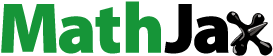
Abstract
Ultradeformable vesicles (UDV) have recently become a promising tool for the development of improved and innovative dermal and transdermal therapies. The aim of this work was to study three related UDV: transfersomes, ethosomes, and transethosomes for the incorporation of actives of distinct polarities, namely, vitamin E and caffeine, and to evaluate the effect of the carrier on skin permeation and penetration. These actives were incorporated in UDV formulations further characterized for vesicles imaging by transmission electron microscopy; mean vesicle size and polydispersity index by photon correlation spectroscopy; zeta potential by laser-Doppler anemometry; deformability by pressure-driven transport; and incorporation efficiency (IE) after actives quantification by high-performance liquid chromatography. Topical delivery studies were performed in order to compare UDV formulations regarding the release, skin permeation, and penetration profiles. All UDV formulations showed size values within the expected range, except transethosomes prepared by “transfersomal method”, for which size was smaller than 100 nm in contrast to that obtained for vesicles prepared by “ethosomal method”. Zeta potential was negative and higher for formulations containing sodium cholate. The IE was much higher for vitamin E- than caffeine-loaded UDV as expected. For flux measurements, the following order was obtained: transethosomes (TE) > ethosomes (E) ≥ transfersomes (T). This result was consistent with the release and skin penetration profiles for Vitamin E-loaded UDV. However, the releasing results were totally the opposite for caffeine-loaded UDV, which might be explained by the solubility and thermodynamic activity of this active in each formulation instead of the UDV deformability attending to the higher non-incorporated fraction of caffeine. Anyway, a high skin penetration and permeation for all caffeine-loaded UDV were obtained. Transethosomes were more deformable than ethosomes and transfersomes due to the presence of both ethanol and surfactant in their composition. All these UDV were suitable for a deeper skin penetration, especially transethosomes.
Introduction
Several strategies have been used in order to overpass the stratum corneum (SC) barrier. Among other drug delivery systems, liposomes were used for topical drug delivery.Citation1 Liposomes are typically hollow spheres surrounded by a lipid doubled layer. Once applied on skin surface, they only remain in the upper layer of the SC, acting as a drug reservoir.Citation2 Thus, due to their unstable nature and poor skin permeability, they are only suitable for topical drug delivery.Citation3
In order to overcome these limitations, novel lipid vesicles known as deformable or elastic (flexible) liposomes – ultradeformable vesicles (UDV) – were developed in the beginning of the 1990s.Citation1 As UDV are more deformable than conventional liposomes, they have demonstrated a great ability to cross the intact skin and deliver the loaded drugs into the epidermis and dermis layers or even to the systemic circulation. Phospholipids, ethanol, bile salts, and many surfactants have been used for the preparation of these elastic vesicles.Citation4
UDV present the advantages of being nontoxic and thermodynamically stable formulations.Citation5 They have been used for dermal and transdermal delivery of many molecules including peptides and proteins.Citation6 In addition, their production is relatively simple and easy to scale up.
Currently, there are many types of UDV that have been successfully developed for both pharmaceuticals and cosmeceuticals, particularly transfersomes, ethosomes, and, more recently, transethosomes ().Citation7
Figure 1 Schematic representation of ultradeformable vesicles.
Notes: (A) Transfersomes. (B) Ethosomes. (C) Transethosomes. Adapted from: Vinod KR, Kumar MS, Anbazhagan S, et al. Critical issues related to transfersomes – novel vesicular system. Acta Sci Pol Technol Aliment. 2012;11(1):67–82.Citation7
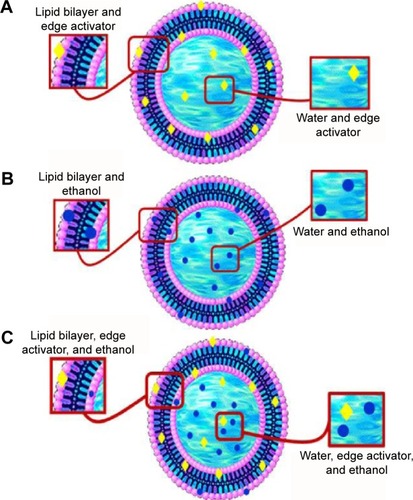
Transfersomes are elastic nanovesicles essentially made of phospholipids and edge activators (EAs) like sodium cholate (NaCo), sodium deoxycholate, Span 60, Span 65, Span 80, Tween 20, Tween 60, Tween 80, and dipotassium glycyrrhizinate.Citation8 This type of vesicle was firstly introduced in 1992 by CevcCitation9 (transfersomes, a trademark of IDEA AG, Munich, Germany), and it represents the first generation of UDV. The skin permeation and penetration of these elastic vesicles result from a synergic mechanism between the carrier properties and the permeation enhancement ability. Transfersomes can cross the skin layers by different mechanisms depending on their composition, in which these vesicles maintain their intact structure or fuse and mix with skin lipids.Citation10 They can easily change their shape and cross the skin barrier due to the EA action in response to mechanical stress by relocating inside the vesicle to zones with smaller curvature, thus reducing the membrane elastic energy to a minimal level.Citation11 Following this mechanism, transfersomes can easily squeeze through channels with one-tenth of the vesicles diameter,Citation10 and cross the SC driven by an osmotic transdermal gradient. These elastic vesicles can only penetrate through skin layers under nonocclusive conditions in order to permit the excess water evaporation from the formulation and maintain this hydration gradient. Therefore, they pass in a nondiffusive way, which means that the penetration rate will not depend on the concentration gradient.Citation12 Transfersomes also have the capability to protect the drug against rapid clearance to skin blood vessels and to promote the drug retention in the skin layers if needed.Citation13
Transfersomes have been studied as carriers for dermal or transdermal delivery for different drugs.Citation14–Citation18 However, one main disadvantage of these vesicles corresponds to the difficulty of loading hydrophobic drugs into the vesicles without compromising their deformability and elastic properties.Citation10 In general, transfersomes have been proven superior to conventional gel-state and liquid-state vesicles as well as conventional liposomes in terms of enhancement of drug permeation and interactions with the human skin.Citation19
Ethosomes are special type of UDV developed by Touitou et al in 1997.Citation20 Due to their size (approximately 150–200 nm) and high deformability, they are also referred to as elastic nanovesicles. Ethosomal systems are vesicles consisting essentially of phospholipids, water, and a high quantity of ethanol. Phospholipids can be used at 0.5%–10% concentration range, and are obtained from natural semisynthetic and synthetic sources such as soybean and egg. Examples of phospholipids include phosphatidylethanolamine, phosphatidylinositol, phosphatidylcholine, and hydrogenated phosphatidylcholine.Citation21 Ethanol can be used at 20%–45%, functioning as an efficient skin enhancer.Citation1 This molecule interacts with the polar head group of the SC lipid molecules, leading to the reduction of the melting point of the SC lipids, thus increasing the fluidity of lipid bilayers and cell membrane permeability.Citation3,Citation5
In general terms, ethosomes could be considered a type of transfersome. The difference is that ethanol evaporates from the formulation once applied on the skin under nonocclusive conditions. In the case of transfersomes, the edge active molecules, most often surfactants, remain on the skin surface after water evaporation from the formulation.
