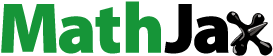
Abstract
The severe inflammation observed during sepsis is thought to cause diaphragm dysfunction, which is associated with poor patient prognosis. Cerium oxide (CeO2) nanoparticles have been posited to exhibit anti-inflammatory and antioxidative activities suggesting that these particles may be of potential use for the treatment of inflammatory disorders. To investigate this possibility, Sprague Dawley rats were randomly assigned to the following groups: sham control, CeO2 nanoparticle treatment only (0.5 mg/kg iv), sepsis, and sepsis+CeO2 nanoparticles. Sepsis was induced by the introduction of cecal material (600 mg/kg) directly into the peritoneal cavity. Nanoparticle treatment decreased sepsis-associated impairments in diaphragmatic contractile (Po) function (sham: 25.6±1.6 N/cm2 vs CeO2: 23.4±0.8 N/cm2 vs Sep: 15.9±1.0 N/cm2 vs Sep+CeO2: 20.0±1.0 N/cm2, P<0.05). These improvements in diaphragm contractile function were accompanied by a normalization of protein translation signaling (Akt, FOXO-1, and 4EBP1), diminished proteolysis (caspase 8 and ubiquitin levels), and decreased inflammatory signaling (Stat3 and iNOS). Histological analysis suggested that nanoparticle treatment was associated with diminished sarcolemma damage and diminished inflammatory cell infiltration. These data indicate CeO2 nanoparticles may improve diaphragmatic function in the septic laboratory rat.
Introduction
The pharmacological application of nanotechnology has become an area of intense interest in recent years, and some have posited that cerium oxide (CeO2) nanoparticles may be uniquely suited for several different types of biomedical applications.Citation1 Ceria is an element of the lanthanide series that when complexed with oxygen allows it to effectively transition between oxidative states (Ce+3 and Ce+4) where it can participate in redox cycling and function as antioxidant.Citation2 Other data have suggested that CeO2 nanoparticles are thought to be largely nontoxic under physiologic conditions,Citation3 that they appear to be well tolerated in vivo,Citation4 that may also exhibit antimicrobial effects,Citation5 and that they may be useful for the treatment of disease states characterized by elevations in cellular reactive oxygen species.Citation6 Consistent with these data, recent studies have demonstrated that CeO2 nanoparticles may be useful for the treatment of conditions associated with elevated oxidative stress including cardiac dysfunction, some types of neurodegeneration, cancer, and inflammation.Citation7–Citation10 Despite numerous medical advancements, the mortality rate associated with sepsis-associated multiple organ dysfunction syndrome remains unacceptably high as it is the leading cause of death in the hospital intensive care unit.Citation11 Sepsis and other severe inflammatory disorders are often associated with diaphragm dysfunction, which if uncorrected oftentimes results in decreased survivability and the need for ventilator support.Citation12–Citation14
Recent work by our laboratory has suggested that treatment with CeO2 nanoparticles is associated with diminished sepsis-induced mortality and decreased levels of systemic inflammation after polymicrobial insult.Citation15 Whether this increase in survivability following CeO2 nanoparticle intervention also associated with improvements of diaphragm function is currently unclear. On the basis of previous data demonstrating that rodent diaphragm is particularly susceptible to elevations in oxidative stress and circulating cytokines,Citation16–Citation18 we hypothesized that septic animals that had been treated with CeO2 nanoparticles would exhibit improved diaphragmatic function compared with that observed in the untreated counterparts. Our findings suggest that CeO2 nanoparticle treatment improves diaphragm contractility following a severe polymicrobial insult, and that this finding is associated with evidence of diminished muscle damage and inflammation.
Materials and methods
Materials
All chemicals were purchased from Sigma-Aldrich Co. (St Louis, MO, USA), unless otherwise specifically stated. Antibodies against Phospho-Akt (Ser473) (C31E5E)(#4060), Akt (pan) (40D4) (#2920), Phospho-Stat3 (Tyr705) (D3A7) XP (#9145), Stat3 (#9132), α-tubulin (#2144), Bax (#2772), Bcl-2 (#2876), Ubiquitin (p4D1) (#3936), 4E-BP1 phos(thr37/46) (#9459), and 4E-BP1 Non phos (thr46) (#4923) were purchased from Cell Signaling Technology (Beverly, MA, USA). Horseradish peroxidase-conjugated anti-mouse (#7076) and anti-rabbit (#7074) secondary antibodies were also purchased from Cell Signaling Technology. Caspase 8 primary antibody (sc-7890) was obtained from Santa Cruz Biotechnology Inc. (Dallas, TX, USA). iNOS primary antibody (PA1-036) was from Pierce (Rockford, IL, USA).
