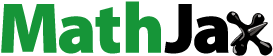
Abstract
Background
The natural compound n-butylidenephthalide (BP) can pass through the blood–brain barrier to inhibit the growth of glioblastoma multiforme tumors. However, BP has an unstable structure that reduces its antitumor activity and half-life in vivo.
Objective
The aim of this study is to design a drug delivery system to encapsulate BP to enhance its efficacy by improving its protection and delivery.
Methods
To protect its structural stability against protein-rich and peroxide solutions, BP was encapsulated into a lipo-PEG-PEI complex (LPPC). Then, the cytotoxicity of BP/LPPC following preincubation in protein-rich, acid/alkaline, and peroxide solutions was analyzed by MTT. Cell uptake of BP/LPPC was also measured by confocal microscopy. The therapeutic effects of BP/LPPC were analyzed in xenograft mice following intratumoral and intravenous injections.
Results
When BP was encapsulated in LPPC, its cytotoxicity was maintained following preincubation in protein-rich, acid/alkaline, and peroxide solutions. The cytotoxic activity of encapsulated BP was higher than that of free BP (~4.5- to 8.5-fold). This increased cytotoxic activity of BP/LPPC is attributable to its rapid transport across the cell membrane. In an animal study, a subcutaneously xenografted glioblastoma multiforme mouse that was treated with BP by intratumoral and intravenous administration showed inhibited tumor growth. The same dose of BP/LPPC was significantly more effective in terms of tumor inhibition.
Conclusion
LPPC encapsulation technology is able to protect BP’s structural stability and enhance its antitumor effects, thus providing a better tool for use in cancer therapy.
Background
The natural compound n-butylidenephthalide (BP), isolated from the chloroform extract of Angelica sinensis, has been reported to have antitumor activity in glioblastoma multiforme (GBM),Citation1–Citation4 prostate cancer,Citation5,Citation6 oral squamous cell carcinoma,Citation7 lung cancer,Citation8 and hepatoma.Citation9 In particular, BP has been developed to treat brain tumors due to its ability to permeate through the blood–brain barrier and enter into the brain.Citation1 BP induces Nur77-mediated apoptosis via the protein kinase C/JNK pathway.Citation4,Citation7,Citation9,Citation10 BP downregulates the expression of S-phase kinase–associated protein (Skp2), which leads to an increase in p16 and p21 expression, causing G0/G1 arrest.Citation2 BP inhibits telomerase activity by repressing hTERT transcriptional activity via the downregulation of Sp1 or AP-2 expression.Citation3,Citation8 BP also regulates the eFAS-dependent pathway, the mitochondrial pathway, and the ER stress pathway that is involved in cell apoptosis.Citation5,Citation6 BP regulates multiple signaling pathways and possesses several therapeutic benefits, including antiangiogenic,Citation11 anti-inflammatory,Citation12,Citation13 and antioxidant properties.Citation12 However, the clinical application of BP is limited due to several problems. For instance, the unstable structure of BP in oxygenic environments causes a loss of its cytotoxicity. In addition, the water insolubility of BP leads to low bioavailability and poor pharmacokinetics in vivo. Its nonspecific distribution throughout the body when administered intravenously also limits its application. To protect the structure of BP during administration and to increase its aqueous solubility/dispersibility, a drug delivery system must be developed for BP encapsulation and treatment.
Currently, various nanoparticles, especially polymer-based liposomes, have been developed to encapsulate anti-tumor drugs, which can enhance their therapeutic efficacy.Citation14 We recently reported the development of a polycationic liposome complex containing PEI and PEG (lipo-PEG-PEI complex [LPPC]) that can encapsulate curcumin and enhance its cytotoxicity.Citation14,Citation15 LPPC helped curcumin rapidly traverse the cell membrane and enabled its delivery into tumor areas to inhibit tumor growth in tumor-bearing mice.Citation14 LPPC has also been used as an adjuvant because it can strongly capture antigens or immunomodulators onto its surface, which allows it to enhance or switch immune responses.Citation16 These results therefore suggest that LPPC may serve as an effective drug carrier and a useful anticancer tool.
In this study, LPPC was used as a drug carrier to encapsulate BP for drug delivery. LPPC protected BP’s structure and cytotoxicity against protein-rich and peroxide solutions. Furthermore, BP encapsulated into LPPC had higher cytotoxic activity than free BP. This increased cytotoxic activity of BP/LPPC was attributable to its rapid transport across the cell membrane. In an animal study, a xenograft GBM mouse was treated with BP/LPPC by intratumoral and intravenous (IV) administration, and BP/LPPC inhibited the growth of subcutaneous GBM tumors. Therefore, LPPC encapsulation technology is able to protect BP’s structural stability and enhance its antitumor effects, providing a better tool for use in cancer therapy. Our Institutional Review Board does not require ethical approval for these types of studies.