The exact mechanism of skin permeation and penetration of Ethosomes is not totally understood. The synergistic combination of phospholipids and ethanol is thought to be responsible for a deeper drug loaded distribution and penetration into the skin layers.Citation8 The elasticity of ethosomes associated to the disruption of the lipophilic SC barrier appears to be the most important contributor to its better performance for skin delivery compared to alcoholic solutions or classic liposomes.Citation7,Citation8,Citation12
Transethosomes are lipid vesicles based on transfersomes and ethosomes. These novel UDV were firstly introduced by Song et al in 2012,Citation22 and are characterized by having a high content of ethanol (up to 30%) together with an EA. Transethosomes may contain both advantages of transfersomes and ethosomes. Therefore, the mechanism of skin penetration might be a fusion of both mechanisms.Citation22
Transethosomes have shown an irregular spherical shape and higher values in both vesicle elasticity and skin permeation/penetration studies.Citation22 This fact may be due to the combination of ethanol and EA that causes a rearrangement in the lipid bilayer of these vesicles.Citation22 Amphiphilic fluorescent probes or dyes (eg, rhodamine red, 6-carboxy fluorescence, fluorescence isothiocyanate, rhodamine-123, etc) with different physicochemical properties can also be incorporated into UDV for fluorescence microscopy studies. These studies contribute to further characterize the ability of UDV to penetrate through the skin ().Citation5
Figure 2 Schematic representation of ultradeformable vesicles permeation and penetration through the skin.
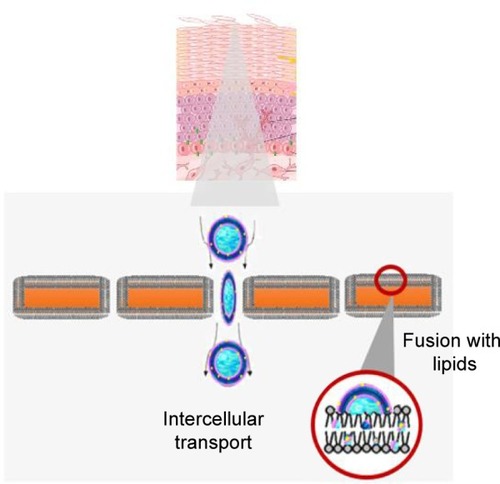
Generally, a hydrophilic molecule is expected to reside in the aqueous vesicle compartment; on the contrary, hydrophobic molecules are expected to interact with lipid membranes and incorporate in the carriers in a higher extent. In fact, most studies are limited to lipophilic actives and to the comparison between elastic and rigid vesicles.Citation2,Citation23 To the best of our knowledge, there is only one comparative study between transfersomes, ethosomes, and transethosomes.Citation22
Charge interaction between actives and vesicles are also determinant for incorporation in vesicles. In structural terms, UDV are related to liposomes. Therefore, many of the techniques used for liposome characterization can be used for UDV as well. For liposomes, playing with different combinations of lipid type, charge, mixture and concentration, ionic strength of the hydration medium, and pH will affect the efficiency of actives incorporation and immobilization in vesicle type structures. On the other hand, UDV properties are responsible for membrane flexibility, and consequently, for vesicle deform-ability necessary for through-the-skin passagework. These properties are the result of a proper defined composition which is determinant for IE. The attempt to increase actives incorporation thus might compromise vesicles’ deformability. In this study, the results of vitamin E and caffeine () incorporated in three types of UDV (transfersomes, ethosomes, and transethosomes) are reported. The effect of incorporation capacity on drug permeation in not usually discussed. Thus, the role of the carrier on active-loaded UDV permeation will be addressed in this work.
Materials and methods
Materials
Caffeine was purchased from EMD Millipore (Billerica, MA, USA). α-tocopherol, Span® 20, NaCo, and phosphotungstic acid (PTA) were purchased from Sigma-Aldrich Co. (St Louis, MO, USA). Soybean phosphatidylcholine (SPC) S 100® was purchased from Lipoid (Ludwigshafen, Germany). TAGAT CH 40® was purchased from Goldschimidt (Essen, Germany). Ultrapure water was obtained in a MILLI-Q System from EMD Millipore. All other reagents were of analytical grade.
Methods
UDV formulations
Different actives with distinct polarity (vitamin E and caffeine) were incorporated in various UDV (transfersomes, ethosomes, and transethosomes) prepared with two different surfactants: NaCo or sorbitan laurate (Span 20) according to . The controls of each formulation (empty UDV) were also prepared. UDV formulations were prepared as described below. At the end, all formulations were filtered under pressure through track-etched polycarbonate membranes in order to obtain a final and similar size of approximately 150±50 nm.
