Abstract
Introduction
The kidney is one of the main organs affected by microvascular damage wrought by hypertension. We developed an approach to investigate renal microcirculatory disturbance in live mice by measuring post-occlusive reactive hyperemia (PORH), a reactivity test exploring endothelial and neuro-microvascular functioning. Laser speckle-contrast analysis (LASCA) assesses microvascular blood flow; it provides real-time images of spatial and temporal blood flow dynamics. We compared basal blood flow and PORH test between control and angiotensin-II-treated mice (Ang-II) to validate the model.
Objective
The study objective was to develop an approach to investigate renal microcirculation, and then to compare microvascular reactivity assessed on LASCA in control versus Ang-II mice.
Methods
Thirty 7-week-old wild-type C57BL/6J mice were allocated into two groups. One received angiotensin-II via osmotic minipumps (Ang-II; n=15); the other served as control (n=15). Basal blood flow was measured on LASCA. The PORH test was then performed in the two groups.
Results
Control mice had significantly lower basal renal microcirculatory flow, expressed in perfusion units (PU), than Ang-II-treated mice (1448 ± 96 vs 1703 ± 185 PU, respectively; P < 0.05). Peak flow was lower in controls than in Ang-II mice (1617±104 vs.1724 ± 205 PU, respectively; P=0.21). Control mice had significantly higher kidney PORH than Ang-II mice (8±3 vs 1±4%, respectively; P < 0.05).
Conclusion
We developed an innovative technique to study renal microcirculation in mice. Ang-II-treated mice showed significantly higher basal blood flow than controls, while PORH was significantly higher in controls than in Ang-II mice.
Introduction
Hypertension is a significant stimulus for arteriolar remodeling and microvascular rarefaction.Citation1 Under physiological conditions, the fraction of non-perfused vessels constitutes a useful reserve in various pathophysiological conditions.Citation2 There is a dissociation between microvascular damage in hypertension and hemodynamic parameters:Citation3–Citation5 experimental studies showed distinct trajectories for macro- and micro-circulatory damage. Various organs may sustain microvascular damage induced by hypertension.Citation6 In humans, cutaneous circulation is easily accessible, and robust minimally invasive techniques are available to investigate skin microcirculation,Citation7 and this has often been used as a surrogate to evaluate microvascular damage in human subjects. Specifically, previous studies examined post-occlusive reactive hyperemia (PORH) as a means of assessing microvascular reactivity,Citation8 exploring endothelial and neuro-microvascular functioning.Citation8,Citation9 PORH can detect recruitment of initially non-perfused capillaries, and reflects both functional and structural parameters.Citation10 In essential hypertension, this recruitment is impaired.Citation2
Laser speckle-contrast analysis (LASCA) is a real-time non-contact technique with good spatiotemporal accuracy and good reproducibility for the assessment of PORH.Citation11
However, cutaneous microcirculation has certain anatomical and physiological specificitiesCitation8 that are distinct from the renal vascular bed. Specifically, the kidney is perfused with high blood flow and its self-regulating properties are distinct from those of the skin. The kidney is particularly exposed to microvascular damage, as high blood pressure induces early onset of microvascular impairment.Citation12 Thus, deficits in cutaneous circulation may not faithfully recapitulate renal damage. Consequently, it is of interest to develop techniques to study renal blood flow, which very few studies have examined.Citation13–Citation15 Moreover, the development of a robust approach to studying renal microcirculation in the mouse model will facilitate mechanistic studies of the pathways responsible for microvascular rarefaction in response to hypertension, by enabling use of genetically modified mice and experimental hypertension models.
The aim of the present study was therefore to develop a reliable technique to assess renal microvascular reactivity. We then compared 2 mouse populations to validate the model and its feasibility and to evaluate the difference in vascular perfusion. We used the well-established angiotensin-II-induced hypertensive mouse modelCitation16 and normotensive controls to compare renal microcirculation and microvascular reactivity between control and Ang-II-mice on LASCA. To our knowledge, renal microvascular reactivity in mouse has not previously been analyzed on LASCA.