Materials and methods
Preparation of LPPC and encapsulation of BP in LPPCs or liposomes
LPPCs were prepared according to a previously described protocol.Citation15 For BP/LPPC preparation, different volume ratios of 1 mM BP (Lancaster Synthesis Ltd, Newgate, Morecambe, UK) and 1 mg of LPPC were vigorously mixed for 15 seconds and incubated for 15 minutes. This procedure was conducted twice. After incubation, the mixture of BP and LPPC was centrifuged at 5,900× g for 5 minutes to remove free BP. The concentration of BP remaining in the supernatant was then measured using a fluorescence spectrophotometer (F-4500; Hitachi Ltd., Tokyo, Japan) with an excitation wavelength of 350 nm and an emission wavelength of 430 nm. The particle size and zeta potential of BP/LPPC and empty LPPC particles were determined by a Zetasizer instrument (Zetasizer 3000HS; Malvern Instruments, Malvern, UK). BP liposomes devoid of PEI and PEG were prepared as described earlier. Briefly, 50 mg DOPC and 50 mg DLPC with 5 mg BP in chloroform were mixed and were coated onto a round-bottom flask by a rotary evaporator (EYELA, N-1000S, Tokyo, Japan) to yield BP liposomes. The lipid films containing BP were hydrated by steam for 2 hours and then 5 mL of ddH2O was added into the container. The BP/lipid films were vigorously resuspended for 10 minutes, and the suspension was extruded through a LiposoFast extruder (Avestin Inc., Ottawa, Canada) via a 200-nm mesh nine times to give the correct particle size.
In vitro drug release from BP/LPPC
BP/LPPCs were suspended in 1 mL of phosphate-buffered saline (PBS; pH 7.4). Following this, the BP/LPPC solution was placed into a dialysis bag (Spectra/Por; Spectrum Laboratories Inc., Rancho Dominguez, CA, USA) with a 6–8 kDa molecular weight cutoff and immersed into 20 mL of PBS at 4°C, 25°C, or 37°C with continuous stirring. In addition, to mimic the release of drug in circulation, the BP/LPPC solution in the dialysis bag was submerged in a protein-rich solution (10% fetal bovine serum [FBS; Thermo Fisher Scientific, Waltham, MA, USA] in PBS) at 37°C. One-milliliter samples were collected from the incubation media at different time intervals, and the BP concentrations in the samples were measured as described earlier. The release rate was calculated as follows:
Cells and culture conditions
GBM tumor cell lines and normal cell lines were purchased from Bioresources Collection and Research Center (Hsinchu, Taiwan). Four human GBM cell lines, DBTRG-05MG, G5T/TGH, GBM8401, and GBM8901 and the N18 mouse neuroblastoma cell line were cultured in RPMI-1640 (Thermo Fisher Scientific) growth media supplemented with 10% heat-inactivated FBS (Thermo Fisher Scientific), 1% sodium pyruvate (Thermo Fisher Scientific), 1% HEPES buffer solution (Thermo Fisher Scientific), and 1% penicillin/streptomycin (Thermo Fisher Scientific). RG2 rat GBM cells, SVEC mouse endothelial cells, and MDCK dog normal kidney cells were cultured in Dulbecco’s Modified Eagle’s Medium (Thermo Fisher Scientific) growth media supplemented with 10% heat-inactivated FBS (Thermo Fisher Scientific), 1% sodium pyruvate (Thermo Fisher Scientific), 1% HEPES buffer solution (Thermo Fisher Scientific), and 1% penicillin/streptomycin (Thermo Fisher Scientific). All cell lines were cultured in a humidified atmosphere of 5% CO2 at 37°C. The cells were subcultured using an enzymatic procedure (0.1% trypsin, 2 mM EDTA solution).
Cytotoxicity of BP/LPPC
The different cell lines were seeded into 96-well tissue culture plates at a concentration of 5×103 cells/100 µL/well and were allowed to grow overnight. The cells were subsequently treated with serial dilutions of the various agents, namely empty LPPC, BP/LPPC, and BP. After 48 hours of incubation, the cell viability of each cell line was determined by an MTT colorimetric assay. Briefly, 100 µL of 2 mg/mL MTT reagent (Sigma-Aldrich, St Louis, MO, USA) was added to each well, and the solution was allowed to incubate for 4 hours at 37°C. Then, the media was aspirated, and 100 µL of dimethyl sulfoxide was added to each well. Finally, the OD595 of each well was measured by an ELISA reader (TECAN, Austria). Cell viability was plotted as a percentage of the untreated control, and the 50% inhibitory concentration (IC50) of each reagent was determined from the dose–effect curve. Specifically, this was the drug concentration that decreased the cell viability to 50%. The following equation was used to calculate the enhancement in cytotoxicity when using the encapsulated form of BP compared with the nonencapsulated form:
The drug-protective effects of LPPC
To evaluate the drug-protective effects of LPPC, changes in the cytotoxic activity of BP and BP/LPPC were measured. First, both nonencapsulated BP and BP/LPPC were incubated in H2O and in protein-rich solutions (10% FBS in PBS) with different pH values at 4°C and 37°C. In addition, nonencapsulated BP and BP/LPPC were exposed to oxygen for 24 hours. Finally, DBTRG-05MG and RG2 cells were treated with pretreated BP and BP/LPPC. Changes in the IC50 values of BP and BP/LPPC were determined by an MTT colorimetric assay.
