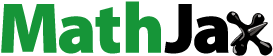
Abstract
Positively surface-charged poly(lactide-co-glycolide) (PLGA)/Eudragit RL nanoparticles (NPs) were designed to increase retention time and sustain release profile in joints after intra-articular injection, by forming micrometer-sized electrostatic aggregates with hyaluronic acid, an endogenous anionic polysaccharide found in high amounts in synovial fluid. The cationic NPs consisting of PLGA, Eudragit RL, and polyvinyl alcohol were fabricated by solvent evaporation technique. The NPs were 170.1 nm in size, with a zeta potential of 21.3 mV in phosphate-buffered saline. Hyperspectral imaging (CytoViva®) revealed the formation of the micrometer-sized filamentous aggregates upon admixing, due to electrostatic interaction between NPs and the polysaccharides. NPs loaded with a fluorescent probe (1,1′-dioctadecyl-3,3,3′,3′ tetramethylindotricarbocyanine iodide, DiR) displayed a significantly improved retention time in the knee joint, with over 50% preservation of the fluorescent signal 28 days after injection. When DiR solution was injected intra-articularly, the fluorescence levels rapidly decreased to 30% of the initial concentration within 3 days in mice. From these findings, we suggest that PLGA-based cationic NPs could be a promising tool for prolonged delivery of therapeutic agents in joints selectively.
Introduction
Intra-articular (IA) administration of therapeutic agents has been usually explored as a treatment for joint diseases, including osteoarthritis and rheumatoid arthritis, and for joint pain.Citation1–Citation3 The approach seeks to selectively deliver the agents to local tissues while minimizing overall systemic exposure.Citation1,Citation2 However, the therapeutic outcome following IA injection is considerably lessened by drug delivery issues that include rapid clearance into the systemic circulation from the synovial ultrastructure, which offers little barrier to the diffusion of molecules in and out of a joint.Citation4,Citation5 Knight and LevickCitation6 found that the synovial surface consists of a discontinuous layer of synoviocytes (covering 80% of the synovial lining) with wide intercellular gaps measuring 0.1–5.5 μm with no basal membrane. The IA route is even comparable to intramuscular or subcutaneous routes pharmacokinetically with regard to rapid redistribution of drug into the bloodstream.Citation7
Several polymeric microparticle and nanoparticle (NP) systems have been attempted with the goal of increasing the retention time of therapeutic agents within the joint cavity. The various polymers that have been explored include poly(lactide), poly(lactide-co-glycolide) (PLGA), albumin, and chitosan.Citation8–Citation12 However, NPs smaller than 250 nm in size rapidly escape from the joint cavityCitation13 and micro-sized particulates are prone to phagocytosis by macrophages in synovial linings.Citation14,Citation15 Recently, a positively surface-charged NP composed of an uncharged polymer (ethyl cellulose or dextran derivatives) and a positively charged polymer (cationic dextran) was introduced as an alternative tool to increase the retention time in joints.Citation16 The cationic NPs form filamentous structures in synovial fluid due to electrostatic interaction with endogenous hyaluronic acid (HA), which increase the retention time in the knees to 7 days when compared with the solution form. However, these encouraging results are tempered by the lack of data on safety and tolerability of ethyl cellulose and/or dextran derivatives, which is a roadblock to the use of injectable cationic NPs in clinical applications. In addition, more prolonged retention profiles are needed to permit less frequent IA injection and decrease patient discomfort.
PLGA has been extensively studied for the development of devices that enable the controlled delivery of small molecule drugs, proteins, and other macromolecules.Citation17,Citation18 PLGA is the most popular biodegradable polymer by virtue of its long clinical use, favorable degradation characteristics, and possibilities for sustained drug delivery. In particular, PLGA monomers are endogenous and easily metabolized by the body via the Krebs cycle.Citation17 Thus, minimal systemic toxicity is associated with the use of PLGA for drug delivery and/or biomaterial applications. Desired drug release profiles and administration interval could be effectively obtained by adjusting overall physical properties, such as polymer molecular weight, ratio of lactide to glycolide, and drug concentration in the polymeric particles.Citation19–Citation21
To these ends, cationic NPs composed of PLGA as the matrix polymer and the quaternary ammonium-containing polymer, Eudaragit RL, as the cationic polymer were prepared by solvent evaporation and the feasibility of these NPs as a platform for achieving prolonged retention in the joint of interest was investigated. Physicochemical characteristics of the novel particulates were characterized in terms of particle size, surface charge, loading amount of a fluorescent probe, and its release profile from the NPs. The morphology of the cationic NP and HA aggregates (NPs/HAs aggregates) and the distribution of probe-loaded NPs in the electrostatic aggregates were visualized using a hyperspectral imaging system (CytoViva, Auburn, AL, USA). Retention profiles of the fluorescence probe-loaded cationic NPs after IA injection into mouse knees were evaluated in vivo using an IVIS fluorescence imaging system (Xenogen, Hopkinton, MA, USA) in comparison to probe in solution.
