Abstract
In order to enhance the bioavailability of poorly water-soluble curcumin, solution-enhanced dispersion by supercritical carbon dioxide (CO2) (SEDS) was employed to prepare curcumin nanoparticles for the first time. A 24 full factorial experiment was designed to determine optimal processing parameters and their influence on the size of the curcumin nanoparticles. Particle size was demonstrated to increase with increased temperature or flow rate of the solution, or with decreased precipitation pressure, under processing conditions with different parameters considered. The single effect of the concentration of the solution on particle size was not significant. Curcumin nanoparticles with a spherical shape and the smallest mean particle size of 325 nm were obtained when the following optimal processing conditions were adopted: P =20 MPa, T =35°C, flow rate of solution =0.5 mL·min−1, concentration of solution =0.5%. Fourier transform infrared (FTIR) spectroscopy measurement revealed that the chemical composition of curcumin basically remained unchanged. Nevertheless, X-ray powder diffraction (XRPD) and thermal analysis indicated that the crystalline state of the original curcumin decreased after the SEDS process. The solubility and dissolution rate of the curcumin nanoparticles were found to be higher than that of the original curcumin powder (approximately 1.4 μg/mL vs 0.2 μg/mL in 180 minutes). This study revealed that supercritical CO2 technologies had a great potential in fabricating nanoparticles and improving the bioavailability of poorly water-soluble drugs.
Introduction
Curcumin (1,7-bis(4-hydroxy-3-methoxyphenyl)-1-6-heptadiene-3,5-dione), derived from the rhizome of the plant Curcuma longa, is a yellow-orange polyphenol compound. It is widely used as a spice, food preservative, and flavoring and coloring agent.Citation1 Curcumin exhibits many therapeutic properties, including anti-inflammation, anti-oxidation and anticancer, anti-HIV, and antimicrobial activities. Besides, it can inhibit lipid peroxidation and scavenge superoxide anion, singlet oxygen, nitric oxide, and hydroxyl radicals.Citation2
Despite displaying multiple beneficial pharmacological effects, curcumin suffers from extremely low aqueous solubility in water at physiological pH.Citation3 The poor solubility of curcumin limits its absorption and results in low bioavailability.Citation4 Many new drug delivery systems have been developed to increase the aqueous solubility and the bioavailability of curcumin by using a variety of drug carriers, including liposomes,Citation5 phospholipid,Citation6 cyclodextrin,Citation7 chitosan nanoparticles,Citation8 protein nanoparticles such as silk fibroin,Citation9 zein,Citation10 and bovine serum albumin,Citation11 synthetic polymer nanoparticles such as PLGA,Citation12,Citation13 PCL-PEG-PCL triblock copolymer,Citation14 and polymer nanofibers such as polyvinyl alcohol (PVA),Citation15 poly-(ε-caprolactone),Citation16 and zein.Citation17 Another approach for improving the bioavailability of poorly water-soluble drugs is the micronization of these drugs. Decreasing the particle size of these drugs can increase their surface area, thus enhancing the solubility and dissolution rate of these drugs.Citation18 Recently, the wet-milling technique, a type of mechanical communication process, was used to prepare the curcumin nanoparticles of size ranging from 2 to 40 nm.Citation2 However, mechanical communication does not represent the ideal micronization process since both the properties of the compound and the surface properties of the particles might be altered in an uncontrolled manner. Flash nanoprecipitation from a coarse oil-in-water emulsion was developed to fabricate the curcumin nanoparticles with a mean size of about 40 nm.Citation19 In this process, spray-drying was employed to obtain fine curcumin powders. However, this method may suffer from the residual organic solvent and surfactants. Furthermore, spray-drying requires high temperatures and may induce denaturation of active substances.
Recently, supercritical carbon dioxide (CO2) (scCO2) technologies have shown great potential in the field of particle formation, especially drug micronization.Citation20 The scCO2 technologies present many advantages, including low critical conditions (Tc =304.1 K, Pc =7.38 MPa), few or no solvent residue, non-flammability, low cost, and being environmentally benign.Citation21 So far, the most commonly used technique for particle formation using scCO2 is the supercritical antisolvent (SAS) process, in which scCO2 is used as an antisolvent for the precipitation of drugs dissolved in organic solvents. In the SAS process, an organic solution containing the solute is sprayed via a nozzle to a high-pressure vessel with scCO2, resulting in the formation of a complete mixture of the organic solution and scCO2. Continuous feeding of scCO2 induces the supersaturation of active substances. Finally, particles can be precipitated in the vessel.