Animals
Ten-week-old male Sprague Dawley rats were purchased from Hilltop Laboratories (Scottdale, PA, USA) and housed for 1–2 weeks to allow acclimation before the initiation of any experiments. Animals were housed two to a cage with 12–12 dark–light cycles at 25°C. Food and water were provided ad libitum. All animal care and experimental procedures were performed after approval by the Marshall University Institutional Animal Care and Use Committee, and in accordance with the Guide for the Care and Use of Laboratory Animals.Citation19
Cecal inoculum peritonitis model
Animals were anesthetized using isoflurane and fecal peritonitis was induced by making a small abdominal incision followed by the introduction of cecal material and closure of the incision as described previously.Citation20 The cecal material was prepared by mixing cecal contents obtained from fresh donor rats with 5% dextrose water to yield a concentration of 600 mg cecal material in 5 mL/kg. The cecal material was used immediately and prepared fresh for each experiment. The cecal inoculums (CI) and CI+CeO2 nanoparticle treatment animals received fresh inoculum from the same donor animal for each experiment. Sham control and CeO2-only animals underwent an identical surgery to induce fecal peritonitis but received sterile dextrose water (5 mL/kg, ip) only.
CeO2 nanoparticle treatment
The characterization of CeO2 nanoparticles (US Research Nanomaterials, Inc., Houston, TX, USA) were imaged using a JEOL JEM-2010 (JEOL, Tokyo, Japan) transmission electron microscope as previously described.Citation21,Citation22 Scanning transmission electron microscopy images were acquired using an aberration-corrected analytical electron microscope (transmission electron microscope [TEM]/scanning transmission electron microscope [STEM] JEOL JEM-ARM200CF) operated at 200 keV.
For the nanoparticle treatment groups, CeO2 nanoparticles (0.5 mg/kg) in 200 µL of sterile distilled water were given via the tail vein at the time of peritonitis induction or sham surgery. This dose was chosen based on our preliminary data from pilot studies examining animal mortality and previously published data.Citation15,Citation21 The sham control and the CI groups received vehicle (200 µL of sterile distilled water). For the biochemical assessments, animals were sacrificed at 3 or 18 hours while functional assessments of diaphragm contractility were performed at 18 hours. These time points were selected based on previous data suggesting that the 3-hour time point appears to represent the initial systemic inflammatory response syndrome phase while the 18-hour time point coincides with the development of multiple organ dysfunction.Citation15
In vitro diaphragm contraction
Diaphragm contractile function was measured as previously described.Citation23,Citation24 Briefly, the entire diaphragm with ribs and the central tendon attached was removed from isoflurane anesthetized animals and quickly placed in ice cold Krebs buffer solution containing (mM) 118 NaCl, 4.7 KCl, 2.5 CaCl2, 1.2 MgSO4, 10 glucose, 1.2 NaH2PO4, and 25 NaHCO3 oxygenated with 95% O2 plus 5% CO2 to maintain pH 7.4. Two rectangular muscle strips approximately 3–4 mm in width from the costal region of each hemidiaphragm were dissected from the central tendon to the rib along the fiber orientation. The central tendon and ribs were tied with silk sutures with loops to mount the muscle strips in the organ bath. The muscle strips were mounted on the two stainless pins in a glass organ bath, and electrical field stimulation (EFS) was delivered using two platinum electrodes along either side of the muscle (Grass Stimulator S88). The Krebs buffer solution in the organ bath contained 10 µM d-tubocurarine chloride (Sigma-Aldrich Co.) to block nicotinic acetylcholine receptors during electric stimulations, and the bath temperature was kept at 25°C. After the 15-minute equilibration, EFS with 2 milliseconds single square-wave pulse was delivered at super maximal voltage set at 140 V to contract the muscle strips. To determine optimal tension (Lo), diaphragm muscle strips were stretched in a stepwise manner, and the single-pulse twitch protocol was repeated to achieve the maximum active tension. Thereafter, tetanic tension was assessed at Lo by measuring active tension developed in response to the sequential stimulation with trains of 1–150 Hz stimuli (train duration 500 milliseconds). An interval of 3 minutes was used between the muscle contractions. Isometric contraction was recorded using a force displacement transducer (Grass Technologies, Warwick, RI) interfaced with a Grass polygraph, and the data were digitized at 5 kHz using a storage oscilloscope (DSO-8502, Link Instrument Inc., Fairfield, NJ, USA). Specific tension was normalized to the estimated muscle cross-sectional area by the following equation:
Immunoblot analysis
Semiquantitiative immunodetection was performed as detailed previously.Citation26 Briefly, diaphragms were pulverized and then homogenized in Tissue Protein Extraction Reagent (Pierce) supplemented with 1 mM Dithiothreitol (DTT), protease, and phosphatase inhibitor cocktails (Sigma-Aldrich Co.). Lysates were sonicated on ice and centrifuged at 10,000× g at 4°C for 10 minutes. The supernatant was removed, and protein concentration was determined by 660 protein assay reagent (Thermo Fisher Scientific, Waltham, MA, USA). Samples were diluted in Laemmli sample buffer, and equal amounts of protein were separated by SDS-PAGE, followed by electroblotting onto nitrocellulose membranes. Membranes were blocked with 5% milk in Tris Buffered Saline with Tween (TBST) for 1 hour at room temperature and washed three times in TBST before incubation overnight at 4°C with primary antibodies. Membranes were incubated with the secondary antibody conjugated with horseradish peroxidase (HRP) for 1 hour at room temperature. An enhanced chemiluminescence (ECL) was used to detect immunoreactivity using a FluorChem E System (Cell Biosciences, Santa Clara, CA, USA).