Materials and Methods
Animal models: All animals were maintained in the Johns Hopkins University School of Medicine animal care facility according to the recommendations of the Animal Care and Use Committee, which approved all experimental procedures. Thirty wild-type C57BL/6J mice (Jackson Labs) were used. The 7-week-old mice were distributed 1:1 between 2 groups: one group received angiotensin II (Ang-II; 1 mg/kg/day n=15) via osmotic mini-pumps (Alzet model 1004, Durect Corp, Cupertino, CA) for 21 days, and the other, which did not, served as controls (n=15).
Osmotic pump implantation: Animals were anesthetized with 1.5% isoflurane, and the upper back and neck area were depilated. Under sterile conditions, a dorsal midline incision was made just below the neck and a subcutaneous pocket was created. The minipump containing Ang-II was inserted with its cap facing away from the incision. Local anesthetic was then injected in the suture area (bupivacaine 0.25%, up to 0.3mL); animals were provided with acetaminophen, and their recovery was monitored.
Renal hyperemic response on Laser Speckle Doppler: Mice were anesthetized with 2% isoflurane, positioned in right lateral decubitus on a heated pad, and maintained at 2% isoflurane. The left kidney was exposed through a small flank incision. The connective tissue anterior to the kidney and left renal artery was gently broken by blunt dissection, under binocular magnification. The kidney was externalized and carefully removed from the connective tissue, avoiding the adrenal gland and vessels. Next, the kidney was turned ventral side down into a lucite cup (Klaus Effenberger Med.-Techn. Gerätebau, Pfaffing, Germany) for imaging and to prevent image distortion due to respiratory movement, without exerting tension on the renal vessels ().
Figure 1 Set-up before measurement. (A) Mouse is placed in right lateral decubitus and the kidney is externalized and placed in a kidney cup. (B) Ventral face of left kidney and left renal artery (white arrow), which divides in two and travels above the renal vein.
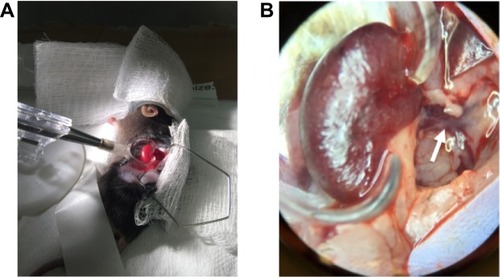
LASCA is a technique based on speckle-contrast analysis that provides an index of blood flow. The laser light illuminates the kidney and the diffusely backscattered light is collected on the screen. This interference pattern is called “speckle”. If the object, such as red blood cells, moves, the pattern will be dynamic. This is caused by the Doppler shift of the light interacting with the moving particles.
Cortical blood flow in the left kidney was then measured by a moorFLPI-2 Laser Speckle Contrast Imager (Moor Instruments Inc., Wilmington, DE), with the following settings: camera gain: 93; exposure time: 20 ms; time constant: 0.3 sec; mode: temporal; filter: 25 frames (1 sec/frame); sample interval: 1000 ms; image resolution: 752 x 580. The laser was positioned 12 cm above the region of interest, and zoom and focus were adjusted to acquire high-resolution images of the left kidney.
Blood-flow measurements: Basal blood-flow was first imaged for 15 sec. The PORH test was then performed by carefully clamping the left renal artery for 6 sec with iris forceps (Kent Scientific Corporation, Torrington, CT). The choice of 6 sec occlusion duration was guided by prior studiesCitation17 and based on successful ischemia confirmed visually by a marked decline in blood-flow (). Clamping the renal vein was avoided, as it could lead to venous congestion and increased capillary density.Citation10
Figure 2 Laser speckle-contrast imaging of the kidney. (A) Kidney before occlusion. (B) Drop in blood-flow during occlusion. (C) Example of degraded outflow. (D) Hemi-kidney not vascularized.
