the successful internalization of negatively charged NPs. An interesting study conducted by Harush-Frenkel et alCitation43 concluded that both anionic and cationic PEG-D-L-polylactide (PLA) NPs accumulated within MDCK and HeLa cells. The internalized anionic NPs underwent a degradative lysosomal process and were unable to undergo further transcytosis, rendering cationic NPs more suitable for cellular drug delivery. Likewise, negatively charged quantum dot NPs were, in fact, endocytosed through a caveolae-mediated pathway, while cationic quantum dot NPs used a clathrin-mediated pathway, internalizing HEK cells.Citation50 Both charged quantum dots were internalized via endocytosis with no specific rationalization to endocytic preference. Despite the hypothesis of NPs with a negative charge having a slower uptake rate due to repulsive forces, research has shown that certain anionic NPs internalize more readily. From this discussion, it can be deduced that anionic NPs have the ability to undergo internalization via caveolae pathways, whereas cationic NPs commonly use the clathrin pathways.Citation43,Citation50 Highly charged negative NPs also favor good stability since the Coulombic repulsion forces arising from their surface charge can overcome the Van der Waals attractive forces between them and prevent aggregation.Citation51
Particle shape
Functional behavior and internalization of particles in drug delivery are strongly influenced by their shape.Citation52 Although few researchers have focused on shape, those who have, have made noteworthy contributions, but with contradicting results.Citation53 In many of the following studies, NP shape was the key factor for enhanced internalization, proving its pivotal role in NP fabrication. Chithrani et alCitation36 investigated the uptake of gold spherical and rod-shaped NPs. The 74 nm and 14 nm spherical NPs had a higher uptake when compared to 74×14 nm rod-shaped NPs by 500% and 375%, respectively, which support their claim that spherical particles have a higher internalization probability. The speculation is that the difference in curvature between the two shapes determines cell surface binding. When the longitudinal axis of the rod-shaped NP is in contact with the cell surface, it has a larger area of contact with the cell membrane receptors compared to spherical NPs, and therefore blocks the remaining available membrane receptors, reducing the number of NPs being internalized. Han et alCitation54 have reported similar results that prove that spherical particles internalize substantially quicker than asymmetrically shaped particles. Champion and MitragotriCitation55 reported on the correlation between contact angle and particle internalization, concluding that rod-shaped NPs have a higher likelihood of internalization when their major axis is perpendicular to the cell membrane. The long axis of the rod aligned perpendicular to the cell will increase the internalization rate and the rate will decrease as ø increases (). This theory is based on the orientation of the NP to the cell membrane and could further dictate the synthesis of NP shapes with several short aspects to enhance internalization.
Figure 6 Particle internalization based on orientation to the membrane.
Notes: (A) Schematic showing the angle between the long axis of the particle and the bilayer normal; (B) minimum driving forces required to guide the ellipsoid with different initial orientations of the long axis through the lipid bilayer; (C) time evolution of particle orientations during the ellipsoid penetration processes with different initial orientations. Reprinted by permission from Macmillan Publishers Ltd: Nature Nanotechnology. Yang K, Ma YQ. Computer simulation of the translocation of nanoparticles with different shapes across a lipid bilayer. Nat Nanotechnol. 2010;5(8):579–583. Copyright © 2010.Citation56
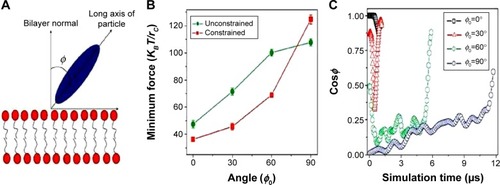
Another theory was reported by Gratton et alCitation57 through a study using various shaped PEG-based PRINT (particle replication in non-wetting templates) particles. Among different shapes investigated, nano-cylinders were internalized to a considerably larger extent than micro-cylinders and nano-cubes. The higher cell uptake was speculated to be due to the larger surface area, allowing for more multivalent ionic interactions with the cell membrane, which then undergo endocytosis and phagocytosis.Citation57 This theory was supported by Sadeghi et alCitation58 who based the highest antibacterial activity of silver nano-plate NPs compared to nano-spheres and nano-rods on the larger surface area that binds with the bacterial cells, as well as by Hao et alCitation53 who proved that mesoporous silica long-rod NPs had higher internalization and retention than spheres and short rods. In addition, various nano-shaped particles have also been shown to have diverse accumulation capabilities in different organ systems. Decuzzi et alCitation59 identified the shape effect of silicon NPs and their accumulation in specific tissues, which they evaluated from their biodistribution data, that in the lung discoidal-shaped NPs tended to internalize more than spherical, cylindrical, and quasi-hemispherical NPs. In the liver, cylindrical NPs accumulated more than the other three shapes, discoidal-shaped NPs accumulated most in the heart, and discoidal and quasi-hemispherical NPs had the highest internalization in the spleen tissue. These results were further corroborated by Park et al,Citation60 who demonstrated that nano-worms had a higher tumor uptake in a fibrosarcoma and breast cancer cell line compared with spherical NPs, and Devarajan et alCitation61 who showed preferential accumulation of irregular spherical NPs in the spleen, while regular spherical NPs accumulated in the liver.
