Abstract
Purpose
Asthma substantially affects the quality of life and health of children. Lipocalin 2 (LCN2) is an immune-related protein, which is predicted to be highly expressed in asthma. Here, we investigated the role of LCN2 in ovalbumin (OVA)-induced asthma mouse model.
Methods
We knocked down LCN2 in an asthma mouse model and performed histopathological analysis using hematoxylin and eosin (H&E) staining assay. Differentiated cells were assessed using Diff-Quick staining assay. We investigated the regulatory T (Treg) cell/ T helper 17 (Th17) cell balance using flow cytometry and enzyme-linked immunosorbent assay (ELISA). Inflammatory factors were measured using quantitative real-time reverse transcription PCR (qRT-PCR). The involved pathways were assessed using Western blotting.
Results
LCN2 was upregulated in patients with asthma. OVA promoted pathological deterioration in the lungs, increased IgE levels in the plasma, and elevated the number of differentiated inflammatory cells, whereas LCN2 knockdown abrogated the OVA-induced effects. Additionally, the Treg/Th17 imbalance and increased inflammatory cytokine levels were improved by LCN2 knockdown in OVA-treated mice. Moreover, LCN2 knockdown reversed the activation of the janus kinase (JNK) pathway.
Conclusion
LCN2 knockdown improved the Treg/Th17 balance, alleviated inflammation, and inactivated the JNK pathway in OVA-induced asthma mouse model, suggesting that LCN2 may be a novel therapeutic target for asthma in children.
Introduction
Asthma is a chronic respiratory disease characterized by airway inflammation and remodeling.Citation1 Patients with asthma usually present with cough, shortness of breath, and chest tightness. Asthma affects more than 300 million people, especially children; its prevalence in children below the age of 18 years is approximately 8%.Citation2,Citation3 Severe asthma causes poor outcomes in children, such as drug side effects, poor quality of life, and death.Citation4 Because asthma is a heterogeneous condition with different phenotypes, it cannot be completely cured despite the availability of several biologics that target type 2 inflammation.Citation5 Thus, the pathogenesis of asthma should be elucidated.
CD4+ T cells participate in the progression of asthma and determine the phenotype of asthma, ie, eosinophilic or neutrophilic.Citation6 Naïve CD4+ T cells differentiate into regulatory T (Treg) cells and T helper 17 (Th17) cells.Citation7,Citation8 Th17 cells induce inflammation, leading to autoimmune and inflammatory disorders, whereas the Treg cells act antagonistically and maintain immune homeostasis and prevent diseases.Citation9 Moreover, previous studies have reported Treg/Th17 imbalance during asthma. Therefore, reducing Treg/Th17 imbalance could be a potential strategy to attenuate asthma.Citation10,Citation11
Lipocalin 2 (LCN2), is associated with innate immunity. It functions as an iron regulator under inflammatory conditions.Citation12 In normal tissues, LCN2 protects against bacterial infection and oxidative stress damage. Moreover, LCN2 is expressed in several types of cells, such as macrophages, neutrophils, granulocytes, and epithelial cells.Citation13 LCN2 expression is high in CD4+ T cells, and it plays a crucial role in type 1 helper T (Th1) cell differentiation.Citation14 Previous studies have reported that LCN2 is involved in the pathological progression of many diseases, such as malignancy, cardiovascular disease, and inflammation.Citation13,Citation15,Citation16 Dysregulation of LCN2 has been observed in the serum and plasma of patients with asthma, indicating that LCN2 is associated with the development of asthma.Citation17,Citation18 However, the underlying mechanisms of LCN2 are not yet understood.
Therefore, in the present study, we investigated the role of LCN2 in the pathogenesis of asthma. We evaluated the effect of LCN2 knockdown on the histopathology, inflammatory cytokine levels, and Treg/Th17 balance on an asthma mouse model. The findings of this study suggest that LCN2 is a potential therapeutic target for asthma in children.
Materials and Methods
Microarray Analysis
The GSE31773 dataset was downloaded from the Gene Expression Omnibus (GEO) database (https://www.ncbi.nlm.nih.gov/geo/query/acc.cgi?acc=GSE31773). Abnormally expressed genes were analyzed using the R programming language. Differentially expressed genes were defined based on the following criteria: |log2(fold change)| >1 and P value <0.05.