Materials and methods
Materials
PLGA polymer with a lactide/glycolide ratio of 50:50 and a molecular weight 38,000–54,000 kDa (Resomer® 504, Evonik, Darmstadt, Germany), polyvinyl alcohol (PVA), sodium lauryl sulfate (SLS), dimethyl sulfoxide (DMSO), and phosphate-buffered saline (PBS) tablets were purchased from Sigma-Aldrich (St Louis, MO, USA). Sodium hyaluronate of microbial origin (molecular weight 1,500–2,500 kDa) was purchased from Humedix (Sungnam, South Korea). Eudragit RL (a copolymer of ethyl acrylate, methyl methacrylate, and a low content of methacrylic acid ester with quaternary ammonium groups) was kindly provided by Evonik. The fluorescent probe 1,1′-dioctadecyl-3,3,3′,3′ tetramethylindotricarbocyanine iodide (DiR) was purchased from Marker Gene Technologies, Inc. (Eugene, OR, USA). Ethyl acetate and dichloromethane were purchased from Samchun Pure Chemical (Kyunggi-do, South Korea). Acetonitrile and methanol were obtained from J.T. Baker (Phillipsburg, NJ, USA). All other chemicals used were of the highest commercial grade available.
Preparation of cationic NPs
Blank or DiR-loaded cationic NPs were fabricated by emulsification and solvent evaporation method.Citation22,Citation23 PLGA and Eudragit RL (total 125 mg) was dissolved in 5 mL of a 1:1 (v/v) mixture of dichloromethane and ethyl acetate, followed by addition of 250 μL of DiR dichloromethanic solution (10 mg/mL) to the organic phase. This organic mixture was emulsified in 20 mL of an aqueous PVA solution (0.1% w/v) by sonication using a model 2210 bath-type sonicator (Branson Ultrasonics, Danbury, CT, USA) operating at an output power of 90 W. The organic phase was evaporated with stirring at 400 rpm for 4 hours, and the obtained NPs were centrifuged at 20,000× g for 30 minutes. Supernatant was then removed, and the deposited NPs were washed three times with distilled water. The weight of the precipitated NPs was measured and an appropriate amount of distilled water was added to adjust the final polymer concentration to 6.25 mg/mL.
Physicochemical characterization of cationic NPs
Measurement of particle size and zeta potential
The NP samples (10 μL) were diluted to 990 μL with 10 mM PBS solution and were examined for size distribution and polydispersity index (PDI) using a Zetasizer Nano-ZS dynamic light-scattering particle size analyzer (Marlvern Instrument, Worcestershire, UK) equipped with a 50 mV laser at a scattering angle of 90°. Zeta potential measurements were taken with disposable capillary cells and the M3-PALS measurement technology built into the Zetasizer system. All measurements were carried out in triplicate under ambient conditions.
Hyperspectral observation of DiR-loaded NPs
A hyperspectral imaging system (CytoViva) was used to visualize the entrapped probe within the NPs by collecting all visible-near infrared spectral data and selecting and mapping spectral signals in the scanned samples.Citation24 Approximately 10 μL of DiR-loaded NP suspension was dropped onto a cover glass and analyzed using hyperspectral imaging system. The hyperspectral imaging equipment involves an Olympus microscope (Olympus Corporation, Tokyo, Japan) equipped with a visible-near infrared hyperspectral camera system, dual-mode fluorescence module, and high-resolution adaptor. The brightness intensity was controlled by a DC-stabilized 150 W halogen light source and lamp voltage was 11 V. HyperVisual Software ENVI 4.8 (Exelis Visual Information Solutions, Boulder, CO, USA) was used to obtain hyperspectral images.
Determination of loading amount and loading efficiency of DiR in NPs
The NP suspension (100 μL) was dissolved in DMSO (900 μL) by sonication for 15 minutes using the aforementioned sonicator at an output power of 90 W.Citation25 Then, the concentration of the fluorescent probe was calculated according to a DiR calibration curve constructed using a UV/Vis spectrometer (Flex station 3 microplate reader, Molecular Devices, Sunnyvale, CA, USA) at 700 nm.