Table 1 Ultradeformable vesicle composition
Preparation of transfersomes – method A
Transfersomes were prepared by adding the active compound to the mixture of SPC, bilayer softening agent (surfactant), and purified water. This mixture was stirred for a period of at least 7 days. The formulations were always protected from light and air exposure.
Preparation of ethosomes – method B
Ethosomes were prepared according to the classical “cold method”.Citation3,Citation5 Briefly, the active compound was previously dissolved in the mixture of pure ethanol and SPC, stirred, and immersed in a water bath maintained at 30°C±2°C. After achieving the active dissolution, purified water was added drop by drop through a syringe needle inserted in the bottle cap. The formulation continued to be stirred for an additional time of 5 minutes at the same temperature, after which it was kept at room temperature for about 30 minutes.
Preparation of transethosomes
Transethosomes were prepared according to the methods A and B above described.
Characterization of UDV formulations
Visualization of vesicles by transmission electron microscopy
Transfersomes, ethosomes, and transethosomes were visualized using the electron microscope H-8100 from Hitachi Ltd. (Tokyo, Japan). All samples (10 µL) were previously diluted with 1 mL of distilled water. Then, a drop of the diluted sample was left to dry on a microscopic copper-coated grid (transmission electron microscopy grid support films of Formvar/carbon, 200 mesh Cu). After drying completely, another drop of a 1% aqueous solution of PTA was added for negative staining. Forty-five seconds later, the excess solution was wiped with filter paper. Then, the specimen was viewed under the microscope with an accelerating voltage of 75 kV and at different magnifications.
Size and zeta potential
The mean size and polydispersity index (PDI) of UDV were determined by dynamic light scattering with Zetasizer Nano-S equipment (Malvern Instruments, Malvern, UK). PDI less than 0.2 indicates a homogenous and monodisperse population. Zeta potential was determined by laser-Doppler anemometry with Zetasizer Nano-Z equipment (Malvern instruments)
For both measurements, 10 µL of transfersome, transethosome, and ethosome formulations were diluted with purified water.
Active high-performance liquid chromatography quantification
The quantification of vitamin E and caffeine was performed using a high-performance liquid chromatography (HPLC) system (Tokoy, Shimadzu, Japan) with a SPD-10A UV-VIS detector according to . This methodology was previously validated as reported in the literature.Citation24–Citation26
Table 2 Experimental conditions for HPLC quantification of the active compounds tested
SPC quantification
SPC concentration was determined by an enzymatic and colorimetric method (Phospholipids kit; Spinreact, Sant Esteve de Bas, Spain) at 505 nm and 37°C.
Determination of incorporation parameters
The initial formulation was diluted and centrifuged at 180,000× g, 15°C for 4 hours (Beckman Optima XL-90, Ultracentrifuge, GMI, Ramsey, Minnesota, USA). After this time, the supernatant was removed and the precipitate was suspended with purified water. IE, active entrapment yield, and active loading were calculated according to the following equations:
Pressure-driven transport
The flow of the vesicles was measured by pressure-driven transport. Briefly, vesicles diluted at 1% lipid final concentration were forced to pass through 30 nm pore size track-etched polycarbonate membranes under 0.6 MPa pressure, created by a nitrogen stream, during at least 5 minutes. The suspension was collected in a container on a Sartorius LA620P scale (Data Weighing Systems, Inc., Elk Grove, IL, USA). The data were collected with the Wedge software for Windows (TAL Technologies Inc., Philadelphia, PA, USA).
Viscosity measurement
The viscosity of UDV formulations was determined by using a Brookfield® Digital Viscometer (Model DV-II) with a spindle number 21 at 5 rpm (23°C±2°C). Readings were taken after 30 seconds.
Topical Delivery of UDV
Release study
Release studies have been performed using vertical Franz diffusion cells (diffusion area of 1.0 cm2) with hydrophilic polysulfone membranes (Tuffryn®; Pall Corporation, Port Washington, NY, USA) after its saturation in receptor phase (RP) for 30 minutes. The RPs were previously studied in order to ensure the sink conditions. A higher solubilization of Caffeine and vitamin E was obtained using a 0.9% (m/v) NaCl aqueous solutionCitation27 and 0.1% (m/v) Tagat® in 0.01 M saline phosphate buffer (pH 7.4), respectively. The RP was maintained at 32°C–37°C under stirring (Julabo U3 Thermostat and Multimagnetic stirrer SBS®; Labexchange, Paris, France).
About 0.5 g of each formulation was applied in the donor compartment under nonocclusive conditions. Attending to the lowest IE obtained for the hydrophilic active (caffeine)-loaded UDV, the respective solution controls were also studied. The solution composition was similar to UDV, except for the absence of lipid.
Samples of 200 µL were collected after 1, 2, 3, 4, 5, and 6 hours according to international guidelinesCitation28–Citation30 and the same volume was replaced with fresh RP kept at the same temperature. These samples were quantified by HPLC as previously described for each active. Data (n=6) was expressed as cumulative amount of active (M) permeated through the membrane filter, considering the total amount as the active dose assayed in each formulation according to EquationEquation 4(4) .
Permeation and penetration studies
In vitro skin permeation study was performed according to the method described by OECD Guideline 428Citation28 at the same conditions as described before, except for the use of fresh pig ear skin (dermatomized to 500 µm). Vitamin E- and caffeine-loaded UDV formulations as well as caffeine solution controls (500 µL) were spread over the skin (0.68 cm2) in contact with 3.5 mL of RP, and 24 hours later, the skin samples were rinsed to remove excess formulation and dried with filter paper. After the skin samples had been attached and fixed on a smooth surface, the SC was removed using 20 adhesive tapes (Scotch®; 3M, S. Paulo, Brazil). In order to improve the reproducibility of the tape stripping technique, a cylinder (2 kg) on a foam and an acrylic disk (both with an area of 5.73 cm2) were used resulting in a pressure of 349.3 g/cm2 applied for 10 seconds for each tape. All the tapes with the SC removed and the remaining skin (viable epidermis and dermis) were cut into small pieces used for the extraction process, which had been previously validated (>80% recovery). In this extraction process, 3–5 mL of methanol were added to both SC tapes and epidermis/dermis (ED) pieces. These samples were stirred for 1 minute in a vertical mixer, sonicated for 15 minutes, and centrifuged at 400× g for 5 minutes. After filtration (0.45 µm), the supernatant (50 µL) was injected on HPLC to quantify the amount of vitamin E and caffeine retained in each skin layer.