Contrary to the abovementioned observations that promote nonspherical NPs as possessing higher internalization, we cannot deduce that spherical NPs are less conducive for internalization, as several studies attest to their dynamic characteristics and unmatched high surface area to volume ratio. In fact, if considering therapeutic potential of NPs, then spheres should be superior to nonspherical NPs due to their excellent drug-loading capacity. Many researchers claim hypothetical theories on the internalization kinetics based on NP shape; however, we cannot deny that all of these NPs investigated do not have constant additional NP parameters, and these would also impact on the rate of cellular uptake.
Surface properties
The surface properties of NPs are as fundamental as the other key characteristics that dictate internalization. For targeted drug delivery, high circulation time of NPs in the body is required for the NPs to recognize their specific site of interest. Opsonins adsorbing to the surface of hydrophobic NPs decrease circulation time by initiating the immune response cascade which allows phagocytosis of the NPs following recognition as foreign objects. If the drug is unnecessarily taken up by the reticuloendothelial system, drug bioavailability is reduced and undesirable effects are exerted on the immune system and pose the threat of toxicity within the host.Citation62,Citation63 NP hydrophobicity may instigate redundant interaction with plasma proteins, phagocytic internalization, immune cell stimulation and particle clearance.Citation64 Minimizing the recognition of NPs by the reticuloendothelial system and subsequent immune system will enhance the probability of uptake by the target cells. Hence, recent research is focused on modifying conventional hydrophobic NP surfaces with a hydrophilic protective layer. This layer creates a cloud of chains at the NP surface to cause steric repulsive forces against plasma proteins and increase the blood circulation half-life of targeted nanocarriers as shown in .Citation24,Citation65–Citation67 Hydrophilic-coated NPs can be fabricated by making use of polymer types such as PEG, PEG-based copolymers and poly-vinyl pyyrolidone (PVP).Citation65,Citation68
Figure 7 (1) Pathway of uncoated hydrophobic nanoparticle; (2) pathway of coated hydrophilic nanoparticle.
Notes: (1) (A) Nanoparticle in blood circulation; (B) opsonins recognize nanoparticle as a foreign body due to the hydrophobic surface; (C) opsonization of nanoparticle; (D) and (E) phagocytosis by phagocyte and elimination of nanoparticle. (2) (A) Hydrophilic polymer-coated nanoparticle in blood circulation; (B) steric hindrance maintains repulsive forces between opsonins and nanoparticle; (C) nanoparticle continues to circulate until target site reached; (D) and (E) endocytosis by target cell.
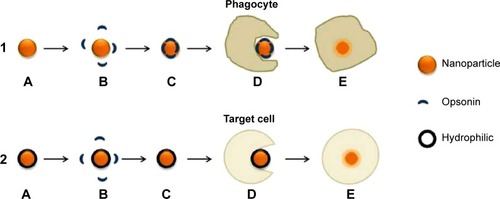
Physicochemical surface parameters can affect cellular uptake as reported in the recent study conducted by Loh et al.Citation42 The study ascertained the theory of concentration-dependent chitosan molecules increasing NP transport through membranes by disrupting the integrity of intercellular TJs involved in paracellular transport.Citation69 Chitosan redistributes cytoskeleton proteins such as actin and tubulin found in the apical membrane resulting in the opening of TJs.Citation70 Using chitosan-coating as a surface modification for NPs could optimize paracellular transport and deliver a higher amount of drug-loaded nanocarriers to the targeted site.
An interesting recent advance in research shows the coating of a polymeric NP core with red blood cell (RBC) membranes. Luk et alCitation71 synthesized a cloaked NP utilizing the RBC membranes to evade the immune response cascade resulting in a prolonged in vivo circulation time. This bio-inspired nano-system enhances the probability of NP uptake and if coupled with other NP factors to promote increased cellular uptake may prove to be yet another breakthrough drug delivery system. Therefore, modulating surface characteristics can control internalization rate, extent and even transport pathways. However, in order to achieve the desired outcome, the selection as well as manipulation of appropriate biomaterials for coating is imperative.
Proteins and ligand attachments
Cell-penetrating proteins or peptides (CPPs) can increase cellular uptake of surface-modified drug-loaded NPs by employing direct cell penetration or receptor-mediated endocytic pathways and localizing NPs at the required site.Citation72,Citation73 CPPs are small amphipathic or cationic polypeptides (10–30 amino acids long) inclusive of trans-activating (TAT) peptide, penetratin, transportan, toxins, poly-arginine, and rabies virus glycoprotein (RVG).Citation74,Citation75 A possible mechanism of the TAT protein is that it binds to cell surface heparin sulfate proteoglycans and is then internalized through receptor-mediated uptake ().
Figure 8 Stimulating endocytosis through CPP and antibody conjugation of nanoparticles.
Abbreviation: CPP, cell-penetrating protein or peptide.