Clinical Samples
This study was approved by the Ethics Committee of Liyang People’s Hospital (No. [2022]02-283-01). Thirty-three children (age: 3–12 years) diagnosed with asthma participated in this study. Patients with the following conditions were excluded from the study: other respiratory diseases, lung disease, allergic disease, history of asthma, or those taking drugs. Moreover, 33 age-matched healthy children were included in the control group. Fasting peripheral blood samples were collected from all participants. Written informed consent was obtained from the legal guardians of all participants. Blood samples were collected according to the Declaration of Helsinki.
Animal Study
The animal experiments were approved by the Ethics Committee of Liyang People’s Hospital (No. [2022]02-283-01) and were performed in accordance with the Guide for the Care and Use of Laboratory Animals (National Institutes of Health). Male BALB/c mice (4-week-old; Shanghai Laboratory Animal Centre, Shanghai, China) were housed in specific pathogen-free (SPF) environment, with 12-h light/12-h dark cycle, 23 ± 2°C, 50–60% humidity, and free water and food. The mice were randomly divided into the following four groups, with six mice per group: control, ovalbumin (OVA), OVA + sh-NC, and OVA + sh-LCN2. To establish the asthma model, OVA (25 μg; Sigma-Aldrich, St. Louis, MO, USA) and aluminum hydroxide (1 mg; Sigma-Aldrich) were dissolved in 200 μL of normal saline and intraperitoneally injected into the mice from OVA, OVA + sh-NC, and OVA + sh-LCN2 groups on days 0, 7, and 14. On day 15, the mice were injected with 5% OVA in the airway for 30 min every day using an ultrasonic sprayer until day 22. The control mice were sensitized using normal saline as per the abovementioned dosage of OVA. Lentiviruses carrying shRNA (sh)-negative control (NC) and sh-LCN2 (GenePharma, Shanghai, China) were intravenously injected into the mice from groups OVA + sh-NC, and OVA + sh-LCN2 before model establishment. All mice were sacrificed 23 d after peripheral blood collection. Bronchoalveolar lavage fluid (BALF) and lung tissue samples were obtained from all mice.
BALF Collection
After sacrificing the mice, the trachea was intubated using polyethylene tubing. An incision was made at the midline of the neck to expose the trachea. Tracheostomy was performed using a 14-gauge needle. Cold sterile phosphate-buffered saline (PBS; 0.5 mL) was added through the needle to the right lung of each mouse, and the lung was washed twice. The liquid obtained via bronchoalveolar lavage or BALF was collected and centrifuged at 500 × g for 10 min. The collected BALF was stored at −20°C until further use.
Hematoxylin and Eosin (H&E) Staining Analysis
The left lung tissue samples were fixed in 10% formalin for histopathological testing and cut into paraffin sections. After dewaxing and rehydration, the sections were stained using hematoxylin (Beyotime, Shanghai, China) for 5 min and eosin (Beyotime) for 3 min. The cells were visualized under a microscope (Olympus, Tokyo, Japan).
Inflammation score was quantified as the following criteriaCitation19: 0 point (no inflammatory cells), 1 point (inflammatory cells were observed occasionally), 2 points (1–3 layers of inflammatory cells around the bronchi), 3 points (4–5 layers of inflammatory cells around the bronchi or vessels), and 4 points (more than 5 layers of inflammatory cells around the bronchi or vessels).
Diff-Quick Staining Assay
The differentiated cells in the BALF were evaluated using a Diff-Quick stain kit (Solarbio, Beijing, China). Briefly, BALF was prepared as a BALF smear, which was incubated with Diff-Quick I and Diff-Quick II for 10 and 15s, respectively. The differentiated cells were observed under a microscope, and their number was counted. The total number of inflammatory cells was counted using a hemocytometer. The percentage of neutrophils, macrophages, eosinophils, and lymphocytes were calculated by the number of differentiated cells/total inflammatory cells *100%.
