Abstract
Background
Recombinant von Willebrand factor (rVWF, vonicog alfa) is a purified VWF concentrate produced from Chinese hamster ovary cells. rVWF is not exposed to the VWF-cleaving protease ADAMTS13 and so is not subject to proteolytic degradation of large (L) and ultra-large (UL) VWF multimers by that enzyme.
Purpose
To compare the structure and function of rVWF with the human plasma-derived VWF [pdVWF] concentrates Haemate P®/Humate-P®, Voncento®, Wilate®/Eqwilate®, and Wilfactin®/Willfact®; to investigate the relationship between VWF multimeric pattern and VWF:ristocetin cofactor (VWF:RCo) activity through population pharmacokinetic (PK) modeling in patients with severe von Willebrand disease (VWD) treated with rVWF.
Methods
Analyses included VWF:RCo activity, VWF:collagen-binding activity, VWF:platelet glycoprotein Ib receptor binding, factor VIII (FVIII) binding capacity, and VWF-mediated platelet adhesion under flow conditions. VWF multimeric structure was determined by agarose gel electrophoresis. Population PK models describing the activity-time profile of small, medium, and L/UL multimers following intravenous administration of rVWF in patients with severe VWD were developed.
Results
Findings demonstrate that rVWF contains a non-degraded VWF multimer pattern including the UL multimers not present in pdVWF concentrates. rVWF displayed higher specific platelet-binding activity, and faster mediation of platelet adhesion to collagen under shear stress versus pdVWF concentrates. rVWF also demonstrated higher FVIII binding capacity than Haemate P®, Voncento® and Wilate®. Modeling provided evidence that VWF:RCo activity in patients with severe VWD treated with rVWF is associated with L/UL VWF multimers in the circulation.
Conclusions
Findings suggest that the L and UL multimers preserved in rVWF contribute to high biological activity and might be important for providing hemostatic efficacy.
Introduction
von Willebrand factor (VWF) is a large adhesive glycoprotein synthesized in endothelial cells (ECs) and megakaryocytes.Citation1 Following post-translational modifications in the Golgi apparatus, up to 95% of VWF is constitutively secreted into the plasma and the remainder is stored in EC Weibel-Palade bodies or in the α-granules of platelets.Citation1 VWF is essential for hemostasis: under high shear flow it mediates initial platelet adhesion by acting as a bridge between subendothelial collagen and platelet surface glycoproteins. VWF also functions as a carrier for factor VIII (FVIII), protecting it from rapid clearance and increasing its plasma half-life.Citation2,Citation3
Plasma VWF circulates as a series of multimers of different sizes that are comprised of identical subunits linked by disulfide bonds. The linking of two subunits produces a dimer of approximately 500 kDa; these are further combined with disulfide bonds to produce multimers ranging in size from 500 to over 10,000 kDa.Citation4–6 In healthy individuals, ultra-large (UL) multimers (>10,000 KDa, >20 mers) are secreted into the plasma and are degraded by the naturally occurring VWF protease ADAMTS13.Citation7 Large (L) multimers (5500–10,000 KDa, 11–20 mers) and UL multimers are the most hemostatically active, as measured by binding capacity for collagen and platelet receptors, and platelet aggregation under conditions of high fluid shear.Citation8–12
von Willebrand disease (VWD) is an inherited bleeding disorder caused by a deficiency of VWF or functional or structural defects in the protein.Citation13,Citation14 Management of bleeding in VWD involves the mainstay treatments of desmopressin (1-deamino-8-D-arginine vasopressin) and replacement therapy with VWF-containing concentrates from human plasma (plasma-derived VWF [pdVWF]). However, desmopressin is not effective in severe VWD, as well as in certain subtypes of VWD, and is associated with tachyphylaxis.Citation15,Citation16 VWF-containing concentrates from human plasma vary in the content and composition of VWF, FVIII, and other constituents.Citation3,Citation17,Citation18 Recombinant VWF (rVWF, vonicog alfa, Vonvendi® [US]/Veyvondi® [Europe]; Baxalta US Inc., a Takeda company, Lexington, MA, USA) is a replacement therapy approved for on-demand treatment and control of bleeding episodes, as well as perioperative management of bleeding in adults diagnosed with VWD.Citation19,Citation20 In the US, rVWF has also been approved by the FDA for routine prophylaxis to reduce the frequency of bleeding episodes in adult patients with severe type 3 VWD receiving on-demand therapy.Citation19 It is a purified VWF protein produced in Chinese hamster ovary cells without the addition of any exogenous human- or animal-derived protein.Citation21 At administration, rVWF has an intact multimer pattern, including UL multimers, because it is not exposed to proteolytic degradation during manufacture, particularly by ADAMTS13.Citation22
Although comparisons of pdVWF and rVWF concentrates have been published,Citation3,Citation23–26 a comprehensive analysis of multimers and functional characteristics of VWF products is lacking. The objective of this study was to conduct a structural and functional comparison of rVWF versus the clinically used pdVWF (Wilfactin®/Willfact®) and pdFVIII/VWF (Haemate P®/Humate-P®, Voncento®, Wilate®/Eqwilate®) products, hereafter collectively referred to as pdVWF concentrates. In addition, population pharmacokinetic (PopPK) modeling was used to investigate the relationship between VWF multimeric pattern and VWF:ristocetin cofactor (VWF:RCo) activity in patients with severe VWD treated with VWF.