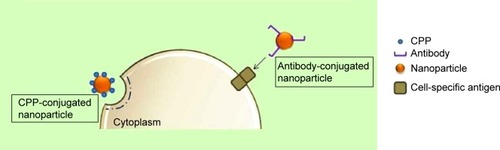
Bareford and SwaanCitation76 reviewed cell adhesion molecules (CAMs), which form part of the subfamily of immunoglobulins. CAMs bind to cell adhesion receptors (CARs) on cell surfaces and stimulate clathrin-mediated uptake. Peptides such as Arg-Gly-Asp (RGD) bind to CARs and have been used extensively to promote the uptake of drug-loaded NPs. Furthermore, cationic proteins have a higher affinity to negatively charged cell membranes, which further promotes cellular internalization.Citation33,Citation76 Ligands for enhanced cellular uptake include folic acid, albumin, and cholesterol, which are internalized using caveolae-mediated uptake, and are common, attractive methods to stimulate spontaneous uptake through receptors.Citation76 In contrast, Pujals and GiraltCitation77 reviewed the highly improved internalization efficiency of fatty acids or silaproline, which is a hydrophobic derivative of proline-rich, amphipathic CPPs. Targeting tissue-specific receptors and molecules by using antibodies has also proven to be useful for enabling internalization of drug-carriers intended for site-specific delivery. This strategy requires the conjugation of receptor-specific ligands and proteins to the NP coat. The potential for binding to the target tissue is dependent on the abundance of the ligand and its specificity and affinity for binding to the target cell membrane.Citation3
By targeting the monoclonal antibody to the protein aminopeptidase P (APP) in the lung, in vivo transport across the endothelial barrier to lung tissue occurs within seconds.Citation78 NPs that are surface modified with cyclic RGD peptides preferably bind to αvβ3 integrin receptors. In comparison to unmodified NPs, these peptide functionalized NPs internalize more readily into HeLa cells.Citation79 This concept can be applied to tumor cells that contain specific membrane antigens where the surface of the drug carrier is modified with the corresponding antibody for increased binding and uptake. This is experimentally shown by targeting prostate-specific membrane antigen,Citation80 transferrin-conjugated NPs that demonstrated higher cellular uptake in a prostate cancer cell line,Citation81 and monoclonal antibody–conjugated PLGA NPs for increased tumor cell uptake.Citation82 In contrast, quantitative and biodistributive data from the study conducted by Huang et alCitation83 showed that three different ligands (single-chain variable fragment, amino terminal fragment, and cyclic RGD peptide) only marginally improved gold NP accumulation in tumor tissue in comparison with nontargeted controls.Citation83 Similarly, Temsamani and VidalCitation84 reviewed the construct of phosphopeptides linked with penetratin as having inhibitory effects on ligand-dependent transduction pathways in various cell lines, even though individually, these CPPs promote internalization. The use of serum protein attachments on gold NPs was shown to improve their uptake half-life, rate, and extent.Citation36 α- and β-globulin proteins are known to be internalized by cells and increasing the diversity of protein attachments may allow entrance into cells via the receptor-mediated pathways. However, another study reported that serum protein attachments inhibited the uptake of polyvalent gold NPs, and that instead, uptake is dependent on scavenger receptors.Citation85
Taking note of the use of a cationic mixed monolayer of CPPs and PEG, Liu et alCitation86 proved its efficiency on internalization rates of gold NPs by combining these factors. The multifunctional NPs show superior uptake when compared to NPs synthesized exclusive of the addition of CPP or having an anionic surface charge. Thus, drug delivery scientists have capitalized on the knowledge of receptors and ligands as targeting moieties for specific cellular organelle targeting.Citation76,Citation87 This principle is not only applied to general cells but can also be adopted for specific targeting to tumors for increasing the bioavailability of drugs to the target site.
Current advances in trans-barrier internalization
It has been discussed how the inherent physical and chemical properties of NPs such as size, shape, surface charge, solubility, surface characteristics, and ligand complexes can dictate the degree of biocompatibility and internalization kinetics for NPs, as well as the selectivity of these factors for specific cell types. The additional key parameter for consideration in the uptake of NPs is the environment the nanosystem comes into contact withCitation29 and the manner in which we can engineer these parameters to trigger different biological responses.Citation69 As discussed by Brannon-Peppas and Blanchette,Citation88 the size of NPs for crossing biological barriers is dependent on the tissue, target site, and circulation. Likewise, all other NP characteristics need to be fabricated in consideration of inherent target tissue requirements for cellular internalization. Barrier capacity and trans-compartment transport of particles vary considerably between different tissue types.Citation89 Limited transport across the epithelia is one of the prime obstacles for therapeutic agents and nanomedicines reaching the adequate biological compartment,Citation1 as barrier systems are unable to evaluate and differentiate drug delivery systems for translocation from foreign particles, and therefore restrict the entry of the drug carriers, rendering the system invaluable and reducing its efficacy.
The delivery of therapeutic agents requires successful negotiation of these barriers in order to attain a sufficient therapeutic index.Citation90 To transverse these barriers using smart nanosystems, the development of efficient nanomedicines requires a thorough understanding of the characteristics of the body’s systems, biological barriers, and mechanisms to evade foreign particle interactions in the body. Once the characteristics of the biological systems are defined, we can identify NP parameters that enhance transmembrane transport or cellular uptake (). From the data reported in , it is evident that various nanosystems contributing different parameters and characteristics are able to transverse biological barriers dictated by the barrier’s set of limitations and specific NP criteria for internalization.