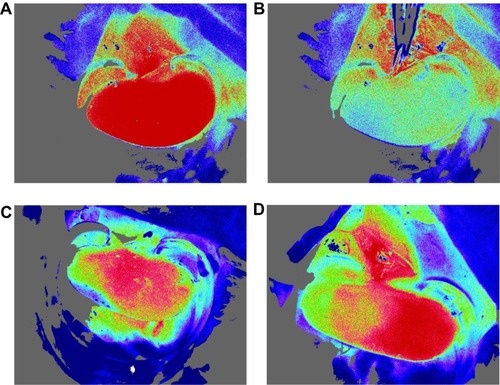
Laser Speckle-Contrast Analysis: The following data were recorded and stored, using PeriSoft software for Windows: 1) basal renal blood-flow; 2) peak renal blood-flow (ie, highest value recorded after ischemia); and 3) PORH, expressed as percentage change in peak flow above baseline: (peak flow – basal flow) x 100/basal flow. Flux vs time was analyzed offline by moorFLPI Review software (V3; Moor Instruments, Devon, UK). The region of interest (ROI) was set to correspond to kidney shape, avoiding proximity to any large blood vessels ().
Statistical analysis: Data were reported as mean ± standard deviation or box-and-whisker plots. The three horizontal lines shown in the boxes are the 25th, 50th and 75th percentiles; the two lines above and below each box are the highest and lowest values, respectively. The Wilcoxon rank-sum test was used to compare means between Ang-II-treated and control mice. All statistical analyses were performed with SPSS Statistics® software (IBM, NY). The significance threshold was set at P < 0.05.
Results
In the Ang-II cohort, 2 mice died 1 day after osmotic pump insertion. One Ang-II mouse presented immediate poor-quality blood-flow and was removed from analysis. Four mice (3 controls and 1 Ang-II) with severely degraded images after the occlusion test were also removed from analysis, including 2 control mice with non-vascularized hemi-kidney after releasing occlusion (). Analysis thus concerned 23 mice: 12 control and 11 Ang-II. Results are summarized in . Control mice had significantly lower basal renal microcirculatory flow than Ang-II-treated mice (1448 ± 96 vs 1703 ± 185 PU, respectively; P < 0.05) (). Peak flow was lower in controls than Ang-II mice (1617±104 vs.1724 ± 205 PU, respectively; P=0.21). Control mice had significantly higher kidney PORH than Ag-II mice (8±3 vs 1±4%, respectively; P < 0.05).
Figure 3 Basal blood-flow in control and Ang-II-treated mice. Data are presented as box plots. The three horizontal lines in the boxes are the 25th, 50th and 75th percentiles. The two lines outside the boxes represent the highest and lowest values. PU: perfusion unit. Asterisk indicates significant blood-flow differences at p < 0.05 level between control and Ang-II-treated mice.
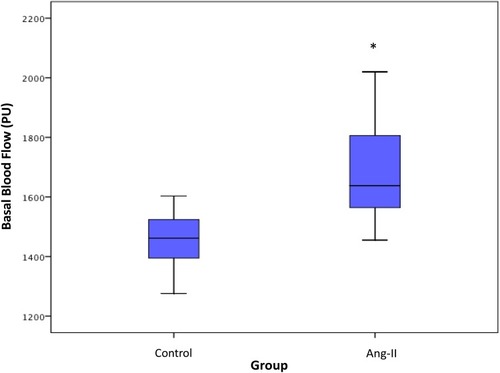
Table 1 Results of Laser Speckle-Contrast Analysis Measurements, in All 23 Mice, and in Control and Ang-II-Treated Mice. Data are Mean and Standard Deviation
Discussion
The present study describes a methodology to assess renal microvascular reactivity in mice. Results showed significantly higher renal basal microcirculatory flow in Ang-II-treated mice than in control mice, with higher PORH in controls than in Ang-II-treated mice. Renal microcirculation was studied as microvascular damage in the kidney is well known to occur with hypertension.