Methods
Preparation of VWF Products
The VWF products () were reconstituted according to the manufacturer’s instructions, using diluents provided by the manufacturer. Briefly, exact volumes of each diluent were added to sample vials. Vials were held at room temperature until complete dissolution of the product. Aliquots of the reconstituted materials were stored at –60°C or below, and quick-thawed for analysis.
Table 1 VWF Products Analyzed in This Study
Desmopressin Challenge Study
The effect of desmopressin on the multimeric structure of VWF was investigated using samples from a previously published study.Citation27 In that study, changes in ADAMTS13 activity were measured after infusion of desmopressin in 10 healthy volunteers and three patients with VWD.Citation27 The present analysis used plasma samples from the healthy volunteers, which comprised seven men and three women (median age, 29.5 years) who received an infusion of desmopressin (Octostim®; Aventis AG, Vienna, Austria) at a dose of 0.3 μg per kilogram of body weight in 50 mL saline over 30 minutes. Blood was collected at baseline and 1, 2, 6, and 24 hours after infusion was initiated. Platelet-poor citrated plasma was prepared according to standard methods and was analyzed for VWF multimers.
VWF Assays
The VWF:RCo activity, VWF:collagen-binding activity (VWF:CB), and VWF:platelet glycoprotein Ib receptor binding (VWF:GPIbM) assays were performed as published previouslyCitation28 and are described below in brief. One vial per product/strength was reconstituted and liquid aliquots were frozen. For analysis, two independent measurements of VWF:RCo and VWF:GpIbM were taken from a fresh aliquot, and the mean of the two assay runs was reported. Single assay runs with multiple dilutions tested were performed for VWF:Ag, VWF:CB and VWF:FVIII-binding and the mean of the different dilutions tested was reported. To allow comparison between products, the specific VWF activity was calculated as the ratio relative to VWF antigen (VWF:Ag).
VWF:Ag
VWF:Ag was determined by enzyme-linked immunosorbent assay (ELISA) using an in-house assay and had a lower limit of quantification (LLOQ) of 4 IU/dL. VWF was captured using polyclonal rabbit antihuman VWF (DAKO, Glostrup, Denmark) coated on microplate wells. Polyclonal rabbit antihuman VWF/horseradish peroxidase (HRP) conjugate (DAKO) was added and incubated. After a washing step, bound VWF/HRP conjugate complex was detected using tetramethyl-benzidine reagent. The absorbance was read at 450 nm using a microplate reader. VWF:Ag was calculated relative to a human plasma reference (citrated; Siemens AG, Munich, Germany) calibrated versus the World Health Organization plasma standard.Citation29
VWF:RCo
VWF:RCo was measured using a von Willebrand reagent containing lyophilized platelets and ristocetin (BC reagent, Siemens AG, Munich, Germany) on an automated analyzer (BCS® XP, Siemens AG, Munich, Germany). The VWF:RCo activity was calculated from a reference curve constructed by different dilutions of the in-house rVWF reference standard, calibrated versus the World Health Organization 2nd International Standard for VWF Concentrate (National Institute for Biological Standards and Control [NIBSC] code 09/182).Citation29 The LLOQ of the assay was 20 IU/dL.
VWF:GPIbM
VWF activity via GpIb binding was measured on an automated instrument (BCS® XP, Siemens AG, Munich, Germany) using a commercial assay kit (Innovance® VWF Ac, Siemens AG, Munich, Germany) with minor modifications. The product-specific in-house reference standard was calibrated versus NIBSC code 09/182.Citation29 The LLOQ of the assay was 19 IU/dL.
VWF:CB
VWF:CB was measured using the ZYMUTEST™ VWF:CBA ELISA (Hyphen BioMed, Neuville-sur-Oise, France). VWF was captured by fibrillar collagen (type 1 and 3) coated on microplate wells. Following a washing step, collagen-bound VWF was detected by adding polyclonal antihuman VWF antibodies coupled with peroxidase. Peroxidase activity was detected by a substrate (tetramethylbenzidine) and absorbance read at 450 nm. The VWF:CB of the samples was calculated relative to the assay reference standard (normal human plasma [citrated; Siemens AG, Munich, Germany] calibrated against World Health Organization Plasma StandardCitation29) and expressed as VWF:CB IU/mL. The LLOQ of the assay was 12 IU/dL.