Solution-enhanced dispersion by scCO2 (SEDS) is a modified SAS process. In this process, a solution containing the solute and scCO2 are atomized via a specially designed coaxial nozzle to obtain droplets of small size and enhance mixing to increase mass transfer rates. In addition to acting as an antisolvent, scCO2 is used as a “dispersing agent” to improve mass transfer between scCO2 and droplets. Therefore, very small particles can be produced.Citation22 Particle size distribution and morphology of the solute can also be controlled by adjusting the parameters of the SEDS process, including the concentration of the solute, flow rate of the solution, temperature, and pressure of scCO2. However, studies on the formation of curcumin nanoparticles by scCO2 technologies, especially the SEDS process, remain unreported, although scCO2 technologies offer great advantages for the formation of nanoparticles.
In the present study, the SEDS process was used to prepare curcumin nanoparticles. A full factorial experiment was designed to systematically investigate the actual effects of the processing parameters, including the flow rate of curcumin solution, precipitation pressure, concentration of curcumin solution, and precipitation temperature, on particle size. Fourier transform infrared (FTIR) spectroscopy measurement, X-ray powder diffraction (XRPD), differential scanning calorimetry (DSC), and thermogravimetric (TG) analysis were used to study the chemical and physical properties of the nanoparticles fabricated using the SEDS process. Furthermore, the solubility and dissolution rate of curcumin were also measured to evaluate its pharmaceutical performance.
Materials and methods
Materials
Curcumin was purchased from International Laboratory (USA). CO2 with a purity of 99.9% was supplied by Hong Kong Specialty Gases Co., Ltd. (Hong Kong). The solvent, acetone, was purchased from Advanced Technology & Industrial Co., Ltd. (Hong Kong). All other compounds were of analytical purity.
Preparation of curcumin nanoparticles by the SEDS process
The experimental apparatus of the SEDS process consists of three major sections: a CO2 supply system, an organic solution delivery system, and a high-pressure vessel. The schematic diagram of the SEDS process is shown in . In this process, when the desired experimental conditions were reached, curcumin dissolved in acetone was injected into the high-pressure vessel via a stainless steel coaxial nozzle simultaneously with scCO2 using a high performance liquid chromatography pump. After all the solution was delivered into the high-pressure vessel, fresh CO2 was pumped to wash precipitated particles for about 30 minutes to eliminate any residual organic solvent. During the washing process, the precipitation temperature and pressure were maintained as described above. After the washing process, the CO2 flow was stopped and the CO2 in the high-pressure vessel was slowly depressurized to atmospheric pressure. The curcumin nanoparticles were then collected on the filter at the bottom of the high-pressure vessel for characterization.
Full factorial designs (FFD)
To investigate the influence and significance of the four process parameters (the flow rate of curcumin solution, precipitation pressure, concentration of curcumin solution and precipitation temperature) in the SEDS process on both the morphology and particle size of curcumin nanoparticles, a 24 full factorial experiment, was designed and conducted, as shown in . According to FFD, there are 16 experiments (shown in ). The MINITAB software version 15 was applied to set the experiments and analyze the experimental data.
Table 1 Experimental factors and levels
Table 2 Experimental design and results of full factorial design
Surface morphology, particle size, and particle size distribution
The surface morphology of curcumin nanoparticles was visualized by scanning electron microscopy (FE-SEM) (JSM-6490; JEOL, Tokyo, Japan). Before analysis, samples were attached onto carbon paint and placed on an aluminum sample holder. These samples were then gold sputter-coated under argon prior to imaging. Curcumin nanoparticles were made conductive by sputtering a thin layer of gold onto their surface. The particle size and particle size distribution of the samples were analyzed by SmileView software from the SEM photographs.
FTIR analysis
The samples were combined with potassium bromide, and the mixtures of the samples and potassium bromide were pressed into a transparent tablet. The FTIR spectra for the samples were recorded on an FTIR Perkin Elmer 1720 (Perkin Elmer, USA) in the transmission mode with the wave number ranging from 4,000 to 400 cm−1.
