Abstract
Bacterial magnetosomes, because of their nano-scale size, have a large surface-to-volume ratio and are able to carry large quantities of bioactive substances such as enzymes, antibodies, and genes. Gangliosides, a family of sialic acid-containing glycosphingolipids, function as distinctive cell surface markers and as specific determinants in cellular recognition and cell-to-cell communication. Exogenously added gangliosides are often used to study biological functions, transport mechanisms, and metabolism of their endogenous counterparts. Absorption of gangliosides into cells is typically limited by their tendency to aggregate into micelles in aqueous media. We describe here a simple strategy to remove proteins from the magnetosome membrane by sodium dodecyl sulfate treatment, and efficiently immobilize a ganglioside (GM1 or GM3) on the magnetosome by mild ultrasonic treatment. The maximum of 11.7±1.2 µg GM1 and 11.6±1.5 μg GM3 was loaded onto 1 mg magnetosome, respectively. Complexes of ganglioside-magnetosomes stored at 4°C for certain days presented the consistent stability. The use of GM1-magnetosome complex resulted in the greatest enhancement of ganglioside incorporation by cells. GM3-magnetosome complex significantly inhibited EGF-induced phosphorylation of the epidermal growth factor receptor. Both of these effects were further enhanced by the presence of a magnetic field.
Introduction
Magnetic Fe3O4 nanoparticles, termed magnetosomes, are synthesized by Magneto-spirillum magneticum and related bacteria.Citation1 Magnetosome chains are enclosed by a lipid bilayer membrane with high content of transmembrane proteins.Citation2 Because of their high magnetization, monodispersity, and range of size (~35–120 nm), magnetosomes have many potential applications in biomedical diagnostics and nanoscience fields, notably the delivery of targeted drugs and genes and detection of pathogens.Citation3,Citation4 Their degradability and biocompatibility with a variety of cell types and structures make magnetosomes useful in many in vitro and in vivo applications.Citation5,Citation6
Glycosphingolipids, characteristic components of mammalian cell membranes, are involved in cell growth, adhesion, migration, and many other processes.Citation7,Citation8 Gangliosides, a family of glycosphingolipids that contain one or more sialic acids, are distinctive surface markers and often function as specific determinants in cellular recognition and cell-to-cell communication. Ganglioside GM3 affects receptor-associated tyrosine kinases of many cellular factors, including epidermal growth factor receptor (EGFR), platelet-derived growth factor, fibroblast growth factor, neuronal growth factor, and insulin.Citation9–Citation11 Ganglioside GM1, a major component of total ganglioside mixtures from mammalian brains, has been applied for therapy of a variety of neurological disorders.Citation12 Exogenously applied GM1 promotes neurite outgrowth following neural injury in several animal models.Citation13,Citation14 Nanocapsulized, chemically modified gangliosides have been developed and incorporated into various polymers or liposomes to improve delivery efficiency, increase blood circulation time, or stimulate immune responses.Citation15–Citation17 The primary obstacle to the administration of gangliosides to cells is their tendency to aggregate into micelles in aqueous media. In studies by Orlando et alCitation18 and Ghidoni et alCitation19, only 1%–3% of administered GM1 actually entered brain cells in mouse and rat models.Citation18,Citation19
We developed a novel strategy using bacterial magnetosomes to enhance cellular uptake of gangliosides. Magnetosomes were isolated and purified from Magnetospirillum gryphiswaldense,Citation20 proteins and lipopolysaccharides were removed from their membranes by sodium dodecyl sulfate (SDS) treatment, and specific gangliosides were grafted onto the modified magnetosome by mild ultrasonic treatment ().
Materials and methods
Cell lines and culture
Human epidermoid carcinoma cell line A431 and human mammary epithelial cell line MCF-10A were obtained from Cell Bank of the Chinese Academy of Sciences (Shanghai, People’s Republic of China). A431 and MCF-10A cells were cultured in Dulbecco’s Modified Eagle’s Medium containing 10% fetal bovine serum (FBS), 100 IU/mL penicillin, and 100 µg/mL streptomycin at 37°C in a 5% CO2 atmosphere. Human highly malignant, invasive bladder cancer cell line YTS-1 was a kind gift from Dr Sen-itiroh Hakomori (The Biomembrane Institute, Seattle, WA, USA). YTS-1 cells were cultured in RPMI 1640 medium supplemented with 10% FBS (Hyclone, Logan, UT, USA) and 1% penicillin/streptomycin at 37°C in a 5% CO2 atmosphere. As only cell lines were used in this study, no ethics approval was required.