VWF Binding to FVIII
FVIII binding capacity of VWF products (VWF:FVIIIB) was measured using the ELISA Asserachrom® VWF:FVIIIB test kit (Stago, Asnières-sur-Seine, France). VWF was captured by antihuman VWF antibodies coated on microplate wells. Endogenous FVIII of the sample was eliminated by a high-salt washing step, and the remaining immobilized VWF was incubated with an excess amount of recombinant FVIII. After washing, FVIII bound to VWF was detected by antihuman FVIII antibodies labeled with peroxidase. The VWF:FVIIIB of the samples was calculated relative to the assay reference standard (normal human plasma [Siemens AG, Munich, Germany] calibrated against the World Health Organization Plasma StandardCitation29) and expressed as a percentage of VWF:FVIIIB.
VWF-Mediated Platelet Adhesion (Under Flow Conditions)
Platelet adhesion to collagen under shear stress (flow conditions) was measured in a perfusion chamber (BioFlux 1000z System, Fluxion BioSciences, San Francisco, CA, USA) using a 48-well plate. Citrated blood from healthy volunteers obtained from donations made to the Austrian Red Cross (consent was provided by donors as part of the routine blood donation process) was spiked with VWF product samples at a concentration of 1 IU/mL VWF:Ag, or with buffer only, and perfused over wells coated with fibrillar collagen type 1 (Horm collagen reagent, Takeda, Linz, Austria). All perfusion experiments were carried out with fluorescent-labeled platelets (calcein) at a wall shear rate of 1500 s–1 (60 dyn/cm2). The degree of platelet adhesion was expressed as a percentage of coverage of the total surface.
Semi-Quantitative Structural Analysis of VWF Multimer Composition
Sodium dodecyl sulfate (SDS)-agarose gel electrophoresis was performed with home cast low-resolution 1% and high-resolution 2.5% SDS-agarose gels (SeaKem, Lonza, Basel, Switzerland) to visualize the multimerization grade of VWF concentrates and assess the presence of satellite bands resulting from ADAMTS13 cleavage. In a separate set of experiments, a 3% SDS-agarose gel was used for higher resolution of the multimer-band structure in human plasma samples. Multimer bands were detected by in-gel two-step immunostaining with an anti-VWF antibody (DAKO) and a goat antirabbit alkaline phosphatase conjugate (Jackson, West Grove, PA, USA). Multimer bands were visualized using an alkaline phosphatase conjugate substrate kit (Bio-Rad, CA, USA). Normal human plasma pool (citrated; Siemens AG, Munich, Germany) was analyzed for reference. Agarose electrophoresis and immunodetection were run twice per sample; displayed gels are representative for the analysis.
Low-resolution multimer patterns were evaluated visually and by densitometry (GS-900, Bio-Rad). Patterns were divided into two categories: (i) all multimers up to the highest multimer of normal human plasma and (ii) exceeding normal plasma up to the top end of the separation gel and thus representing UL multimers. UL multimers were defined as multimers larger than those found in normal human plasma. Relative volumes (area under the curve) were calculated for small (S) (1–5 mers), medium (M) (6–10 mers), and L/UL (>10 mers) multimers. High-resolution agarose gels were evaluated visually for appearance and structure of ADAMTS13-mediated satellite bands.
VWF concentrates were also analyzed with a Hydrasys 2 system using the Hydragel von Willebrand Multimers kit (Sebia, Lisses, France) to validate findings from the home cast gel electrophoresis. This semi-automated instrument allowed assessment of the overall size distribution of VWF multimers using 2% agarose gel electrophoresis, direct immunofixation, and visualization with peroxidase-labeled antibodies. Analysis was performed according to the manufacturer’s instructions and evaluated visually. This method separates VWF multimers in the high to UL range less well than the home cast 1% agarose gels but is less time-consuming and is based on ready-to-use gels and reagents.
