Abstract
Background
Since cancer cells are normally over-expressed cathepsin B, we synthesized dendrimer-methoxy poly(ethylene glycol) (MPEG)-doxorubicin (DOX) conjugates using a cathepsin B-cleavable peptide for anticancer drug targeting.
Methods
Gly-Phe-Leu-Gly peptide was conjugated with the carboxylic acid end groups of a dendrimer, which was then conjugated with MPEG amine and doxorubicin by aid of carbodiimide chemistry (abbreviated as DendGDP). Dendrimer-MPEG-DOX conjugates without Gly-Phe-Leu-Gly peptide linkage was also synthesized for comparison (DendDP). Nanoparticles were then prepared using a dialysis procedure.
Results
The synthesized DendGDP was confirmed with 1H nuclear magnetic resonance spectroscopy. The DendDP and DendGDP nanoparticles had a small particle size of less than 200 nm and had a spherical morphology. DendGDP had cathepsin B-sensitive drug release properties while DendDP did not show cathepsin B sensitivity. Further, DendGDP had improved anticancer activity when compared with doxorubicin or DendDP in an in vivo CT26 tumor xenograft model, ie, the volume of the CT26 tumor xenograft was significantly inhibited when compared with xenografts treated with doxorubicin or DendDP nanoparticles. The DendGDP nanoparticles were found to be relatively concentrated in the tumor tissue and revealed stronger fluorescence intensity than at other body sites while doxorubicin and DendDP nanoparticles showed strong fluorescence intensity in the various organs, indicating that DendGDP has cathepsin B sensitivity.
Conclusion
DendGDP is sensitive to cathepsin B in tumor cells and can be used as a cathepsin B-responsive drug targeting strategy. We suggest that DendGDP is a promising vehicle for cancer cell targeting.
Introduction
Drug targeting to a specific site of action is still a major problem in the field of bio-medicine and drug delivery.Citation1,Citation2 Because most drugs diffuse freely throughout the body, conventional drug formulations induce a variety of side effects in the human body and lose their intrinsic therapeutic effects. The serious adverse effects of anticancer drugs in the clinical setting are often mentioned in the literature.Citation3–Citation5 Site-specific targeting of anticancer drugs to tumor tissues remain a major challenge in cancer chemotherapy.Citation5–Citation7 To overcome these drawbacks, nanomaterials, nanoparticles, polymer-drug conjugates, and nanodevices have been extensively investigated in the last 2 decades.Citation8–Citation12 Nanomaterials and devices based on polymeric conjugates have been widely investigated in an effort to facilitate drug trafficking efficiently in tumor tissues. Enhanced tumor targeting by nanoparticles is based on the enhanced permeation and retention (EPR) effect in tumor tissues.Citation10 Then, nanoparticles are selectively targeted at the tumor tissues through physicochemical and biological pathway.Citation10 Compared with conventional drug carriers, nanomaterials such as nanotubes, polymeric micelles, dendrimers, liposomes, and nanoparticles have several advantages, including a large surface area, ease of chemical modification, a small diameter for site-specific targeting of anticancer drugs, solubilization of lipophilic drugs, and physicochemical functionality.Citation8–Citation16 For example, their large surface area and small particle size enable conjugation of cancer cell-targeting ligands, such as monoclonal antibodies, folic acid, and RGD peptides.Citation15–Citation18
Among the nanomaterials, dendrimers have been extensively investigated in the biomedical field.Citation19–Citation23 Dendrimers are a class of macromolecular hyperbranched polymers having an intrinsic structure composed of a core, branches, void spaces, and terminal groups.Citation19 Due to their structural peculiarity, dendrimers have better physicochemical behavior than linear or branched polymers.Citation21,Citation22 For example, dendrimers tend to have lower viscosity, unique molecular behavior, and a lower hydrated radius when compared with linear polymers.Citation21,Citation22 Due to these peculiarity, dendrimer is able to manipulate at mild condition and it can be endowed with multifunctionality.Citation19 In particular, dendrimers is are suitable vehicles for anticancer drugs and a targeting moiety because they have multivalent functional groups on their terminal groups. This characteristic enables dendrimers to conjugate with various molecules, such as anticancer drugs and poly(ethylene glycol) (PEG), and a targeting moiety and peptide can be simultaneously conjugated to the dendrimer.Citation20,Citation23,Citation24 In a report by He et al an RGD peptide for targeting cancer cells, PEG for avoidance of the reticuloendothelial system, and an anticancer drug were simultaneously introduced to a poly(amidoamine) (PAMAM) dendrimer.Citation24 These authors showed that a multifunctional dendrimer efficiently targeted U87MG cells via a receptor-mediated pathway and could inhibit cell viability. Further, Kaminskas et al reported that PEGylated dendrimer-methotrexate conjugates having matrix metalloproteinase-2/9 cleavable peptide are efficiently targeted to tumor cells, suppressed growth of cancer cells, and circulated in the blood stream for a longer period.Citation25
In this study, we synthesized doxorubicin-conjugated dendrimer nanoparticles using glycylphenylalanyl-leucylglycine tetrapeptide (Gly-Phe-Leu-Gly, GFLG) for cathepsin B-responsive drug targeting of cancer cells. Cathepsin B, which is a lysosomal cysteine protease, is known to degrade extracellular matrix components during invasion and metastasis of cancer cells.Citation26 To determine if this conjugate could be considered a promising vehicle for cancer-targeting drug carriers, we carried out a physicochemical/biological analysis in vitro and tested this conjugate in an animal tumor xenograft model using CT26 colorectal carcinoma cells.