Statistical analysis
The results were reported as mean ± standard deviation of at least three measurements per formulation. The results were statistically analyzed by analysis of variance using SigmaPlot 11.0 software®. The differences were considered statistically significant when P<0.05.
Results and discussion
Characterization of UDV formulations
The macroscopic and microscopic appearances of UDV formulations (empty controls) are represented in and . It was observed that both transfersomes and ethosomes were whitish, and transethosomes were the most translucent formulation due to the presence of both ethanol and surfactant (NaCo) in the formulation composition. Regarding the transmission electron microscopy imaging, PTA was used as the negative stain for these samples since it is an electron dense or opaque reagent for electrons. In fact, it reacted well with the vesicles allowing visualizing and discerning the vesicles’ membrane and lamellae, especially in the case of transethosomes (probably due to the presence of ethanol and less turbidity). In contrast, transfersomes were quite difficult to visualize since a lower contrast was obtained. Although all vesicles appeared to have a multilamellar spherical form, other forms were also observed in some aggregates (mainly formed after the drying process). This was possible due to the high deformability of the vesicles’ membrane which permits them to adapt its form to the surrounding space. In addition, all vesicles have a diameter of 150±50 nm, with transethosomes being the smallest vesicles, as further confirmed by dynamic light scattering.
Figure 3 Macroscopic appearance of ultradeformable vesicles formulations.
Note: Transfersomes, ethosomes, and transethosomes (from left to right).
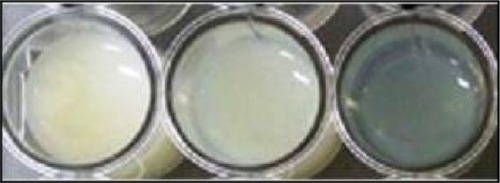
Figure 4 Microscopic appearance of ultradeformable vesicle formulations obtained by transmission electron microscopy.
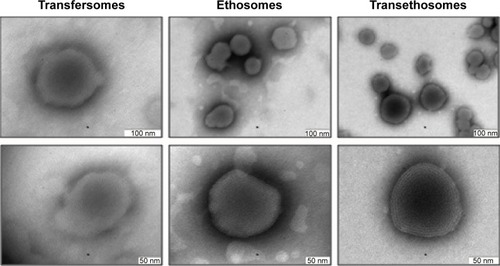
The results of the tested parameters used to characterize all UDV formulations are presented in . An easier comparability between these formulations was achieved by standardizing all procedures like the vesicles’ composition or their preparation (eg, number of filtrations, pore filter, etc) and characterization methods, including the respective formulation controls.
Table 3 Physicochemical properties of ultradeformable vesicle formulations containing vitamin E and caffeine and respective controls (results expressed as mean ± standard deviation, n=3)
Regarding the vesicles’ size, all formulations showed values within the expected range (100–200 nm), except transethosomes prepared by method A, for which diameter was smaller than 100 nm in contrast to that obtained by vesicles prepared by method B. This was probably due to differences in stirring time: while the formulations prepared by method B (“ethosomal” method) were stirred only during the addition of water, the formulations prepared by method A (“transfersomal” method) were stirred for 7 days which probably led to a more compact structural rearrangement of surfactant and ethanol molecules, with a consequent decrease of the vesicles size in the latter case. Therefore, only transetho-somes prepared by method B with a similar and comparable size with the other formulations were used for topical delivery experiments.
Concerning the PDI, a value of <0.2 (homogeneous population) was obtained for all formulations, except transfersomes with vitamin E (PDI =0.428). This value may be explained by the specific location of vitamin E in the vesicles’ membrane due to its lipophilicity, and the higher IE which contributes to increase the vesicles size and PDI especially when compared with the formulation control (empty transfersomes).
Zeta potential is an important physical parameter for predicting the vesicles’ stability.Citation31 All UDV formulations showed a negative zeta potential as expected. The formulations containing NaCo had the highest (modular) zeta potential due to the negative charge of this EA in contrast to the nonionic Span 20. Although SPC lipid has a zwitterionic behavior, it is important to consider the negative charge of the phosphate groups of SPC phospholipids which also contributed to the negative surface charge of the vesicles.Citation32 Finally, the contribution of both caffeine and vitamin E to the zeta potential was almost negligible when compared with the respective UDV control.
The IE as well as active entrapment yield and active loading were much higher for vitamin E- than for caffeine-loaded UDV as expected according to the respective Log P (8.84 versus −0.55, respectively). The IE was low (<10%) and similar for caffeine-loaded transfersomes, transethosomes (prepared by either method A or B with both surfactants), and ethosomes (prepared by adding this active in either ethanol or aqueous phase [data not shown]). In this last case, the active entrapment yield remained around 3% because most of aqueous phase remains outside the vesicles. The hydrophilicity of caffeine may reduce the encapsulation efficiency to the inner aqueous compartment of UDV in contrast to the lipophilic vitamin E which is easily incorporated in the vesicles’ membrane resulting in a higher IE (>77%). This result is consistent with the values obtained by Pham et alCitation33 for caffeine-loaded liposomes and niosomes formulations.
SPC yield was high (>65%) for all UDV formulations proving that there was minimal vesicle loss during the centrifugation step. Thus, this parameter did not influenced the IE obtained.