Histochemical analysis
Procion orange
A bolus administration of 1.5 mL (3% Procion orange in 0.9% sterile saline) was infused via femoral vein catheter. After allowing the dye to circulate for 15 minutes, the diaphragm muscle was removed and quickly frozen in liquid nitrogen-cooled isopentane.Citation27 In some animals, muscle strips were immersed in HEPES buffer containing 0.2% Procion orange (Sigma-Aldrich Co.) for 1 hour at room temperature and quickly frozen as described above.Citation28 Frozen tissues were serially sectioned in 10 µm and placed on poly-lysine-coated slides before imaging with an inverted fluorescent microscope (EVOS AMG, Bothell, WA, USA) under a green fluorescent protein filter (excitation 450 nm/emission 505 nm).
Hematoxylin and eosin staining
Frozen tissue sections from animals without Procion orange perfusion were fixed in 4% formalin in phosphate buffer solution (PBS), and the sections were stained with H&E according to the manufacturer (BBC Biochemical, Mount Vernon, WA, USA). Images were obtained using an inverted microscope (EVOS AMG).
Real-time polymerase chain reaction
Real-time polymerase chain reaction (PCR) was performed for quantification of iNOS and GAPDH using a 7500 Real Time PCR system (Thermo Fisher Scientific, Waltham, MA, USA) as described previously.Citation26 Briefly, total RNA was extracted using Trizol reagent (Sigma-Aldrich Co.) according to the manufacturer’s guidelines, and cDNA was synthesized using 1 µg of RNA with the High-Capacity cDNA Reverse Transcription Kit (Thermo Fisher Scientific). SYBR green PCR amplification was performed with SensiMix™ SYBR low-ROX kit (Bioline, London, UK) using the following primers: iNOS (NM_012611.3; 5′-GGA AGA GAC GCA CAG GCA GAG-3′ and 5′-GCA GGC ACA CGC AAT GAT GG-3′; product size 126 bp) and GAPDH (NM_017008.4; 5′-GTT ACC AGG GCT GCCTTC TC-3′ and 5′-GGG TTT CCC GTT GAT GAC C-3′; product size 168 bp) according to the manufacturer’s guidelines. Each sample was analyzed in triplicate in 25 µL reactions. A nontemplate control was used as a negative control and included in all PCR reactions. Specific products were determined by the melting curve analysis as well as agarose gel electrophoresis using ethidium bromide. Expression levels between groups were compared using the relative comparison method (2−∆∆CT).
Statistics
Data are presented as the mean and standard error of the mean as described in the text and Z legends. Statistical comparisons were made using paired t-tests and one- or two-way ANOVA with Bonferroni post hoc tests as appropriate. A P<0.05 was considered as significant.
Results
CeO2 nanoparticle characterization
Details regarding the characterization of CeO2 nanoparticles used in this study have been previously published.Citation15 SEM and TEM analyses suggested that the particles were likely spherical in shape and ~15–20 nm in diameter ( and B).
CeO2 nanoparticle treatment improves diaphragmatic function in the septic rat
There were significant differences in pre-/post-body weight and serum hematocrit levels for the CI and CI+CeO2 groups but not for the sham and CeO2-only treated animals at 18 hours after the surgery (). The muscle length at which maximal tension was developed (Lo) was not different between groups (). Muscle strips from all groups demonstrated frequency-dependent increased tension (). Peak twitch tension was 93%±5%, 68%±4%, and 82%±5% of sham control for CeO2 only, CI, and CI+CeO2, respectively (; P<0.05). Similarly, peak tetanic tension was 89%±3%, 62%±4%, and 78%±4% of sham control for CeO2 only, CI, and CI+CeO2, respectively (; P<0.05). Consistent with these data, sepsis appeared to increase Procion orange dye diffusion into the diaphragm compared with that observed in the other groups (). Histological analysis following H&E staining suggested that sepsis was associated with increased cell infiltration that appeared to be diminished with nanoparticle treatment ().