Materials and methods
Materials
The dendrimer (NTN1956) was purchased from Frontier Scientific Inc (USA). GFLG tetrapeptide was purchased from Peptron Co (Korea). Methoxy PEG (MPEG) amine (molecular weight 2,000 g/mol) was obtained from Sunbio Chem Co (Korea). Doxorubicin HCl was purchased from LC Laboratories (USA). Cathepsin B (from bovine spleen), dimethyl sulfoxide (DMSO), triethylamine, 3-(4,5-dimethylthiazol-2-yl)-2,5-diphenyl tetrazolium bromide (MTT), N-(3-dimethylaminopropyl)-N-ethylcarbodiimide hydrochloride (EDAC), and N-hydroxysuccinimide (NHS) were sourced from Sigma Chemicals Co (USA). Spectra/Por™ dialysis membranes (molecular weight cut-off 2,000 and 8,000 g/mol) were purchased from Spectrum Laboratories Inc (USA). Roswell Park Memorial Institute (RPMI) 1640 medium and fetal serum bovine albumin were purchased from Gibco (Invitrogen Co, USA). All other chemicals used were high-performance liquid chromatography or extra pure grade.
Synthesis of dendrimer nanoparticles
Dendrimer-peptide conjugates
First, 13.4 mg of dendrimer was dissolved in DMSO with 12 equivalents of EDAC and NHS and magnetically stirred for 6 hours. Twelve equivalents of tetrapeptide were then added to this reaction and stirred for a further 48 hours. To remove the unreacted chemicals and byproducts, the reactants were introduced into a dialysis membrane (molecular weight cut-off 2,000 g/mol) and dialyzed against deionized water for 2 days, with exchange of water at 2–3-hour intervals. The dialyzed solution was then lyophilized for 3 days, after which a white powder (dendrimer-peptide conjugates) was obtained. The final yield of conjugates was approximately 91% (w/w).
Dendrimer-peptide-MPEG conjugates
Sixty milligrams of the dendrimer-peptide conjugate in DMSO were mixed with 4 equivalents of EDAC/NHS and stirred for 6 hours. Eight milligrams of MPEG-amine was then added to this reaction and stirred for a further 48 hours. To remove the unreacted MPEG-amine, the reactants were introduced into a dialysis membrane (molecular weight cut-off 8,000 g/mol) and dialyzed against deionized water for 2 days, with exchange of water at 2–3-hour intervals. The dialyzed solution was lyophilized for 3 days, after which a white powder was obtained, with a product yield of approximately 93% (w/w).
Dendrimer-peptide-MPEG-DOX conjugates (DendGDP)
First, 140 mg of dendrimer-peptide-MPEG in DMSO was mixed with 8 equivalents of EDAC/NHS and stirred for 3 hours. Eight equivalents of doxorubicin HCL with trace amounts of triethylamine were then added to this reaction, with magnetic stirring for 48 hours. The reactants were then dialyzed against water using a dialysis membrane (molecular weight cut-off 8,000 g/mol) and dialyzed against deionized water for a further 2 days, with exchange of water at 3 hour intervals.
Dendrimer-MPEG-DOX conjugates (DendDP)
First, 13.4 mg of dendrimer in DMSO was mixed with 4 equivalents of EDAC/NHS and magnetically stirred for 6 hours. Eighty milligrams of MPEG-amine was then added to this reaction, with stirring for another 48 hours. To remove the unreacted MPEG-amine, the reactants were introduced into a dialysis membrane (molecular weight cutoff 8,000 g/mol) and dialyzed against deionized water for 2 days following lyophilization for 3 days (the product yield of dendrimer-MPEG conjugates was approximately 92% [w/w]). Next, 93 mg of the dendrimer-MPEG conjugates in DMSO were mixed with 8 equivalents of doxorubicin HCL and trace amounts of triethylamine. This reaction was then magnetically stirred for 48 hours, followed by dialysis against deionized water for 2 days, and then solution was lyophilized for 3 days.
Analysis
1H nuclear magnetic resonance (NMR) spectroscopy (using a 500 MHz FT-NMR spectrometer, Varian Inc, USA) was used to analyze the dendrimer and its related conjugates.