Bacterial strain, gangliosides
Magnetospirillum gryphiswaldense strain MSR-1 (DSM6361) was a kind gift from Prof Ying Li (China Agricultural University, Beijing, People’s Republic of China). Gangliosides GM1 and GM3 were from Matreya LLC (Pleasant Gap, PA, USA).
Preparation of magnetosomes
MSR-1 cells were cultured as described previouslyCitation20 and collected by centrifugation at 8,000× g for 10 minutes at room temperature (RT). An aliquot (~1 g wet weight) was resuspended in 10 mL of 10 mM phosphate-buffered saline (PBS; pH 7.4) and disrupted by two passes through a French press (ATS Engineering Inc., Brampton, ON, Canada) at 1,000 psi. Magnetosomes were collected using neodymium-iron-boron (Nd-Fe-B) magnets and washed 8–10× with PBS in a mild ultrasonic bath (50 W; TY92-II; Xinyi, Ningbo, People’s Republic of China). Purified magnetosomes were lyophilized for 20 hours and stored at −20°C.
Preparation of ganglioside-magnetosomes
A 1 mg magnetosome sample was boiled with 1% SDS for 5 minutes to remove membrane proteins. The modified magnetosomes were isolated using a Nd-Fe-B magnet, washed 3× with 1 mL of 10 mM PBS (pH 7.4), and resuspended in 1 mL of 20 µg/mL GM3 solution in 10 mM PBS. The mixture was washed in an ultrasonic bath (100 W) for 10 minutes at 4°C and then incubated for 2 hours at RT. Resulting GM3-magnetosomes were washed with 10 mM PBS. GM3 concentration before and after reaction with magnetosomes was determined by high-performance thin layer chromatography (HPTLC). GM1-magnetosomes and control phosphatidylethanolamine-magnetosomes were prepared in a similar manner. Ganglioside-magnetosome complex was stored at 4°C, and sterilized by UV irradiation prior to experiments.
High-performance thin layer chromatography
Lipids were extracted from magnetosomes as described previously.Citation21 In brief, a 2 mg magnetosome sample was suspended in 950 mL chloroform-methanol solution (chloroform/methanol/distilled water 1:2:0.8), incubated for 2 hours, and lipid and non-lipid fractions were separated using a Nd-Fe-B magnet. The same volume of chloroform-methanol solution was used to extract equivalent amounts SDS-treated magnetosomes, GM1-magnetosomes, and GM3‒magnetosomes. Purified lipid fractions were subjected to HPTLC (silica gel 60 plates; Merck, Whitehouse Station, NJ, USA) with chloroform/methanol/water (65:35:4, v/v/v) as developing solvent, and visualized using iodine.
Transmission electron microscopy
Magnetosome samples were applied to carbon-coated 150-mesh copper grids (Nisshin EM Co., Tokyo, Japan) and dried at RT. Transmission electron microscopy (TEM) samples were prepared by drying a drop of solution in a dark room on amorphous carbon-coated nickel grids. Magnetosome size and morphology were observed by TEM (model H700-H; Hitachi, Tokyo, Japan).
Fourier-transform infrared spectroscopy
Samples were dispersed in distilled water. Dry particles were mixed with ground KBr crystals, and samples were pressed as pellets. Spectra were acquired using a Nicolet Nexus670 iS10 Fourier-transform infrared spectroscopy (Thermo Fisher Scientific, San Jose, CA, USA).
Protein capture by GM3-magnetosomes
GM3-magnetosomes (1 mg) were mixed with 1 mL A431 cell protein lysate (1 mg/mL), incubated overnight at 4°C, and washed 3× with Tris-buffered saline (+) (130 mM NaCl/10 mM Tris-HCl (pH 8.0) containing 0.9 mM CaCl2, 0.5 mM MgSO4, and 0.1 mM MnCl2). Protein bound to GM3‒magnetosomes was solubilized by boiling in 50 µL SDS/polyacrylamide gel electrophoresis (PAGE) sample buffer for 5 minutes, and analyzed by Western blotting.
Western blotting
GM3-magnetosomes were incubated with A431 cell lysate as above, mixed with boiling 1% SDS solution, and proteins interacting with the magnetosomes were extracted. Each extracted protein sample (30 mL) was mixed with SDS sample buffer (final concentration: 62.5 mM Tris-HCl, pH 6.8, 5% 2-mercaptoethanol, 2% SDS, 5% sucrose, 0.002% bromophenol blue), denatured, and subjected SDS-PAGE on 7.5% (w/v) polyacrylamide gel. Protein was transferred onto a polyvinylidene fluoride membrane and blotted with anti-EGFR antibody (dilution 1:1,000; Cell Signaling Technology, Beverly, MA, USA) and goat anti-rabbit antibody (dilution 1:5,000; Cell Signaling Technology). Signals were detected with a ChemiDoc XRS + system (Bio-Rad, Hercules, CA, USA).