Table 1 Characteristics of diaphragm strip and animal each group at 18 hours
Figure 2 Cerium oxide (CeO2) nanoparticle treatment improves diaphragmatic contractility in cecal inoculum (CI) rat.
Notes: Representative recording traces of contractile response are shown from each group (A). Group data showed that nanoparticle treatment improves diaphragm tension development (B, control n=8 strips from 4 rats, CeO2 only n=6 strips from 3 rats, cecal inoculum (CI) n=16 strips from 8 rats and CI+CeO2 n=18 strips from 9 rats). *vs Control, #vs CI: P<0.05 by two-way repeated measures ANOVA.
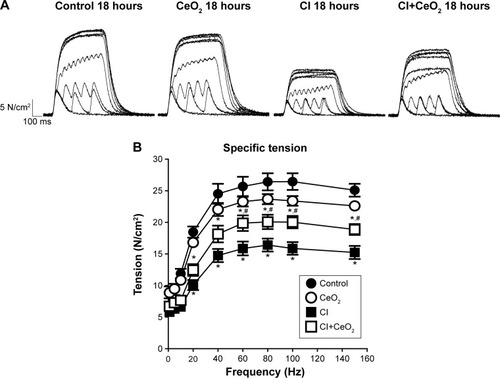
Figure 3 Cerium oxide (CeO2) nanoparticle treatment decreases cecal inoculum (CI) induced muscle damage.
Notes: Nanoparticle treatment functions to protect the muscle membrane as suggested by diminished Procion orange signal in the treated animals. Images were obtained using an inverted fluorescent microscope with 10× objective lens using a GFP filter (A). Sepsis increases and nanoparticle treatment decreases cellular infiltration. Representative bright field images were captured at 20× objective lens (B).
Abbreviations: GFP, green fluorescent protein; H&E, hematoxylin and eosin.
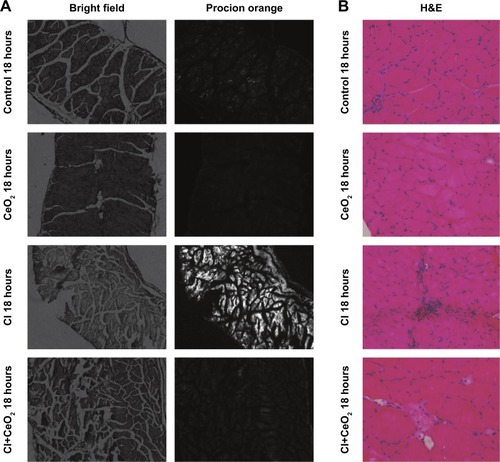
CeO2 nanoparticle treatment attenuates sepsis-associated diaphragmatic inflammation
Nanoparticle treatment decreased sepsis-induced increases in Stat3 phosphorylation at 3- but not 18 hours (; P<0.05). Nanoparticle treatment significantly decreased iNOS transcript levels and protein in the septic animals (; P<0.05).
Figure 4 Cerium oxide (CeO2) nanoparticle treatment attenuates diaphragmatic inflammation after septic insult.
Notes: Cecal inoculum (CI) induced increases in Stat3 phosphorylation are diminished with nanoparticle treatment (A, n=6 rats/each group). Real-time PCR showed that iNOS gene expression levels was increased with CI group and diminished with nanoparticle treatment at 18 hours (B, n=6 rats/each group). Immunoblot for iNOS/α-tubulin is shown (C). *vs Control, **vs CI: P<0.05 by one-way ANOVA.
Abbreviation: PCR, polymerase chain reaction.
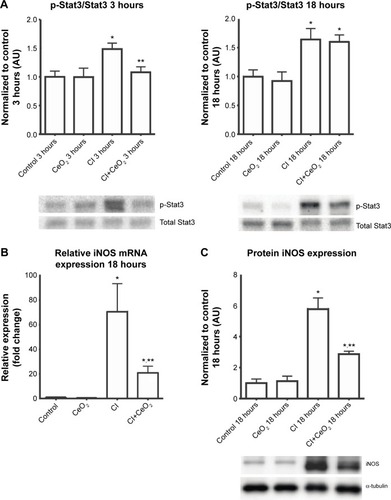
CeO2 nanoparticle treatment suppresses translation-related signaling, caspase 8 cleavage, and protein ubiquination during sepsis
Compared with the control animals, the phosphorylation of Akt, FOXO-1, and 4EBP-1 was not changed at 3 hours (data not shown). Nanoparticle treatment attenuated sepsis-induced decreases in the phosphorylation of Akt, FOXO-1, and 4EBP-1 at 18 hours (; P<0.05). Similarly, nanoparticle treatment reduced sepsis-associated protein ubiquination at 3 hours (; P<0.05) and caspase 8 cleavage at 18 hours (; P<0.05). Neither sepsis nor nanoparticle treatment was associated with changes in caspase 3 cleavage, apoptosis-inducing factor, or the ratio of Bax/Bcl-2 (data not shown).