Preparation and characterization of nanoparticles
Fifty milligrams of DendGDP or DendDP dissolved in 4 mL of DMSO was slowly dropped into 10 mL of deionized water. This solution was dialyzed (dialysis membrane molecular weight cut-off 8,000 g/mol) against water for 2 days to remove the organic solvent, with exchange of water at 2–3-hour intervals. The morphology of the nanoparticles was observed using a transmission electron microscope (H-7600, Hitachi Instruments Ltd, Japan). The size of the dendrimer nanoparticles was analyzed using a Nano-ZS (Malvern Instruments, UK).
Drug release study
Five milligrams of the lyophilized nanoparticles were reconstituted in 1 mL of deionized water and then diluted with 4 mL of sodium acetate buffer (pH 5.4) with or without cathepsin B (50 U). The nanoparticle solution was introduced into a dialysis membrane (molecular weight cut-off 2,000 g/mol). The dialysis membrane was then introduced into a 50 mL Falcon tube with 40 mL of buffer solution. The drug release study was performed in a shaking incubator (at 100 rpm and 37°C). At predetermined time intervals, 1 ml of media was taken to measure concentration of the released drug and then the whole media were replaced with fresh media to prevent drug saturation. The doxorubicin concentration released was analyzed using an UV-1801 spectrophotometer (Shimadzu Co, Japan) at 479 nm.
In vitro cell culture study
For the in vitro cell culture study, CT26 mouse colorectal carcinoma cells were obtained from the Korean Cell Line Bank and maintained in RPMI 1640 medium supplemented with 10% (v/v) fetal bovine serum and antibiotics in a 5% CO2 incubator at 37°C.
Doxorubicin-resistant CT26 cells were prepared as reported previously.Citation18 CT26 cells (1×105) in serum-free RPMI medium were exposed to doxorubicin for 3 hours, after which the medium was replaced with fresh RPMI 1640 medium supplemented with fetal bovine serum/antibiotics. Exposure to doxorubicin was repeated 2–3 times at 3-day intervals for 3 months, with the doxorubicin concentration gradually being increased from 0.001 µg/mL to 1.0 µg/mL.
The in vitro cell viability study was performed as follows. CT26 cells (3×104) were seeded into 96-well plates, cultured overnight, and then treated with doxorubicin, DendDP, or DenGDP. For the doxorubicin group, doxorubicin HCl in DMSO was diluted with RPMI 1640 medium (final DMSO concentration less than 0.5%) and added to the 96-well plate containing the cultured CT26 cells. For the DendDP and DendGDP groups, the nanoparticles were reconstituted in deionized water and then diluted with RPMI 1640 medium for treatment of CT26 cells. The cells used for each treatment group were incubated for a further 24 hours in a CO2 incubator. MTT (30 µL, 5 mg/mL in PBS [phosphate-buffered saline]) was then added to the 96-well plates, with incubation for a further 4 hours. Finally, 0.1 mL of DMSO was added to dissolve the formazan crystals generated in viable cells. Cell viability was then evaluated by measurement of absorbance (560 nm test/630 nm reference) using a microplate reader (Molecular Devices Inc, USA).
In vivo fluorescence imaging
To study the biodistribution of the dendrimer nanoparticles, doxorubicin or nanoparticles were injected intravenously via the tail vein into each mouse at a dose of 10 mg/kg as a DOX concentration. Injection volume was 100 µl. One and 2 days later, the mice were observed using a Maestro™ 2 small animal imaging system (Cambridge Research and Instrumentation Inc, USA). The mice were sacrificed 48 hours after injection of the study formulations to observe the distribution of the nanoparticles in the organs.
In vivo antitumor activity study
The antitumor activity of doxorubicin and the nanoparticles was also evaluated an in vivo tumor xenograft model using male nude BALB/c mice (aged 4–5 weeks, weight 20 g). CT26 cells (1×104) in PBS (pH 7.4, 0.01 mM) were subcutaneously injected in the dorsum of each mouse. Ten days later, the animals were divided into four groups, ie, control, doxorubicin, DendDP, and DendGDP, with each treatment group containing 4–5 mice. PBS (control), doxorubicin, or nanoparticles (containing a doxorubicin dose equivalent to 5 mg/kg) were injected intravenously via the tail vein. The injection volume was 0.1 mL. The day of the first injection was designated day 0; 3 days later, a second intravenous dose of doxorubicin or nanoparticles was injected. Tumor growth was monitored at 3–5-day intervals using Vernier calipers. The tumor volume was calculated using the following equation: (length × widthCitation2)/2. All animal experiments were carried out following the guidelines approved by the Committee for the Care and Use of Laboratory Animals at Chonnam National University.
Statistical analysis
All results are expressed as the mean ± standard deviation. The statistical analysis was performed using the Student’s t-test and results were considered to be statistically significant when the P-value was less than 0.05.