The cutaneous microcirculation is readily accessible and provides a powerful model to study human hypertension. Several vascular anatomical and physiological variables, however, differ between organs. Microcirculatory disturbance, for example, develops earlier in the skin than in skeletal muscle.Citation6 The skin has a higher density of nerve fibers than other tissues, which explains the major influence of neural control on skin microvascular reactivity.Citation8 Therefore, it is important to know whether findings in the skin can be directly extrapolated to other vascular beds. Some similarities in response and signaling mechanisms despite kinetic differences were revealed in a previous study, where renal vasodilatory response was evaluated using local pressure application and compared with cutaneous response.Citation15 Coulon et al demonstrated a correlation between renal resistive index (interlobular arteries) and skin PORH parameters on laser Doppler flowmetry (LDF) in hypertensive and normotensive subjects.Citation14 While it is clear that changes in microcirculation in both organs occur in parallel under hypertension, further studies are required to directly investigate renal microcirculation in hypertension, in order to determine whether the underlying cellular and molecular causal mechanisms in microvascular dysfunction are the same in these distinct vascular beds. Directly detecting renal microvascular damage is important for another reason: the link between blood pressure and microvascular damage is not well established. Capillary recruitment during post-occlusive reactive hyperemia has been found to correlate inversely with blood pressure.Citation18 However, blood pressure measurement does not exhaustively track kidney microvascular damage. Structural alterations occur before blood pressure rises in spontaneously hypertensive rats (SHR).Citation4 Rizzoni et al showed significant persistent regression of vascular hypertrophy after short calcium entry blocker treatment in SHRs, while blood pressure progressively increased.Citation5 ACE (angiotensin-converting enzyme) inhibitors can also limit microvascular changes in mesenteric small arteries, without any statistically significant hemodynamic effects being induced.Citation19
There are various ways of assessing renal microcirculation. Rodriguez-Porcel et al demonstrated impaired kidney response to vasodilators (acetylcholine and sodium nitroprusside), but they used electron-beam computed tomography, which is difficult to implement routinely.Citation20 LDF has been extensively used to assess renal microcirculation in mice in ischemia-reperfusion,Citation21,Citation22 preconditioning,Citation23 renal cryoinjuryCitation24 or other models.Citation25 LASCA and LDF both use laser speckle patterns, but the analysis techniques differ.Citation26 LASCA has been applied to skin, retina, mesenteric microcirculation and to monitor cerebral blood flow.Citation27 In humans, LASCA has been used to assess microcirculation changes in response to treatmentCitation28 or other stimuli.Citation29 It has been applied extensively in various mouse models, to assess neurological microcirculation,Citation30,Citation31 and for mesentericCitation32 and liver analysis in rats.Citation33 LASCA on the kidney surface has been described in rats.Citation34,Citation35 To our knowledge, LASCA has only once been reported for assessment of renal cortical blood flow in a dedicated hypertensive mouse model,Citation36 where a reduction in renal cortical blood flow was induced by Ang-II treatment. LASCA provides an index of blood flow,Citation37 with an arbitrary unit. Since absolute blood flow rates vary with temperature, heart rate and metabolic rate, a normalized approach is needed for measurement. The most relevant value is thus PORH, as it is a ratio, and was therefore used in our study.
Untreated and recently treated hypertensive patients showed significantly higher basal skin blood-flow than controls.Citation38,Citation39 Hypertension impairs myogenic tone.Citation1 Hypertensive patients fail to react by adequate vasodilatation on ischemia test (PORH).Citation6,Citation38,Citation40 PORH involves complex mechanosensitive and metabolic mechanisms.Citation41 Untreated hypertensive patients showed significantly lower skin PORH than controls.Citation38 Our results in the mouse kidney are in good agreement with these prior studies. After treatment, patients did not differ from controls in skin PORH,Citation38 and this remains to be evaluated in mouse models of hypertension.
The duration of ischemia is a critical factor to consider in measuring PORH.