Viscosity is an important parameter to further characterize the physical behavior of the developed formulations, especially regarding the topical application. All formulations showed a similar viscosity being higher in the presence of ethanol. Ethanol molecules contain both hydrophobic and hydrophilic groups involving two hydrogen bonds, which leads to a network differently structured than water. In the case of ethanolic aqueous solutions, alcohol molecules firstly fill the voids of the hydrogen-bond network of the water structure and “fortify” the structure due to the hydrogen bonds between the hydroxyl oxygen of alcohol and water.Citation34 This explains the increase of the viscosity of formulations containing ethanol. Although UDV formulations were suitable for topical application, other dosage forms could be considered depending on the application site. For example, eyebrows and neck require especial care when applying liquid formulations. The modification of the final formulation rheology could be achieved by the inclusion of UDV suspension in a gel. This approach is commonly found in the literature.Citation13,Citation35
The pressure-driven transport of UDV formulations is represented in and .
Figure 5 Flux of the different formulations after pressure filtration expressed as mean ± standard deviation (g/s).
Notes: A and B at the end of the legend refer to the method employed for the vesicles preparation, method A or method B, respectively. For each formulation, the flux was performed in triplicate (n=3). Statistical analysis: One Way Anova with All Pairwise Multiple Comparison Procedures; means with different letters (a,b,c) and different numbers are significantly different (P<0.05). The letters are also numerated according to each group.
Abbreviations: E caf, caffeine-loaded ethosomes; empty E, empty ethosomes; empty TE NaCo, empty sodium cholate transethosomes; empty TE span, empty Span 20 transethosomes; empty T Naco, empty sodium cholate transfersomes; empty T span, empty Span 20 transfersomes; E vitE, vitamin E-loaded ethosomes; T caf NaCo, caffeine-loaded sodium cholate transfersomes; T caf span, caffeine-loaded Span 20 transfersomes; TE caf NaCo, caffeine-loaded sodium cholate transethosomes; TE caf span, caffeine-loaded Span 20 transethosomes; TE vitE NaCo, vitamin E-loaded sodium cholate transethosomes; T vitE NaCo, vitamin E-loaded sodium cholate transfersomes.
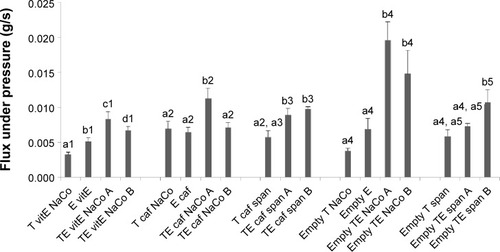
Considering all the results, the following order for UDV flux was obtained: transethosomes (TE) > ethosomes (E) ≥ transfersomes (T) for both vitamin E and caffeine formulations. The difference between transethosomes and the other UDV was statistically different (P<0.05) for all tested formulations, especially the controls (empty UDV) prepared with NaCo. This is easily explained by the presence of both ethanol and surfactant in transethosomes composition, acting synergically on the flexibility of the membranes and thus on the vesicles’ deformability. The same order was obtained in other previous experiments for elasticity index determination (data not shown).
Topical delivery
The release profiles of vitamin E UDV formulations are shown in .
Figure 6 Cumulative amount of vitamin E ultradeformable vesicle formulation released over 6 hours, expressed as mean (absolute value and %) ± standard deviation.
Notes: Data were collected from six samples (n=6 per formulation) at each hour using Franz cells. Statistical analysis: two-way analysis of variance with duplicates and all pairwise multiple comparison procedures; means with different letters (a,b,c) are significantly different (P<0.05).
Abbreviations: E vitE, vitamin E-loaded ethosomes; TE vitE NaCo, vitamin E-loaded sodium cholate transethosomes; T vitE NaCo, vitamin E-loaded sodium cholate transfersomes.

The release profile was consistent with the flux data, as theoretically expected: TE > E > T with significant differences (P<0.05), especially for transethosomes and ethosomes. Transethosomes are the most deformable vesicles, easily changing their conformation and allowing a higher membrane passage. In addition, a sustained and controlled release as already reported for UDV, mainly transfersomes, was also observed.Citation9,Citation36 The passage of vesicles and active to the RP was confirmed by dynamic light scattering and HPLC, respectively. Although it is not usual to analyze the donor compartment, the active was assayed in both donor and receptor compartments at each hour considering the nonocclusive conditions necessary for this study. However, the active amount did not increase along time in donor phase in contrast to RP because the evaporation rate was lower than the release rate.
The release profiles of caffeine UDV formulations (prepared with NaCo) are represented in . In contrast to vitamin E, the release profiles of caffeine UDV formulations were much higher, and showed the following order: T > E > TE with significant differences (P<0.05). The same order was observed for caffeine UDV formulations prepared with Span 20 (data not shown) and caffeine solution controls but with lower differences between them in the latter case. Comparing these solution controls with the respective UDV formulations, the release was significantly higher for the controls except transfersome control solution, for which release was statistically similar to caffeine-loaded transfersomes. The similar order observed was due to the low IE of caffeine in all tested UDV. Therefore, the results obtained might be more related with caffeine solubility and thermodynamic activity in each UDV formulation rather than UDV deformability. In fact, the solubility of caffeine in water and in ethanol is 0.105 M and 0.029 M, respectively.
Figure 7 (A) Cumulative amount of caffeine UDV formulation (prepared with sodium cholate) released over 6 hours, expressed as mean ± SD. (n=6 per formulation). (B) Cumulative amount of caffeine UDV formulation (prepared with sodium cholate) released over 6 hours relative to the applied amount, expressed as mean ± SD (n=6 per formulation). (C) Cumulative amount of Caffeine solution controls released over 6 hours, expressed as mean ± SD (n=3 per control). (D) Cumulative amount of caffeine solution controls released over 6 hours relative to the applied amount, expressed as mean ± SD (n=3 per control).