XRPD analysis
XRPD was performed by an X-ray diffractometer with a Cu Kα (λ =1.5405 Å) radiation (D8 Advance; Bruker AXS, Germany). The measurement was carried out in a 2θ range of 5°~45° with a 0.02° step size and 10° min−1 scan speed with a 2 D detector at 40 kV and 40 mA.
Thermal analysis
DSC samples were analyzed by DSC thermogram analysis (Simultaneous Thermal Analyzer [STA] 6000; Perkin Elmer, MA, USA) at a heating rate of 10°C min−1 over a temperature range of 25°C–400°C in a nitrogen (N2) atmosphere. Each sample was sealed separately in a standard aluminum pan. TG analysis was carried out using a Shimadzu TG analyzer (TGA50; Kyoto, Japan) in a nitrogen atmosphere. The heating rate of the experiment was 10°C min−1. Nitrogen flow was maintained at 50 mL·min−1.
Solubility and dissolution rate measurements
Solubility and dissolution rate tests of the original curcumin and the curcumin nanoparticles were conducted using a modified dialysis bag method. An excessive amount of each sample (10 mg) was dispersed in 5 mL of PBS (pH 7.4) and placed in a pretreated dialysis bag (Molecular Weight: 12,000–14,000 Daltons), and then the bag was put into 50 mL of PBS and incubated in a water bath at 37°C and 60 rpm. At a specific time, 1 mL of the solution was removed, followed by centrifugation (10,000× g, 10 minutes). Next, the concentration of the supernatant was detected by UV spectrometry (PerkinElmer Lambda 18; USA) at 410 nm. The dissolution rate was calculated in terms of the curcumin concentration and incubation time. Each experiment was carried out in triplicate.
Results and discussion
Surface morphology and particle size of curcumin nanoparticles
shows the surface morphology of the original curcumin powders before the SEDS process. The original curcumin powders were irregular and exhibited a mean size of approximately 3.58 μm. The experimental design and results of 24 FFD experiments are presented in . shows the SEM micrographs of the curcumin nanoparticles fabricated by the SEDS process in different runs, the details of which are listed in . It can be concluded from that the curcumin nanoparticles possessed an irregular spherical morphology with a mean particle size from approximately 325 to 1,024 nm. The surface morphology of the biggest and smallest curcumin particles are shown in , respectively. Therefore, optimal conditions for preparing the smallest curcumin nanoparticles (325 nm) were as follows: P =20 MPa; T =35°C; flow rate of the solution =0.5 mL·min−1; and concentration of the solution =0.5%. Evidently, the SEDS process is an effective way to prepare curcumin nanoparticles. Moreover, by adjusting the different process parameters of the SEDS process, curcumin nanoparticles with controllable particle size can be prepared successfully.
Figure 2 SEM micrograph of the original curcumin powders.
Abbreviation: SEM, scanning electron microscopy.
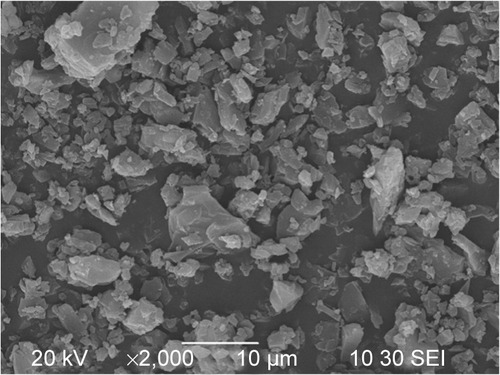
Figure 3 SEM micrographs of curcumin nanoparticles prepared by the SEDS process under different conditions (A) 0.5%-35°C-0.5 mL/min-20 Mpa; (B) 0.5%-45°C-1 mL/min-10 Mpa; (C) 0.5%-45°C-0.5 mL/min-10 Mpa; (D) 5%-45°C-0.5 mL/min-20 Mpa; (E) 0.5%-35°C-1 mL/min-10 Mpa; (F) 1%-45°C-0.5 mL/min-20 Mpa; (G) 1%-45°C-1 mL/min-20 Mpa; (H) 1%-35°C-1 mL/min-20 Mpa; (I) 0.5%-35°C-1 mL/min-20 Mpa.