Flow Cytometry
For flow cytometry, peripheral blood mononuclear cells (PBMCs) were isolated from peripheral blood using Percoll solution (Sigma-Aldrich). After centrifugation at 800 × g for 30 min and washing with PBS, the PBMCs were maintained in Dulbecco’s Modified Eagle Medium (DMEM; Hyclone, South Logan, UT, USA) supplemented with 10% fetal bovine serum (FBS; Hyclone) at 37°C with 5% CO2.
To investigate Th17 cells, the PBMCs were stimulated with 25 ng/mL PMA, 1 mg/mL ionomycin, and 1 μg/mL BFA at 37°C for 5 h. Next, the samples were incubated with a fluorescein isothiocyanate (FITC)-conjugated anti-CD4 antibody at 4°C for 45 min, followed by incubation with a phycoerythrin (PE)-conjugated anti-interleukin-17 (IL-17) antibody for 30 min in the dark. To investigate Treg cells, CD4+ T cells were isolated using a CD4+ T-cell isolation kit (Novo Biotechnology, Beijing, China). The samples were incubated with an FITC-conjugated anti-CD25 antibody at 4°C for 45 min, followed by incubation with a PE-conjugated anti-forkhead box p3 (Foxp3) antibody for 30 min in the dark. Finally, all samples were analyzed using a flow cytometer. The antibodies were purchased from BioLegend (San Diego, CA, USA).
Enzyme-Linked Immunosorbent Assay (ELISA)
The levels of tumor growth factor beta (TGF-β), interleukin-10 (IL-10), IL-17, and interleukin-23 (IL-23) in the serum and the levels of immunoglobulin E (IgE) in the plasma of mice were measured using specific mouse ELISA kits for each molecule, according to the manufacturer’s protocol. All ELISA kits were purchased from Thermo Fisher Scientific (Waltham, MA, USA).
Quantitative Reverse Transcription PCR (qRT-PCR)
Total RNA was isolated from the peripheral blood and lung tissue samples using TRIzol reagent (Invitrogen, Carlsbad, CA, USA). RNA (1 μg) was reverse-transcribed to cDNA using PrimeScript RT Master Mix. qPCR was conducted using a real-time PCR master mix (SYBR Green). mRNA expression was calculated using the 2−ΔΔCT method and normalized to that of GAPDH. The primer sequences are shown in .
Table 1 The Primer Sequences Used in qRT-PCR
Western Blotting
Proteins were isolated from lung tissue samples of the mice using RIPA buffer (Beyotime). After the measurement of protein concentration using a BCA kit (Beyotime), equal amount of protein was added to sodium dodecyl sulfate-polyacrylamide gel electrophoresis (SDS-PAGE) for separation, followed by the transfer of the proteins to polyvinylidene fluoride (PVDF) membranes (Beyotime). The membranes were blocked with 5% not-fat milk at 22°C for 1 h, incubated with primary antibodies at 4°C overnight, and incubated with a secondary antibody at 22°C for 2 h. Each protein band was visualized using BeyoECL plus (Beyotime). The antibodies used are listed in .
Table 2 The Antibodies Used in Western Blot
Statistical Analysis
The data are shown as the mean ± standard deviation (SD) and were analyzed using GraphPad Prism 8.0 software. For comparison of multiple groups, one-way analysis of variance was used, and the comparison of two groups, Student’s t-test was used. Statistical significance was defined as P <0.05.
Results
LCN2 Was Highly Expressed in Patients with Asthma
To identify the genes involved in the pathogenesis of asthma, we investigated the differentially expressed genes using microarray analysis; several genes were upregulated or downregulated. LCN2 was upregulated in patients with severe asthma compared with that in healthy donors ( and ). Moreover, LCN2 levels in the peripheral blood samples of patients with asthma were higher than those in the healthy controls (). These results demonstrated that LCN2 expression increased in patients with asthma.
Figure 1 Lipocalin 2 (LCN2) is highly expressed in patients with asthma. (A) Differentially expressed genes predicted via the analysis of dataset GSE31773 are presented in the heatmap. Blue: low expression; red: high expression. (B) Differentially expressed genes predicted via the analysis of dataset GSE31773 are presented in the bubble diagram. Green: downregulated genes; red: upregulated genes. (C) LCN2 levels in the peripheral blood samples of children with asthma (n=33) and healthy controls (n=33). Data were analyzed using Student’s t-test. ***P<0.001.