rVWF Multimer PopPK Model
PopPK models describing the activity-time profile of small (S) (1–5 mers), medium (M) (6–10 mers), and L/UL (>10 mers) multimers following intravenous administration of rVWF were developed for a prototypic patient with a body weight of 75 kg, based on pharmacokinetic (PK) data from patients with type 1/2 or type 3 VWD. L/UL multimers were analyzed together because of the semi-quantitative nature of the densitometric method. Guidelines on PopPK modeling from the United States Food and Drug Administration and the European Medicines Agency were followed.Citation30,Citation31 The multimer PK profiles were derived through scaling the proportion of S, M, and L/UL multimers by the total antigen levels; they were calculated as VWF:Ag × VWF:S, VWF:Ag × VWF:M, and VWF:Ag × VWF:L/UL, respectively, where the input dose for the multimer PK models was the rVWF dose as measured by VWF:RCo activity in international units. The proportion of S, M, and L/UL multimers was determined by densitometric scanning of agarose gels as described previously.Citation32 These models were used to compare multimer kinetics with that of VWF:RCo. The data for the development of multimer PopPK models originated from multimer distribution data collected in the phase 3 study of patients with severe VWD receiving rVWF for on-demand treatment of bleeding (NCT01410227),Citation33 whereas the information on rVWF PopPK was obtained from a previously presented PopPK/pharmacodynamic model.Citation34
Ethics Approval and Informed Consent
Patient samples for the Desmopressin Challenge Study and Multimer PopPK Model were derived from two previously published clinical studies,Citation27,Citation33 and subjected to secondary analyses at the time of those studies. As described in the original articles, all participants provided written informed consent prior to any screening procedures. Approvals to conduct the studies were granted by the institutional review boards or independent ethics committees of all participating sites. The trials were conducted in accordance with the Declaration of Helsinki.
Results
Functional Activity of VWF Concentrates
For all pdVWF concentrates, the ratios of VWF:RCo to VWF:Ag were below 1, whereas rVWF had a ratio above 1, indicating a higher functional activity than the pdVWF concentrates (). Similar results were obtained for the ratios of VWF:CB to VWF:Ag. For VWF:GPIbM measurements, ratios obtained for rVWF were also higher than that for pdVWF concentrates. The coefficient of variation of the ELISA VWF:FVIIIB method was 8.9% (intermediate precision), FVIII binding capacity of rVWF was expressed as the percentage of the FVIII binding of normal human plasma. Although the FVIII binding capacity of rVWF (113% and 106%) was similar to that of Willfact (100%), it was higher than that of the other concentrates tested (71% to 92%) ().
Figure 1 Static binding activity of VWF concentrates. (A) Binding to platelet receptor and collagen. (B) FVIII binding capacity. Data in both graphs represent mean ± SD. Error bars were calculated from the known imprecision of the assays with relative SDs between 3.7% and 13.0% depending on the assay. Numbers below graph (A) are mean ratios (IU/IU VWF:Ag). Numbers below graph (B) are mean VWF:FVIII binding percentages.
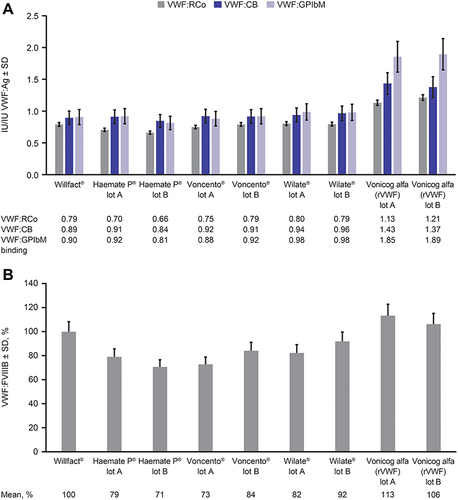
VWF-Mediated Platelet Adhesion to Collagen Under Shear Stress (Flow Conditions)
When human whole blood was spiked with pdVWF or rVWF samples to a final VWF:Ag concentration of 1 IU/mL, the increase in platelet binding above baseline indicated a time-dependent increase in platelet adhesion to immobilized collagen. This increase varied between VWF products, and rVWF was highly effective at mediating platelet adhesion to collagen, displaying a higher mean efficacy compared with the pdVWF concentrates ().
Figure 2 VWF activity: platelet adhesion to collagen under shear stress (flow conditions). VWF-mediated platelet adhesion to immobilized collagen type 1 under shear stress (1500 s–1). Human whole blood was spiked with VWF samples at a final concentration of 1 IU/mL VWF antigen (or buffer) to determine time-dependent platelet binding. Perfusion time was 0–120 seconds. Comparison was based on the same amount of VWF antigen. The data are means of three independent measurements, with average values calculated for two product lots of each VWF sample, except for Willfact®, for which one lot was tested.
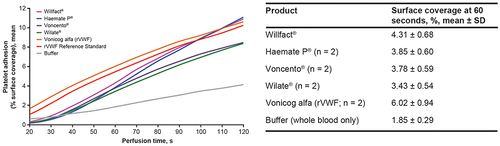
VWF Multimers in Human Plasma Samples
Multimer analysis of human plasma from healthy donors with a 3% SDS-agarose gel showed a characteristic satellite band pattern comprising an intense main band with one faster- and one slower-migrating satellite band. A schematic representing cleavage of UL multimers by ADAMTS13 into smaller molecules is included alongside the image of the gel (). Desmopressin infusion stimulated the secretion of UL multimers, which was observed at 1 hour post-baseline but had declined by 24 hours. Similar images of VWF multimers were seen in all healthy volunteers. shows a representative SDS-agarose gel from a single healthy volunteer.