Hyperspectral observation of NP/HA aggregates
A hyperspectral imaging system was even used to locate DiR-loaded NPs in NP/HA aggregates by tracing the spectral signature of the fluorescent probe. The DiR-loaded NP suspension (6.25 mg/mL) was added to 0.5 mL of HA dissolved in 10 mM PBS (2.2 mg/mL). The mixture was gently stirred then spread on glass slides and imaged as previously described.
Release profile of DiR from NPs and NP/HA aggregates
The release patterns of the probe from the cationic PLGA NPs or NP/HA aggregates were evaluated with a cellulose ester dialysis membrane (Spectra/Por Float-A-Lyzer G2 with 50 kDa molecular weight cut-off; Spectrum Labs, Rancho Dominguez, CA, USA).Citation26 In brief, 330 μL of DiR-encapsulated NP suspension and/or the aggregates contained in a dialysis bag was loaded to 60 mL of 1% (w/v) SLS-containing PBS solution (pH 7.4) at 37°C and rotated at 100 rpm. At scheduled intervals, 300 μL of the release medium was collected for the fluorescence assay (Flex station 3 microplate reader, Molecular Devices), with the excitation and emission wavelength selected at 748 and 792 nm, respectively. The same volume of fresh PBS buffer at the same temperature was added immediately to maintain a constant release volume.
Retention time of DiR-loaded NPs after IA injection in mouse knee
Animals
Five-week-old male hairless mice were obtained from Orient bio (Kyunggi-do, South Korea). Mice were housed under conditions that included a controlled light cycle and controlled temperature (23°C±1°C). Tap water and standard laboratory chow were available ad libitum. Mice were allowed to habituate to the housing facilities for at least 3 days before agent treatments. All animal experiments were performed in accordance with the “Principles of Laboratory Animal Care” (NIH publication number 85-23, revised 1996), and were approved by the Committee for Animal Experiments of Bundang Seoul National University Hospital (Kyunggi-do, South Korea).
IA administration and microscope fluorescent imaging
Mice were assigned to two groups by a stratified randomization scheme designed to achieve similar group mean body weights. One group received 10 μL of DiR solution (50 μg/mL) IA by an insulin syringe (31 G) into the right knee. DiR solution was prepared by solubilizing the hydrophobic probe in the 1% (w/v) SLS solution. Mice in the second group were given the same volume of DiR-loaded cationic NPs suspension by IA injection. The fluorescence images were obtained using an IVIS Imaging System.Citation27 The fluorescence intensities were estimated using the region of interest tool, which can determine the light flux. The in vivo fluorescence images of the mice were obtained using the image system at λex =745 nm and λem =840 nm (n=3).
Statistical analysis
All data are expressed as mean ± standard deviation (SD). Significance was determined by Student’s t-test, and the results were considered to be significant at P<0.05.
Results and discussion
Preparation and physicochemical characteristics of cationic NPs
The cationic NPs consisting mainly of PLGA, Eudragit RL, and PVA were fabricated by emulsion and evaporation procedure. The positively charged NPs might form core/shell structures in which the charged moiety of Eudragit RL predominantly resided on the surface of the NP and the neutral PLGA polymer and hydrophobic structure of the methacrylic acid derivatives preferentially resided inside the core of the NPs. Several studies found that the glass transition temperature (Tg) of PLGA/Eudragit RL NPs prepared by emulsion and solvent evaporation method was positioned between the Tg’s of the individual polymers, indicating that the two polymers were completely miscible when determined by differential scanning calorimeter.Citation28,Citation29 PLGA is a biocompatible and biodegradable polymer, and the parenteral products using this polymer have been already approved by the US Food and Drug Administration for human use.Citation30 Eudragit RL, which contains positively charged quaternary ammonium groups, was used in combination with PLGA to electrostatically interact with the anionic HA, forming an ionically cross-linked network. Aksungur et alCitation31 reported that there was no difference in the cytotoxicity of PLGA/Eudragit RL NPs and PLGA NPs after 24 or 48 hours of cell incubation. In addition, Yamashita et alCitation32 reported that histological studies revealed no acute inflammatory reaction within 7 days when the cationic methacrylate derivatives were injected to canine or rat renal arteries through microcatheter. A cationic methacrylate derivative was safe in swine when it was injected into retia mirabilia.Citation33 PVA remains on the surface of the PLGA NPs, forming an interconnected network with the polymer at the interface. The hydrophobic acetate moieties of PVA can be adsorbed to PLGA NPs during particle formulation, leading to excellent colloidal dispersibility in an aqueous medium with no aggregation.Citation34
The particle preparation process was studied by assessing PVA concentration in external phase, PLGA and Eudragit RL ratios, and phase ratio to clarify the influence of these factors on particle size and surface charge of blank NPs (). The effect of PVA content on the physical properties of the PLGA/Eudragit RL (50:50) NPs is shown in . There was a decrease in particle size from 250 to 150 nm when the PVA concentration in the external aqueous phase was increased from 0% to 1% (w/v). On the other hand, the surface charge of the NPs was rapidly decreased and neutralized with PVA concentration above 0.3%. The internal/external phase ratio did not significantly influence the droplet size and zeta potential of PLGA/Eudragit RL (50:50) NPs (). The effect of PLGA and Eudragit RL ratio was further investigated, and the results are shown in . In the PLGA/Eudragit RL NPs with 0.1% PVA, as the proportion of the cationic polymer was increased, surface charge of NPs increased and they reached a plateau at 10% Eudragit RL, with a particle size below 200 nm (). However, in the case of the NPs with 1.0% PVA, the particle size was affixed to 140–180 nm with a neutral surface charge, regardless of PLGA/Eudragit RL ratio (). Therefore, the PLGA/Eudragit RL ratio was determined to weight ratio of 70:30, and the PVA concentration in external phase was determined to 0.1% to formulate positively surface-charged NPs. This optimized NP composition provides appropriate particle size below 200 nm, with a surface potential of over 20 mV, and was used for further in vitro and in vivo studies.