Effect of GM3 on EGF-induced tyrosine phosphorylation of EGFR
A431 cells (1×105 per well) were cultured in 12-well plates in Dulbecco’s Modified Eagle’s Medium containing 10% FBS at 37°C until ~80% confluence. Cells were starved for 12 hours, incubated with GM3 or GM3-magnetosomes with/without magnetic field for 2 hours, stimulated with EGF for 5 minutes, washed in ice-cold PBS, and lysed in 100 µL lysis buffer. Protein in the supernatant (equal amount for each sample) was subjected to SDS-PAGE (7% gel) and Western blotting with primary anti-phosphorylated EGFR (pEGFR; dilution 1:1,000; Cell Signaling Technology) or anti-EGFR (dilution 1:1,000) antibody and horseradish peroxidase-conjugated anti-rabbit secondary antibody. Immunoreactive proteins were visualized using an ECL Kit (PA112-02; Tiangen, Beijing, People’s Republic of China).
Confocal laser scanning microscopy
YTS-1 cells (1×104 per well in 24-well plates) were cultured on sterilized coverslips (diameter 12 mm; Fisherbrand, San Jose, CA, USA) in RPMI 1640 until ~70% confluence, then cultured for 24 hours with PBS, GM1 plus magnetosomes (10 µg/mL), GM1-magnetosome complex (10 µg/mL), or GM1-magnetosome complex (10 µg/mL) under magnetic field, fixed with 4% fresh paraformaldehyde for 20 minutes, washed 3× with PBS, blocked with 5% bovine serum albumin in PBS (w/v) at RT for 2 hours, incubated with 0.4 µg/mL Fluorescein isothiocyanate (FITC)-labeled cholera toxin B subunit (CTB; specifically recognizes GM1; Sigma-Aldrich Co., St Louis, MO, USA), rinsed with PBS to remove excess dye, and stained with DAPI (5 µg/mL) in PBS for 30 minutes. Coverslips were mounted with Glycergel (DakoCytomation, Carpinteria, CA, USA) and observed by laser confocal fluorescence microscopy (model Eclipse Ti-U; Nikon, Tokyo, Japan).
Flow cytometry
YTS-1 cells (1×105 per well) were cultured in 12-well plates in RPMI 1640 until ~70% confluence, then cultured for 24 hours in fresh medium supplemented with PBS, GM1 (1 µg/mL) plus magnetosomes (10 µg/mL), GM1‒magnetosome complex (concentration of total complex added to medium =10 µg/mL; amount of GM1 per 1 mg magnetosome =0.104 µg), or GM1-magnetosome complex under magnetic field, digested with trypsin, washed with PBS, blocked with 5% bovine serum albumin/PBS for 1 hour, stained with FITC-labeled CTB for 1 hour at RT, and analyzed by flow cytometry (model Accuri C6; Becton Dickinson, Franklin Lakes, NJ, USA).
Statistical analysis
The data were analyzed by t-test using Prism 5 program. Differences in results were considered significant when P-value was ≤0.05.
Results
Characterization of ganglioside-magnetosome complexes
Removal of the proteins from the magnetosome membrane by SDS treatment is the first step to generate the ganglioside-magnetosome complexes. The released protein from magnetosome after SDS treatment was examined by the bicinchoninic acid method (). The highest concentration of released protein in the supernatant was reached in 5 minutes after SDS treatment. The membranes of SDS-treated and -untreated magnetosomes were then characterized by Fourier-transform infrared spectroscopy. SDS-treated magnetosomes showed lower signals of amide I at 1,656 cm−1 (from C=O stretching vibration), amide II at 1,537 cm−1 (from N–H bending vibration and C–N stretching vibration), and NH bending vibration at 3,282 cm−1 ().Citation22 These findings indicate that most proteins on the magnetosomes were removed successfully.
Figure 2 Characteristics of ganglioside-magnetosome complex.