The delivery-enhancing effects of LPPC in vitro
DBTRG-05MG cells were seeded in six-well tissue culture plates on glass coverslips at a density of 2×105 cells per well. Subsequently, the cells were treated with PBS and 50 mg/mL of BP or BP/LPPC (containing 50 mg/mL of BP). After incubation at 37°C for 0 minutes, 15 minutes, 30 minutes, 60 minutes, and 120 minutes, the media was removed from the cells, and the cells were then washed with PBS, fixed with 4% (w/w) paraformaldehyde in PBS, and imaged at 400× magnification using a Zeiss LSM 510 META confocal microscope (Carl Zeiss Meditec AG, Jena, Germany). Incubation under each condition was performed in triplicate.
To measure the accumulation of BP, 2.5×105 cells/well were treated with 50 mg/mL of BP or BP/LPPC. After incubation at 37°C for 0 minutes, 15 minutes, 30 minutes, 45 minutes, and 60 minutes, the cells were lysed, and BP was extracted by phenol/chloroform and measured by a fluorescence spectrophotometer (Hitachi Ltd.).
For analysis of the colocalization of BP/LPPC, LPPC was first labeled with DiO fluorescent dye and was then used to encapsulate BP. Subsequently, the cells that were seeded on glass coverslips were treated with BP/DiO-LPPC (containing 50 mg/mL of BP). After incubation at 37°C for 0 minutes, 15 minutes, 60 minutes, and 120 minutes, the media was removed from the cells, and the cells were then washed with PBS, fixed with 4% (w/w) paraformaldehyde in PBS, and then incubated with LysoTracker Red DND-99 lysosomal staining probe (Thermo Fisher Scientific). The colocalization of BP and LPPC was imaged at 400× magnification using a Zeiss LSM 510 META confocal microscope (Carl Zeiss).
Antitumor activity of BP/LPPC
Female athymic mice (4–6 weeks of age) were obtained from the National Laboratory Animal Center (Taipei, Taiwan). All procedures were conducted in compliance with the standard operating procedures of the Laboratory Animal Center of Chung Shan Medical University (Taichung, Taiwan). Nude mice (n=10) were implanted via subcutaneous (sc) injection with 5×106 DBTRG-05MG cells. The tumor-bearing animals were treated with BP (100 mg BP/kg), BP/LPPC, or vehicle by IV injection every 2 days after tumor establishment (~50 mm3 tumor volume). In another set of experiments, the tumor-bearing animals were treated with BP (100 mg BP/kg), BP/LPPC, or vehicle by intratumoral injection every 2 days after tumor implantation for 3 days. Tumor size was measured with a caliper, and tumor volume was calculated as L × H × W ×0.5236. The animals were sacrificed when the tumor volume exceeded 2,000 mm3. Tumor and normal tissue sections were observed and photographed under a light microscope at a magnification of 400×.
Immunohistochemical staining
Paraffin-embedded sections were obtained from the tumors treated with BP/LPPC by IV or intratumoral injection and were processed for immunohistochemical staining. Briefly, the slides were treated with 3% hydrogen peroxide in 1× PBS for 10 minutes to block endogenous peroxidase activity after being dewaxed and rehydrated. Next, the sections were washed three times with 1× TBS containing 0.05% Tween 20 for 5 minutes each time, and nonspecific antibody binding was blocked by 10% FBS in PBS for 10 minutes at room temperature. The sections were incubated with a rabbit polyclonal anticleaved caspase-3 (Asp175) antibody (1/1,000 dilution; Cell Signaling Technology) at 4°C overnight, and the immune complexes were visualized using a horseradish peroxidase–conjugated antigoat IgG secondary antibody (1/1,000 dilution; Santa Cruz Biotechnology inc., Dallas, TX, USA). An LSAB2 system (DAKO, Carpinteria, CA, USA) was used to visualize the immune complexes by incubating the sections with 0.5 mg/mL diaminobenzidine and 0.03% (v/v) H2O2 in PBS for 10 minutes. Finally, the sections were counterstained with hematoxylin, mounted, observed under a light microscope at 400× magnification, and photographed.
Statistical analysis
The results were analyzed using the SAS statistical software package (SAS Institute Inc., Cary, NC, USA). The results are expressed as the mean ± standard deviation. An analysis of variance test was used when comparing multiple samples. Differences with P<0.05 were considered statistically significant.