Zatta and Headrickdemonstrated that multiple mediators contribute to coronary reactive hyperemia in mouse.Citation17 Using short (5–10 sec) or long (20–40 sec) occlusion durations, they determined that mechanisms involved in PORH depend on occlusion time. Coronary reactive hyperemia was graded, increasing with occlusion duration from 5 to 40 seconds. We therefore chose a short duration of 6 sec, also to avoid damage to the vessel with longer occlusion times. In humans, occlusion time is much longer.Citation38 Furthermore, PORH amplitude in our experimental set-up was lower than in human skinCitation38 or coronary amplitude.Citation17 This could be due 1) to arterial dissection (as noted in the vascularized hemi-kidney observed in 2 cases after occlusion test) or 2) to the use of forceps to study PORH amplitude. A model for renal ischemic preconditioning has been developed using a hanging-weight system for isolated renal artery occlusion;Citation42 this kind of system should enable precise occlusion and reduce any mechanical damage wrought by the use of forceps.
Conclusion
We described a PORH protocol assessing renal microvascular reactivity using LASCA as an efficient technique to assess renal microcirculation in mice. Ang-II-treated mice were used for comparison and validation of the model as a method to evaluate differences in vascular perfusion. We observed higher microvascular basal blood-flow but lower PORH in Ang-II-treated than control mice. This method can be combined with the use of various drugs and inhibitors to study specific mechanisms contributing to microvascular damage resulting from hypertension.
Ethics and Consent Statement
All animal protocols were approved by the Institutional Animal Care and Use Committee at Johns Hopkins University School of Medicine. Animals were maintained in the Johns Hopkins University School of Medicine animal care facility and were handled according to Animal Care and Use Committee recommendations.
Author Contributions
All authors made substantial contributions to conception and design, acquisition of data, or analysis and interpretation of data; took part in drafting the article or revising it critically for important intellectual content; gave final approval of the version to be published; and agree to be accountable for all aspects of the work.
Acknowledgments
We would like to warmly thank Sandeep Jandu, Hiroshi Matsushita, and Morgan Elliott of Johns Hopkins University for their technical assistance.
Disclosure
The authors report no conflicts of interest in this work.
Additional information
Funding
References
- Feihl F, Liaudet L, Waeber B, et al. Hypertension: a disease of the microcirculation? Hypertens Dallas Tex 1979. 2006;48:1012–1017.
- Serné EH, Gans RO, Ter Maaten JC, et al. Impaired skin capillary recruitment in essential hypertension is caused by both functional and structural capillary rarefaction. Hypertension. 2001;38:238–242.11509483
- Rizzoni D, Agabiti-Rosei C, Agabiti-Rosei E. Hemodynamic consequences of changes in microvascular structure. Am J Hypertens. 2017;30:939–946. doi:10.1093/ajh/hpx03228338956
- Rizzoni D, Castellano M, Porteri E, et al. Vascular structural and functional alterations before and after the development of hypertension in SHR. Am J Hypertens. 1994;7:193–200. doi:10.1093/ajh/7.2.1938179854
- Rizzoni D, Castellano M, Porteri E, et al. Delayed development of hypertension after short-term nitrendipine treatment. Hypertens. 1994;24:131–139.