Figure 5 Cerium oxide (CeO2) nanoparticle treatment normalizes the disruption of protein synthesis signaling in cecal inoculum (CI) peritonitis diaphragm.
Notes: Effect of nanoparticle on protein synthesis signaling was determined by immunoblot. Nanoparticle treatment increases phospho-Akt (A), phospho-Foxo1 (B), and phospho-4E-BP1 (C) levels in the septic diaphragm (n=6 rats/each group). *vs Control: P<0.05 by one-way ANOVA. **vs CI: P<0.05 by t-test.
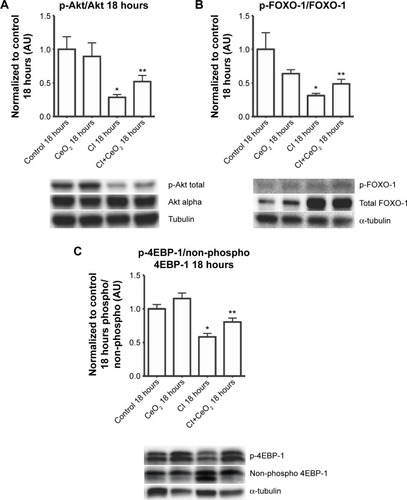
Figure 6 Cerium oxide (CeO2) nanoparticle treatment decreases protein ubiquitination and caspase 8 cleavage during sepsis.
Notes: Effect of nanoparticle on protein synthesis signaling was determined by immunoblot. Sepsis and treatment associated changes in protein ubiquitination (A) and caspase 8 cleavage (B). n=6 rats/each group, *vs Control: **vs CI: P<0.05 by one-way ANOVA.
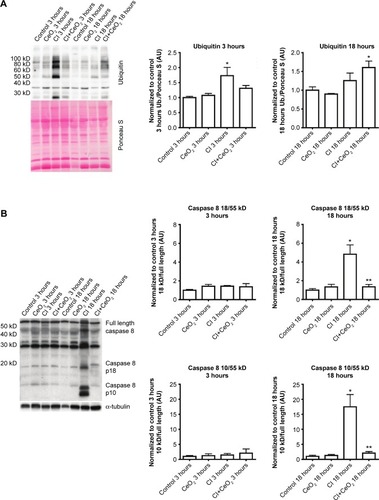
Discussion
Previous work from our laboratory using the CI peritonitis model has demonstrated that CeO2 nanoparticle treatment was associated with improved animal survivability and diminished systemic inflammationCitation15 with the latter occurring most likely due to diminished Kupffer cell activation.Citation21 In this study, we show that CeO2 nanoparticle treatment attenuates the diaphragm dysfunction induced by the septic condition. We believe that these are the first data to demonstrate that nanoparticle treatments designed to target the liver Kupffer cells might be useful for preventing the muscle dysfunction and atrophy seen in clinical conditions characterized by severe systemic inflammation. Similar to earlier studies employing other sepsis models,Citation23,Citation29–Citation31 we show that the CI peritonitis model is also characterized by alterations in diaphragmatic function (). Importantly, we also found that the CeO2 nanoparticle treatment appeared to diminish the effects that the septic condition had on diaphragm tension development.
Whether these improvements in diaphragm contractility also reflect an improved pulmonary function in vivo is currently unclear. Similarly, why muscle function may be decreased with sepsis, or other conditions that are characterized by increased systemic inflammation are not entirely understood; however, it has been suggested that increased cellular infiltration and/or muscle membrane damage may play a role.Citation32 To investigate this possibility, we examined the effects of sepsis and nanoparticle treatment on muscle histology and membrane permeability following exposure to Procion dye. Similar to previous reports,Citation32,Citation33 we found that the diaphragms obtained from septic animals exhibited a marked increase sarcolemma damage and cellular infiltration (). In addition, and consistent with our findings of improved contractile function with nanoparticle treatment, we found that these changes in cellular structure appeared to be lessened with nanoparticle treatment (). To extend these data, we next examined if the nanoparticle treatment modulated inflammatory signaling in diaphragm. Several studies have suggested that elevations in the local (muscle) or circulating tumor necrosis factor (TNF)-alpha may play a key role in regulating diaphragmatic function during infection.Citation16,Citation23,Citation34–Citation37 Our data are consistent with this view and previous work from our laboratory that demonstrated elevations in systemic inflammation in the rat CI peritonitis model. How circulating cytokines cause muscle contractile dysfunction remains unclear; however, it has been shown that the activation of Stat3–iNOS signaling by elevations in IL-6 is associated with diminished cardiac contractility.Citation38 Similar to the mechanisms of the inflammatory response in cardiac muscle, we found that sepsis significantly increased Stat3 phosphorylation in diaphragm, and importantly, that this increase was attenuated with nanoparticle treatment (). Likewise, increases in NO have been shown to directly impair contractile function in a number of different skeletal muscles including the diaphragm.Citation23,Citation30,Citation32,Citation39 Consistent with this premise, we found that sepsis significantly increased iNOS gene expression and protein content in diaphragm (). Similar to our contractile measurements, these alterations in NO signaling were attenuated with nanoparticle treatment. Given that CeO2 nanoparticles have been shown to possess not only redox modulation but also immunomodulation properties in vitro,Citation7,Citation8,Citation40 it is possible that our in vivo CeO2 nanoparticle treatment effects may be involved in the regulation of immune function and NO-associated oxidative stress. Taken together, these data suggest that nanoparticle treatment attenuated peritonitis-induced diaphragm dysfunction and that this improvement in contractility may be associated with diminished inflammatory signaling.