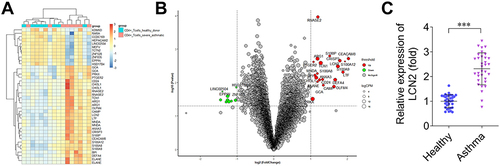
Knockdown of LCN2 Improved the Pathological Changes of Asthma
To investigate the role of LCN2 in the development of asthma, lentiviruses carrying sh-NC or sh-LCN2 were injected into the mice and LCN2 expression was assessed. The results revealed that LCN2 expression in the peripheral blood samples of the mice decreased following sh-LCN2 injection compared with that in the sh-NC group (). The plasma IgE levels increased after OVA treatment compared with those of the control mice; however, decrease in LCN2 levels reduced IgE levels in the OVA-administered groups (). H&E staining revealed that OVA caused lung wall thickening, inflammatory cell infiltration, and alveolar endothelial cell shedding. Nevertheless, LCN2 silencing reversed the lung damage induced by OVA (). Thus, LCN2 knockdown decreased plasma IgE levels and progressive pathology in asthmatic mice.
Figure 2 Knockdown of lipocalin 2 (LCN2) improves the pathological changes that occur due to asthma. (A) LCN2 levels in the peripheral blood samples of mice were measured using quantitative reverse transcription PCR (qRT-PCR) after sh-NC and sh-LCN2 were intravenously injected into the mice. (B) Plasma IgE levels in the mice of each group. (C) Histopathology of lung tissue samples was assessed using hematoxylin and eosin (H&E) staining. (magnification: 100×, scale bar: 200 μm; magnification: 400×, scale bar: 50 μm). (D) Inflammation score; n=6. Data were analyzed using Student’s t-test in (A) and using one-way ANOVA in (B–D). ***P<0.001. ###P<0.001.
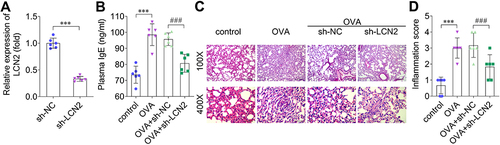
LCN2 Knockdown Regulated Differentiated Inflammatory Cells
Next, we stained the mice BALF samples using Giemsa, and counted the number of differentiated cells according to cell size. We observed that LCN2 knockdown reduced the percentage of OVA-induced neutrophils, macrophages, and eosinophils (); in contrast, OVA decreased the number of lymphocytes, whereas LCN2 knockdown reversed this effect (). Thus, LCN2 knockdown reduced the number of neutrophils, macrophages, and eosinophils, and increased the number of lymphocytes in OVA-treated mice.
LCN2 Knockdown Promoted Treg/Th17 Balance and Regulated Cytokine Levels
To evaluate the Treg/Th17 balance, we performed flow cytometry and measured the levels of Treg and Th17 cells. The results elucidated that OVA increased the number of Th17 cells and decreased that of Treg cells, whereas LCN2 knockdown counteracted this OVA-induced effect (). Thus, OVA reduced the Treg/Th17 cell ratio, which increased after LCN2 knockdown (). Serum TGF-β and IL-10 levels reduced in OVA-treated mice, and sh-LCN2 reversed this effect ( and ). Moreover, OVA elevated serum IL-17 and IL-23 levels, whereas LCN2 knockdown reversed this effect ( and ). The levels of the inflammation-related factors IL-5, IL-13, and IL-33 increased in the lung tissue and BALF samples of OVA-treated mice, and LCN2 knockdown decreased their levels (). Thus, LCN2 knockdown improved the Treg/Th17 imbalance in the serum samples of OVA-treated mice and inhibited inflammation in their lung tissue and BALF samples.