Results
The characteristics of BP/LPPC
The particle sizes of different BP/LPPC mixtures (volume ratios of BP/LPPC from 0.5:10 to 3:10) ranged from 200 nm to 280 nm (). The average zeta-potential of these different BP/LPPC mixtures was ~38 mV (). For BP encapsulation, the maximal encapsulation capacity of 1 mg of LPPC was ~830 µg of BP at 3:10 and 4:10 (v/v) ratios of BP/LPPC (). According to the results of particle size, zeta-potential, and BP encapsulation capacity assessments, a 3:10 ratio of BP/LPPC was chosen for the following experiments.
Figure 1 BP/LPPC characteristics.
Notes: BP solution (1 M) encapsulated in LPPC at various volume ratios. The (A) particle size, (B) zeta-potential, and (C) BP encapsulation of BP/LPPC were measured. (D) BP release from BP/LPPC in H2O at 4°C, 25°C, and 37°C. *P<0.05, compared with the 25°C group; #P<0.05, compared with the 37°C group. (E) BP release from BP/LPPC in protein-rich solution (containing 10% FBS) at 37°C. After the incubation, the percentage of BP in each supernatant was measured and compared with the total amount of BP (n=6). *P<0.05, compared with the control group.
Abbreviations: BP, n-butylidenephthalide; FBS, fetal bovine serum; LPPC, lipo-PEG-PEI complex.
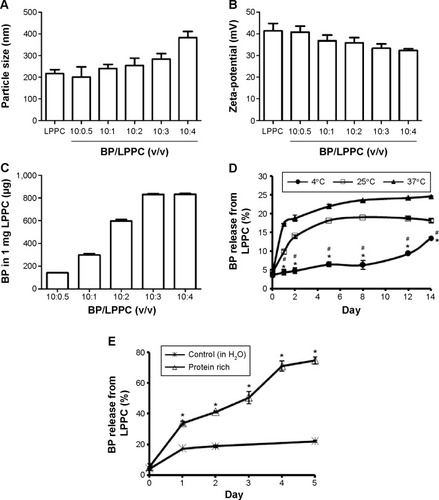
The in vitro drug release of BP from BP/LPPC in H2O was measured, and the kinetics of drug release showed that only 6%–13% of the encapsulated BP was released from BP/LPPC after incubation at 4°C (). Approximately 25% of the encapsulated BP had been released into the media after 12 days at 37°C. However, when BP/LPPC was incubated in protein-rich solution at 37°C, over 70% of the encapsulated BP was released into the media after 4 days ().
The cytotoxic activity of BP/LPPC against tumor cells
To further determine whether the encapsulation of BP into BP/LPPC could enhance its cytotoxicity, different GBM cell lines were analyzed for cell viability after BP or BP/LPPC treatment. The results indicated that the IC50 levels for nonencapsulated BP ranged from 54.9 µg/mL (DBTRG-05MG cells) to 122.7 µg/mL (GBM8901 cells); however, treating the cells with BP/LPPC resulted in lower IC50 values in all six of the cancer cell lines that were tested. Compared with nonencapsulated BP, the IC50 levels of BP/LPPC were markedly decreased in all cancer cell lines, ranging from 11.6 µg/mL to 17.5 µg/mL (). Thus, BP/LPPC was able to enhance the cytotoxic activity of BP by between 4.5- and 8.5-fold. These results were dramatic when compared with other delivery methods, such as BP/liposomes (). In addition, the IC50 levels of BP/LPPC ranged from 24.3 µg/mL to 32.2 µg/mL for normal cells, such as SVEC4-10 and MDCK cells (). In this study, empty LPPC did not have cytotoxic effects on any cancer cell lines when applied at a dosage equivalent to the IC50 of BP/LPPC.
Table 1 The cytotoxicity of BP/LPPC (IC50) in different brain tumor and normal cells
LPPC protected BP against instability induced by proteins, pH and, oxygen
To verify the stability of BP in BP/LPPC for cancer therapy, nonencapsulated BP and BP/LPPC were preincubated either in H2O at 4°C (storage condition) or in a 10% FBS solution at 37°C (bioactive condition), and changes in the IC50 values of BP and BP/LPPC were measured. The results, shown in , indicated that the IC50 values of freshly prepared BP and BP/LPPC in both cell lines were 55 µg/mL and 12 µg/mL, respectively. For BP preincubated in H2O at 4°C for 4 hours, the IC50 values dramatically increased (). A similar increase in IC50 was also observed for BP preincubated in 10% FBS solution at 37°C (). However, regardless of preincubation conditions, the IC50 value of BP/LPPC was similar to that of a freshly prepared BP/LPPC ().
Figure 2 LPPC protected BP against the loss of its antitumor activity in protein-rich, oxygenic, and acid/alkaline solutions.