Figure 1 Effect of formulation variables on size distribution and surface charge of PLGA/Eudragit RL NPs.
Notes: Influence of (A) PVA concentration and (B) organic phase and aqueous phase ratio on the particle diameter and surface charge of PLGA/Eudragit RL (50:50) NPs. Influence of PLGA and Eudragit RL ratio on particle size and surface charge with (C) 0.1% PVA and (D) 1.0% PVA aqueous phase.
Abbreviations: PLGA, poly(lactide-co-glycolide); NP, nanoparticle; PVA, polyvinyl alcohol.
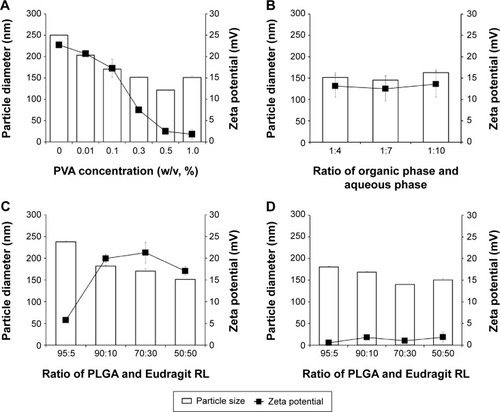
The cationic NPs containing DiR as a fluorescent probe were further characterized in terms of their particle size, zeta potential, DiR loading amount, and efficiency in aqueous medium. An emulsion and evaporation technique yielded narrow size distribution, with a mean hydrodynamic diameter of 170.1 nm (). The particles made of PLGA and PVA alone were negatively charged (−3 mV at pH 7.4, data not shown), while the incorporation of the cationic polymer with neutral PLGA polymer bestowed a positive charge on the surface (+21.3 mV) in 10 mM PBS. The zeta potential value is an important particle characteristic as it can influence physical stability of NPs as well as electrostatic interaction with endogenous HA. The presence of the positively charged groups on the particles could lead to electrical charge interactions with the anionic polymer, constructing micrometer-sized aggregates in the biological circumstances.
Table 1 Physicochemical characteristic of DiR-loaded PLGA/Eudragit RL NPs
Hyperspectral imaging system was used to visualize the entrapped fluorescent probe within the cationic NPs (). First, the spectral library plots of DiR and blank PLGA/Eudragit RL NPs were obtained (data not shown). Then, the microscopic image of each molecule was mapped from each spectrum using HyperVisual Software (Exelis Visual Information Solutions); the spectrum of the fluorescent probe was expressed as red color and NP as yellow color, respectively (). A hyperspectral image of DiR-loaded NPs (), co-mapped the fluorescent probe and NPs, shows that the location of DiR coincides with the position of NPs, suggesting that the fluorescent probe was efficiently entrapped within the NPs. Actually, the NP formulation exhibited a high loading efficiency (>80%) of the probe (). The hydrophobic fluorescence probe (log P>15) was efficiently encapsulated into the NPs, probably due to its poor solubility in the aqueous phase during the fabrication procedure.
Figure 2 Hyperspectral images of DiR-loaded NPs: (A) the probe mapped, (B) NP mapped, and (C) both the probe and NP mapped (bar =0.5 μm).