Notes: Statistical analysis: two-way analysis of variance with duplicates and all pairwise multiple comparison procedures; means with different letters (a,b and c) are significantly different (P<0.05).
Abbreviations: E caf, caffeine-loaded ethosomes; Solution Control E, control solution for ethosomes; Solution Control T, control solution for transfersomes; Solution Control TE, control solution for transethosomes; SD, standard deviation; T caf NaCo, caffeine-loaded sodium cholate transfersomes; TE caf NaCo, caffeine-loaded sodium cholate transethosomes; UDV, ultradeformable vesicles.
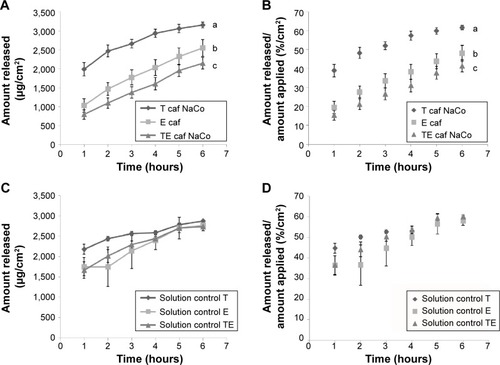
Permeation and penetration studies
Although vitamin E was detected in RP after 24 hours (permeation study) for all three UDV formulations, its concentration (0.085–0.117 µg/mL) was neglected since it was lower than the limit of quantification (1 µg/mL).
The results of the penetration study are represented in .
Figure 8 Retention of vitamin E ultradeformable vesicle formulations in SC and ED after 24 hours, expressed as mean (µg/cm2 or %/cm2) ± standard deviation (n=3 per formulation).
Notes: Statistical analysis: one-way analysis of variance with all pairwise multiple comparison procedures (Holm–Sidak method); means with different letters are significantly different (P<0.05).
Abbreviations: ED, epidermis plus dermis; E vitE, vitamin E-loaded ethosomes; SC, stratum corneum; TE vitE NaCo, vitamin E-loaded sodium cholate transethosomes; T vitE NaCo, vitamin E-loaded sodium cholate transfersomes.
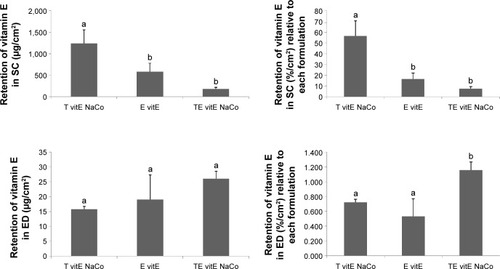
The following order of vitamin E-loaded UDV retained in SC was obtained: T > E ≥ TE, and the opposite order was observed for ED retention (TE > T ≥ E) expressed as %/cm2. The retention profile order of vitamin E-loaded UDV formulations at deeper skin layers was in accordance with the respective release profile and vesicles’ deformability before. This may be attributed to the fusion of these vesicles with skin lipids and the active release along the penetration pathway, resulting in a depot effect. In particular, this effect could be higher in the presence of ethanolCitation22,Citation23,Citation37–Citation39 which increases the skin permeability by intercalation into the polar head group of skin lipids. The synergistic mechanism between ethanol, surfactant, vesicles, and skin lipids is responsible for a higher skin penetration of transethosomes. In addition, the deeper penetration of vitamin E could also be associated to the higher vitamin E IE in UDV containing ethanol.
Lipophilic molecules such as vitamin E, highly incorporated in lipid carriers, are usually used for topical delivery studies. In this study, we have also used caffeine, a hydrophilic molecule that is not expected to be incorporated in liposome-like structures to a great extent, to evaluate if the non-incorporated active fraction (present in a higher percentage than the incorporated fraction) would be more released and accumulated in deeper skin layers than the respective control solution. Regarding the formulations with caffeine (), all tested UDV formulations (including the respective solution controls) permeated through the skin after 24 hours. The smaller molecular size and log P of caffeine contributed to a higher skin permeation compared to vitamin E correspondent to the non-incorporated fraction of UDV formulations.
Figure 9 Retention of caffeine UDV formulations, and the respective solutions controls in SC and ED and skin permeation after 24 hours, expressed as mean (µg/cm2 or %/cm2) ± standard deviation (n=3 per formulation).
Notes: Statistical analysis: one-way analysis of variance with all pairwise multiple comparison procedures (Holm–Sidak method) between each group (UDV versus respective controls and transfersomes versus ethosomes versus transethosomes); means with different letters are significantly different (P<0.05).
Abbreviations: E caf, caffeine-loaded ethosomes; ED, epidermis plus dermis; SC, stratum corneum; T caf NaCo, caffeine-loaded sodium cholate transfersomes; TE caf NaCo, caffeine-loaded sodium cholate transethosomes; UDV, ultradeformable vesicles.
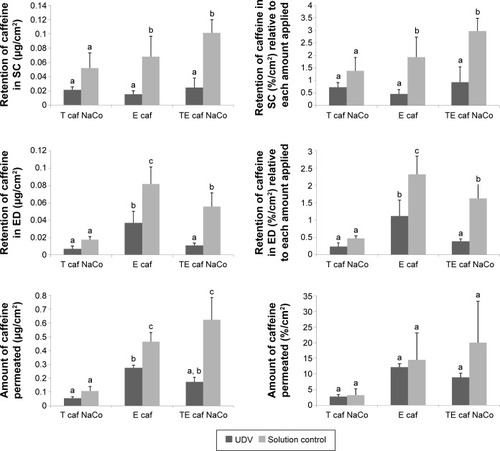
It was initially hypothesized that UDV formulations might smooth the progress of the non-incorporated active fraction through the skin along with its penetration. However, it was observed that the solution controls contributed to both higher skin retention and permeation profiles of caffeine than the respective UDV formulations possibly due to its low IE and, on the other hand, to the lower viscosity, lipids absence, and probably higher caffeine dissolution of solution formulations. Nevertheless, no statistical differences were found for caffeine-loaded UDV containing ethanol and caffeine-loaded transfersomes versus solution controls for skin permeation (%/cm2) and all skin experiments, respectively. There were also no significant differences between UDV formulations, except for caffeine-loaded ethosomes whose ED retention and permeation profile was higher.