CeO2 nanoparticle treatment improves protein degradation/synthesis signaling in the septic diaphragm
In addition to muscle damage and increased inflammatory signaling, it is also thought that increases in protein degradation may also be associated with diminished contractile function.Citation41 It is thought that protein degradation is controlled, at least in part, by the activity of the muscle E3 ligases, MURF-1 and MAFbx, which, in turn, are thought to be regulated by FOXO-1. The activity of FOXO-1 is controlled by its phosphorylation by Akt, with increased FOXO-1 phosphorylation causing a decrease in its transcriptional activity.Citation42 We found that total FOXO-1 expression was increased in CI peritonitis groups (). This finding is in agreement with previous reports that suggested that both cecal ligation and puncture (CLP)- and lipopolysaccharide (LPS)-induced sepsis models are associated with increased skeletal muscle FOXO-1 mRNACitation43,Citation44 and protein levels.Citation45 Conversely, we noted that peritonitis was associated with diminished ratio of Akt and FOXO-1 phosphorylated (active)/total protein and that this ratio that appeared to be attenuated with the treatment (). These data are consistent with the possibility that the CeO2 nanoparticle intervention might function to diminish the degree of sepsis-induced muscle breakdown. Why sepsis might increase total FOXO-1 expression is currently unclear, but it is possible that this change could be a compensatory mechanism to counteract sepsis-associated decreases in FOXO-1 activity. Further studies will be required to address the mechanism(s) responsible for this change, and perhaps, more importantly, the physiological relevance of why sepsis might affect FOXO-1 protein levels in skeletal muscle. In addition to its ability to protect the muscle against apoptosis and protein degradation, it is also known that septic insults cause decrease protein translation signaling.Citation46,Citation47 To investigate this possibility, we next determined the effect of sepsis and the nanoparticle intervention on the phosphorylation of 4EBP-1. It is thought that 4EBP-1 functions to inhibit translation by binding to the translation initiation factor elF4E. Consistent with previous work from other groups showing both endotoxemia and polymicrobial sepsis models decrease phosphorylation of 4EBP-1 in rat skeletal muscle,Citation46,Citation47 we found that sepsis was associated with decreased phosphorylation of 4EBP-1 (). Importantly, we also found that the nanoparticle treatment normalized this decreased phosphorylation of 4EBP-1 in diaphragm.
Similar to that observed for the effects of protein degradation on muscle function, it is also thought that increased muscle apoptosis can also diminish muscle function. Similar to previous reports using the LPS endotoxemia modelCitation48 and other work from our laboratory examining the effects of sepsis on circulating cytokine levels,Citation15 we also found that this model was associated with increased caspase-8 cleavage (). To determine whether increases in caspase-8 cleavage led to increased muscle apoptosis, we next examined if peritonitis was associated with increased cleavage of caspase-3. Unlike previous data showing that LPS challenge increased caspase-3 activity in the rat diaphragm, we failed to find any change caspase-3 cleavage or caspase-3 activity in CI peritonitis model (data not shown). Supporting these data, we also failed to find any change in the ratio of Bax/Bcl-2 or in the amount of Apoptosis inducing factor (AIF) protein. Why endotoxemia but not the sepsis induced by peritonitis may be associated with increased caspase-3 activation is currently unclear.
Conclusion
In conclusion, we have demonstrated that CeO2 nanoparticle treatment attenuated diaphragm dysfunction in a laboratory rat model of sepsis. We believe that this may be the first study to demonstrate that the systemic delivery of nanoparticles can be used to improve muscle function in a preclinical animal model of sepsis. How the nanoparticle treatment improved diaphragm contractility is currently unclear and will require additional experimentation.