- Jung F, Kolepke W, Spitzer S, et al. Primary and secondary microcirculatory disorders in essential hypertension. Clin Investig. 1993;71:132–138. doi:10.1007/BF00179994
- Holowatz LA, Thompson-Torgerson CS, Kenney WL. The human cutaneous circulation as a model of generalized microvascular function. J Appl Physiol (1985). 2008;105:370–372.17932300
- Roustit M, Cracowski J-L. Assessment of endothelial and neurovascular function in human skin microcirculation. Trends Pharmacol Sci. 2013;34:373–384. doi:10.1016/j.tips.2013.05.00723791036
- Taddei S, Virdis A, Ghiadoni L, et al. Endothelial dysfunction in hypertension. J Cardiovasc Pharmacol. 2001;38 Suppl 2:S11–S14. doi:10.1097/00005344-200111002-0000411811368
- Antonios TF, Rattray FE, Singer DR, et al. Maximization of skin capillaries during intravital video-microscopy in essential hypertension: comparison between venous congestion, reactive hyperaemia and core heat load tests. Clin Sci (Lond). 1999;97:523–528.10491353
- Roustit M, Millet C, Blaise S, et al. Excellent reproducibility of laser speckle contrast imaging to assess skin microvascular reactivity. Microvasc Res. 2010;80(3):505–511. doi:10.1016/j.mvr.2010.05.01220542492
- Chade AR. Small vessels, big role: renal microcirculation and progression of renal injury. Hypertension. 2017;69:551–563.28193706
- Stewart J, Kohen A, Brouder D, et al. Noninvasive interrogation of microvasculature for signs of endothelial dysfunction in patients with chronic renal failure. Am J Physiol Heart Circ Physiol. 2004;287:H2687–H2696. doi:10.1152/ajpheart.00287.200415297253
- Coulon P, Constans J, Gosse P. Impairment of skin blood flow during post-occlusive reactive hyperhemy assessed by laser doppler flowmetry correlates with renal resistive index. J Hum Hypertens. 2012;26:56–63. doi:10.1038/jhh.2010.11721248780
- Begey A-L, Liu KL, Lo M, et al. Cutaneous and renal vasodilatory response to local pressure application: a comparative study in mice. Microvasc Res. 2017;115:44–51. doi:10.1016/j.mvr.2017.08.00728859929
- Zimmerman MC, Lazartigues E, Sharma RV, et al. Hypertension caused by angiotensin II infusion involves increased superoxide production in the central nervous system. Circ Res. 2004;95(2):210–216. doi:10.1161/01.RES.0000135483.12297.e415192025
- Zatta AJ, Headrick JP. Mediators of coronary reactive hyperaemia in isolated mouse heart. Br J Pharmacol. 2005;144:576–587. doi:10.1038/sj.bjp.070609915655499
- Serné EH, Gans RO, Ter Maaten JC, et al. Capillary recruitment is impaired in essential hypertension and relates to insulin’s metabolic and vascular actions. Cardiovasc Res. 2001;49:161–168. doi:10.1016/S0008-6363(00)00198-X11121808
- Rizzoni D, Castellano M, Porteri E, et al. Effects of low and high doses of fosinopril on the structure and function of resistance arteries. Hypertension. 1995;26:118–123.7607714
- Rodriguez-Porcel M, Krier JD, Lerman A, et al. Combination of hypercholesterolemia and hypertension augments renal function abnormalities. Hypertension. 2001;37:774–780.11230372
- Shi H, Patschan D, Epstein T, et al. Delayed recovery of renal regional blood flow in diabetic mice subjected to acute ischemic kidney injury. Am J Physiol Renal Physiol. 2007;293(5):F1512–F1517. doi:10.1152/ajprenal.00215.200717881464
- Ikeda M, Swide T, Vayl A, et al. Estrogen administered after cardiac arrest and cardiopulmonary resuscitation ameliorates acute kidney injury in a sex- and age-specific manner. Crit Care Lond Engl. 2015;19:332.
- Oba T, Yasukawa H, Nagata T, et al. Renal nerve-mediated erythropoietin release confers cardioprotection during remote ischemic preconditioning. Circ J. 2015;79:1557–1567.25833080
- Abdulmahdi W, Zullo J, Nesi L, et al. Charting the course of renal cryoinjury. Physiol Rep. 2015;3.