Figure 4 Lipocalin 2 (LCN2) promotes the Treg/Th17 balance and regulates cytokines levels. (A) Foxp3, which regulates regulatory T (Treg) cells, was investigated using flow cytometry and quantified in (B). (C) Interleukin-17 (IL-17), the regulator of T helper 17 (Th17) cells, was detected using flow cytometry and quantified in (D). (E) Treg/Th17 cell-ratio. The levels of (F) tumor growth factor beta (TGF-β), (G) interleukin-10 (IL-10), (H) IL-17, and (I) interleukin-23 (IL-23) were detected in the serum of mice from all groups using enzyme-linked immunosorbent assay (ELISA). The levels of (J) interleukin-5 (IL-5), (K) interleukin-13 (IL-13), and (L) interleukin-33 (IL-33) were examined in the lung tissue samples of mice using quantitative reverse transcription PCR (qRT-PCR). (M) IL-5, (N) IL-13, and (O) IL-33 expression in bronchoalveolar lavage fluid (BALF) of mice was measured using qRT-PCR; n=6. Statistical analysis was performed using one-way ANOVA. ***P<0.001. ###P<0.001.
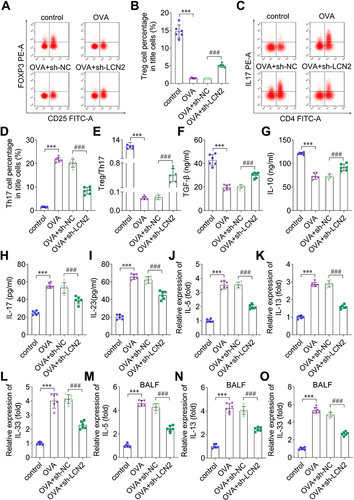
Knockdown of LCN2 Inactivated the Janus Kinase (JNK) Pathway
To evaluate the signaling pathways involved in the progression of asthma, we performed Western blotting and investigated several pathway-related factors. The results revealed that LCN2 knockdown did not affect the protein levels of PI3K/AKT, Wnt/β-catenin, and NF-κB pathway-related factors in the lung tissue samples of the mice (). Additionally, LCN2 knockdown downregulated p-JNK, but not JNK (). OVA upregulated p-JNK, whereas LCN2 lockdown abrogated this effect (). Thus, we demonstrated that LCN2 knockdown inhibited the activation of the JNK pathway in OVA-treated mice.
Figure 5 Knockdown of lipocalin 2 (LCN2) inactivates the janus kinase (JNK) pathway. Effects of LCN2 knockdown on the (A) PI3K/AKT pathway, (B) Wnt/β-catenin pathway, (C) NF-κB pathway, and (D) JNK pathway-related factors in lung tissue samples were evaluated using Western blotting. (E) Protein levels of p-JNK and JNK were detected in the lung tissue samples of mice from all groups using Western blotting; n=6.
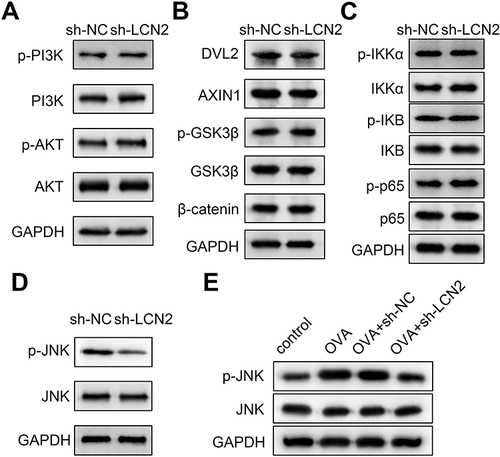
Discussion
Asthma is a common chronic disease affecting both adults and children. The pathogenesis of asthma is complex, which could be due to the interactions between various genes and the environment.Citation20 LCN2 is an important regulator of the immune response, which is upregulated in asthma. Moreover, because LCN2 is a biomarker of early intervention in asthma,Citation19 we investigated the role of LCN2 in asthma. IgE is a pro-allergic regulator, and IgE is commonly used for allergic asthma treatment.Citation21 In this study, we predicted differentially expressed genes in asthma and normal controls via microarray analysis. LCN2 was predicted to be upregulated in patients with asthma, which was confirmed via the analysis of clinical samples. Next, we elucidated that LCN2 knockdown inhibited IgE levels in the plasma of OVA-treated mice, suggesting that LCN2 reduces allergic reactions.