Notes: (A) The protein released from magnetosome with SDS treatment for 30 seconds, 1, 3, 5, 7 and 10 minutes was determined by BCA assay. (B) FT-IR spectra of magnetosomes before and after SDS treatment. (Ci) Equivalent amounts of control magnetosomes (lane 1), SDS-treated magnetosomes (lane 2), GM1-magnetosomes (lane 3), and GM3-magnetosomes (lane 4) were extracted, subjected to HPTLC, and visualized as described in M&M/“high-performance thin layer chromatography”. Equivalent amounts of GM1-magnetosomes (Cii) or GM3-magnetosomes (Ciii) stored at 4°C for 2 (shown as 2d), 4 (4d), 6 (6d), and 8 (8d) days were extracted, subjected to HPTLC, and visualized as described in M&M/“high-performance thin layer chromatography”. (D) TEM imaging of four types of magnetosomes as in (B). Upper row: 200,000× magnification. Scale bar =50 nm. Lower row: close-ups of images in upper row.
Abbreviations: BCA, bicinchoninic acid; FT-IR, Fourier-transform infrared spectroscopy; HPTLC, high-performance thin layer chromatography; M&M, materials and methods; SDS, sodium dodecyl sulfate; TEM, transmission electron microscopy.
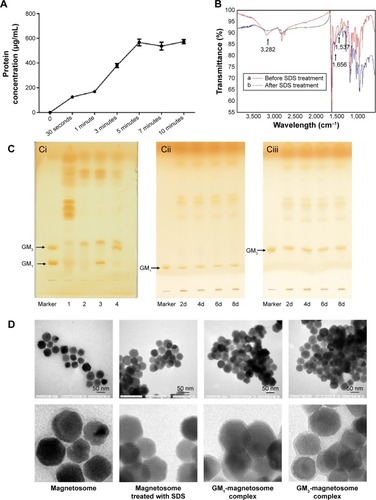
Protein removal by SDS treatment created more space on the magnetosome lipid membrane. The lipids were partially disrupted by SDS treatment (, lanes 1, 2). Incubation of gangliosides with magnetosomes in an ultrasonic bath resulted in insertion of ceramides (the hydrophobic portion of gangliosides) into the lipidic core of the membrane. Gangliosides inserted into the membrane were clearly detectable by HPTLC (, lanes 3, 4). After storage at 4°C for 2, 4, 6, or 8 days, gangliosides on the ganglioside-magnetosome complexes were extracted and clearly shown on a thin layer chromatography plate by HPTLC analysis (). TEM micrographs revealed that the membranes were made thinner or partially eliminated by the SDS treatment, but were restored by incubation with GM1 or GM3 (). The zeta potential of GM1-magnetosome complexes and GM3-magnetosome complexes were −17.84 and −14.84, respectively, and that obtained from magnetosomes was −42.18 (). Typical particle sizes of magnetosomes are ~30 nm, whilst the size of ganglioside-magnetosome reached at 150–200 nm (). These findings suggest that gangliosides were stably inserted into the outer membrane layer through lipid–lipid interaction.
We next investigated the binding capacity of gangliosides on magnetosomes. The binding capacity of coupled GM1 or GM3 on magnetosomes was maximal at 30 minutes incubation in an ultrasonic bath, whereas incubation for longer periods induced GM1 or GM3 degradation (), consistent with a previous report.Citation23 Maximal loading capacity of gangliosides was 11.7±1.2 µg per 1 mg magnetosome for GM1, and 11.6±1.5 µg per 1 mg magnetosome for GM3. The same ganglioside load was observed in both 50 and 100 µg gangliosides, indicating that the magnetosome loading capacity was saturated. We therefore used 50 µg gangliosides and 1 mg magnetosomes for reparation of ganglioside-magnetosome complexes in subsequent experiments. The magnetosomes and ganglioside-magnetosome complex were sterilized by ultrafiltration for following toxicity assay. Magnetosomes and the sterilized ganglioside-magnetosome complex showed no obvious toxic effect on cell growth (), consistent with previous studies.Citation4
Uptake of GM1-magnetosome complex by YTS-1 cells
Our working hypothesis was that the uptake of ganglioside into cells is promoted by the use of ganglioside–magnetosome complex to enhance ganglioside solubility and distribution. With bladder cancer cell line YTS-1 (low GM1 expressor)Citation24 as a model, we measured the cell surface expression of GM1 based on binding to CTB.Citation25 The incubation of YTS-1 cells with GM1-magnetosome complex significantly enhanced the fluorescence signal of FITC-labeled CTB for GM1 (), observed under laser confocal fluorescence microscopy. Cellular uptake of GM1-magnetosome complex was much higher under magnetic field (). The lower level of FITC signal for GM1 in YTS-1 cells was determined by flow cytometric analysis, whereas cellular uptake of GM1 contributed by GM1-magnetosome complex was significantly increased. And the magnetic field was able to further promote the uptake of GM1-magnetosome complex (). Notably, the fluorescence signal showed that a portion of GM was internalized inside the cells, it was resulted from that GM1 were ingested into YTS-1 cells together with magnetosomes, but not finalizing the transportation of GM1 to the outer of the plasma membranes. These findings indicate that the uptake of GM1-magnetosome complex by YTS-1 cells was enhanced by biocompatibility of the magnetosome membrane, and further enhanced by the presence of a magnetic field.