Acknowledgments
This work was supported by DOE (DE-SC0005162) to ERB. The authors would like to acknowledge Niraj Nepal and Geeta Nandyala for sample collection and their technical assistance. The abstract of this article was presented at the Experimental Biology 2015 as a poster presentation with interim findings. The poster’s abstract was published in FASEB journal. Asano et al (2015) Cerium oxide (CeO2) nanoparticle treatment ameliorates peritonitis induced diaphragm dysfunction. The actual article, however, has never been published.
Disclosure
The authors declare that there are no conflicts of interests regarding the publication of this article.
References
- DasSDowdingJMKlumpKEMcGinnisJFSelfWSealSCerium oxide nanoparticles: applications and prospects in nanomedicineNanomedicine2013891483150823987111
- NicoliniVGambuzziEMalavasiGEvidence of catalase mimetic activity in Ce(3+)/Ce(4+) doped bioactive glassesJ Phys Chem B2015119104009401925710332
- BabuKSAnandkumarMTsaiTYKaoTHInbarajBSChenBHCytotoxicity and antibacterial activity of gold-supported cerium oxide nanoparticlesInt J Nanomed2014955155531
- HirstSMKarakotiASinghSBio-distribution and in vivo antioxidant effects of cerium oxide nanoparticles in miceEnviron Toxicol201328210711821618676
- WangQPerezJMWebsterTJInhibited growth of Pseudomonas aeruginosa by dextran- and polyacrylic acid-coated ceria nanoparticlesInt J Nanomed2013833953399
- WasonMSZhaoJCerium oxide nanoparticles: potential applications for cancer and other diseasesAm J Transl Res20135212613123573358
- AliliLSackMvon MontfortCDownregulation of tumor growth and invasion by redox-active nanoparticlesAntioxid Redox Signal201319876577823198807
- HirstSMKarakotiASTylerRDSriranganathanNSealSReillyCMAnti-inflammatory properties of cerium oxide nanoparticlesSmall20095242848285619802857
- KongLCaiXZhouXNanoceria extend photoreceptor cell lifespan in tubby mice by modulation of apoptosis/survival signaling pathwaysNeurobiol Dis201142351452321396448
- NiuJAzferARogersLMWangXKolattukudyPECardioprotective effects of cerium oxide nanoparticles in a transgenic murine model of cardiomyopathyCardiovasc Res200773354955917207782
- SchoenbergMHWeissMRadermacherPOutcome of patients with sepsis and septic shock after ICU treatmentLangenbecks Arch Surg1998383144489627170
- AubierMTrippenbachTRoussosCRespiratory muscle fatigue during cardiogenic shockJ Appl Physiol Respir Environ Exerc Physiol19815124995086790504
- HussainSNRoussosCDistribution of respiratory muscle and organ blood flow during endotoxic shock in dogsJ Appl Physiol1985596180218084077788
- ScharfSMBarkHFunction of canine diaphragm with hypovolemic shock and beta-adrenergic blockadeJ Appl Physiol Respir Environ Exerc Physiol19845636486556142877
- SelvarajVManneNDArvapalliREffect of cerium oxide nanoparticles on sepsis induced mortality and NF-kappaB signaling in cultured macrophagesNanomedicine20151081275128825955124
- LiXMoodyMREngelDCardiac-specific overexpression of tumor necrosis factor-alpha causes oxidative stress and contractile dysfunction in mouse diaphragmCirculation2000102141690169611015349
- KhawliFAReidMBN-acetylcysteine depresses contractile function and inhibits fatigue of diaphragm in vitroJ Appl Physiol19947713173247961253
- ShindohCHidaWOhkawaraYTNF-alpha mRNA expression in diaphragm muscle after endotoxin administrationAm J Respir Crit Care Med19951525 Pt 1169016967582314
- Institute for Laboratory Animal ResearchGuide for the Care and Use of Laboratory Animals8th edWashington (DC)National Academies Press2011
- SharmaACMotewSJFariasSSepsis alters myocardial and plasma concentrations of endothelin and nitric oxide in ratsJ Mol Cell Cardiol1997295146914779201631
- SelvarajVNepalNRogersSInhibition of MAP kinase/NF-kB mediated signaling and attenuation of lipopolysaccharide induced severe sepsis by cerium oxide nanoparticlesBiomaterials20155916017125968464
- KolliMBManneNDParaRCerium oxide nanoparticles attenuate monocrotaline induced right ventricular hypertrophy following pulmonary hypertensionBiomaterials201435379951996225224369
- KrauseKMMoodyMRAndradeFHPeritonitis causes diaphragm weakness in ratsAm J Respir Crit Care Med19981574 Pt 1127712829563751
- NetheryDDiMarcoAStofanDSupinskiGSepsis increases contraction-related generation of reactive oxygen species in the diaphragmJ Appl Physiol19998741279128610517753