One of the hallmarks of asthma is airway inflammation; thus, inflammatory cells mediate asthma development. For example, eosinophil count increases in type 2 asthma; macrophages release allergenic cytokines; and neutrophils are associated with non-allergic asthma. The infiltration by these inflammatory cells causes the narrowing of the airway.Citation22,Citation23 Herein, we found that LCN2 knockdown reduced the counts of neutrophils, macrophages, and eosinophils and increased those of lymphocytes in OVA-treated mice, suggesting that downregulation of LCN2 improved the inflammation caused due to asthma.
Th17 cells promote inflammation, whereas Treg cells prevent inflammation. Therefore, the balance between Treg and Th17 cells is important for normal physiological processes, and an imbalance between them has been proven to be closely associated with inflammation.Citation24 Moreover, the Treg/Th17 balance regulates the development of neutrophilic, eosinophilic, or allergic asthma.Citation10,Citation11,Citation25 Thus, the Treg/Th17 balance plays an important regulatory in the pathogenesis of asthma. Foxp3, TGF-β, and IL-10 are regulators of Treg cells, and IL-17 and IL-23 are regulators of Th17 cells.Citation26 In the present study, OVA decreased Treg cell count and reduced the levels of TGF-β and IL-10. Additionally, OVA increased Th17 cell count and IL-17 and IL-23 levels in the mice serum samples. LCN2 knockdown abrogated the OVA-induced Treg/Th17 imbalance. Moreover, we investigated the inflammatory factors in the lung tissue samples of mice. IL-5, IL-13, and IL-33 are type 2 cytokines that increase eosinophilia and mucus in the airway and promote IgE production.Citation27,Citation28 We found that the LCN2 knockdown decreased the OVA-induced elevation in IL-5, IL-13, and IL-33 levels in the lung tissue samples of mice. Thus, these findings suggest that LCN2 knockdown inhibits OVA-induced Treg/Th17 imbalance and inflammation.
The JNK pathway is involved in biological processes, including inflammation, cell differentiation, proliferation, and apoptosis.Citation29 It is activated under various stress conditions.Citation30 In asthma, the JNK pathway regulates airway inflammation.Citation31,Citation32 Moreover, LCN2 mediates the JNK pathway in several diseases, such as cancer and acute liver injury.Citation33,Citation34 However, the effect of LCN2 on the JNK pathway in asthma is unclear. We elucidated that LCN2 knockdown only inhibited the activation of the JNK pathway and not that of the PI3K/AKT,-catenin, and NF-κB pathways. These data suggested that the JNK pathway participates in the progression of LCN2-mediated asthma.
Conclusions
Therefore, this is the first study to reveal that LCN2 knockdown inhibits inflammation, Treg/Th17 imbalance, and JNK pathway activation in OVA-induced asthma mouse model, suggesting that LCN2 silencing could relieve asthma, and LCN2 is a potential new target for asthma treatment.
Abbreviations
BALF, bronchoalveolar lavage fluid; DMEM, Dulbecco’s Modified Eagle Medium; ELISA, enzyme-linked immunosorbent assay; FITC, fluorescein isothiocyanate; Foxp3, forkhead box p3; GEO, Gene Expression Omnibus; IgE, immunoglobulin E; IL-10, interleukin-10; IL-17, interleukin-17; IL-23, interleukin-23; LCN2, lipocalin 2; MSFI, migration stimulating factor inhibitor; NGAL, neutrophil gelatinase-associated lipocalin; OVA, ovalbumin; PBS, phosphate-buffered saline; PBMCs, peripheral blood mononuclear cells; PE, phycoerythrin; PVDF, polyvinylidene fluoride; SDS-PAGE, sodium dodecyl sulfate-polyacrylamide gel electrophoresis; SPF, specific pathogen-free; TGF-β, tumor growth factor beta.
Data Sharing Statement
The datasets used and/or analyzed during the current study are available from the corresponding author on reasonable request.
Ethics Approval and Informed Consent
The study was approved by the Ethics Committee of LiYang People’s Hospital (No. [2022]02-283-01). Informed consent was obtained from all individual participants included in the study. All animal experiments should comply with the ARRIVE guidelines.
Disclosure
The authors declare there is no conflicts of interest in this work.