Abbreviations: SEM, scanning electron microscopy; SEDS, solution-enhanced dispersion by supercritical carbon dioxide (CO2).
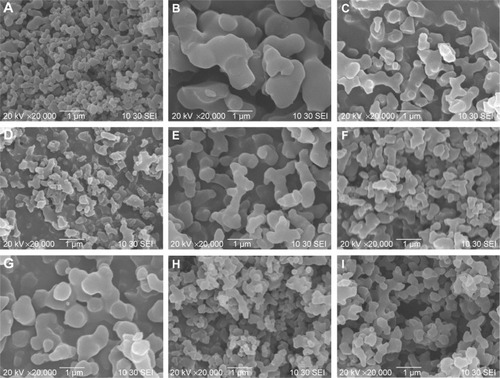
Influence of process parameters on particle size
The FFD is a suitable technique to estimate the effects of process parameters on the process outcomes. For the evaluation of the main effect of process parameters including the precipitation pressure (A), precipitation temperature (B), flow rate of curcumin solution (C), and concentration of curcumin solution (D) on the mean particle size of curcumin nanoparticles, the quantitative data analysis for the results shown in was performed. and shows the standardized effect of the factors on particle size and the main effects plot for particle size, respectively. lists the estimated effects and coefficients for particle size.
Table 3 Estimated effects and coefficients for particle size (nm) (coded units)
As shown in and , A–C (P<0.05) significantly affected the size of curcumin nanoparticles. However, D (P>0.05) had an insignificant effect on particle size. According to the slopes of the lines in , the order of importance of the factors affecting particle size can be summarized as follows: B > A > D > C. Within the range of parameters studied, particle size increased with increased temperature or flow rate of solution, and decreased precipitation pressure. The effect of concentration of the solution alone on particle size was not significant, and decreasing the concentration can reduce the particle size of curcumin nanoparticles slightly.
Effect of precipitation pressure
As shown in , an increase in the precipitation pressure induced a reduction in the particle size of curcumin. Runs 3 and 4 () show that increasing the pressure from 10 to 20 Mpa led to a decrease in the particle size of curcumin nanoparticles from 694 to 337 nm. This trend was also observed in the morphology of curcumin nanoparticles shown in . Zhao et al also reported a similar phenomenon.Citation23
The nucleation and growth mechanism induced by supersaturation can be used to explain the result. In the SEDS process, mutual diffusion between the liquid solution and scCO2 resulted in phase separation and supersaturation of the solution, thus leading to the nucleation and precipitation of particles. Lower supersaturation ratios resulted in fewer nuclei, which in turn yielded larger particles. An increase in pressure led to an increase in the CO2 density and mole fraction, thereby enhancing the antisolvent effect of scCO2. Therefore, supersaturation within the liquid phase was more quickly reached, preventing the crystals from growing and inducing the formation of particles of smaller size.Citation24
Effect of precipitation temperature
and show that precipitation temperature significantly influenced particle size, and increasing the temperature causes an increase in the particle size of curcumin nanoparticles. Runs 5 and 7 () indicated that an increase in temperature from 35°C to 45°C would result in an increase in the size of curcumin nanoparticles from 547 to 1,024 nm. These results were supported by surface morphologies shown in .
Temperature can affect the density of supercritical fluid. A small change in the precipitation temperature can lead to considerable density changes, especially near the critical point.Citation25 The increased in temperature caused decreased density of scCO2, thus resulting in a decrease of the antisolvent effect of scCO2. Therefore, achieving supersaturation was slowed and a bigger particle was formed. Similar results were also observed in other studies.Citation26
Effect of flow rate of solution
and show that the flow rate of the solution significantly affected the size of curcumin nanoparticle, and a higher flow rate of the solution resulted in smaller particles. When the flow rate of curcumin solution was raised from 0.5 to 1 mL·min−1 as indicated in , the size of curcumin nanoparticles increased from 372 nm of Run 12 to 692 nm of Run 16 ().