Results
Synthesis and characterization of conjugates
The cathepsin B-cleavable peptide, GFLG, was conjugated to the carboxylic acid group of a dendrimer molecule, and MPEG was then attached to the end of peptide (). The 12 carboxylic acid moiety of the dendrimer () was then activated with 12 equivalents of EDAC/NHS. The NHS-activated end group of the dendrimer was then conjugated with 13 equivalents of GFLG peptide ( and ). Next, the unreacted GFLG was removed by dialysis, and the lyophilized solid was then used for further conjugation. The degree of conjugation of GFLG to the dendrimer was estimated with a dimethyl group at 0.8 ppm of GFLG peptide and an ethyl proton of the dendrimer at 1.6–2.3 ppm. The degree of substitution of the GFLG peptide was approximately 10.8 for one dendrimer molecule. The carboxylic acid end group of GFLG was then activated again with EDAC and NHS, and conjugated with 4 equivalents of MPEG-amine (). Because specific peaks of the ethyl protons of PEG overlapped at 3.5–4.0 ppm with the dendrimer molecule, the conjugation yield of MPEG was estimated by measurement of the weight of the final product. From this measurement, the conjugation yield of MPEG was approximately 3.78 for one dendrimer-GFLG conjugate. Doxorubicin was conjugated to the remaining carboxylic end groups of the GFLG peptide. The doxorubicin content in the DendGDP conjugates was approximately 17.6% (w/w) ().
Table 1 Characterization of dendrimer nanoparticles
Figure 1 Synthesis scheme for DendGDP.
Abbreviations: DendGDP, dendrimer-MPEG-DOX conjugate with GFLG peptide linkage; DendDP, dendrimer-MPEG-DOX conjugate; DOX, doxorubicin; MPEG, methoxy poly(ethylene glycol); EDAC, N-(3-dimethylaminopropyl)-N-ethylcarbodiimide hydrochloride; GFLG, Gly-Phe-Leu-Gly; TEA, triethylamine; NHS, N-hydroxysuccinimide.
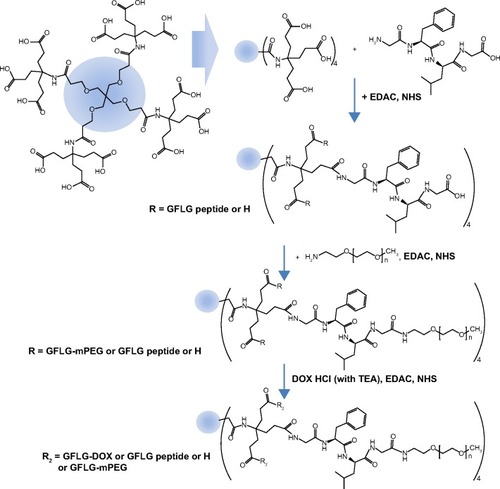
A DendDP conjugate () was also synthesized for comparison with the DendGDP conjugate. The carboxylic acid group of the dendrimer molecule was activated with EDAC/NHS, and 4 equivalents of MPEG-amine were then attached to the NHS-activated dendrimer (data not shown). The conjugation yield of MPEG was approximately 3.83 for one dendrimer molecule. Doxorubicin was conjugated to the remaining carboxylic end groups of the dendrimer-MPEG conjugate. The doxorubicin content in the DendDP conjugate was approximately 24.8% (w/w).
Preparation and characterization of nanoparticles
The DendDP and DendGDP nanoparticles were prepared using a dialysis procedure. The DendDP and DendGDP nanoparticles had a particle size less than 200 nm (). The DendGDP nanoparticles were slightly bigger than the DendDP nanoparticles. Further, the DendDP and DendGDP nanoparticles had a spherical shape, with a small size in the range of approximately 50–100 nm (). The DendDP and DendGDP nanoparticles also had a core-shell structure, ie, doxorubicin and/or the GFLG peptide-conjugated dendrimer formed the core of each nanoparticle while PEG formed its outer shell (). The particle size on transmission electron microscopy was slightly smaller than that obtained by actual measurement of particle size. These differences might be due to the fact that nanoparticles must be shrank by the time of TEM observation while they were hydrated in the aqueous solution.
Figure 2 (A) Particle size distribution and (B) transmission electron micrograph of DendDP and DendGDP nanoparticles.
Abbreviations: DendGDP, dendrimer-MPEG-DOX conjugate with GFLG peptide linkage; DendDP, dendrimer-MPEG-DOX conjugate; DOX, doxorubicin; MPEG, methoxy poly(ethylene glycol); GFLG, Gly-Phe-Leu-Gly.
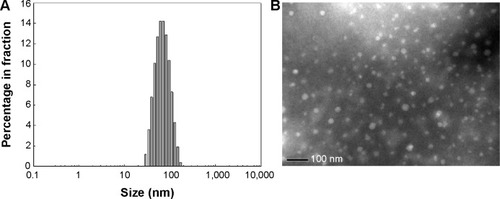
To study the cathepsin B enzyme-mediated drug release properties of the nanoparticles, DendGDP was incubated at 37°C in the presence or absence of cathepsin B, followed by measurement of fluorescence intensity (). Since doxorubicin itself has fluorescence properties, the changes in fluorescence intensity arising from the nanoparticles were measured at 520–700 nm. As shown in , the fluorescence intensity increased gradually with increasing cathepsin B activity. Near-infrared fluorescence images () also indicated an increase in fluorescence intensity. These results suggest that doxorubicin was released via biodegradation of the GFLG peptide upon exposure to cathepsin B, and then fluorescence intensity was increased. Further, the rate of release of doxorubicin was significantly increased in response to treatment with cathepsin B (). Otherwise, DendDP showed a small increase in the rate of release of doxorubicin upon addition of cathepsin B. These results might reflect the fact that the peptide bond formed between doxorubicin and the dendrimer molecule is not specifically degraded by cathepsin B. Otherwise, the rate of release of doxorubicin was significantly increased by degradation of the GFLG peptide in the DendGDP nanoparticles.