Table 2 Overview of biological barriers and nanoparticle advances to overcome physiological limitations
Conclusion
The scope for further research into this concept has immense potential to progress medical care by decreasing side effect profiles due to specific and targeted drug release, improving bioavailability, and bypassing first-pass metabolism. The use of sophisticated nanomedicines as effective vectors of drugs exerts beneficial effects at the molecular level, providing targeted drug delivery. The forefront of nanomedicine research comprises diagnosis, treatment, monitoring, and management of biological conditions. Independently, nanotechnology has surfaced as one of the most successful drug delivery concepts to date, and it is obvious that applications for biomedical nanotechnology are broad. This review encompasses possible techniques of NP manipulation to further improve intracellular delivery of drugs. The benefits of enhanced cellular internalization have been extensively motivated and the scope for further research in this domain is constant. Therapeutic advantages can be demonstrated by increasing NP cellular uptake, targeting site-specific organ systems by increasing selectivity, and even altering the drug release kinetics of the nanosystem and biodistribution. Taking this concept into consideration also allows for a single NP of ideal characteristics to encapsulate several drugs for delivery to enhance efficacy and possibly reduce resistance. Furthermore, the diversity of NP parameters with regards to targeting ligands can be studied thoroughly to assist with the internalization of macromolecule drugs that primarily have difficulty penetrating the cell membrane.
Apart from cellular internalization, focus has been placed on nuclear targeting via a nuclear localization signal (NLS).Citation143–Citation145 At a complex level, drug delivery targeting intracellular organelles postcellular internalization may prove to be a research area of high interest. Included in this paradigm is the possibility of the multistage drug targeting of NPs from bypassing the cellular membrane to the organelle level of therapeutics. By combining many physicochemical and mechanical NP parameters specific to certain cell types or organ systems, an optimal level of cellular uptake can be achieved. Research has specified several NP characteristics that are pertinent for efficient internalization; however, it is immensely limited on more detailed trans-barrier uptake kinetics that would crucially improve NP efficacy and intracellular targeting while maintaining stability and nontoxicity. Nano drug carriers can be greatly exploited provided ex vivo and in vivo research is extensively conducted.
Pertinent to the abovementioned NP factors, we need to understand that by optimizing NP parameters and characteristics that support internalization theories and studies for enhanced intracellular delivery, these designed systems may not promote optimal drug entrapment or have adequate drug release. The issue of drug delivery design can be addressed but other in vivo requirements such as adequate clearance from systemic circulation, release of drugs from nontargeted sites, drug release from the nanosystem, and elimination of the nanocarrier from the body need to be adhered to. As the knowledge of physicochemical and physiological in vivo processes improves, nanomedicine can be further specialized to attain the absolute effect intended.
Disclosure
The authors report no conflicts of interest in this work.
References
- AlonsoMJNanomedicines for overcoming biological barriersBiomed Pharmacother200458316817215082339
- GrattonSENapierMERoppPATianSDeSimoneJMMicrofabricated particles for engineered drug therapies: elucidation into the mechanisms of cellular internalization of PRINT particlesPharm Res200825122845285218592353
- ParveenSMisraRSahooSKNanoparticles: a boon to drug delivery, therapeutics, diagnostics and imagingNanomedicine20128214716621703993
- BrzoskaMLangerKCoesterCLoitschSWagnerTOMallinckrodtCIncorporation of biodegradable nanoparticles into human airway epithelium cells-in vitro study of the suitability as a vehicle for drug or gene delivery in pulmonary diseasesBiochem Biophys Res Commun2004318256257015120637
- KumariAYadavSKYadavSCBiodegradable polymeric nanoparticles based drug delivery systemsColloids Surf B Biointerfaces201075111819782542
- XiangSTongHShiQUptake mechanisms of non-viral gene deliveryJ Control Release2012158337137821982904
- MousaviSMalerdLBergTKjekenRClathrin-dependent endocytosisBiochem J200437711614505490
- XuSOlenyukBZOkamotoCTHamm-AlvarezSFTargeting receptor-mediated endocytotic pathways with nanoparticles: rationale and advancesAdv Drug Deliv Rev201265112113823026636
- KumariSMgSMayorSEndocytosis unplugged: multiple ways to enter the cellCell Res201020325627520125123
- PelkmansLPüntenerDHeleniusALocal actin polymerization and dynamin recruitment in SV40-induced internalization of caveolaeScience2002296556753553911964480
- WangZTiruppathiCMinshallRDMalikABSize and dynamics of caveolae studied using nanoparticles in living endothelial cellsACS Nano20093124110411619919048