Figure 3 Multimeric analysis of plasma samples from healthy volunteers. (A) In the plasma of healthy human donors, normal VWF fragments are produced by ADAMTS13 proteolysis (representative sodium dodecyl sulfate–agarose gel shown). The schematic shows that cleavage at Tyr1605/Met1606 generates fragments linked via disulfide bonds with different sizes/MWs relative to the central band of each multimer and the corresponding satellite bands. (B) Representative gel showing VWF multimers from the plasma of a desmopressin-treated healthy volunteer versus a pretreatment plasma sample and a human reference plasma pool.
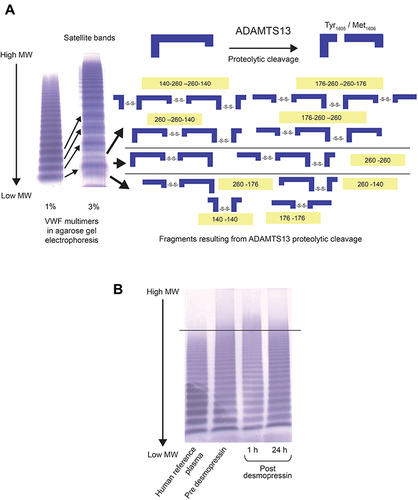
VWF Multimers in VWF Concentrates
Low-Resolution Agarose Gel Electrophoresis
Visual inspection of the immune-stained low-resolution agarose gels showed that rVWF contains UL multimers not present in human plasma or in pdVWF concentrates. In rVWF, the lowest-molecular-weight multimers (up to hexamer) were present in relatively low amounts, whereas pdVWF concentrates showed intense low-molecular-weight multimers (). Semi-quantitative densitometric evaluation showed that the relative percentage area of UL multimers after separation on agarose gel was higher for rVWF samples than for the pdVWF concentrates (). This result was verified by the semi-automated electrophoresis system, which showed that rVWF contains UL multimers that are not observed in human plasma in circulation or in pdVWF concentrates, as well as an absence of the lowest-molecular-weight multimers (data not shown).
Figure 4 Multimer composition of VWF concentrates: low-resolution (1%) agarose gel electrophoresis. The relative percentage area of UL multimers (percentage area above highest plasma multimers) after separation on agarose gel are shown above each lane. Although normal human plasma and the pdVWF concentrates do not contain UL multimers, densitometry of the separation lanes gives an inherent background of 12.3% UL area for normal human plasma and a range of 14.2–18.2% UL area for the pdVWF concentrates. Vonicog alfa (rVWF) shows UL multimers clearly above background.
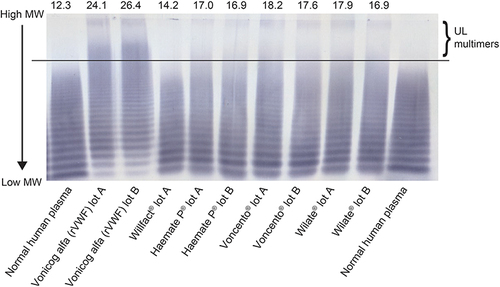
High-Resolution Agarose Electrophoresis
Electrophoresis of human plasma demonstrated a typical satellite band pattern as expected on a high-resolution agarose gel. Voncento® and Haemate P® had a different pattern from human plasma: the faster- and central-migrating bands were more intense, whereas the slower-migrating satellite band was very faint (). This pattern could be indicative of degradation occurring during purification from plasma. No satellite bands were observed with rVWF indicating the intact multimer profile for this concentrate.
Figure 5 Multimer composition of VWF concentrates: high-resolution agarose electrophoresis. Enlarged images of selected lanes are shown in the lower panel; Voncento® and Haemate P® exhibit a different satellite band pattern from normal human plasma, as indicated by the arrows. The faster- and central-migrating bands appear more intense, whereas the slower-migrating satellite band is very faint. Vonicog alfa (rVWF) is composed of intact multimers without satellite bands.