Note: The inset in all figures are magnified ×8 that of the image.
Abbreviations: DiR, 1,1′-dioctadecyl-3,3,3′,3′ tetramethylindotricarbocyanine iodide; PLGA, poly(lactide-co-glycolide); NP, nanoparticle.
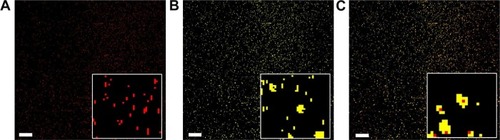
Hyperspectral imaging of NP/HA aggregates
Hyperspectral imaging was further utilized to investigate the morphology of the cationic NP/HA aggregates and the distribution of DiR-loaded NPs in these aggregates (). The concentration of HA in aqueous medium was set to 2.0 mg/mL because the concentration of HA in synovial fluid was reduced from 3.5 to below 2.2 mg/mL in osteoarthritic conditions.Citation30 displays representative optical micrographs of the filamentous NP/HA aggregates. Immediately upon mixing DiR-loaded NPs with HA under gentle agitation, discontinuous and filamentous aggregates ranging in diameter from 5 to 50 μm were precipitated without additional treatment (). DiR-loaded NPs were visualized in red using HyperVisual Software (Exelis Visual Information Solutions) to determine the distribution of NPs in the aggregates (). From the merged image of , we see that DiR-loaded NPs are localized homogeneously on the surface and/or inside of the aggregates (), which indicates that the electrostatic interaction of the cationic PLGA NP with the anionic polymer could be attributed to the formation of ionically cross-linked clusters. From these data, we expected that the construction of micrometer-sized NP/HA structures in synovial cavity restrict diffusion through the interstitium and across capillary walls in joints after IA injection of NP suspension. Also, the steric hindrance effect of the endogenous HA polysaccharides around the surface of the NP would help to avoid uptake by the reticuloendothelial system, thereby prolonging the retention of NP in the joint tissue. Mizrahy et alCitation35 reported that the attachment of HA ranging from 6.4 to 1,500 kDa on the NP surface acts as a steric barrier to reduce clearance of the NPs by macrophages in the liver and promotes enhanced permeation and retention.
Figure 3 Hyperspectral images of the DiR-loaded NPs/HAs aggregates in aqueous medium (bar =0.5 μm).
Notes: (A) Optical micrograph of the NPs/HAs aggregates, (B) DiR-loaded NPs in the aggregates rendered red using HyperVisual Software, and (C) a merged image of panels (A) and (B).
Abbreviations: DiR, 1,1′-dioctadecyl-3,3,3′,3′ tetramethylindotricarbocyanine iodide; PLGA, poly(lactide-co-glycolide); NP, nanoparticle; HA, hyaluronic acid.
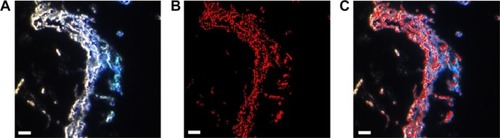
Figure 4 In vitro release of the fluorescent probe from the cationic NPs and NPs/HAs aggregates in 10 mM PBS with 1% (w/v) SLS at 37°C.
Note: Vertical bars represent mean ± SD (n=3).
Abbreviations: NP, nanoparticle; HA, hyaluronic acid; PBS, phosphate-buffered saline; SLS, sodium lauryl sulfate; SD, standard deviation.
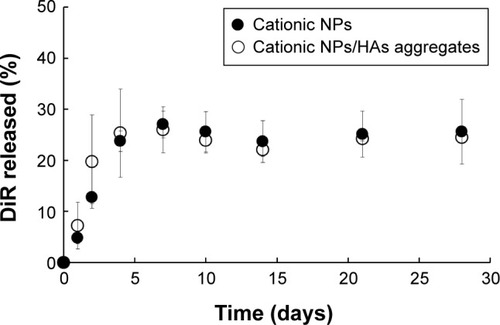
In vitro release profile of DiR from NPs and NP/HA aggregates
The composition of synovial fluid, a viscous liquid in diarthrodial joints, is nearly identical to plasma with respect to the concentrations of sodium, chloride, potassium, and total proteins, with the exception of the large polymers like fibrinogen or larger globulins.Citation36 Thus, physiological saline was judged to be the most appropriate medium to estimate the release profile of the fluorescent probe from the cationic NPs. However, DiR is practically insoluble in PBS (pH 7.4) and thus, an appropriate solubilizing agent was required to provide a sufficient solubility for the probe and to maintain the sink conditions during the release test. The aqueous solubility of DiR was markedly increased in the presence of SLS by self-association in aqueous solution above the critical micellar concentration. In particular, a medium with 1% (w/v) SLS allowed a solubility value of 90 μg/mL, which guaranteed the sink conditions for the probe during the release test.