Figure 3 Uptake of GM1-magnetosome complex by YTS-1 cells.
Notes: YTS-1 cells were treated with PBS, GM1 plus magnetosomes, GM1-magnetosome complex, or GM1-magnetosome complex under magnetic field (A), as described in M&M. Expression of GM1 on the cell surface was determined by confocal laser scanning microscopy (B) and flow cytometric analysis (C).
Abbreviations: FITC, fluorescein isothiocyanate; PBS, phosphate-buffered saline; M&M, materials and methods.
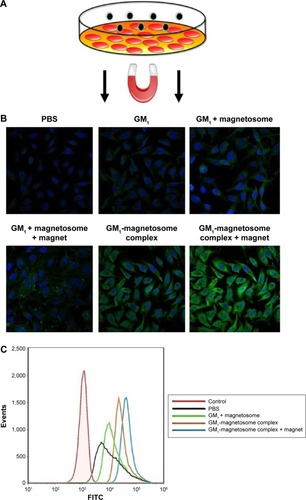
Inhibition of pEGFR by GM3‒magnetosome complex
Exogenous addition of gangliosides is a technique often used to elucidate the function of specific gangliosides as second messenger molecules or receptor modulators in cultured cells.Citation7,Citation26,Citation27 For example, exogenous GM3 blocks tyrosine phosphorylation of EGFR in human epidermoid carcinoma A431 and other cell lines.Citation28,Citation29 The absorption of gangliosides into cells is typically limited by their tendency to aggregate into micelles in aqueous media.Citation30 We examined the inhibitory effect of magnetosomes grafted with GM3 (GM3-magnetosome complex) on EGFR activation. A431 cells (high EGFR expressors) are often used for studying mechanisms whereby the EGFR signal pathway is turned on or off.Citation28 We confirmed the interaction of GM3 with EGFR using GM3-magnetosome complex. A431 cell lysate was incubated with the complex, and specific proteins binding to the complex were separated by magnetic field (). The EGFR protein in A431 cell lysate was clearly pulled down by GM3-magnetosome complex and confirmed by Western blotting, whereas phosphatidylethanolamine-magnetosomes complex had no such effect (). EGF stimulation activated pEGFR expression as expected, and such activation was inhibited by GM3. The inhibitory effect of GM3 on pEGFR activation was significantly enhanced by complexing with magnetosomes, especially together with the presence of a magnetic field ().
Figure 4 Effect of GM3 on EGF-induced tyrosine phosphorylation of EGFR.
Notes: (A) Technique (schematic) for evaluating interaction of GM3-magnetosomes with EGFR. (B) A431 cell lysate was incubated with PE-magnetosomes or GM3-magnetosomes, separated by a magnetic field, boiled in 50 µL sample buffer, subjected to SDS-PAGE, visualized by silver staining (left), or analyzed by Western blotting (right). Lane 1: A431 cell lysate. Lane 2: lysate incubated with PE-magnetosomes. Lane 3: lysate incubated with GM3-magnetosomes. Lane 4: protein bound to PE-magnetosomes. Lane 5: protein bound to GM3-magnetosomes. (C) Inhibitory effect of GM3 and GM3-magnetosomes on EGFR kinase activity in A431 cells. Amount of GM3 per 1 mg magnetosome =0.104 µg. 10*: concentration of total GM3-magnetosome complex added to medium =10 µg/mL. EGF-induced phosphorylation of EGFR was quantified as density of phospho-EGFR divided by that of EGFR, by densitometry using Image J. Experiments were performed in triplicate, and representative Western blot results are shown (left). Data are shown as mean ± SD (right). *P<0.05; ***P<0.001.
Abbreviations: EGF, epidermal growth factor; EGFR, epidermal growth factor receptor; p-EGFR, phosphorylated epidermal growth factor receptor; SDS-PAGE, sodium dodecyl sulfate-polyacrylamide gel electrophoresis.