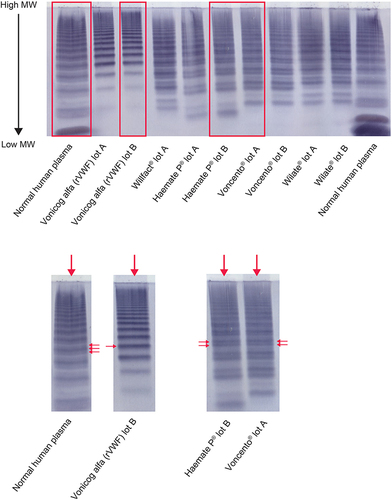
Comparison Between VWF:RCo Activity and S, M, and L/UL rVWF Multimer PK
A PopPK analysis was undertaken to characterize the contribution of various-sized multimer populations to the overall hemostatic potential of rVWF. The PopPK models for VWF:Ag × VWF:S, VWF:Ag × VWF:M, and VWF:Ag × VWF:L/UL were two-compartment models with first-order elimination from the central compartment. Endogenous levels were described as a constant background with identified covariates of the effect of VWD type on endogenous levels (type 3 versus type 1 and 2) and allometric body-weight scaling of clearances and volumes of distribution. Of note, the structural models for VWF:Ag × VWF:S, VWF:Ag × VWF:M, and VWF:Ag × VWF:L/UL were identical to the model for VWF:RCo.Citation34
Visual comparison between the population predictions of VWF multimers and VWF:RCo obtained from the respective final models () suggested a good agreement, as indicated by parallel PK curves, between VWF:RCo and VWF:Ag × VWF:L/UL, up to quantifiable VWF:Ag/VWF:RCo activity levels in both VWD subgroups, whereas VWF:Ag × VWF:M and VWF:Ag × VWF:S were generally characterized by a slower PK. The difference between absolute VWF:RCo and VWF:Ag × VWF:L/UL activity levels could partly be attributed to the assumption regarding the use of VWF:RCo dose as input for the multimer models, although the effect of this is unknown. Another explanation is that the smaller multimer fractions also contribute, to a lesser degree, to the total VWF:RCo activity-time profile. However, the model-based comparison between VWF:RCo and VWF:Ag × VWF:S, VWF:Ag × VWF:M, and VWF:Ag × VWF:L/UL PK revealed that L/UL VWF multimers displayed the highest degree of similarity with VWF:RCo across the three multimers sizes, as indicated by parallel PK curves. This provides evidence that VWF:RCo activity is largely associated with the persistence of L/UL multimers in the systemic circulation.
Figure 6 Population PK predictions of VWF:RCo and VWF multimer activity (VWF:Ag × VWF:S, VWF:Ag × VWF:M, and VWF:Ag × VWF:L/UL activity) over time after a 30 IU/kg (VWF:RCo) vonicog alfa (rVWF) dose for a patient with a body weight of 75 kg with VWD subtypes 1/2 or 3. (A) Type 1/2 VWD. (B) Type 3 VWD. Note that for type 1/2 VWD (A), visual assessment may be distorted after day 3 as curves approach endogenous levels of VWF:Ag and VWF:RCo. This not an issue in type 3 VWD (B) as VWF:Ag and VWF:RCo are undetectable in type 3 VWD. Dotted lines represent LLOQs for VWF:RCo and VWF:Ag (IU/dL) for analysis of human plasma. The graph shows modeled Pop PK data and therefore curves partially cover a range below the LLOQ of the respective methods.
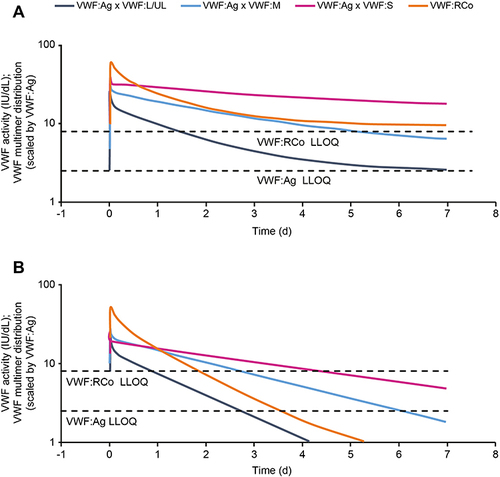
Discussion
Patients with VWD who have an inadequate response or intolerance to desmopressin are potential candidates for replacement therapy with VWF concentrates. The integrity (lack of degradation) and content of VWF in these products is important for their effectiveness. With respect to composition, it is established that the L and UL multimers have higher platelet- and collagen-binding affinity than smaller multimers.Citation8–12 rVWF is the only purified recombinant human VWF approved for adults with VWD for the treatment of bleeding episodes in the on-demand and perioperative settings, and for routine prophylaxis to reduce the frequency of bleeding episodes in adult patients with severe type 3 VWD receiving on-demand therapy.Citation19,Citation20 In this study, the structure and function of rVWF and clinically used pdVWF concentrates were compared under static and dynamic flow conditions.