Notes: (A) IC50 of BP and BP/LPPC in DBTRG-05MG and RG2 cells. BP and BP/LPPC were preincubated in (B) H2O or (C) 10% FBS solution at 37°C, after which both GBM cell lines were treated with the preincubated BP or BP/LPPC. As the preincubation time increased, the IC50 values for BP and BP/LPPC were determined. *P<0.05, compared with DBTRG-BP in H2O; #P<0.05, compared with RG2-BP in H2O. BP and BP/LPPC were also preincubated in (D) H2O or (E) 10% FBS solutions ranging from pH 6 to 8 at 37°C, after which both GBM cell lines were treated with the preincubated BP or BP/LPPC. The IC50 values of BP and BP/LPPC were measured by MTT. BP and BP/LPPC were preincubated in (F) H2O with oxygen or (G) 10% FBS solution with oxygen for 24 hours, and their IC50 values in DBTRG-05MG cells were determined. *P<0.05, compared with nonencapsulated BP. (H) The cytotoxic activity of BP/LPPC in storage.
Abbreviations: BP, n-butylidenephthalide; FBS, fetal bovine serum; GBM, glioblastoma multiforme; IC50, 50% inhibitory concentration; LPPC, lipo-PEG-PEI complex.
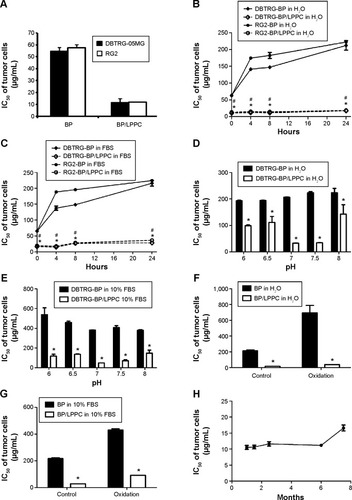
To determine whether LPPC protects BP from damage in acidic or alkaline solution, the IC50 values of BP and BP/LPPC in both solutions following preincubation in buffers of different pH values were measured. When BP was preincubated in solutions at pH 6 and 8, its IC50 in DBTRG-05MG cells significantly increased (). However, following incubation in buffers with these same pH values, BP encapsulated in LPPC retained the same IC50 values at pH 7 and 7.5 (). When BP was exposed to oxygenated buffers, its IC50 was increased to 700 mg/mL. However, LPPC can protect BP against oxidation and maintain the cytotoxicity of BP for up to 9 months, which may be useful for cancer therapy ().
The delivery efficiency of BP/LPPC
To observe changes in delivery efficiency, the cellular accumulation of nonencapsulated BP and BP/LPPC was determined by confocal microscopy. DBTRG-05MG cells were treated with equal concentrations of nonencapsulated BP and BP/LPPC, and the accumulation of BP in the cytosol was measured over time. The results showed that cytosolic drug was undetectable in the cells that were incubated with nonencapsulated BP for 30 minutes (). However, BP/LPPC could rapidly accumulate in the cytosol during a 15-minute incubation (). Furthermore, after determining the amount of BP in the cells, BP/LPPC treatment caused the accumulation of 13 µg more BP in cells within 15 minutes than in cells treated with nonencapsulated BP alone, and BP/LPPC treatment continued to gradually increase cellular BP levels over longer times (). In addition, demonstrates that LPPC transported BP to lysosomes via endocytosis. Substantial transport of BP/LPPC to cytoplasm and lysosomes was observed after only a 15-minute incubation. Subsequently, BP drug was released abundantly in the cytoplasm after a 60-minute incubation. These results provided evidence of the rapid internalization of LPPC and the release of drug from LPPCs within lysosomes at later time points. From these results, one can infer that LPPC enhances the delivery of BP into cells, thereby increasing the cytotoxic efficacy of the drug.
Figure 3 The uptake of BP/LPPC complex in DBTRG-05MG cells.
Notes: (A) BP or (B) BP/LPPC (50 µg/mL) was added to DBTRG-05MG cells and incubated for 15 minutes, 30 minutes, 60 minutes, and 120 minutes. Cell fluorescence was analyzed by confocal microscopy at 400×. PI red fluorescence: nuclei; blue fluorescence: BP drug; DiO green fluorescence: cell membrane. (C) The amount of BP in the cells. After treatment with BP, the cells were harvested and lysed. BP was extracted and measured by a fluorescence spectrophotometer. (D) Colocalization of BP/LPPC. BP/LPPC was added to DBTRG-05MG cells and incubated for 15 minutes and 60 minutes. Cell fluorescence was analyzed by confocal microscopy at 400×. DiO green fluorescence: DiO-labeled LPPC; blue fluorescence: BP drug; LysoTracker red fluorescence: lysosome.
Abbreviations: BP, n-butylidenephthalide; LPPC, lipo-PEG-PEI complex; PI, propidium iodide.