shows the release profiles of the fluorescent probe from the cationic NPs and aggregates under sink condition at 37°C. Both NPs and NP/HA aggregates produced an initial burst release in which approximately 30% of the molecules were released within a week. This fast release could be attributed to the surface-associated probe and/or to the release of the probe encapsulated near to the particle surface.Citation17 After initial burst release, there was no measurable release of the probe from the hydrophobic matrix for a month. There were no significant differences in release profile between NPs alone and NP/HA aggregates. In sink condition, less than 30% of DiR was released from the NPs or those aggregates for a month, suggesting that the florescence signal of DiR could represent NP in the next in vivo study.
In vivo retention time of cationic NPs in knee joint
The retention profile of the DiR-loaded cationic PLGA/Eudaragit RL NPs in the knee joint was investigated by fluorescence imaging after IA injection in mice. There were no abnormal clinical signs indicative of an adverse effect arising from the IA administration of either DiR-loaded NPs or DiR solution. Representative images for solution and cationic NPs after IA injection are shown in . In addition, the relative fluorescent intensity profiles of cationic NPs versus a fluorescence probe solution are plotted in . IA injection of the probe in solution displayed a rapid decline in fluorescence levels to 30% of the initial concentration within 3 days. The average half-life of the probe in the joint determined was approximately 22 hours, indicating the rapid efflux from the joint. The retention time of the probe in the joint was fairly longer than those of small molecules previously reported, due to its extreme hydrophobicity and high molecular weight (1,013 g/mol). The mean half-lives of the nonsteroidal anti-inflammatory agents such as paracetamol, salicylate, and diclofenac were 1.1, 2.4, and 5.2 hours, respectively, after IA injection in human patients with knee rheumatoid arthritis.Citation37
Figure 5 Representative in vivo fluorescence images of (A) DiR-loaded cationic NPs and (B) free DiR solution after IA injection in mice knee at various time points. Notes: The scale bar range is (A) 0.5–2×108 and (B) 0.5–1.5×108 in fluorescence intensity.
Abbreviations: DiR, 1,1′-dioctadecyl-3,3,3′,3′ tetramethylindotricarbocyanine iodide; NP, nanoparticle; IA, intra-articular.
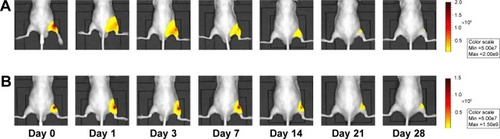
Figure 6 The relative fluorescence intensity profiles of DiR-loaded cationic NPs (■) or free DiR solution (□) after IA injection in mice knee.
Notes: The relative fluorescence intensities were expressed as fold-changes versus those determined immediately after IA injection (day 0) (n=3). The statistical analysis was performed using the Student’s t-test; *P<0.05 versus probe solution; **P<0.01 versus probe solution.
Abbreviations: DiR, 1,1′-dioctadecyl-3,3,3′,3′ tetramethylindotricarbocyanine iodide; NP, nanoparticle; IA, intra-articular; PLGA, poly(lactide-co-glycolide).
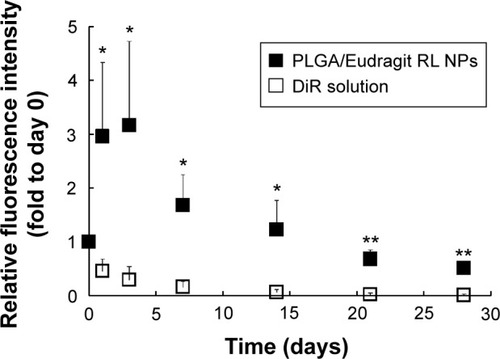
On the other hand, the cationic NPs exhibited significantly higher fluorescence intensity over 28 days after injection ( and ). More than 50% of the fluorescent probe incorporated in the cationic NPs remained detectable in the knee joint 28 days after injection (). Particle size is one critical factor in the IA drug delivery; particles <250 nm in diameter can freely escape from the joint cavity.Citation13 Another study reported that neutral charged PLGA NPs that were 265 nm in diameter were rapidly phagocytosed by macrophages after IA injection and, within a week, these macrophages migrated away from the synovial lining along with the neutral PLGA NPs.Citation38 However, in this study, the novel polymeric NPs exhibited prolonged long retention in the OA joint over 4 weeks. The mechanism of this prolonged retention is likely as follows. The formation of micrometer-sized, discontinuous, and filamentous structures due to electrostatic interaction between cationic NPs and the anionic polysaccharides restricts diffusion through the synovial surface that consists of a discontinuous layer of synoviocytes. In addition, the steric hindrance of the endogenous polymer adsorbed at surface of the cationic NP probably reduces the uptake by the reticuloendothelial system. Moreover, macrophages are enriched in joints, comprising approximately 30% of the cells in the intima and subintima in joints.Citation39,Citation40
The results of this study suggest that PLGA/Eudragit RL NPs can be an alternative to prolong the retention period of therapeutic agents entrapped in the NPs after IA administration. Further investigations including particle size optimization, preclinical local tolerability test of the novel formula in joint tissue, and further in vivo retention assessments in animal models that more closely duplicate the clinical setting will be performed to evaluate the feasibility of the cationic polymeric NPs as a platform for IA delivery for prolonged drug delivery in joint disease.