Figure 3 Enzyme-sensitive drug release from DendDP and DendGDP nanoparticles.
Notes: Fluorescence intensity spectra (A) and near-infrared images (B) of DendGDP nanoparticles in the presence of CaB. Three milligrams of nanoparticles were incubated with CaB for 3 hours. (C) Release of DOX from the nanoparticles in the absence or presence of CaB (50 U).
Abbreviations: au, absorbance units; CaB, cathepsin B; DendGDP, dendrimer-MPEG-DOX conjugate with GFLG peptide linkage; DendDP, dendrimer-MPEG-DOX conjugate; DOX, doxorubicin; MPEG, methoxy poly(ethylene glycol); GFLG, Gly-Phe-Leu-Gly.
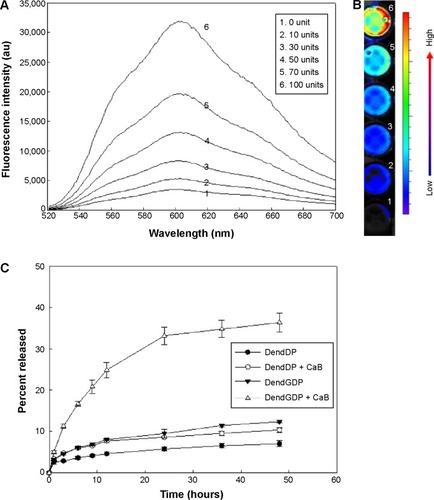
In vitro cell culture study
CT26 colon carcinoma cells were treated with doxorubicin, DendDP, or DendGDP nanoparticles to investigate their anticancer activity. As shown in , growth of CT26 cells was dose-dependently inhibited in response to treatment with doxorubicin. CT26 cells treated with DendDP showed a relatively lower potential for growth inhibition () even though observation of fluorescence intensity indicated higher uptake efficiency by tumor cells when compared with uptake of doxorubicin alone (). A reason for this observation might be the delayed release of doxorubicin from the DendDP nanoparticles (), such that cell viability was not significantly changed. As shown in , release of doxorubicin from the DendDP nanoparticles was slightly increased when cathepsin B was added, and non-specific release of the drug could have contributed to inhibition of cell growth. Otherwise, growth of CT26 cells in response to treatment with DendGDP was significantly inhibited to a greater extent than with doxorubicin alone or with the DendDP nanoparticles (). Further, the fluorescence intensity in cells treated with DendGDP was also higher than that in cells treated with doxorubicin or DendDP (), indicating that the GFLG peptide underwent enzymatic degradation and doxorubicin was released rapidly from the DendGDP nanoparticles, thereby affecting cell growth. Cathepsin B zymography was performed to confirm cathepsin B activity in CT26 cells, and a specific band was confirmed at 20–35 kDa (data not shown), indicating that these cells expressed cathepsin B activity.
Figure 4 Anticancer activity in CT26 colorectal carcinoma cells.
Notes: (A) Cell growth. (B) Fluorescence intensity in CT26 cells. ***P<0.001
Abbreviations: DendGDP, dendrimer-MPEG-DOX conjugate with GFLG peptide linkage; DendDP, dendrimer-MPEG-DOX conjugate; DOX, doxorubicin; MPEG, methoxy poly(ethylene glycol); GFLG, Gly-Phe-Leu-Gly; DAPI, 4,6-diamidino-2-phenylindole.

In vivo antitumor activity study
To study the ability of DendGDP nanoparticles to target tumor tissue, CT26 cells were implanted on the backs of nude mice, and the solid tumors that developed were observed 2 weeks later. Doxorubicin, DendDP nanoparticles, or DendGDP nanoparticles were injected via the tail vein, and the biodistribution of each compound was observed by near-infrared fluorescence imaging. The near-infrared images () showed that the fluorescence intensity in the tumor xenograft was relatively stronger in response to injection of DendDP or DendGDP nanoparticles than after injection of doxorubicin alone. In particular, injection of doxorubicin resulted in weak fluorescence intensity in the solid tumor tissue while strong fluorescence intensity was observed in other organs. Further, the biodistribution study showed that doxorubicin was predominantly distributed to the kidneys, liver, and brain rather than to the solid tumor tissue. Otherwise, the DendDP and DendGDP nanoparticles showed relatively higher fluorescence intensity at the solid tumor site when compared with the organs. Interestingly, the injected DendGDP nanoparticles showed strong fluorescence intensity at the tumor site as early as 48 hours post injection, while the injected DendDP nanoparticles showed fluorescence intensity at both the solid tumor site and other body sites. Further, in terms of organ distribution, the injected DendGDP nanoparticles showed significantly higher fluorescence intensity at the tumor site than in the organs. Even though the DendDP nanoparticles also showed strong fluorescence intensity at the tumor site, they were also distributed to a considerable extent in the liver, kidneys, and lungs. These results indicate that DendGDP nanoparticles have specificity for CT26 solid tumors and tumor-targeting properties.