- CloseRIDynamic properties of mammalian skeletal musclesPhysiol Rev19725211291974256989
- KattaAThulluriSManneNDOverload induced heat shock proteins (HSPs), MAPK and miRNA (miR-1 and miR133a) response in insulin-resistant skeletal muscleCell Physiol Biochem2013312–321922923486111
- HayotMBarreiroEPerezACzaikaGComtoisASGrassinoAEMorphological and functional recovery from diaphragm injury: an in vivo rat diaphragm injuryJ Appl Physiol20019062269227811356792
- PetrofBJShragerJBStedmanHHKellyAMSweeneyHLDystrophin protects the sarcolemma from stresses developed during muscle contractionProc Natl Acad Sci U S A1993908371037148475120
- CallahanLASupinskiGSSepsis induces diaphragm electron transport chain dysfunction and protein depletionAm J Respir Crit Care Med2005172786186815994462
- el-DwairiQComtoisAGuoYHussainSNEndotoxin-induced skeletal muscle contractile dysfunction: contribution of nitric oxide synthasesAm J Physiol19982743 Pt 1C770C7799530109
- SupinskiGSWangWCallahanLACaspase and calpain activation both contribute to sepsis-induced diaphragmatic weaknessJ Appl Physiol200910751389139619661453
- LinMCEbiharaSEl DwairiQDiaphragm sarcolemmal injury is induced by sepsis and alleviated by nitric oxide synthase inhibitionAm J Respir Crit Care Med19981585 Pt 1165616639817721
- ComtoisASBarreiroEHuangPLMaretteAPerraultMHussainSNLipopolysaccharide-induced diaphragmatic contractile dysfunction and sarcolemmal injury in mice lacking the neuronal nitric oxide synthaseAm J Respir Crit Care Med2001163497798211282776
- CheemaIRHermannCPostellSBarnesPEffect of chronic excess of tumour necrosis factor-alpha on contractile proteins in rat skeletal muscleCytobios200010340416917611086712
- JiangJYangBHanGYangMLiSEarly administration of cisatracurium attenuates sepsis-induced diaphragm dysfunction in ratsInflammation201538130531125266883
- StaskoSAHardinBJSmithJDMoylanJSReidMBTNF signals via neuronal-type nitric oxide synthase and reactive oxygen species to depress specific force of skeletal muscleJ Appl Physiol2013114111629163623558387
- WilcoxPGWakaiYWalleyKRCooperDJRoadJTumor necrosis factor alpha decreases in vivo diaphragm contractility in dogsAm J Respir Crit Care Med19941505 Pt 1136813737952566
- YuXKennedyRHLiuSJJAK2/STAT3, not ERK1/2, mediates interleukin-6-induced activation of inducible nitric-oxide synthase and decrease in contractility of adult ventricular myocytesJ Biol Chem200327818163041630912595539
- BoczkowskiJLanoneSUngureanu-LongroisDDanialouGFournierTAubierMInduction of diaphragmatic nitric oxide synthase after endotoxin administration in rats: role on diaphragmatic contractile dysfunctionJ Clin Invest1996987155015598833903
- LeeSSSongWChoMAntioxidant properties of cerium oxide nanocrystals as a function of nanocrystal diameter and surface coatingACS Nano20137119693970324079896
- HasselgrenPOJamesJHBensonDWTotal and myofibrillar protein breakdown in different types of rat skeletal muscle: effects of sepsis and regulation by insulinMetabolism19893876346402661965
- SandriMSandriCGilbertAFoxo transcription factors induce the atrophy-related ubiquitin ligase atrogin-1 and cause skeletal muscle atrophyCell2004117339941215109499
- ReedSASandesaraPBSenfSMJudgeARInhibition of FoxO transcriptional activity prevents muscle fiber atrophy during cachexia and induces hypertrophyFASEB J2012263987100022102632
- SchakmanODehouxMBouchuariSRole of IGF-I and the TNFalpha/NF-kappaB pathway in the induction of muscle atrogenes by acute inflammationAm J Physiol Endocrinol Metab20123036E729E73922739109
- SmithIJAlamdariNO’NealPGonnellaPAversaZHasselgrenPOSepsis increases the expression and activity of the transcription factor Forkhead Box O 1 (FOXO1) in skeletal muscle by a glucocorticoid-dependent mechanismInt J Biochem Cell Biol201042570171120079455
- LangCHFrostRADifferential effect of sepsis on ability of leucine and IGF-I to stimulate muscle translation InitiationAm J Physiol Endocrinol Metab20042874E721E73015186995
- LangCHFrostRAEndotoxin disrupts the leucine-signaling pathway involving phosphorylation of mTOR, 4E-BP1, and S6K1 in skeletal muscleJ Cell Physiol2005203114415515389631
- SupinskiGSJiXWangWCallahanLAThe extrinsic caspase pathway modulates endotoxin-induced diaphragm contractile dysfunctionJ Appl Physiol200710241649165717218430