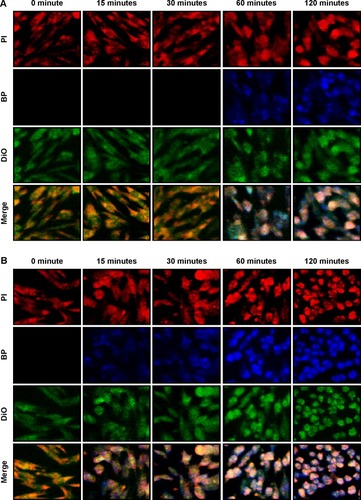
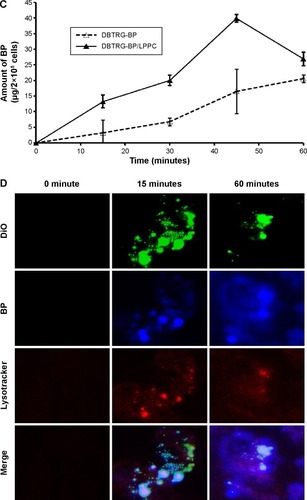
In vivo suppression of tumor growth by BP/LPPC
Mice bearing DBTRG-05MG tumors were treated with BP (100 mg/kg), empty LPPC, or BP/LPPC (containing 100 mg/kg BP) once every 2 days by IV injection. After 14 days, the animals treated with BP/LPPC showed a significant suppression of DBTRG-05MG tumor growth compared with the untreated controls, the nonencapsulated BP-treated animals, and the empty LPPC-treated animals (). This result was validated using a different treatment: intratumoral injection of BP. After 14 days of treatment, significant suppression of tumor cell growth by BP/LPPC was again observed (). In these experiments, both treatments with BP/LPPC (IV and intratumoral injection) inhibited DBTRG-05MG tumor growth bŷ85%–88%. These results show that BP/LPPC was more effective than nonencapsulated BP in inhibiting GBM tumor growth. Additionally, histological analysis showed that GBM tissues treated with BP/LPPC in vivo displayed increased caspase-3 activity and tumor cell apoptosis ().
Figure 4 The effects of BP/LPPC on tumor growth in vivo.
Notes: (A) Nude mice bearing DBTRG-05MG tumors (n=10 per group) were treated with BP, LPPC, or BP/LPPC (100 mg/kg BP) by intravenous injection once every 2 days after their tumor masses reached 50 mm3. (B) Tumor-bearing animals were treated with BP (100 mg BP/kg), BP/LPPC, or vehicle by intratumoral injection every 2 days after tumor implantation for 3 days (n=10 per group). Tumor volume was measured every 2 days after treatment. Significant differences after treatment were found for the BP/LPPC group compared with the BP-treated group (*P<0.05, **P<0.01). (C) Cleaved caspase-3 expression in DBTRG-05MG tumors after BP/LPPC treatment. The sections of tumor masses were measured by immunohistochemistry staining of cleaved caspase-3.
Abbreviations: BP, n-butylidenephthalide; PBS, phosphate-buffered saline; LPPC, lipo-PEG-PEI complex.
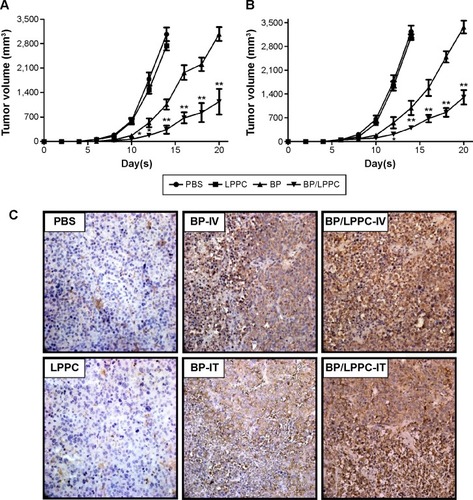
Discussion
In this study, we first used a liposome-based nanoparticle complex (LPPC) to encapsulate BP to overcome its problems of unstable structure and water insolubility. The dimerization of BP under natural conditions through cycloaddition leads to oxidation or ring cleavage of the BP monomer and interferes with BP’s antitumor activity.Citation17 In oxygenic solution, BP changes its formation and loses its function.Citation18 Additionally, after BP was incubated in a 10% FBS solution, which disrupted its antitumor function via the presence of serum proteins, the cytotoxicity of BP was dramatically decreased (). Serum proteins might interact with BP and destroy its structure. These results – that BP loses its cytotoxic activity in the presence of serum – might be similar to camptothecin. Serum proteins such as albumin bind to camptothecin and interact with its lactone, carboxylate, and self-aggregated forms, leading to lower cytotoxic activity.Citation19–Citation21 Various nanoparticles have been developed to encapsulate camptothecin to decrease its interaction with serum albumin, which enhances the stability of the drug.Citation22–Citation24 In our results, LPPC-encapsulated BP retained its cytotoxic activity even when incubated in a protein-rich, oxygenic, or acid/alkaline solution (). Collectively, the results showed that LPPC encapsulation protects BP against serum protein interactions, conformational changes, and oxidation. Therefore, LPPC encapsulation dramatically raised the cytotoxic activity of BP and enhanced its stability in the presence of serum.