An increased in the flow rate of the solution resulted in increased solution content in the precipitator within the same time and decreased the antisolvent effect of scCO2, thus reducing the achievable supersaturation ratio. Lower supersaturation ratios generated fewer nuclei, which in turn yielded larger particles. Additionally, the micronization process shifted toward the growth process, and so, larger particles were produced.Citation27 Previous researchers have also reported similar results.Citation28,Citation29
Effect of concentration of solution
As indicated in and , the concentration of solution had a slight effect on particle size; hence, the higher the concentration of solution, the smaller the particle size. Runs 6 and 14 () showed that an increase in the concentration of curcumin solution from 0.5% to 1% can decrease the size of curcumin nanoparticles from 386 to 334 nm. Surface morphologies shown in demonstrated this trend. Chen et al reported similar results.Citation30
This finding can be explained by considering that a higher condensation rate from a higher concentration tended to result in a higher supersaturation, and thus smaller particles were expected.Citation31 It is consistent with the classical nucleation and growth theory. However, in the operating conditions, the concentration of solution affected the particle size of curcumin nanoparticles slightly.
FTIR analysis
FTIR can reveal the chemical changes by producing an infrared absorption spectrum. shows the FTIR spectra of original curcumin and curcumin nanoparticles prepared by the SEDS process under optimal conditions. It can be seen that FTIR spectra of original curcumin and curcumin nanoparticles prepared by the SEDS process did not show significant differences. Thus, there is no change in the chemical composition of the samples before and after the SEDS process. The characteristic peaks at 1,581 cm−1, 1,511 cm−1, 1,279 cm−1, and 1,152 cm−1 were attributed to the stretching vibrations of the benzene ring, C=C vibrations, aromatic C-O stretching, and C-O-C stretching modes, respectively, in original curcumin.Citation14 After the SEDS process, the main peak at 1,152 cm−1 found in the FTIR spectra of original curcumin shifted to 1,144 cm−1. This finding indicated that minor structural changes occurred at the molecular level. A previous study also reported such a minor structure change of samples after the supercritical CO2 process.Citation30 However, no observable new characteristic peaks were found in the FTIR spectra of curcumin nanoparticles. Therefore, there was no change in the chemical composition of the samples before and after the SEDS process.
XRPD analysis
XRPD is an important technique employed to determine the crystal phase of the samples. shows the XRPD pattern of original curcumin and curcumin nanoparticles prepared by the SEDS process under optimal conditions. The characteristic high-intensity diffraction peaks of the original curcumin indicated it was in a highly crystalline form before the SEDS process.Citation32 However, the intensity of these characteristic diffraction peaks weakened after the SEDS process. Curcumin nanoparticles showed a lower value for the peak areas (indicative of crystallinity) than that of original curcumin (556.5 vs 828.3). Furthermore, the peaks at 2θ =13.4°, 16.3°, 20.1°, and 21.8° disappeared. These results demonstrated that the precipitation by the SEDS process decreased the crystallinity of the original curcumin, and the curcumin nanoparticles exhibited a more amorphous state than original curcumin. A crystalline-amorphous transition resulted in excess free energy and entropy. Compared with the crystalline state, the amorphous state was thermodynamically less stable.Citation31 Consequently, the decreased crystallinity of original curcumin by the SEDS process favors an increased dissolution rate of a drug powder.
Thermal analysis
DSC is widely used to characterize the thermal behavior of various materials, such as melting and crystallization behaviors. The DSC curves of original curcumin and curcumin nanoparticles prepared by the SEDS process under optimal conditions are given in . For original curcumin, a sharp peak at 164.4°C corresponding with the melting point was found.Citation33 After the SEDS process, the melting point of curcumin was unchanged and a sharp peak at 164.4°C was still observed for curcumin nanoparticles. However, curcumin nanoparticles showed a lower value for the endothermic peak areas (indicative of the crystallization extent) than that of original curcumin (705.4 J·g−1 vs 985.1 J·g−1). The reduction in the integral area can be attributed to the disrupted molecule chains and the decreased crystallization extent caused by the SEDS process. This result was consistent with that of XRPD analysis.
Figure 8 DSC curves of original curcumin and curcumin nanoparticles prepared by the SEDS process under optimal conditions.
Abbreviations: DSC, differential scanning calorimetry; SEDS, solution-enhanced dispersion by supercritical carbon dioxide (CO2).