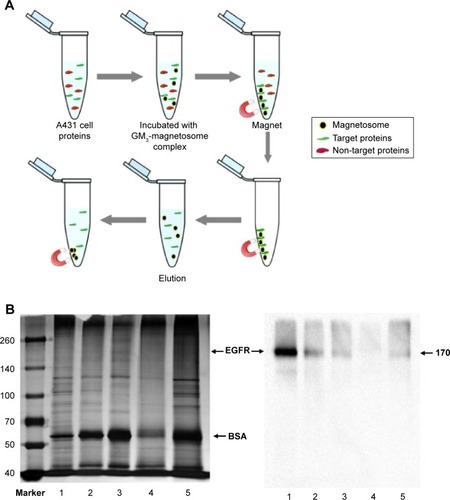
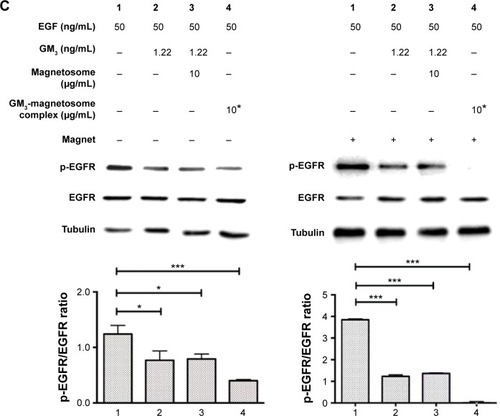
Discussion
The potential of liposomes as biodegradable or biocompatible drug carriers for enhancing the potency of therapeutics is well established. Magnetite nanoparticles are essential components for the development of many novel biotechnological and nanotechnological applications. Among various types, bacterial magnetosomes (synthesized by magnetotactic bacteria) are of particular interest because of their paramagnetism, nano-scale size, and unique biomembrane. The natural origin and magnetic characteristics of bacterial magnetosomes have proved to be useful in a variety of biotechnological applications, including enzyme immobilization, drug delivery, and target cell separation.Citation4,Citation21
Glycoconjugates, located primarily in cell membranes, play essential roles in protein modification, cell–cell interaction, cell adhesion, and many other biological processes.Citation31 In gangliosides, an important glycoconjugate family, one or more monosac-charides are glycosidically linked to a lipid moiety (ceramide). Gangliosides are usually synthesized on the membranes in the endoplasmic reticulum–Golgi pathway and delivered primarily to the outer leaflet of the plasma membranes. The lipid moiety is typically embedded in the cell membrane. The oligosaccharide moiety is extended (exposed) outside the bilayer membrane, and may interact simultaneously with one or more proteins or receptors. Various methods have been developed for studying protein–carbohydrate interactions, including 2-D glycoconjugate arrays on gold surfacesCitation32 and spherical arrays of carbohydrates as represented by glycodendrimers and liposomes.Citation33 Gangliosides (eg, GM1) can be incorporated in the liposome membrane, and the tendency to aggregate can be reduced by conjugation to hydrophilic polymers such as polyethylene glycol.Citation34
We describe here a simple strategy to remove proteins from the magnetosome membrane by SDS treatment, and immobilize a ganglioside (GM1 or GM3) on the magnetosome by mild ultrasonic treatment. Many evidence have shown that complexes that use magnetosomes as carriers can be easily ingested into cells through endocytosis process.Citation4,Citation35 In our study, gangliosides immobilized on magnetosomes was easily ingested into cells. GM1 immobilized on magnetosomes was easily taken up by cells. GM3-magnetosomes had a strong inhibitory effect on EGFR activation, also indicating increased absorption of GM3 by cells. In addition, because magnetosomes are comprised of Fe3O4, the magnetic field could accelerate the process that the particles entering into cells.Citation35,Citation36 Therefore, the effects described above were further enhanced by the presence of a magnetic field, because of the magnetic characteristics of magnetosomes. Our findings provide a basis for the application of magnetosomes in the delivery of specific gangliosides into cells, and the new strategy will be useful in studies of carbohydrate–protein interactions in vitro and in vivo.
Acknowledgments
This study was supported by Jiangsu Province Recruiting Plan for High-level, Innovative and Entrepreneurial Talents, the Fundamental Research Funds for the Central Universities (No JUSRP51319B), Jiangsu Province “Six Summit Talent” Foundation (2013-SWYY-019) and the 111 Project (No 111-2-06). The authors are grateful to Dr S Anderson for English editing of the paper.
Supplementary materials
Methods
Zeta potential and size distribution measurements.
Zeta potential of magnetosomes and ganglioside-magnetosome complexes were performed on a Zetasizer Nano ZS system (Malvern, UK) equipped with a standard laser at 633 nm. The size distribution of the formed complexes was analyzed using the above Zetasizer Nano ZS system.