- SahayGAlakhovaDYKabanovAVEndocytosis of nanomedicinesJ Control Release2010145318219520226220
- KerrMCTeasdaleRDDefining macropinocytosisTraffic200910436437119192253
- HillaireauHCouvreurPNanocarriers’ entry into the cell: relevance to drug deliveryCell Mol Life Sci200966172873289619499185
- GrovesEDartACovarelliVCaronEMolecular mechanisms of phagocytic uptake in mammalian cellsCell Mol Life Sci200865131957197618322649
- ParkHCoxDCdc42 regulates Fcγ receptor-mediated phagocytosis through the activation and phosphorylation of Wiskott-Aldrich syndrome protein (WASP) and neural-WASPMol Biol Cell200920214500450819741094
- BomselMPrydzKPartonRGGruenbergJSimonsKEndocy-tosis in filter-grown Madin-Darby canine kidney cellsJ Cell Biol19891096324332582689455
- Gonzalez-MariscalLHernándezSVegaJInventions designed to enhance drug delivery across epithelial and endothelial cells through the paracellular pathwayRecent Pat Drug Deliv Formul20082214517619075905
- MostovKETransepithelial transport of immunoglobulinsAnnu Rev Immunol199412163848011293
- LuWXiongCZhangRReceptor-mediated transcytosis: a mechanism for active extravascular transport of nanoparticles in solid tumorsJ Control Release2012161395996622617522
- FerratiSMcConnellKIMackACCellular communication via nanoparticle-transporting biovesiclesNanomedicine (Lond)20149558159223731456
- HayashiMTomitaMAwazuSTranscellular and paracellular contribution to transport processes in the colorectal routeAdv Drug Deliv Rev1997282191204
- Salamat-MillerNJohnstonTPCurrent strategies used to enhance the paracellular transport of therapeutic polypeptides across the intestinal epitheliumInt J Pharm2005294120121615814245
- MohanrajVChenYNanoparticles – a reviewTrop J Pharm Res200751561573
- ReddySTBerkDAJainRKSwartzMAA sensitive in vivo model for quantifying interstitial convective transport of injected macromolecules and nanoparticlesJ Appl Physiol (1985)200610141162116916763103
- MoghimiSMHunterACAndresenTLFactors controlling nanoparticle pharmacokinetics: an integrated analysis and perspectiveAnnu Rev Pharmacol Toxicol20125248150322035254
- BaruaSRegeKCancer-cell-phenotype-dependent differential intracellular trafficking of unconjugated quantum dotsSmall20095337037619089841
- SnijderBSacherRRämöPDammEMLiberaliPPelkmansLPopulation context determines cell-to-cell variability in endocytosis and virus infectionNature2009461726352052319710653
- AggarwalPHallJBMcLelandCBDobrovolskaiaMAMcNeilSENanoparticle interaction with plasma proteins as it relates to particle biodistribution, biocompatibility and therapeutic efficacyAdv Drug Deliv Rev200961642843719376175
- GunaseelanSGunaseelanKDeshmukhMZhangXSinkoPJSurface modifications of nanocarriers for effective intracellular delivery of anti-HIV drugsAdv Drug Deliv Rev201062451853119941919
- TorranoAABlechingerJOsseforthCA fast analysis method to quantify nanoparticle uptake on a single cell levelNanomedicine (Lond)20138111815182823384698
- LuoYChenDRenLZhaoXQinJSolid lipid nanoparticles for enhancing vinpocetine’s oral bioavailabilityJ Control Release20061141535916828192
- des RieuxAFievezVGarinotMSchneiderYPréatVNanoparticles as potential oral delivery systems of proteins and vaccines: a mechanistic approachJ Control Release2006116112717050027
- AcostaEBioavailability of nanoparticles in nutrient and nutraceutical deliveryCurr Opin Colloid Interface Sci2009141315
- PatelNRDamannKLeonardiCSabliovCMSize dependency of PLGA-nanoparticle uptake and antifungal activity against Aspergillus flavusNanomedicine (Lond)2011681381139521651442
- ChithraniBDGhazaniAAChanWCDetermining the size and shape dependence of gold nanoparticle uptake into mammalian cellsNano Lett20066466266816608261
- JinHHellerDASharmaRStranoMSSize-dependent cellular uptake and expulsion of single-walled carbon nanotubes: single particle tracking and a generic uptake model for nanoparticlesACS Nano20093114915819206261
- AwaadANakamuraMIshimuraKImaging of size-dependent uptake and identification of novel pathways in mouse Peyer’s patches using fluorescent organosilica particlesNanomedicine20128562763621889475
- RogerELagarceFGarcionEBenoitJLipid nanocarriers improve paclitaxel transport throughout human intestinal epithelial cells by using vesicle-mediated transcytosisJ Control Release2009140217418119699246
- TorchilinVPRecent approaches to intracellular delivery of drugs and DNA and organelle targetingAnnu Rev Biomed Eng2006834337516834560
- NicoleteRDos SantosDFFaccioliLHThe uptake of PLGA micro or nanoparticles by macrophages provokes distinct in vitro inflammatory responseInt Immunopharmacol201111101557156321621649
- LohJWSaundersMLimLYCytotoxicity of monodispersed chitosan nanoparticles against the Caco-2 cellsToxicol Appl Pharmacol2012262327328222609640
- Harush-FrenkelORozenturEBenitaSAltschulerYSurface charge of nanoparticles determines their endocytic and transcytotic pathway in polarized MDCK cellsBiomacromolecules20089243544318189360
- NanABaiXSonSJLeeSBGhandehariHCellular uptake and cytotoxicity of silica nanotubesNano Lett2008882150215418624386