Figure 5 Anticancer activity of dendrimer nanoparticles.
Notes: (A) Anticancer activity of dendrimer nanoparticles and (B) near-infrared fluorescence images for CT26-bearing male nude BALB/c mice. DOX itself or DOX-conjugated dendrimer nanoparticles(5 mg/kg) were injected into the tail vein of each mouse. The major organs were removed from each mouse 48 hours after injection (Dotted circle: solid tumor). The data shown as the mean ± standard deviation (n=4). ***P<0.01; **P<0.001
Abbreviations: DendGDP, dendrimer-MPEG-DOX conjugate with GFLG peptide linkage; DendDP, dendrimer-MPEG-DOX conjugate; DOX, doxorubicin; MPEG, methoxy poly(ethylene glycol); GFLG, Gly-Phe-Leu-Gly.
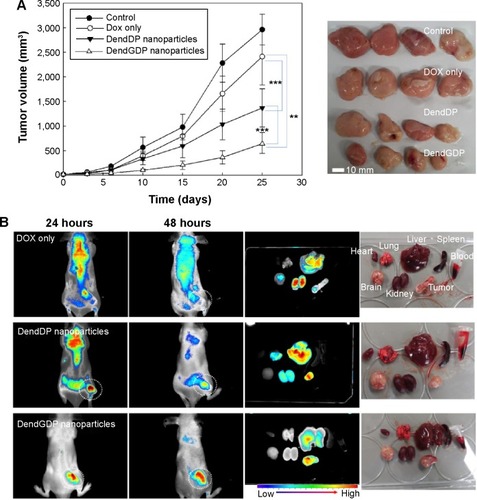
Discussion
Since dendrimers have unique properties, including a hyperbranched structure, they have been extensively investigated as drug-targeting vehicles when conjugated with bioactive agents.Citation19–Citation23 Various molecules, including an anticancer drug, a targeting moiety, a peptide, and PEG can be simultaneously attached in the terminal group of a dendrimer molecule. Especially, dendrimer itself having various biomolecules has nanostructural properties in aqueous solution. For example, a MPEG-conjugated dendrimer has a core-shell structure, ie, PEG can form an outer shell on the conjugate and a drug-conjugated dendrimer can form the inner core of the nanoparticle, as shown in . Furthermore, a targeting moiety can also be decorated on the corona of the structure. Jain and Jain have reported on an arginine-conjugated poly(propylene) imine that was conjugated with endostatin and doxorubicin for dual attack on cancer, ie, inhibition of angiogenesis can be achieved by endostatin release and killing of cancer cells can be achieved by doxorubicin.Citation20 Further, Yang et al synthesized PAMAM conjugated with alkali blue for targeting of the lymphatics.Citation23 They showed that this alkali blue-conjugated PAMAM dendrimer enhanced lymphatic drainage and had a longer residence time in the lymph nodes when compared with methylene blue solution. He et al reported that a RGD peptide-conjugated PAMAM dendrimer can be used as a targeting vehicle for cancer cells overexpressing αvβ3 integrin and that doxorubicin was entrapped in the hydrophobic dendritic core of the aggregates.Citation24 These authors confirmed that this RGD peptide-conjugated dendrimer targeted cancer cells through specific interaction with αvβ3 integrin.