Conclusion
This study aimed to compare the characteristics of different UDV and their influence on skin delivery of caffeine and vitamin E. The release profile is dependent on the actives’ chemical properties and their IE in UDV formulations. A sustained release will be more easily obtained for higher incorporated molecules, such as vitamin E. Even with low IE, it was also possible to achieve a good skin retention and penetration of caffeine-loaded UDV in comparison to solution controls.
Considering the results obtained here, transethosomes are the liposome-like vesicles with more advantages for deeper skin penetration as measured by deformability and permeation experiments. In addition, this vesicular formulation is quite easy to scale up, which constitutes a great advantage for industrial purposes.
Here we proved that all these UDV are useful as skin delivery systems by acting as drug carriers as well as permeation enhancers, and are capable of retaining the active molecules accessible within different skin layers, under certain conditions. These data suggest that the formulation of actives in UDV may be performed in a case-by-case manner.
Author contributions
All authors contributed toward data analysis, drafting and revising the paper and agree to be accountable for all aspects of the work.
Acknowledgments
All authors acknowledge Carla Euletério and Isabel Nogueira for technical support and TEM imaging, respectively. All authors also acknowledge Laboratório Edol – Produtos Farmacêuticos, SA and Sopalmeira, Lda. for financial support.
Supplementary material
Table S1 Physical, chemical, and biologic characteristics of vitamin E and caffeine
References
- HenningASchaeferUFNeumannDPotential pitfalls in skin permeation experiments: influence of experimental factors and subsequent data evaluationEur J Pharm Biopharm200972232433118761087
- Compound Summary for CID 2519: Caffeine [webpage on the Internet] PubChem OpenBethesdaNational Center for Biotechnology Information2013 Available from: https://pubchem.ncbi.nlm.nih.gov/compound/caffeineAccessed May 20, 2014
- NikiETraberMGA history of vitamin EAnn Nutr Metab201261320721223183290
- DrugBank: Caffeine [webapage on the Internet]DrugBank2014 Available from: http://www.drugbank.ca/drugs/DB00201Accessed May 27, 2014
- DrugBank: Vitamin E [webapage on the Internet]DrugBank2014 Available from: http://www.drugbank.ca/drugs/DB00163Accessed May 27, 2014
- ThieleJJTraberMGPackerLDepletion of human stratum corneum vitamin E: an early and sensitive in vivo marker of UV induced photo-oxidationJ Invest Dermatol199811057567619579541
- PotardGLaugelCBailletASchaeferHMartyJPQuantitative HPLC analysis of sunscreens and caffeine during in vitro percutaneous penetration studiesInt J Pharm199918924926010536254
- NikiERole of vitamin E as a lipid-soluble peroxyl radical scavenger: in vitro and in vivo evidenceFree Radic Biol Med20146631223557727
- RozmanBGasperlinMTinois-TessoneaudEPirotFFalsonFSimultaneous absorption of vitamins C and E from topical microemulsions using reconstructed human epidermis as a skin modelEur J Pharm Biopharm2009721697518977295
- Brigelius-FlohéRTraberMGVitamin E: function and metabolismFASEB J199913101145115510385606
- ChemSpider: Caffeine [webpage on the Internet]LondonRoyal Society of Chemistry2014 Available from: http://www.chemspider.com/Chemical-Structure.2424.html?rid=565899e4-2f92-4124-81ef-b317db92ec38Accessed May 20, 2014
- KhazaeliPPardakhtyAShoorabiHCaffeine-loaded niosomes: characterization and in vitro release studiesDrug Deliv20071444745217994362
- ThieleJJEkanayake-MudiyanselageSVitamin E in human skin: organ-specific physiology and considerations for its use in dermatologyMol Aspects Med2007285–664666717719081
- LinJYSelimMASheaCRUV photoprotection by combination topical antioxidants vitamin C and vitamin EJ Am Acad Dermatol200348686687412789176
- NeuzilJDongLFRamanathapuramLVitamin E analogues as a novel group of mitocans: anti-cancer agents that act by targeting mitochondriaMol Aspects Med2007285–660764517499351
- ValiAAsilianAKhalesiEKhoddamiLShahtalebiMMohammadyMEvaluation of the efficacy of topical caffeine in the treatment of psoriasis vulgarisJ Dermatolog Treat200516423423716249145
- SilvaNDrumondIAlmeidaIFTopical caffeine delivery using biocellulose membranes: a potential innovative system for cellulite treatmentCellulose2014211665674
Disclosure
The authors report no conflicts of interest in this work.