TG curves corresponding with the thermal weight losses of original curcumin and the curcumin nanoparticles prepared by the SEDS process under optimal conditions are shown in . Upon heating, original curcumin suffered a pronounced weight loss step occurring near 100°C that can be ascribed to the loss of water composition. However, weight loss was not observed in the curcumin nanoparticles. In addition, based on the tangent method, the decomposition temperatures of original curcumin and curcumin nanoparticles were found to be 273.6°C and 266.7°C, respectively. Thus, the thermal stability of curcumin decreased after the SEDS process owing to decreased crystalline structure. The result well agreed with those of DSC and XPRD analyses.
Solubility and dissolution rate
shows the dissolution profiles of original curcumin and curcumin nanoparticles prepared by the SEDS process under optimal conditions. The kinetic solubility curve of original curcumin very quickly reached the plateau. The maximum solubility of original curcumin was about 0.2 μg/mL at 60 minutes. After the SEDS process, the resulting curcumin nanoparticles exhibited a maximum solubility of approximately 1.4 μg/mL. Moreover, the dissolution rate of curcumin nanoparticles was higher than that of original curcumin, particularly in the first 180 minutes. The dramatically increased dissolution rate and solubility of curcumin nanoparticles can be attributed to the increased specific surface area for better wettability resulting from the reduced particle size. Curcumin nanoparticles also exhibited less crystallinity than original curcumin. A decrease in crystallinity resulted in decreased thermodynamic stability, thereby favoring increased dissolution rate and bioavailability of curcumin powder.
Conclusion
Curcumin nanoparticles were fabricated for the first time using SEDS. The investigation using 24 FFD indicated that an increase in the flow rate of curcumin solution and the precipitation temperature increased the size of curcumin nanoparticles, whereas a reduction in the precipitation pressure increased the particle size. The single effect of the concentration of the solution on particle size was insignificant. Given the reduction in particle size and crystallinity, the fabricated curcumin nanoparticles possessed higher solubility and hence a higher dissolution rate than original curcumin. These results demonstrated that the SEDS process is an effective method for preparing curcumin nanoparticles and improving the solubility and dissolution rate of poorly water-soluble drugs.
Acknowledgments
We thank the Hong Kong Research Grant Council and the Hong Kong Polytechnic University through projects PolyU5242/09E and G-YM63. We would also like to thank the support provided by the Natural Science Foundation of Hubei Province through project 2014CFB839, Doctoral Research Fund of Wuhan University of Technology through project 471-40120093, Guangdong Provincial Department of Science and Technology through projects 2012B050800002 and 2012B091000143.
Disclosure
The authors report no conflicts of interest in this work.
References
- SunYDuLLiuYTransdermal delivery of the in situ hydrogels of curcumin and its inclusion complexes of hydroxypropyl-β-cyclodextrin for melanoma treatmentInt J Pharm20144691313924746691
- BhawanaBasniwalRKButtarHSJainVKJainNCurcumin nanoparticles: preparation, characterization, and antimicrobial studyJ Agr Food Chem20115952056206121322563
- YallapuMMJaggiMChauhanSCCurcumin nanoformulations: a future nanomedicine for cancerDrug Discov Today2012171–2718021959306
- AnandPKunnumakkaraABNewmanRAAggarwalBBBioavailability of curcumin: problems and promisesMol Pharm20074680781817999464
- TakahashiMUechiSTakaraKAsikinYWadaKEvaluation of an oral carrier system in rats: bioavailability and antioxidant properties of liposome-encapsulated curcuminJ Agr Food Chem200957199141914619757811
- MaitiKMukherjeeKGantaitASahaBPMukherjeePKCurcumin-phospholipid complex: preparation, therapeutic evaluation and pharmacokinetic study in ratsInt J Pharm20073301–215516317112692
- MarcolinoVAZaninGMDurrantLRBenassiMDTMatioliGInteraction of curcumin and bixin with β-cyclodextrin: complexation methods, stability, and applications in foodJ Agr Food Chem20115973348335721381747