LPPC provides an advanced encapsulation method that was able to efficiently deliver BP into tumor cells. When compared with nonencapsulated BP, BP/LPPC not only dramatically increased the cytotoxic activity of BP from 4.5- to 8.5-fold in all tested GBM tumor cells in vitro () but also inhibited 85% of subcutaneous tumor growth in vivo (). In our previous report, it was found that LPPC encapsulation could also suppress the proliferation of drug-resistant cells and elevate the antiproliferative effect of a drug.Citation14 The increased antiproliferative effect of BP/LPPC may be due to its increased ability to rapidly penetrate and accumulate in cells (). This high level of efficient transport into cells is supported by the fact that LPPC is a good shuttle for drugs across the cell membrane. LPPC has been shown to be an excellent drug transporter, capable of delivering large quantities of encapsulated molecules across the cell membrane quickly, which would likely explain the benefits observed by BP/PC treatment in GBM cells.
LPPC-encapsulated BP significantly increased the inhibition of subcutaneous tumor growth when administered by an IV route. The mechanism of tumor growth inhibition by IV injection of BP/LPPC may involve the tumor vasculature. It has been proposed that the leakiness of tumor blood vessels may increase the access of therapeutic vehicles to a tumor mass. Usually, tumor blood vessels are structurally abnormal and have endothelial gaps that range in size from 200 nm to 2 µm.Citation25 In addition, cationic liposomes, which are promising carriers for tumorigenic targeting, have been found to selectively target angiogenic endothelial cells in tumors.Citation26 Thus, BP/LPPC, a cationic polymer liposome of ~200–280 nm, should be able to accumulate near tumor cells due to the leakiness of tumor vasculature and therefore selectively deliver BP to a tumor area. This efficient delivery is likely to result in highly selective cytotoxicity against the tumor via its angiogenic endothelial cells. In addition, drug-loaded LPPC should be able to release a drug in a tumor area in a stable and controlled manner.Citation14 LPPC, which permits efficient transmembrane transportation (), should lead to highly efficient penetration of a drug into tumor cells.
In addition, this study demonstrated that the administration of BP/LPPC via intratumoral injection also provided significant tumor growth inhibition. In clinical practice, local administration, such as intratumoral or intracranial injection, is a therapeutic method for treating brain tumors.Citation27–Citation29 This therapy is an alternative to vascular administration, as it bypasses the blood–brain barrier and delivers therapeutic agents directly into the brain.Citation30 Therefore, athymic mice were implanted with GBM tumors subcutaneous and were treated with BP/LPPC by intratumoral injection. These results demonstrated that BP accumulated in tumor areas and maximally enhanced the antitumor effects of cytotoxic chemotherapy. In this study, the improved therapeutic effect of LPPC-encapsulated BP on GBM tumors further demonstrated that LPPC is a good drug carrier for drug transport.
Conclusion
In brief, this study showed that LPPC encapsulation improves the cytotoxicity of BP against cancer cells through rapid internalization. Our findings show that LPPC is a good vector for the encapsulation of hydrophobic antitumor drugs such as BP, as it appears to increase the cytotoxicity of BP in vivo. Future studies are underway to determine the potential of LPPC encapsulation as a means to enhance selective delivery of BP in vivo using antitumor antibodies on the LPPC surface.
Acknowledgments
This work was partially supported by UST-UCSD International Center of Excellence in Advanced Bioengineering sponsored by the Ministry of Science and Technology I-RiCE Program under grant number MOST 103-2911-I-009-101. This work was also partially supported by the MOE ATU Program, Taiwan, and grant PU/CSMU-102-2 from the Chung Shan Medical University Foundation, Taiwan. Zeiss LSM 510 META confocal microscopy was performed in the Instrument Center of Chung Shan Medical University, which is supported by the National Science Council, the Ministry of Education, and Chung Shan Medical University.
Disclosure
The authors report no conflicts of interest in this work.