- IlinaPHyvonenZSauraMSandvigKYliperttulaMRuponenMGenetic block of endocytic pathways reveals differences in the intracellular processing of non-viral gene delivery systemsJ Control Release2012163338539523041276
- RandRPInteracting phospholipid bilayers: measured forces and induced structural changesAnnu Rev Biophys Bioeng1981102773147020577
- JallouliYPaillardAChangJSevinEBetbederDInfluence of surface charge and inner composition of porous nanoparticles to cross blood–brain barrier in vitroInt J Pharm20073441–210310917651930
- KarlssonJUngellAGråsjöJArturssonPParacellular drug transport across intestinal epithelia: influence of charge and induced water fluxEur J Pharm Sci199991475610493996
- LinICLiangMLiuTYMonteiroMJTothICellular transport pathways of polymer coated gold nanoparticlesNanomedicine20128181122024197
- ZhangLWMonteiro-RiviereNAMechanisms of quantum dot nanoparticle cellular uptakeToxicol Sci2009110113815519414515
- MuthuMSSinghSStudies on biodegradable polymeric nanoparticles of risperidone: in vitro and in vivo evaluationNanomedicine (Lond)20083330531918510426
- ChampionJAKatareYKMitragotriSMaking polymeric micro-and nanoparticles of complex shapesProc Natl Acad Sci U S A200710429119011190417620615
- HaoNLiLZhangQThe shape effect of PEGylated mesoporous silica nanoparticles on cellular uptake pathway in Hela cellsMicroporous Mesoporous Mater20121621423
- HanYAlsayedANobiliMZhangJLubenskyTCYodhAGBrownian motion of an ellipsoidScience2006314579962663017068256
- ChampionJAMitragotriSRole of target geometry in phagocytosisProc Natl Acad Sci U S A2006103134930493416549762
- YangKMaYQComputer simulation of the translocation of nanoparticles with different shapes across a lipid bilayerNat Nanotechnol20105857958320657599
- GrattonSERoppPAPohlhausPDThe effect of particle design on cellular internalization pathwaysProc Natl Acad Sci U S A200810533116131161818697944
- SadeghiBGarmaroudiFSHashemiMComparison of the anti-bacterial activity on the nanosilver shapes: nanoparticles, nanorods and nanoplatesAdv Powder Technol20122312226
- DecuzziPGodinBTanakaTSize and shape effects in the biodistribution of intravascularly injected particlesJ Control Release2010141332032719874859
- ParkJHvon MaltzahnGZhangLSystematic surface engineering of magnetic nanoworms for in vivo tumor targetingSmall20095669470019263431
- DevarajanPVJindalABPatilRRMullaFGaikwadRVSamadAParticle shape: a new design parameter for passive targeting in splenotropic drug deliveryJ Pharm Sci20109962576258120091830
- NaahidiSJafariMEdalatFRaymondKKhademhosseiniAChenPBiocompatibility of engineered nanoparticles for drug deliveryJ Control Release2012166218219423262199
- HowardKAPeerDProviding the full picture: a mandate for standardizing nanoparticle-based drug deliveryNanomedicine (Lond)2013871031103323837825
- DobrovolskaiaMAMcNeilSEImmunological properties of engineered nanomaterialsNat Nanotechnol20772846947818654343
- OwensDE3rdPeppasNAOpsonization, biodistribution, and pharmacokinetics of polymeric nanoparticlesInt J Pharm200630719310216303268
- ChiuYLHoYCChenYMThe characteristics, cellular uptake and intracellular trafficking of nanoparticles made of hydrophobically-modified chitosanJ Control Release2010146115215920580915
- BriggerIDubernetCCouvreurPNanoparticles in cancer therapy and diagnosisAdv Drug Deliv Rev200254563165112204596
- Gomes-da-SilvaLCFernándezYAbasoloIEfficient intracellular delivery of siRNA with a safe multitargeted lipid-based nanoplatformNanomedicine (Lond)2013891397141323394132
- SonajeKLinKJTsengMTEffects of chitosan-nanoparticle-mediated tight junction opening on the oral absorption of endotoxinsBiomaterials201132338712872121862121
- SambruyYFerruzzaSRanaldiGDe AngelisIIntestinal cell culture models: applications in toxicology and pharmacologyCell Biol Toxicol2001174–530131711768596
- LukBTHuCMFangRHInterfacial interactions between natural RBC membranes and synthetic polymeric nanoparticlesNanoscale2014652730273724463706
- LinTYLiYPZhangHTumor-targeting multifunctional micelles for imaging and chemotherapy of advanced bladder cancerNanomedicine (Lond)2013881239125123199207
- LoureiroJAGomesBCoelhoMACarmo PereiraMDRochaSTargeting nanoparticles across the blood–brain barrier with monoclonal antibodiesNanomedicine (Lond)20149570972224827845
- PatelLNZaroJLShenWCell penetrating peptides: intracellular pathways and pharmaceutical perspectivesPharm Res207724111977199217443399
- JonesATGateways and tools for drug delivery: endocytic pathways and the cellular dynamics of cell penetrating peptidesInt J Pharm20083541–2343818068916
- BarefordLMSwaanPWEndocytic mechanisms for targeted drug deliveryAdv Drug Deliv Rev200759874875817659804
- PujalsSGiraltEProline-rich, amphipathic cell-penetrating peptidesAdv Drug Deliv Rev2008604–547348418187229
- OhPBorgströmPWitkiewiczHLive dynamic imaging of caveolae pumping targeted antibody rapidly and specifically across endothelium in the lungNat Biotechnol200725332733717334358
- ObaMAoyagiKMiyataKPolyplex micelles with cyclic RGD peptide ligands and disulfide cross-links directing to the enhanced transfection via controlled intracellular traffickingMol Pharm2008561080109219434856