- Yang R, Walther T, Gembardt F, et al. Renal vasoconstrictor and pressor responses to angiotensin IV in mice are AT1a-receptor mediated. J Hypertens. 2010;28:487–494. doi:10.1097/HJH.0b013e328334325019907343
- Fredriksson I, Larsson M. On the equivalence and differences between laser doppler flowmetry and laser speckle contrast analysis. J Biomed Opt. 2016;21:126018. doi:10.1117/1.JBO.21.12.12601828008449
- Briers D, Duncan DD, Hirst E, et al. Laser speckle contrast imaging: theoretical and practical limitations. J Biomed Opt. 2013;18:066018. doi:10.1117/1.JBO.18.6.06601823807512
- Binggeli C, Spieker LE, Corti R, et al. Statins enhance postischemic hyperemia in the skin circulation of hypercholesterolemic patients: a monitoring test of endothelial dysfunction for clinical practice? J Am Coll Cardiol. 2003;42:71–77. doi:10.1016/S0735-1097(03)00505-912849662
- Ruano J, Lopez-Miranda J, Fuentes F, et al. Phenolic content of virgin olive oil improves ischemic reactive hyperemia in hypercholesterolemic patients. J Am Coll Cardiol. 2005;46:1864–1868. doi:10.1016/j.jacc.2005.06.07816286173
- Dunn AK. Laser speckle contrast imaging of cerebral blood flow. Ann Biomed Eng. 2012;40:367–377. doi:10.1007/s10439-011-0469-022109805
- Tarantini S, Fulop GA, Kiss T, et al. Demonstration of impaired neurovascular coupling responses in TG2576 mouse model of Alzheimer’s disease using functional laser speckle contrast imaging. GeroScience. 2017;39:465–473. doi:10.1007/s11357-017-9980-z28578467
- Sand CA, Starr A, Wilder CDE, et al. Quantification of microcirculatory blood flow: a sensitive and clinically relevant prognostic marker in murine models of sepsis. J Appl Physiol Bethesda Md 1985. 2015;118:344–354.
- Sturesson C, Milstein DMJ, Post ICJH, et al. Laser speckle contrast imaging for assessment of liver microcirculation. Microvasc Res. 2013;87:34–40. doi:10.1016/j.mvr.2013.01.00423403398
- Ding C, Ren J, Zhou B, et al. Laser speckle contrast imaging for assessment of abdominal visceral microcirculation in acute peritonitis: does sequential impairments exist? Microvasc Res. 2014;95:26–30. doi:10.1016/j.mvr.2014.06.01125004449
- Postnov DD, Sosnovtseva O, Tuchin VV. Improved detectability of microcirculatory dynamics by laser speckle flowmetry. J Biophotonics. 2015;8:790–794. doi:10.1002/jbio.20150015226110702
- Pushpakumar S, Kundu S, Pryor T, et al. Angiotensin-II induced hypertension and renovascular remodelling in tissue inhibitor of metalloproteinase 2 knockout mice. J Hypertens. 2013;31:2270–2281; discussion 2281. doi:10.1097/HJH.0b013e3283649b33
- Draijer M, Hondebrink E, van Leeuwen T, et al. Review of laser speckle contrast techniques for visualizing tissue perfusion. Lasers Med Sci. 2009;24:639–651. doi:10.1007/s10103-008-0626-319050826
- Rossi M, Bradbury A, Magagna A, et al. Investigation of skin vasoreactivity and blood flow oscillations in hypertensive patients: effect of short-term antihypertensive treatment. J Hypertens. 2011;29(8):1569–1576. doi:10.1097/HJH.0b013e328348b65321720275
- Farkas K, Kolossváry E, Járai Z, et al. Non-invasive assessment of microvascular endothelial function by laser doppler flowmetry in patients with essential hypertension. Atherosclerosis. 2004;173(1):97–102. doi:10.1016/j.atherosclerosis.2003.11.01515177128
- Jung F, Pindur G, Ohlmann P, et al. Microcirculation in hypertensive patients. Biorheology. 2013;50:241–255. doi:10.3233/BIR-13064524398607
- Koller A, Bagi Z. On the role of mechanosensitive mechanisms eliciting reactive hyperemia. Am J Physiol Heart Circ Physiol. 2002;283:H2250–H2259. doi:10.1152/ajpheart.00545.200212427591
- Grenz A, Eckle T, Zhang H, et al. Use of a hanging-weight system for isolated renal artery occlusion during ischemic preconditioning in mice. Am J Physiol Ren Physiol. 2007;292:F475–F485. doi:10.1152/ajprenal.00275.2006