References
- ManosroAJantrawutPKhositsuntiwongNManosroiWManosroiJNovel Elastic Nanovesicles for Cosmeceutical and Pharmaceutical ApplicationsChiang Mai J Sci2009362168178
- VanićŽHafnerABegoMŠkalko-BasnetNCharacterization of various deformable liposomes with metronidazoleDrug Dev Ind Pharm201339348148822455377
- TouitouEDayanNBergelsonLGodinBEliazMEthosomes – novel vesicular carriers for enhanced delivery: characterization and skin penetration propertiesJ Control Release200065340341810699298
- JainSTiwaryAKSapraBJainNKFormulation and evaluation of ethosomes for transdermal delivery of lamivudineAAPS Pharm-SciTech200784E111
- NandureHPPuranikPGiramPLoneVEthosome: A Novel Drug CarrierInternational Journal of Pharmaceutical Research and Allied Sciences2013231830
- SimõesSIDelgadoTCLopesRMDevelopments in the rat adjuvant arthritis model and its use in therapeutic evaluation of novel non-invasive treatment by SOD in TransfersomesJ Control Release2005103241943415763624
- VinodKRKumarMSAnbazhaganSCritical issues related to transfersomes – novel vesicular systemActa Sci Pol Technol Aliment2012111678222230977
- RomeroELMorillaMJHighly deformable and highly fluid vesicles as potential drug delivery systems: theoretical and practical considerationsInt J Nanomedicine201383171318623986634
- CevcGTransfersomes, liposomes and other lipid suspensions on the skin: permeation enhancement, vesicle penetration, and transdermal drug deliveryCrit Rev Ther Drug Carrier Syst1996133–42573889016383
- ChenJLuWLGuWLuSSChenZPCaiBCSkin permeation behavior of elastic liposomes: role of formulation ingredientsExpert Opin Drug Deliv201310684585623550630
- ElsayedMMCevcGThe vesicle-to-micelle transformation of phospholipidcholate mixed aggregates: a state of the art analysis including membrane curvature effectsBiochim Biophys Acta20111808114015320832388
- CevcGBlumeGSchätzleinAGebauerDPaulAThe skin: a pathway for systemic treatment with patches and lipid-based agent carriersAdv Drug Deliv Rev1996183349378
- CevcGVierlUMazgareanuSFunctional characterisation of novel analgesic product based on self-regulating drug carriersInt J Pharm20083601–2182818524509
- NikiETraberMGA history of vitamin EAnn Nutr Metab201261320721223183290
- NikiERole of vitamin E as a lipid-soluble peroxyl radical scavenger: in vitro and in vivo evidenceFree Radic Biol Med20146631223557727
- RozmanBGasperlinMTinois-TessoneaudEPirotFFalsonFSimultaneous absorption of vitamins C and E from topical microemulsions using reconstructed human epidermis as a skin modelEur J Pharm Biopharm2009721697518977295
- JovanovicSVJankovicIJosimovicLElectron-transfer reactions of alkylperoxy radicalsJ Am Chem Soc19921142390189021
- Brigelius-FlohéRTraberMGVitamin E: function and metabolismFASEB J199913101145115510385606
- Dragicevic-CuricNGräfeSGitterBWinterSFahrASurface charged temoporfin-loaded flexible vesicles: in vitro skin penetration studies and stabilityInt J Pharm20103841–210010819819321
- TouitouEAlkabesMDayanNEliazMEthosomes: novel vesicular carriers for enhanced skin deliveryPharm Res199714305306
- CevcGVierlUNanotechnology and the transdermal route: A state of the art review and critical appraisalJ Control Release20101413277299 Available from: http://www.sciencedirect.com/science/article/pii/S016836599900222919850095
- SongCKBalakrishnanPShimCKChungSJChongSKimDDA novel vesicular carrier, transethosome, for enhanced skin delivery of voriconazole: characterization and in vitro/in vivo evaluationColloids Surf B Biointerfaces20129229930422205066
- BragagniMMenniniNMaestrelliFCirriMMuraPComparative study of liposomes, transfersomes and ethosomes as carriers for improving topical delivery of celecoxibDrug Deliv201219735436123043648
- LinJYSelimMASheaCRUV photoprotection by combination topical antioxidants vitamin C and vitamin EJ Am Acad Dermatol200348686687412789176
- PauloMGMarquesHMMoraisJAAlmeidaAJAn isocratic LC method for the simultaneous determination of vitamins A, C, E and beta-caroteneJ Pharm Biomed Anal199921239940610703996
- ChowdhurySRMalequeMShihanMHDevelopment and Validation of a Simple RP-HPLC Method for Determination of Caffeine in Pharmaceutical Dosage FormsAsian J Pharm Ana20122114
- NicoliSColomboPSantiPRelease and permeation kinetics of caffeine from bioadhesive transdermal filmsAAPS J200571E218E22316146342
- CevcGRational design of new product candidates: The next generation of highly deformable bilayer vesicles for noninvasive, targeted therapyJ Control Release2012160213514622266051
- FDAGuidance for Industry, In Vitro Release Testing and In Vivo Available from: http://www.fda.gov/downloads/Drugs/.../Guidances/UCM070930.pdfAccessed April 5, 2014
- Pharmacopeial Forum (PF) [webpage on the Internet]RockvilleUSP2014 Available from: http://www.usp.org/usp-nf/pharmacopeial-forumAccessed April 5, 2014
- DominguesMMSantiagoPSCastanhoMASantosNCWhat can light scattering spectroscopy do for membrane-active peptide studies?J Pept Sci200814439440018189339
- AscensoACruzMEuletérioCNovel tretinoin formulations: a drug-in-cyclodextrin-in-liposome approachJ Liposome Res201323321121923631723
- PhamTTJaafar-MaalejCCharcossetCFessiHLiposome and niosome preparation using a membrane contactor for scale-upColloids Surf B Biointerfaces201294152122326648
- LebretteSPagnouxCAbélardPStability of aqueous TiO2 suspensions: influence of ethanolJ Colloid Interface Sci2004280240040815533413
- AhadAAqilMKohliKSultanaYMujeebMNano vesicular lipid carriers of angiotensin II receptor blocker: Anti-hypertensive and skin toxicity study in focusArtif Cells Nanomed Biotechnol201522416 Epub ahead of print
- AscensoASalgadoAEuletérioCIn vitro and in vivo topical delivery studies of tretinoin-loaded ultradeformable vesiclesEur J Pharm Biopharm2014881485524854884
- GoindiSDhattBKaurAEthosomes-based topical delivery system of antihistaminic drug for treatment of skin allergiesJ Microencapsul201431771672424963956
- AscensoAPinhoSEleutérioCLycopene from tomatoes: vesicular nanocarrier formulations for dermal deliveryJ Agric Food Chem201361307284729323826819
- ScognamiglioIDe StefanoDCampaniVNanocarriers for topical administration of resveratrol: a comparative studyInt J Pharm201344017918722909994