Additional information
Funding
References
- Chung KF, Dixey P, Abubakar-Waziri H, et al. Characteristics, phenotypes, mechanisms and management of severe asthma. Chin Med J (Engl). 2022;135(10):1141–1155. doi:10.1097/CM9.0000000000001990
- Zahran HS, Bailey CM, Damon SA, Garbe PL, Breysse PN. Vital Signs: asthma in Children - United States, 2001-2016. MMWR Morb Mortal Wkly Rep. 2018;67(5):149–155. doi:10.15585/mmwr.mm6705e1
- GBD 2015 Chronic Respiratory Disease Collaborators. Global, regional, and national deaths, prevalence, disability-adjusted life years, and years lived with disability for chronic obstructive pulmonary disease and asthma, 1990-2015: a systematic analysis for the Global Burden of Disease Study 2015. Lancet Respir Med. 2017;5(9):691–706. doi:10.1016/S2213-2600(17)30293-X
- Haktanir Abul M, Phipatanakul W. Severe asthma in children: evaluation and management. Allergol Int. 2019;68(2):150–157. doi:10.1016/j.alit.2018.11.007
- Pijnenburg MW, Fleming L. Advances in understanding and reducing the burden of severe asthma in children. Lancet Respir Med. 2020;8(10):1032–1044. doi:10.1016/S2213-2600(20)30399-4
- Jeong J, Lee HK. The Role of CD4+ T Cells and Microbiota in the Pathogenesis of Asthma. Int J Mol Sci. 2021;22(21):11822. doi:10.3390/ijms222111822
- Lee GR. The Balance of Th17 versus Treg Cells in Autoimmunity. Int J Mol Sci. 2018;19(3):730. doi:10.3390/ijms19030730
- Yan JB, Luo MM, Chen ZY, He BH. The Function and Role of the Th17/Treg Cell Balance in Inflammatory Bowel Disease. J Immunol Res. 2020;2020:8813558. doi:10.1155/2020/8813558
- Cai F, Jin S, Chen G. The Effect of Lipid Metabolism on CD4+ T Cells. Mediators Inflamm. 2021;2021:6634532. doi:10.1155/2021/6634532
- Zhou Y, Hu L, Zhang H, et al. Guominkang formula alleviate inflammation in eosinophilic asthma by regulating immune balance of Th1/2 and Treg/Th17 cells. Front Pharmacol. 2022;13:978421. doi:10.3389/fphar.2022.978421
- Wei C, Huang L, Zheng Y, Cai X. Selective activation of cannabinoid receptor 2 regulates Treg/Th17 balance to ameliorate neutrophilic asthma in mice. Ann Transl Med. 2021;9(12):1015. doi:10.21037/atm-21-2778
- Xiao X, Yeoh BS, Vijay-Kumar M. Lipocalin 2: an Emerging Player in Iron Homeostasis and Inflammation. Annu Rev Nutr. 2017;37:103–130. doi:10.1146/annurev-nutr-071816-064559
- Xu WX, Zhang J, Hua YT, Yang SJ, Wang DD, Tang JH. An Integrative Pan-Cancer Analysis Revealing LCN2 as an Oncogenic Immune Protein in Tumor Microenvironment. Front Oncol. 2020;10:605097. doi:10.3389/fonc.2020.605097
- Chen W, Li W, Zhang Z, et al. Lipocalin-2 Exacerbates Lupus Nephritis by Promoting Th1 Cell Differentiation. J Am Soc Nephrol. 2020;31(10):2263–2277. doi:10.1681/ASN.2019090937
- Jang HM, Lee JY, An HS, et al. LCN2 deficiency ameliorates doxorubicin-induced cardiomyopathy in mice. Biochem Biophys Res Commun. 2022;588:8–14. doi:10.1016/j.bbrc.2021.12.048
- Ma J, Chen J, Xue K, et al. LCN2 Mediates Skin Inflammation in Psoriasis through the SREBP2‒NLRC4 Axis. J Invest Dermatol. 2022;142(8):2194–2204.e11. doi:10.1016/j.jid.2022.01.012