Conclusion
Cationic polymeric NPs were successfully prepared by conventional solvent evaporation procedure. The optimized NPs consisted of PLGA, Eudragit RL, and PVA, were 170.1 nm in size, displayed a zeta potential of 21.3 mV, and had a PDI value <0.3. Hyperspectral imaging confirmed the electrostatic interaction of the cationic NPs with the anionic HA polysaccharides, attributed to the rapid formation of micrometer-sized, ionically cross-linked clusters that localized the NPs on the surface and/or inside of the aggregates. In in vivo study in mice, the NPs were efficiently retained in the knee joint for over 4 weeks, demonstrating that the novel particulate system could prolong the retention interval in joint cavity after IA injection. With desirable retention behavior in joints, this formulation is a promising tool for prolonged delivery of various therapeutic agents, via IA injection, in diseased joints.
Acknowledgments
This research was supported by Basic Science Research Program through the National Research Foundation of Korea (NRF) funded by the Ministry of Science, ICT, and Future Planning (NRF-2013R1A1A1058935).
Disclosure
The authors report no conflicts of interest in this work.
References
- MountziarisPMKramerPRMikosAGEmerging intra-articular drug delivery systems for the temporomandibular jointMethods200947213414018835358
- EdwardsSHIntra-articular drug delivery: the challenge to extend drug residence time within the jointVet J20111901152120947396
- KangMLImGIDrug delivery systems for intra-articular treatment of osteoarthritisExpert Opin Drug Deliv201411226928224308404
- WiggintonSMChuBCWeismanMHHowellSBMethotrexate pharmacokinetics after intraarticular injection in patients with rheumatoid arthritisArthritis Rheum19802311191226965451
- AyralXInjections in the treatment of osteoarthritisBest Pract Res Clin Rheumatol200115460962611567543
- KnightADLevickJRMorphometry of the ultrastructure of the blood-joint barrier in the rabbit kneeQ J Exp Physiol19846922712886729017
- SchurmanDJKajiyamaGAntibiotic absorption from infected and normal joints using a rabbit knee joint modelJ Orthop Res1985321851883998894
- HorisawaEHirotaTKawazoeSProlonged anti-inflammatory action of DL-lactide/glycolide copolymer nanospheres containing betamethasone sodium phosphate for an intra-articular delivery system in antigen-induced arthritic rabbitPharm Res200219440341012033371
- Fernández-CarballidoAHerrero-VanrellRMolina-MartıńezITPastorizaPBiodegradable ibuprofen-loaded PLGA microspheres for intraarticular administration: effect of Labrafil addition on release in vitroInt J Pharm20042791–2334115234792
- TunçayMCalisSKaşHSErcanMTPeksoyIHincalAAIn vitro and in vivo evaluation of diclofenac sodium loaded album in microspheresJ Microencapsul200017214515510738690
- ThakkarHSharmaRKMishraAKChuttaniKMurthyRSEfficacy of chitosan microspheres for controlled intra-articular delivery of celecoxib in inflamed jointsJ Pharm Pharmacol20045691091109915324477
- LiangLSWongWBurtHMPharmacokinetic study of methotrexate following intra-articular injection of methotrexate loaded poly(L-lactic acid) microspheres in rabbitsJ Pharm Sci20059461204121515858840
- LevickJRA method for estimating macromolecular reflection by human synovium, using measurements of intra-articular half livesAnn Rheum Dis19985763393449771207
- ChampionJAWalkerAMitragotriSRole of particle size in phagocytosis of polymeric microspheresPharm Res20082581815182118373181
- EdwardsSHCakeMASpoelstraGReadRABiodistribution and clear-ance of intra-articular liposomes in a large animal model using a radiographic markerJ Liposome Res2007173–424926118027245
- MorgenMTungDBorasBMillerWMalfaitAMTortorellaMNanoparticles for improved local retention after intra-articular injection into the knee jointPharm Res201330125726822996566