Figure S2 MTT assay of ganglioside-magnetosome complexes.
Notes: (A) Human mammary epithelial MCF10A cells (1×103 per well in 96-well plates) were cultured in DMEM for 48 hours. Aliquots (10 µg/mL) of control magnetosomes, SDS-treated magnetosomes, GM1 + SDS-treated magnetosome mixture, GM1-magnetosome complex, GM3 + SDS-treated magnetosome mixture, and GM3-magnetosome complex were added to the culture medium, and culture was continued for 24 hours. Each well was added with 20 µL MTT solution and incubated 4 hours at 37°C. The reaction was stopped by addition of 700 µL DMSO, and absorption at OD595 was recorded immediately. Experiments were performed in triplicate, and data are shown as mean ± SD. (B) Magnetosomes, SDS-treated magnetosomes and 5, 10, and 20 µg/mL GM1-magnetosomes complex were added to YTS-1 cells, respectively,and cell viability assay was performed as described above. Experiments were performed in triplicate, and data are shown as mean ± SD. (C) Magnetosomes, SDS-treated magnetosomes and 5, 10, and 20 µg/mL GM3-magnetosomes complex were added to A431 cells, respectively, and cell viability assay was performed as described above. Experiments were performed in triplicate, and data are shown as mean ± SD.
Abbreviations: DMEM, Dulbecco’s Modified Eagle’s Medium; DMSO, dimethyl sulfoxide; MTT, 3-(4,5-dimethylthiazol-2-yl)-2,5-diphenyltetrazolium bromide; SDS, sodium dodecyl sulfate; SD, standard deviation.
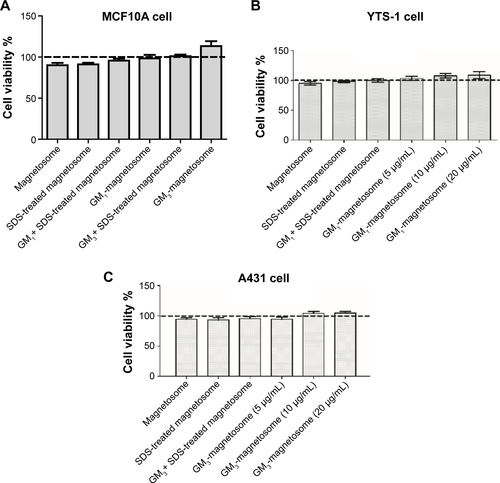
Table S1 Zeta potential of magnetosomes and ganglioside-magnetosome complexes
Table S2 Grafting efficiency of gangliosides onto magnetosomes
Disclosure
The authors report no conflicts of interest in this work.
References
- ArakakiANakazawaHNemotoMMoriTMatsunagaTFormation of magnetite by bacteria and its applicationJ R Soc Interface200852697799918559314
- TanakaMOkamuraYArakakiATanakaTTakeyamaHMatsunagaTOrigin of magnetosome membrane: proteomic analysis of magnetosome membrane and comparison with cytoplasmic membraneProteomics20066195234524716955514
- DunnillPLillyMDLetter: purification of enzymes using magnetic bio-affinity materialsBiotechnol Bioeng19741679879904606748
- XiangLBinWHualiJBacterial magnetic particles (BMPs)-PEI as a novel and efficient non-viral gene delivery systemJ Gene Med20079867969017605136
- NakamuraNHashimotoKMatsunagaTImmunoassay method for the determination of immunoglobulin G using bacterial magnetic particlesAnal Chem19916332682721824012
- SarikayaMAn introduction to biomimetics: a structural viewpointMicrosc Res Techn1994275360375
- van EchtenGSandhoffKGanglioside metabolism. Enzymology, Topology, and regulationJ Biol Chem19932688534153448449895
- SukKKimSKimYHIdentification of a novel human member of the DEAD box protein familyBiochim Biophys Acta200015011636910727850
- BremerEGHakomoriSBowen-PopeDFRainesERossRGanglioside-mediated modulation of cell growth, growth factor binding, and receptor phosphorylationJ Biol Chem198425911681868256327695
- MutohTTokudaAMiyadaiTHamaguchiMFujikiNGanglioside GM1 binds to the Trk protein and regulates receptor functionProc Natl Acad Sci U S A19959211508750917539142
- NojiriHStroudMHakomoriSA specific type of ganglioside as a modulator of insulin-dependent cell growth and insulin receptor tyrosine kinase activity. Possible association of ganglioside-induced inhibition of insulin receptor function and monocytic differentiation induction in HL-60 cellsJ Biol Chem19912667453145371999434
- AureliMMauriLCiampaMGGM1 ganglioside: past studies and future potentialMol Neurobiol Epub2015312
- CuelloACGlycosphingolipids that can regulate nerve growth and repairAdv Pharmacol1990211502265124
- TettamantiGRiboniLGangliosides and modulation of the function of neural cellsAdv Lipid Res1993252352678368150