References
- TsaiNMChenYLLeeCCThe natural compound n-butylidenephthalide derived from Angelica sinensis inhibits malignant brain tumor growth in vitro and in vivoJ Neurochem2006991251126216987298
- HuangMHLinSZLinPCBrain tumor senescence might be mediated by downregulation of S-phase kinase-associated protein 2 via butylidenephthalide leading to decreased cell viabilityTumour Biol2014354875488424464249
- LinPCLinSZChenYLButylidenephthalide suppresses human telomerase reverse transcriptase (TERT) in human glioblastomasAnn Surg Oncol2011183514352721553143
- ChangLFLinPCHoLIOverexpression of the orphan receptor Nur77 and its translocation induced by PCH4 may inhibit malignant glioma cell growth and induce cell apoptosisJ Surg Oncol201110344245021246566
- PangCYChiuSCHarnHJZhaiWJLinSZYangHHProteomic-based identification of multiple pathways underlying n-butylidenephthalide-induced apoptosis in LNCaP human prostate cancer cellsFood Chem Toxicol20135928128823770345
- ChiuSCChenSPHuangSYInduction of apoptosis coupled to endoplasmic reticulum stress in human prostate cancer cells by n-butylidenephthalidePLoS One20127e3374222470469
- LiuPYSheuJJLinPCExpression of Nur77 induced by an n-butylidenephthalide derivative promotes apoptosis and inhibits cell growth in oral squamous cell carcinomaInvest New Drugs201230798920809206
- WeiCWLinCCYuYLn-Butylidenephthalide induced apoptosis in the A549 human lung adenocarcinoma cell line by coupled down-regulation of AP-2alpha and telomerase activityActa Pharmacol Sin2009301297130619701232
- ChenYLJianMHLinCCThe induction of orphan nuclear receptor Nur77 expression by n-butylenephthalide as pharmaceuticals on hepatocellular carcinoma cell therapyMol Pharmacol2008741046105818577687
- LinPCChenYLChiuSCOrphan nuclear receptor, Nurr-77 was a possible target gene of butylidenephthalide chemotherapy on glioblastoma multiform brain tumorJ Neurochem20081061017102618419761
- YehJCCindrova-DaviesTBelleriMThe natural compound n-butylidenephthalide derived from the volatile oil of radix Angelica sinensis inhibits angiogenesis in vitro and in vivoAngiogenesis20111418719721327473
- SawCLWuQSuZYEffects of natural phytochemicals in Angelica sinensis (Danggui) on Nrf2-mediated gene expression of phase II drug metabolizing enzymes and anti-inflammationBiopharm Drug Dispos20133430331123640758
- FuRHHranHJChuCLLipopolysaccharide-stimulated activation of murine DC2.4 cells is attenuated by n-butylidenephthalide through suppression of the NF-κB pathwayBiotechnol Lett20113390391021267764
- LinYLLiuYKTsaiNMA lipo-PEG-PEI complex for encapsulating curcumin that enhances its antitumor effects on curcumin-sensitive and curcumin-resistance cellsNanomedicine2012831832721704596
- LiuYKLinYLChenCHA unique and potent protein binding nature of liposome containing polyethylenimine and polyethylene glycol: a nondisplaceable propertyBiotechnol Bioeng20111081318132721192002
- ChenCHLinYLLiuYKLiposome-based polymer complex as a novel adjuvant: enhancement of specific antibody production and isotype switchInt J Nanomedicine2012760762122346354
- DengSChenSNYaoPSerotonergic activity-guided phytochemical investigation of the roots of Angelica sinensisJ Nat Prod20066953654116643021
- YanRKoNLLiSLTamYKLinGPharmacokinetics and metabolism of ligustilide, a major bioactive component in rhizoma chuanxiong, in the ratDrug Metab Dispos20083640040818039808
- FleuryFKudelinaINabievIInteractions of lactone, carboxylate and self-aggregated forms of camptothecin with human and bovine serum albuminsFEBS Lett19974061511569109407
- BurkeTGMiZThe structural basis of camptothecin interactions with human serum albumin: impact on drug stabilityJ Med Chem19943740468289200
- MiZBurkeTGDifferential interactions of camptothecin lactone and carboxylate forms with human blood componentsBiochemistry19943310325103368068669
- OpanasopitPYokoyamaMWatanabeMKawanoKMaitaniYOkanoTInfluence of serum and albumins from different species on stability of camptothecin-loaded micellesJ Control Release200510431332115907582
- OpanasopitPNgawhirunpatTChaidedgumjornAIncorporation of camptothecin into N-phthaloyl chitosan-g-mPEG self-assembly micellar systemEur J Pharm Biopharm20066426927616870407
- NgawhirunpatTWonglertnirantNOpanasopitPIncorporation methods for cholic acid chitosan-g-mPEG self-assembly micellar system containing camptothecinColloids Surf B Biointerfaces20097425325919695847
- HobbsSKMonskyWLYuanFRegulation of transport pathways in tumor vessels: role of tumor type and microenvironmentProc Natl Acad Sci U S A199895460746129539785
- ThurstonGMcLeanJWRizenMCationic liposomes target angiogenic endothelial cells in tumors and chronic inflammation in miceJ Clin Invest1998101140114139525983
- GeletnekyKHuesingJRommelaereJPhase I/IIa study of intratumoral/intracerebral or intravenous/intracerebral administration of parvovirus H-1 (ParvOryx) in patients with progressive primary or recurrent glioblastoma multiforme: ParvOryx01 protocolBMC Cancer2012129922436661
- FigueiredoEGFariaJWTeixeiraMJTreatment of recurrent glioblastoma with intra-arterial BCNU [1, 3-bis (2-chloroethyl)-1-nitrosourea]Arq Neuropsiquiatr20106877878221049193
- CarpentierAMetellusPUrsuRIntracerebral administration of CpG oligonucleotide for patients with recurrent glioblastoma: a phase II studyNeuro Oncol20101240140820308317
- BoboRHLaskeDWAkbasakAMorrisonPFDedrickRLOldfieldEHConvection-enhanced delivery of macromolecules in the brainProc Natl Acad Sci U S A199491207620808134351