- Hong L, Herjan T, Bulek K, et al. Mechanisms of Corticosteroid Resistance in Type 17 Asthma. J Immunol. 2022;209(10):1860–1869. doi:10.4049/jimmunol.2200288
- Kawagoe J, Kono Y, Togashi Y, et al. Serum Neutrophil Gelatinase-associated Lipocalin (NGAL) Is Elevated in Patients with Asthma and Airway Obstruction. Curr Med Sci. 2021;41(2):323–328. doi:10.1007/s11596-021-2350-1
- Louten J, Mattson JD, Malinao MC, et al. Biomarkers of disease and treatment in murine and cynomolgus models of chronic asthma. Biomark Insights. 2012;7:87–104. doi:10.4137/BMI.S9776
- Papi A, Brightling C, Pedersen SE, Reddel HK. Asthma. Lancet. 2018;391(10122):783–800. doi:10.1016/S0140-6736(17)33311-1
- Bush RK. The use of anti-IgE in the treatment of allergic asthma. Med Clin North Am. 2002;86(5):1113–1129. doi:10.1016/s0025-7125(02)00036-6
- Boonpiyathad T, Sözener ZC, Satitsuksanoa P, Akdis CA. Immunologic mechanisms in asthma. Semin Immunol. 2019;46:101333. doi:10.1016/j.smim.2019.101333
- Nakagome K, Nagata M. Involvement and Possible Role of Eosinophils in Asthma Exacerbation. Front Immunol. 2018;9:2220. doi:10.3389/fimmu.2018.02220
- Hanidziar D, Koulmanda M. Inflammation and the balance of Treg and Th17 cells in transplant rejection and tolerance. Curr Opin Organ Transplant. 2010;15(4):411–415. doi:10.1097/MOT.0b013e32833b7929
- Wang L, Wan H, Tang W, et al. Critical roles of adenosine A2A receptor in regulating the balance of Treg/Th17 cells in allergic asthma. Clin Respir J. 2018;12(1):149–157. doi:10.1111/crj.12503
- Talaat RM, Mohamed SF, Bassyouni IH, Raouf AA. Th1/Th2/Th17/Treg cytokine imbalance in systemic lupus erythematosus (SLE) patients: correlation with disease activity. Cytokine. 2015;72(2):146–153. doi:10.1016/j.cyto.2014.12.027
- Lambrecht BN, Hammad H, Fahy JV. The Cytokines of Asthma. Immunity. 2019;50(4):975–991. doi:10.1016/j.immuni.2019.03.018
- Drake LY, Kita H. IL-33: biological properties, functions, and roles in airway disease. Immunol Rev. 2017;278(1):173–184. doi:10.1111/imr.12552
- Kumar A, Singh UK, Kini SG, et al. JNK pathway signaling: a novel and smarter therapeutic targets for various biological diseases. Future Med Chem. 2015;7(15):2065–2086. doi:10.4155/fmc.15.132
- Cui J, Zhang M, Zhang YQ, Xu ZH. JNK pathway: diseases and therapeutic potential. Acta Pharmacol Sin. 2007;28(5):601–608. doi:10.1111/j.1745-7254.2007.00579.x
- Huang G, Su J, Zhao W, et al. JNK modulates RAGE/β-catenin signaling and is essential for allergic airway inflammation in asthma. Toxicol Lett. 2021;336:57–67. doi:10.1016/j.toxlet.2020.10.002
- Jin X, Jin W, Li G, Zheng J. Zeaxanthin attenuates OVA-induced allergic asthma in mice by regulating the p38 MAPK/β-catenin signaling pathway. Allergol Immunopathol. 2022;50(5):75–83. doi:10.15586/aei.v50i5.664
- Schröder SK, Asimakopoulou A, Tillmann S, Koschmieder S, Weiskirchen R. TNF-α controls Lipocalin-2 expression in PC-3 prostate cancer cells. Cytokine. 2020;135:155214. doi:10.1016/j.cyto.2020.155214
- Borkham-Kamphorst E, van de Leur E, Zimmermann HW, et al. Protective effects of lipocalin-2 (LCN2) in acute liver injury suggest a novel function in liver homeostasis. Biochim Biophys Acta. 2013;1832(5):660–673. doi:10.1016/j.bbadis.2013.01.014