- AkhtarFRizviMMKarSKOral delivery of curcumin bound to chitosan nanoparticles cured Plasmodium yoelii infected miceBiotechnol Adv201230131032021619927
- GuptaVAsehARiosCNAggarwalBBMathurABFabrication and characterization of silk fibroin-derived curcumin nanoparticles for cancer therapyInt J Nanomed200941115122
- PatelAHuYCTiwariJKSynthesis and characterization of zein-curcumin colloidal particlesSoft Matter201062461926199
- JithanAVMadhaviKMadhaviMPrabhakarKPreparation and characterization of albumin nanoparticles encapsulating curcumin intended for the treatment of breast cancerInt J Pharm Invest201112119125
- BeloquiACocoRMemvangaPBUcakarBdes RieuxAPréatVpH-sensitive nanoparticles for colonic delivery of curcumin in inflammatory bowel diseaseInt J Pharm20144731–220321225014369
- YallapuMMGuptaBKJaggiMChauhanSCFabrication of curcumin encapsulated PLGA nanoparticles for improved therapeutic effects in metastatic cancer cellsJ Colloid Interf Sci20103511929
- FengRSongZZhaiGPreparation and in vivo pharmacokinetics of curcumin-loaded PCL-PEG-PCL triblock copolymeric nanoparticlesInt J Nanomedicine201274089409822888245
- SunXZWilliamsGRHouXXZhuLMElectrospun curcumin-loaded fibers with potential biomedical applicationsCarbohyd Polym2013941147153
- MerrellJGMcLaughlinbSWTieLLaurencindCTChenAFNairLSCurcumin loaded poly(ε-caprolactone) nanofibers: wound dressing with antioxidant and anti-inflammatory propertiesClin Exp Pharmacol P2009361211491156
- BrahatheeswaranDMathewAAswathyRGHybrid fluorescent curcumin loaded zein electrospun nanofibrous scaffold for biomedical applicationsBiomed Mater20127404500122556150
- TagamiTImaoYItoSNakadaAOzekiTSimple and effective preparation of nano-pulverized curcumin by femtosecond laser ablation and the cytotoxic effect on C6 rat glioma cells in vitroInt J Pharm20144681–2919624709219
- MargulisKMagdassiSLeeHSMacoskoCWFormation of curcumin nanoparticles by flash nanoprecipitation from emulsionsJ Colloid Interface Sci2014434657025168584
- LiuGWangWWangHJiangYPreparation of 10-hydroxycamptothecin proliposomes by the supercritical CO2 anti-solvent processChem Eng J2014243289296
- ZhaoZChenAZLiYFabrication of silk fibroin nanoparticles for controlled drug deliveryJ Nanopart Res2012144736745
- ZhaoZLiYZhangYDevelopment of silk fibroin modified poly(l-lactide)-poly(ethylene glycol)-poly(l-lactide) nanoparticles in supercritical CO2Powder Technol2014268118125
- ZhaoZLiYChenAZGeneration of silk fibroin nanoparticles via solution-enhanced dispersion by supercritical CO2Ind Eng Chem Res2013521037523761
- JinHYHemingwayMXiaFLiNSZhaoYPProduction of β-Carotene nanoparticles by the solution enhanced dispersion with enhanced mass transfer by ultrasound in supercritical CO2 (SEDS-EM)Ind Eng Chem Res201150231347513484
- KalaniMYunusRApplication of supercritical antisolvent method in drug encapsulation: a reviewInt J Nanomedicine201161429144221796245
- LeeBMKimSJLeeBCKimHSKimHLeeYWPreparation of micronized β-HMX using supercritical carbon dioxide as antisolventInd Eng Chem Res2011501591079115
- WangQGuanYXYaoSJZhuZQMicroparticle formation of sodium cellulose sulfate using supercritical fluid assisted atomization introduced by hydrodynamic cavitation mixerChem Eng J2010159220229
- ReverchonEDe MarcoISupercritical antisolvent precipitation of cephalosporinsPowder Technol20061643139146
- SuCSLoWSLienLHMicronization of fluticasone propionate using supercritical antisolvent processChem Eng Technol2011344535541
- ChenAZLiLWangSBNanonization of methotrexate by solution-enhanced dispersion by supercritical CO2J Supercrit Fluid201267713
- MontesATenorioAGordillMDPereyraCMartinez de la OssaEJScreening design of experiment applied to supercritical antisolvent precipitation of amoxicillin: exploring new miscible conditionsJ Supercrit Fluid201051399403
- SongZZhuWLiuNYangFFengRLinolenic acid-modified PEG-PCL micelles for curcumin deliveryInt J Pharm20144711–231232124939613
- XieXTaoQZouYPLGA nanoparticles improve the oral bioavailability of curcumin in rats: characterizations and mechanismsJ Agr Food Chem201159179280928921797282