rVWF is manufactured in the absence of human plasma proteins, thus avoiding the risk of transmission of human blood-borne pathogens and eliminating the effects of co-purifying plasma proteins. As rVWF is not exposed to proteolytic enzymes from human blood during the manufacturing process, it preserves the L and UL multimers.Citation21 Thromboembolic reactions can occur with rVWF, especially in patients with thrombotic risk factors.Citation19,Citation20 However, clinical trial results indicate that the risk is low. During the clinical development of rVWF, two patients treated with rVWF experienced thromboembolic events. Of these, one patient experienced thrombosed hemorrhoid not considered related to rVWF in a Phase 3 study (NCT01410227). A second patient experienced 2 adverse events of deep vein thrombosis in the perioperative setting, of which one was considered possibly related to rVWF.Citation35 The risk of thrombosis with pdVWF products is potentially greater because the exogenous FVIII in the product adds to the endogenous FVIII stabilized by VWF replacement and can lead to FVIII accumulation in the plasma.Citation36
The current study of the multimer composition and functional activity of VWF concentrates demonstrated that rVWF contained UL multimers not present in pdVWF concentrates and displayed single-band multimers because of no previous exposure to ADAMTS13. In a phase 1 clinical trial that evaluated the pharmacokinetics of rVWF:rFVIII in patients with severe VWD, VWF cleavage products were present in patient samples as early as 15 mins post-infusion indicating rapid proteolysis of VWF multimers.Citation24 However, UL multimers were present in samples from rVWF-treated patients for up to 3 hours after infusion but not in samples from pdVWF-treated patients at any post-infusion timepoint.Citation24 This suggests that the most active multimeric fraction for promoting primary hemostasis is present in the plasma for a short period after infusion of rVWF.
The functional activity of the VWF concentrates for static binding of platelets was measured using the VWF:RCo, VWF:GPIbM, and VWF:CB assays. The VWF:RCo assay measures the ability of a sample to bind to GpIb on fixed normal platelets after ristocetin is added in vitro.Citation37 The GPIbM assay provided relatively higher values for rVWF compared with the VWF:RCo assay, regardless of whether the calibrated in-house rVWF reference or the World Health Organization reference standardCitation29 were used (latter not shown). This suggests greater sensitivity of the GPIbM assay, which is based on the binding of VWF to recombinant gain-of-function mutated GPIb that does not require ristocetin.Citation37 Regardless of assay used, the results were consistent, demonstrating that the ratio of measurements of VWF activity to VWF protein content (VWF:Ag) were close to or above 1 for rVWF, whereas pdVWF concentrates had ratios below 1. In normal human plasma, the ratio of VWF:RCo/VWF:Ag is close to 1.Citation3 Therefore, ratios below 1 in the pdVWF concentrates indicate some degree of VWF multimer degradation. VWF binding to collagen was also higher for rVWF, indicating a high functionality of L and UL VWF multimers present in the recombinant product. With respect to FVIII binding capacity, the results of the VWF:FVIIIB ELISA demonstrated higher binding capacity for rVWF than the majority of pdVWF concentrates tested. Overall, the results from these binding experiments are in line with those from other studies,Citation3,Citation25 indicating that the specific activity for binding of collagen and platelets is higher for rVWF than for pdVWF products.
VWF circulates in an inactive globular form, and requires sufficient shear force to change its conformation to an extended form that exposes domains critical for collagen and platelet receptor binding.Citation38 In this study, primary hemostatic activity of rVWF was demonstrated by VWF-induced platelet adhesion to collagen under shear stress (flow conditions), which was faster than for the pdVWF concentrates. This suggests that the presence of UL multimers in rVWF results in improved platelet binding under dynamic flow conditions, presumably because larger-sized multimers have more available binding sites for collagen and GPIb bindingCitation39 and/or these sites are more available to their respective ligands in the context of larger molecular structures. This is also consistent with previous reports of a linear correlation between the content of L multimers in VWF concentrates and the specific activity of VWF:RCo.Citation3,Citation26
Visual inspection and semi-quantitative densitometric evaluation of low-resolution agarose gels of VWF concentrates indicated that rVWF contained UL multimers not present in pdVWF concentrates. High-resolution multimer analysis of rVWF displayed single-band multimers, thus demonstrating an integral multimer pattern of VWF that is not degraded by proteases. The amount of lowest-sized multimers was reduced in rVWF as a result of the manufacturing process, which includes chromatography steps aimed at purifying rVWF to homogeneity and preserving the intact multimer pattern of rVWF secreted into the cell culture medium. However, these multimers support platelet aggregation less effectively than larger ones and, therefore, do not affect overall functionality.Citation25
High-resolution multimer analysis of the normal human plasma samples and pdVWF concentrates demonstrated a characteristic triplet structure consisting of a central band and two flanking bands. In fact, it is known that each pdVWF multimer is accompanied by two slower- and two faster-migrating bands, but further resolution is required to observe the quintuplet structure.Citation40 The satellite bands are degradation products of VWF resulting from physiologic proteolysis by ADAMTS-13 in plasma.Citation4,Citation40,Citation41 For some of the pdVWF concentrates, the satellite structure was altered compared with normal human plasma, ie, the quantity of the slower-migrating band was lower, whereas the faster-migrating satellite band was more intense. These results are in line with those from previous studies that reported variable VWF multimeric patterns compared with normal human plasma in sixCitation26 and twelveCitation3 different pdVWF concentrates analyzed using SDS-agarose gel electrophoresis, and suggest that some degree of degradation occurs during purification from plasma.