- KumariAYadavSKYadavSCBiodegradable polymeric nanoparticles based drug delivery systemsColloids Surf B Biointerfaces201075111819782542
- DanhierFAnsorenaaESilvaJMCocoRLe BretonAPréatVPLGA-based nanoparticles: An overview of biomedical applicationsJ Control Release2012161250552222353619
- AllisonSDEffect of structural relaxation on the preparation and drug release behavior of poly(lactic-co-glycolic)acid microparticle drug delivery systemsJ Pharm Sci20089762022203517828755
- MundargiRCBabuVRRangaswamyVPatelPAminabhaviTMNano/micro technologies for delivering macromolecular therapeutics using poly(D, L-lactide-co-glycolide) and its derivativesJ Control Release2008125319320918083265
- MohamedFVan der WalleCFEngineering biodegradable polyester particles with specific drug targeting and drug release propertiesJ Pharm Sci2008971718717722085
- SoppimathKSAminabhaviTMKulkarniARRudzinskiWEBiodegradable polymeric nanoparticles as drug delivery devicesJ Control Release2001701–212011166403
- MainardesRMEvangelistaRCPLGA nanoparticles containing praziquantel: effect of formulation variables on size distributionInt J Pharm20052901–213714415664139
- LeeESLimCSongHTA nanosized delivery system of super-paramagnetic iron oxide for tumor MR imagingInt J Pharm20124391–234234822959991
- BaoWZhouJLuoJWuDPLGA microspheres with high drug loading and high encapsulation efficiency prepared by a novel solvent evaporation techniqueJ Microencapsul200623547147916980270
- ZhangTSturgisTFYouanBCpH-responsive nanoparticles releasing tenofovir intended for the prevention of HIV transmissionEur J Pharm Biopharm201179352653621736940
- HuXWangQLiuYOptical imaging of articular cartilage degeneration using near-infrared dipicolylamine probesBiomaterials201435267511752124912814
- ParkMHBaekJSLeeCAKimDCChoCWThe effect of Eudragit type on BSA-loaded PLGA nanoparticlesJ Pharm Invest201444339349
- DillenKVandervoortJVan den MooterGLudwigAEvaluation of ciprofloxacin-loaded Eudragit® RS100 or RL100/PLGA nanoparticlesInt J Pharm2006314728216600538
- MaoSGuoCShiYLiLCRecent advances in polymeric microspheres for parenteral drug delivery – part 2Expert Opin Drug Deliv20129101209122322924745
- AksungurPDemirbilekMDenkbaşEMDevelopment and characterization of Cyclosporine A loaded nanoparticles for ocular drug delivery: cellular toxicity, uptake, and kinetic studiesJ Control Release201115128629421241752
- YamashitaKTakiWIwataHKikuchiHA cationic polymer, Eudragit-E, as a new liquid embolic material for arteriovenous malformationsNeuroradiology199638151156
- ArakawaHMurayamaYDavisCREndovascular embolization of the swine rete mirabile with eudragit-E 100 polymerAJNR Am J Neuroradiol2007281191119617569986
- PisaniEFattalEParisJRingardCRosilioVTsapisNSurfactant dependent morphology of polymeric capsules of perfluorooctyl bromide: influence of polymer adsorption at the dichloromethane-water interfaceJ Colloid Interface Sci20083261667118674774
- MizrahySRazSRHasgaardMHyaluronan-coated nanoparticles: the influence of the molecular weight on CD44-hyaluronan interactions and on the immune responseJ Control Release2011156223123821745506
- GerwinNHopsCLuckeAIntraarticular drug delivery in osteoarthritisAdv Drug Deliv Rev200658222624216574267
- OwenSGFrancisHWRobertsMSDisappearance kinetics of solutes from synovial fluid after intra-articular injectionBr J Clin Pharmacol19943843493557833225
- HorisawaEKubotaKTuboiISize-dependency of DL-lactide/glycolide copolymer particulates for intra-articular delivery system on phagocytosis in rat synoviumPharm Res200219213213911883639
- GreisenHASummersBALustGUltrastructure of the articular cartilage and synovium in the early stages of degenerative joint disease in canine hip jointsAm J Vet Res19824311196319717181195
- Pasquali-RonchettiIFrizzieroLGuerraDAging of the human synovium: An in vivo and ex vivo morphological studySemin Arthritis Rheum19922164004141626286