We synthesized a PEG and DOX-conjugated dendrimer using a GFLG spacer to target cancer cells via cathepsin B-mediated drug release. Since cathepsin B is specifically excreted by cancer cells during invasion or metastasis, a cathepsin B-sensitive peptide, ie, GFLG, can be used for cancer cell targeting through conjugation of anticancer drugs.Citation26 DendGDP nanoparticles showed cathepsin B-mediated drug release and tumor targeting, while DendDP, a non-cleavable conjugate, was not sensitive with cathepsin B ( and ). As shown in , treatment with DendGDP showed strong fluorescence intensity at tumor sites in mice, with minimal fluorescence intensity at other body sites. However, fluorescence intensity of DenDP treatment group was relatively stronger at liver tissues than tumor tissues (). Duncan et al reported that DOX-N-(2-hydroxypropyl)-methacrylamide conjugates using cathepsin B-cleavable peptide linkage showed approximately 6-fold accumulation in tumor tissue when compared with free doxorubicin.Citation27 Chen et al also reported that a cell-penetrating doxorubicin-peptide conjugate using GFLG showed cathepsin B-mediated drug release properties and an ability to target cancer cells.Citation28 Furthermore, sorbitol linked with a cathepsin B-cleavable peptide and doxorubicin showed cathepsin B-mediated drug release kinetics and cancer cell specificity.Citation29 Poly(L-lysine)-PEG copolymers with near-infrared fluorescence probes can be used to detect orthotopically implanted colon cancer model of CT26 cells.Citation30 Our results also showed that DendGDP became relatively concentrated in CT26 solid tumors when compared with DendDP or doxorubicin alone (). DendGDP has the highest fluorescence intensity in the tumor tissue rather than in the body organs, while doxorubicin alone and DendDP showed strong fluorescence in the organs, indicating that DendGDP has potential for use as a diagnostic tool for cancer. Adenis et al reported that expression of cathepsin B was significantly elevated in malignant colorectal carcinoma when compared with normal adjacent colorectal tissue.Citation31 They also suggested that cathepsin B expression can be used to monitor progression of colorectal cancer. Members of the cathepsin family are known to have a strong relationship with invasion and metastasis of colorectal cancer and are used for prognostication.Citation32 Furthermore, Funovics et al showed that levels of cathepsin B gradually increased from healthy colon to adenoma to adenocarcinoma in a CT26 cell-implanted mouse model of colorectal carcinoma.Citation33
In conclusion, we synthesized a dendrimer-DOX conjugate via a cathepsin B-cleavable peptide. This nanoconjugate had a small particle size. DendGDP had cathepsin B-sensitive drug release properties, whereas the drug release kinetics of DendDP were not significantly changed. Further, DendGDP had improved anticancer activity when compared with doxorubicin or DendDP in an vivo mouse CT26 tumor xenograft model. DendGDP became relatively concentrated in the tumor tissue and had stronger fluorescence intensity compared with doxorubicin alone or DendDP nanoparticles. We suggest that DendGDP can be used as a promising vehicle for cancer cell targeting.
Acknowledgments
This study was supported by a grant (2015–25) from the Biomedical Research Institute, Pusan National University Hospital.
Supplementary materials
Figure S1 1H nuclear magnetic resonance spectra for the dendrimer molecule.
Note: Peaks of a and b was indicated methylene protons of dendrimer core.
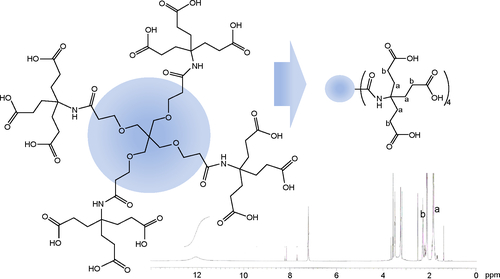
Figure S3 1H nuclear magnetic resonance spectra for dendrimer-GFLG conjugate.
Note: Peaks of a and b was indicated methylene protons of dendrimer core.
Abbreviations: GFLG, Gly-Phe-Leu-Gly; EDAC, N-(3-dimethylaminopropyl)-N-ethylcarbodiimide hydrochloride; NHS, N-hydroxysuccinimide.
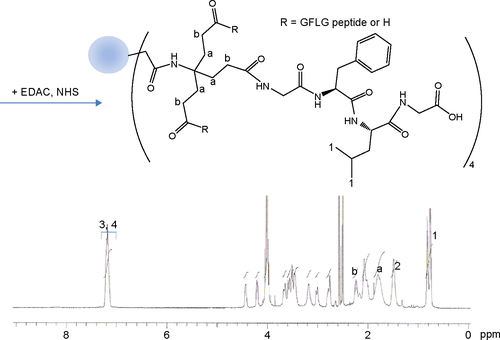
Figure S4 1H nuclear magnetic resonance spectra for the dendrimer-GFLG-mPEG conjugate.
Note: Peaks of a and b was indicated methylene protons of dendrimer core.
Abbreviations: GFLG, Gly-Phe-Leu-Gly; EDAC, N-(3-dimethylaminopropyl)-N-ethylcarbodiimide hydrochloride; NHS, N-hydroxysuccinimide; PEG, poly(ethylene glycol); MPEG, methoxy poly(ethylene glycol).

Disclosure
The authors report no conflicts of interest in this work.