- LiuJKopeckováPBühlerPBiorecognition and subcellular trafficking of HPMA copolymer-anti-PSMA antibody conjugates by prostate cancer cellsMol Pharm20096395997019344119
- SahooSKMaWLabhasetwarVEfficacy of transferrin-conjugated paclitaxel-loaded nanoparticles in a murine model of prostate cancerInt J Cancer2004112233534015352049
- KocbekPObermajerNCegnarMKosJKristlJTargeting cancer cells using PLGA nanoparticles surface modified with monoclonal antibodyJ Control Release20071201182617509712
- HuangXPengXWangYA reexamination of active and passive tumor targeting by using rod-shaped gold nanocrystals and covalently conjugated peptide ligandsACS Nano20104105887589620863096
- TemsamaniJVidalPThe use of cell-penetrating peptides for drug deliveryDrug Discov Today20049231012101915574317
- PatelPCGiljohannDADanielWLZhengDPrigodichAEMirkinCAScavenger receptors mediate cellular uptake of polyvalent oligonucleotide-functionalized gold nanoparticlesBioconjug Chem201021122250225621070003
- LiuYShiptonMKRyanJSynthesis, stability, and cellular internalization of gold nanoparticles containing mixed peptide-poly(ethylene glycol) monolayersAnal Chem20077962221222917288407
- FortierCDurocherYDe CrescenzoGSurface modification of non-viral nanocarriers for enhanced gene deliveryNanomedicine (Lond)20149113515124354815
- Brannon-PeppasLBlanchetteJONanoparticle and targeted systems for cancer therapyAdv Drug Deliv Rev200456111649165915350294
- VllasaliuDFowlerRGarnettMEatonMStolnikSBarrier characteristics of epithelial cultures modelling the airway and intestinal mucosa: a comparisonBiochem Biophys Res Commun2011415457958522079636
- FerrariMFrontiers in cancer nanomedicine: directing mass transport through biological barriersTrends Biotechnol201028418118820079548
- MasaokaYTanakaYKataokaMSakumaSYamashitaSSite of drug absorption after oral administration: assessment of membrane permeability and luminal concentration of drugs in each segment of gastrointestinal tractEur J Pharm Sci2006293–424025016876987
- KuoTRWuCLHsuCTChemical enhancer induced changes in the mechanisms of transdermal delivery of zinc oxide nanoparticlesBiomaterials200930163002300819232716
- Chen-yuGChun-fenYQi-luLDevelopment of a quercetin-loaded nanostructured lipid carrier formulation for topical deliveryInt J Pharm20124301–229229822486962
- BaroliBEnnasMGLoffredoFIsolaMPinnaRLópez-QuintelaMAPenetration of metallic nanoparticles in human full-thickness skinJ Invest Dermatol200712771701171217380118
- ShimJSeok KangHParkWSHanSHKimJChangISTransdermal delivery of mixnoxidil with block copolymer nanoparticlesJ Control Release200497347748415212879
- Ryman-RasmussenJPRiviereJEMonteiro-RiviereNAPenetration of intact skin by quantum dots with diverse physicochemical propertiesToxicol Sci200691115916516443688
- WangJChenJYeNAbsorption, pharmacokinetics and disposition properties of solid lipid nanoparticles (SLNs)Curr Drug Metab201213444745622443539
- TingSRWhitelockJMTomicRCellular uptake and activity of heparin functionalised cerium oxide nanoparticles in monocytesBiomaterials201334174377438623478040
- GuptaHAqilMKharRKAliABhatnagarAMittalGSparfloxacin-loaded PLGA nanoparticles for sustained ocular drug deliveryNanomedicine20106232433319857606
- FreddoTFA contemporary concept of the blood-aqueous barrierProg Retin Eye Res20123218119523128417
- GuinediASMortadaNDMansourSHathoutRMPreparation and evaluation of reverse-phase evaporation and multilamellar niosomes as ophthalmic carriers of acetazolamideInt J Pharm20053061–2718216263229
- de la FuenteMRaviñaMPaolicelliPSanchezASeijoBAlonsoMJChitosan-based nanostructures: a delivery platform for ocular therapeuticsAdv Drug Deliv Rev201062110011719958805
- MautesAEWeinzierlMRDonovanFNobleLJVascular events after spinal cord injury: contribution to secondary pathogenesisPhys Ther200080767368710869130
- DasMPatilSBhargavaNAuto-catalytic ceria nanoparticles offer neuroprotection to adult rat spinal cord neuronsBiomaterials200728101918192517222903
- Garcia-GarciaEAndrieuxKGilSA methodology to study intracellular distribution of nanoparticles in brain endothelial cellsInt J Pharm2005298231031415923094
- CostantinoLBoraschiDIs there a clinical future for polymeric nanoparticles as brain-targeting drug delivery agents?Drug Discov Today201217736737822094246
- HynynenKUltrasound for drug and gene delivery to the brainAdv Drug Deliv Rev200860101209121718486271
- StamRElectromagnetic fields and the blood–brain barrierBrain Res Rev2010651809720550949
- ReidDMPerryVHAnderssonPBGordonSMitosis and apoptosis of microglia in vivo induced by an anti-CR3 antibody which crosses the blood–brain barrierNeuroscience19935635295338255420
- KuoYCLeeCLMethylmethacrylate-sulfopropylmethacrylate nanoparticles with surface RMP-7 for targeting delivery of antiretroviral drugs across the blood–brain barrierColloids Surf B Biointerfaces201290758222024400
- DhanikulaRSArgawABouchardJHildgenPMethotrexate loaded polyether-copolyester dendrimers for the treatment of gliomas: enhanced efficacy and intratumoral transport capabilityMol Pharm20085110511618171013
- PangZGaoHYuYBrain delivery and cellular internalization mechanisms for transferrin conjugated biodegradable polymersomesInt J Pharm2011415128429221651966