- PolatoLBenedettiLMCallegaroLCouvreurPIn vitro evaluation of nanoparticle formulations containing gangliosidesJ Drug Target19942153598069584
- PuryearWBYuXRamirezNPReinhardBMGummuluruSHIV-1 incorporation of host-cell-derived glycosphingolipid GM3 allows for capture by mature dendritic cellsProc Natl Acad Sci U S A2012109197475748022529395
- HoshinoTMahmoodMIMoriKMatsuzakiKBinding and aggregation mechanism of amyloid beta-peptides onto the GM1 ganglioside-containing lipid membraneJ Phys Chem B2013117278085809423688073
- OrlandoPCoccianteGIppolitoGMassariPRobertiSTettamantiGThe fate of tritium labeled GM1 ganglioside injected in micePharmacol Res Commun1979119759773531082
- GhidoniRFiorilliATrincheraMVenerandoBChigornoVTettamantiGUptake, cell penetration and metabolic processing of exogenously administered GM1 ganglioside in rat brainNeurochem Int198915445546520504520
- XiangLWeiJJianboSGuiliWFengGYingLPurified and sterilized magnetosomes from Magnetospirillum gryphiswaldense MSR-1 were not toxic to mouse fibroblasts in vitroLett Appl Microbiol2007451758117594464
- YoshinoTHirabeHTakahashiMKuharaMTakeyamaHMatsunagaTMagnetic cell separation using nano-sized bacterial magnetic particles with reconstructed magnetosome membraneBiotechnol Bioeng2008101347047718421798
- WiSPancoskaPKeiderlingTAPredictions of protein secondary structures using factor analysis on Fourier transform infrared spectra: effect of Fourier self-deconvolution of the amide I and amide II bandsBiospectroscopy199842931069557904
- LawrenceMBSpringerTALeukocytes roll on a selectin at physiologic flow rates: distinction from and prerequisite for adhesion through integrinsCell1991658598731710173
- SatohMItoANojiriHEnhanced GM3 expression, associated with decreased invasiveness, is induced by brefeldin A in bladder cancer cellsInt J Oncol200119472373111562747
- CartonIMalininaLRichterRPDynamic modulation of the glycosphingolipid content in supported lipid bilayers by glycolipid transfer proteinBiophys J20109992947295621044592
- BremerEGSchlessingerJHakomoriSGanglioside-mediated modulation of cell growth. Specific effects of GM3 on tyrosine phosphorylation of the epidermal growth factor receptorJ Biol Chem19862615243424402418024
- KawamuraSOhyamaCWatanabeRGlycolipid composition in bladder tumor: a crucial role of GM3 ganglioside in tumor invasionInt J Cancer200194334334711745412
- YoonSJNakayamaKHikitaTHandaKHakomoriSIEpidermal growth factor receptor tyrosine kinase is modulated by GM3 interaction with N-linked GlcNAc termini of the receptorProc Natl Acad Sci U S A200610350189871899117142315
- HakomoriSIHandaKGM3 and cancerGlycoconj J2015321–21825613425
- CantuLDel FaveroEBroccaPCortiMMultilevel structuring of ganglioside-containing aggregates: from simple micelles to complex bio-mimetic membranesAdv Colloid Interface Sci201420517718624188828
- CrockerPRFeiziTCarbohydrate recognition systems: functional triads in cell-cell interactionsCurr Opin Struct Biol1996656796918913692
- HoranNYanLIsobeHWhitesidesGMKahneDNonstatistical binding of a protein to clustered carbohydratesProc Natl Acad Sci U S A19999621117821178610518527
- de La FuenteJMBarrientosAGRojasTCGold glyconano-particles as water-soluble polyvalent models to study carbohydrate interactionsAngew Chem Int Ed Engl200140122257226111433487
- LianTHoRJTrends and developments in liposome drug delivery systemsJ Pharm Sci200190666768011357170
- LeiHLiSYYongYZhaoFMJieHJinCResearch on the structure and performance of bacterial magnetic nanoparticlesJ Biomater Appl200822543344817623711
- AlphanderyEdouardChains of magnetosomes extracted from AMB-1 magnetotactic bacteria for application in alternative magnetic field cancer therapyACS Nano2011586279629621732678