References
- MitragotriSBurkePALangerROvercoming the challenges in administering biopharmaceuticals: formulation and delivery strategiesNat Rev Drug Discov20141365567225103255
- McLornanDPListAMuftiGJApplying synthetic lethality for the selective targeting of cancerN Engl J Med20143711725173525354106
- RousseauAMarquetPApplication of pharmacokinetic modelling to the routine therapeutic drug monitoring of anticancer drugsFundam Clin Pharmacol20021625326212570013
- PaciAVealGBardinCReview of therapeutic drug monitoring of anticancer drugs. Part 1. CytotoxicsEur J Cancer2014502010201924889915
- WidmerNBardinCChatelutEReview of therapeutic drug monitoring of anticancer drugs. Part two – targeted therapiesEur J Cancer2014502020203624928190
- MarchettiCPalaiaIGiorginiMTargeted drug delivery via folate receptors in recurrent ovarian cancer: a reviewOnco Targets Ther201471223123625031539
- McCruddenCMMcCarthyHOCurrent status of gene therapy for breast cancer: progress and challengesAppl Clin Genet2014720922025419154
- RyanSMBraydenDJProgress in the delivery of nanoparticle constructs: towards clinical translationCurr Opin Pharmacol201418C12012825450066
- KajimotoKSatoYNakamuraTYamadaYHarashimaHMultifunctional envelope-type nano device for controlled intracellular trafficking and selective targeting in vivoJ Control Release201419059360624794902
- NicholsJWBaeYHEPR: evidence and fallacyJ Control Release201419045146424794900
- ChoHYLeeYBNano-sized drug delivery systems for lymphatic deliveryJ Nanosci Nanotechnol20141486888024730304
- OrzaACascianoDBirisANanomaterials for targeted drug delivery to cancer stem cellsDrug Metab Rev20144619120624697156
- HubbellJAChikotiANanomaterials for drug deliveryScience201233730330522822138
- Kapse-MistrySGovenderTSrivastavaRYergeriMNanodrug delivery in reversing multidrug resistance in cancer cellsFront Pharmacol2014515925071577
- BegSRizwanMSheikhAMHasnainMSAnwerKKohliKAdvancement in carbon nanotubes: basics, biomedical applications and toxicityJ Pharm Pharmacol20116314116321235578
- CabralHKataokaKProgress of drug-loaded polymeric micelles into clinical studiesJ Control Release201419046547624993430
- ZhaoYLinDWuFDiscovery and in vivo evaluation of novel RGD-modified lipid-polymer hybrid nanoparticles for targeted drug deliveryInt J Mol Sci201415175651757625268623
- JeongYIKimYWJungSDelivery of transferrin-conjugated polysaccharide nanoparticles in 9L gliosarcoma cellsJ Nanosci Nanotechnol201515125129
- AbbasiEAvalSFAkbarzadehADendrimers: synthesis, applications, and propertiesNanoscale Res Lett2014924724994950
- JainKJainNKSurface engineered dendrimers as antiangiogenic agent and carrier for anticancer drug: dual attack on cancerJ Nanosci Nanotechnol2014145075508724757983
- LyulinSVVattulainenIGurtovenkoAAComplexes comprised of charged dendrimers, linear polyelectrolytes, and counterions: insight through coarse-grained molecular dynamics simulationsMacromolecules20084149614968
- EleftheriouEKaratasosKModeling the formation of ordered nano-assemblies comprised by dendrimers and linear polyelectrolytes: the role of Coulombic interactionsJ Chem Phys201213714490523061863
- YangRXiaSYeTSynthesis of a novel polyamidoamine dendrimer conjugating with alkali blue as a lymphatic tracer and study on the lymphatic targeting in vivoDrug Deliv11192014 Epub ahead of print
- HeXAlvesCSOliveiraNRGD peptide-modified multifunctional dendrimer platform for drug encapsulation and targeted inhibition of cancer cellsColloids Surf B Biointerfaces2015125828925437067
- KaminskasLMKellyBDMcLeodVMCapping methotrexate α-carboxyl groups enhances systemic exposure and retains the cytotoxicity of drug conjugated PEGylated polylysine dendrimersMol Pharm2011833834921171585
- BervarAZajcISeverNKatunumaNSloaneBFLahTTInvasiveness of transformed human breast epithelial cell lines is related to cathepsin B and inhibited by cysteine proteinase inhibitorsBiol Chem200338444745512715895
- DuncanRSat-KlopschYNBurgerAMBibbyMCFiebigHHSausvilleEAValidation of tumour models for use in anticancer nanomedicine evaluation: the EPR effect and cathepsin B-mediated drug release rateCancer Chemother Pharmacol20137241742723797686
- ChenZZhangPCheethamAGControlled release of free doxorubicin from peptide-drug conjugates by drug loadingJ Control Release201419112313024892976
- ManigandaSSankarVNairJBRaghuKGMaitiKKA lysosome-targeted drug delivery system based on sorbitol backbone towards efficient cancer therapyOrg Biomol Chem2014126564656925062087
- AlencarHFunovicsMAFigueiredoJSawayaHWeisslederRMahmoodUColonic adenocarcinomas: near-infrared microcatheter imaging of smart probes for early detection – study in miceRadiology200724423223817507718
- AdenisAHuetGZerimechFHecquetBBalduyckMPeyratJPCathepsin B, L, and D activities in colorectal carcinomas: relationship with clinico-pathological parametersCancer Lett1995962672757585467
- KuesterDLippertHRoessnerAKruegerSThe cathepsin family and their role in colorectal cancerPathol Res Pract200820449150018573619
- FunovicsMAAlencarHMontetXWeisslederRMahmoodUSimultaneous fluorescence imaging of protease expression and vascularity during murine colonoscopy for colonic lesion characterizationGastrointest Endosc20066458959716996355