- MartinsSThoIReimoldIBrain delivery of camptothecin by means of solid lipid nanoparticles: formulation design, in vitro and in vivo studiesInt J Pharm20124391–2496223046667
- WenCJYenTCAl-SuwayehSAChangHWFangJYIn vivo real-time fluorescence visualization and brain-targeting mechanisms of lipid nanocarriers with different fatty ester: oil ratiosNanomedicine (Lond)2011691545155922077462
- ThorneRGNicholsonCIn vivo diffusion analysis with quantum dots and dextrans predicts the width of brain extracellular spaceProc Natl Acad Sci U S A2006103145567557216567637
- ZhangXChenGWenLNovel multiple agents loaded PLGA nanoparticles for brain delivery via inner ear administration: in vitro and in vivo evaluationEur J Pharm Sci2013484–559560323354153
- MistryAStolnikSIllumLNanoparticles for direct nose-to-brain delivery of drugsInt J Pharm2009379114615719555750
- GengYDalhaimerPCaiSShape effects of filaments versus spherical particles in flow and drug deliveryNat Nanotechnol20072424925518654271
- JacobsFWisseEDe GeestBThe role of liver sinusoidal cells in hepatocyte-directed gene transferAm J Pathol20101761142119948827
- JohnstonHJSemmler-BehnkeMBrownDMKreylingWTranLStoneVEvaluating the uptake and intracellular fate of polystyrene nanoparticles by primary and hepatocyte cell lines in vitroToxicol Appl Pharmacol20102421667819799923
- LinALiuYHuangYGlycyrrhizin surface-modified chitosan nanoparticles for hepatocyte-targeted deliveryInt J Pharm2008359124725318457928
- KimTHParkIKNahJWChoiYJChoCSGalactosylated chitosan/DNA nanoparticles prepared using water-soluble chitosan as a gene carrierBiomaterials200425173783379215020154
- DoshiNMitragotriSMacrophages recognize size and shape of their targetsPLoS One201054e1005120386614
- LaiSKWangYYHanesJMucus-penetrating nanoparticles for drug and gene delivery to mucosal tissuesAdv Drug Deliv Rev200961215817119133304
- MadhavNVShakyaAKShakyaPSinghKOrotransmucosal drug delivery systems: a reviewJ Control Release20091401–221119665039
- TeublBJAbsengerMFröhlichELeitingerGZimmerARobleggEThe oral cavity as a biological barrier system: design of an advanced buccal in vitro permeability modelEur J Pharm Biopharm201384238639323291061
- KochutADerschPBacterial invasion factors: tools for crossing biological barriers and drug delivery?Eur J Pharm Biopharm201384224225023207324
- CocoRPlapiedLPourcelleVDrug delivery to inflamed colon by nanoparticles: comparison of different strategiesInt J Pharm2012440131222820482
- YangMLaiSKWangYYBiodegradable nanoparticles composed entirely of safe materials that rapidly penetrate human mucusAngew Chem Int Ed Engl201150112597260021370345
- O’NeillMJGuoJByrneCDarcyRO’DriscollCMMechanistic studies on the uptake and intracellular trafficking of novel cyclodextrin transfection complexes by intestinal epithelial cellsInt J Pharm20114131–217418321530624
- des RieuxARagnarssonEGGullbergEPréatVSchneiderYJArturssonPTransport of nanoparticles across an in vitro model of the human intestinal follicle associated epitheliumEur J Pharm Sci205525445546515946828
- BrandenbergerCRothen-RutishauserBMühlfeldCEffects and uptake of gold nanoparticles deposited at the air-liquid interface of a human epithelial airway modelToxicol Appl Pharmacol20102421566519796648
- RotoliBMBussolatiOBianchiMGNon-functionalized multi-walled carbon nanotubes alter the paracellular permeability of human airway epithelial cellsToxicol Lett200817829510218403140
- WanakulePLiuGWFleuryATRoyKNano-inside-micro: disease-responsive microgels with encapsulated nanoparticles for intracellular drug delivery to the deep lungJ Control Release2012162242943722841795
- VllasaliuDAlexanderCGarnettMEatonMStolnikSFc-mediated transport of nanoparticles across airway epithelial cell layersJ Control Release2012158347948622200577
- HaraldssonBNyströmJDeenWMProperties of the glomerular barrier and mechanisms of proteinuriaPhysiol Rev200888245148718391170
- HeBJiaZDuWThe transport pathways of polymer nanoparticles in MDCK epithelial cellsBiomaterials201334174309432623478037
- ChristensenEIVerroustPJNielsenRReceptor-mediated endocytosis in renal proximal tubulePflugers Arch200945861039104819499243
- BertrandNLerouxJCThe journey of a drug-carrier in the body: an anatomo-physiological perspectiveJ Control Release2012161215216322001607
- KodairaHTsutsumiYYoshiokaYThe targeting of anionized polyvinylpyrrolidone to the renal systemBiomaterials200425184309431515046921
- YamamotoYTsutsumiYYoshiokaYPoly(vinylpyrrolidone-co-dimethyl maleic acid) as a novel renal targeting carrierJ Control Release200495222923714980771
- YuanZXZhangZRZhuDSpecific renal uptake of randomly 50% N-acetylated low molecular weight chitosanMol Pharm20086130531419035784
- NarayananKYenSKDouQMimicking cellular transport mechanism in stem cells through endosomal escape of new peptide-coated quantum dotsSci Rep20133218423851637
- TkachenkoAGXieHColemanDMultifunctional gold nanoparticle-peptide complexes for nuclear targetingJ Am Chem Soc2003125164700470112696875
- ChenJWuCOupickýDBioreducible hyperbranched poly(amido amine)s for gene deliveryBiomacromolecules200910102921292719743843