Multimer analysis of plasma samples from desmopressin-treated healthy volunteers showed that desmopressin induced secretion of VWF multimers, including a fraction containing UL multimers observable at 1-hour post-stimulation. The human reference sample had a lower content of UL multimers than a fresh pre-sample collected before administration of desmopressin. The reference plasma was a pool from healthy volunteers, whereas the pre- and post-desmopressin samples were from single individuals. Therefore, plasma pretreatment could vary, explaining differences seen in the multimer pattern because VWF is extremely sensitive to minor differences in manipulation, resulting in variable degradation by ADAMTS13 in the human plasma samples. Preparation of a plasma pool requires at least one additional manipulation step, which is unnecessary when a single plasma sample is freshly frozen following centrifugation to remove cells.
A PopPK model described activity-time profiles of the different-sized multimers using data from patients with severe VWD treated with rVWF in a phase 3 clinical trial (NCT01410227).Citation33 In the model-based comparison between VWF biological activity (VWF:RCo) and VWF:Ag × VWF:S, VWF:Ag × VWF:M, and VWF:Ag × VWF:L/UL PK, L/UL multimers displayed the highest degree of similarity with VWF:RCo PK compared with S and M multimers. These results indicate that VWF:RCo activity in patients with severe VWD treated with rVWF is associated with the persistence of L/UL VWF multimers in the systemic circulation, which are known to be the most effective multimers with respect to interactions with collagen and platelet receptors, and also display increased FVIII binding capacity.Citation8–12
In healthy individuals, VWF is derived from multiple sources: plasma, platelet α-granules, and EC Weibel-Palade bodies.Citation42 Although the relative contribution of each of these sources to hemostasis remains the subject of investigation, the lower-molecular-weight multimers that predominate in the plasma pool are not nearly as efficient at mediating platelet adhesion as the UL forms in the EC storage granules.Citation8–12 In type 3 VWD and most forms of type 2, the EC storage pools of UL forms are absent, and the management of bleeding in affected patients depends principally on the repletion of VWF in the plasma compartment.Citation7,Citation43 The results of the present studies provide a clearer basis for the hemostatic efficacy demonstrated by rVWF at a molecular level in recent clinical studies.Citation33,Citation35
Conclusions
This analysis of the multimer composition and functional activity of VWF concentrates demonstrated that rVWF contained UL multimers not present in pdVWF concentrates and displayed single-band multimers because of no previous exposure to ADAMTS13. rVWF had higher specific platelet-binding activity and faster mediation of platelet adhesion to collagen under shear stress relative to pdVWF concentrates. rVWF also demonstrated higher FVIII binding capacity than Haemate P®, Voncento® and Wilate®. The results of the model-based analysis provide supporting evidence that the VWF:RCo activity in patients with severe VWF deficiency treated with rVWF is associated with the persistence of L and UL VWF multimers in the systemic circulation. Taken together, these findings suggest that the L and UL multimers preserved in rVWF contribute to high biological activity and might be important for providing hemostatic efficacy.
Abbreviations
EC, endothelial cell; ELISA, enzyme-linked immunosorbent assay; FVIII, factor VIII; HRP, horseradish peroxidase; L, large; M, medium; NIBSC, National Institute for Biological Standards and Control; pdVWF, plasma-derived VWF; PK, pharmacokinetic; PopPK, population pharmacokinetic; rVWF, recombinant VWF; S, small; SDS, Sodium dodecyl sulfate; UL, ultra-large; VWD, von Willebrand disease; VWF, von Willebrand factor; VWF:Ag, VWF antigen; VWF:CB, VWF collagen binding; VWF:FVIIIB, FVIII binding capacity of VWF products; VWF:GPlbM, VWF platelet glycoprotein Ib mutant receptor binding; VWF:RCo, VWF ristocetin cofactor.
Data Sharing Statement
The datasets analyzed in this study are available upon reasonable request from the corresponding author.
Author Contributions
All authors made a significant contribution to the work reported, whether that is in the conception, study design, execution, acquisition of data, analysis and interpretation, or in all these areas; took part in drafting, revising or critically reviewing the article; gave final approval of the version to be published; have agreed on the journal to which the article has been submitted; and agree to be accountable for all aspects of the work.
Disclosure
HG, GS, and NW are employees of Baxalta Innovations GmbH, a Takeda company, and hold Takeda stock or stock options. BM is an employee of Takeda Development Center Americas, Inc., and holds Takeda stock or stock options. BE was an employee of Takeda Development Center Americas, Inc., during the analysis and development of the manuscript, and holds Takeda stock. PLT is an employee of Baxalta Innovations GmbH, a Takeda company, and holds relevant Takeda patents and Takeda stock or stock options. The authors report no other conflicts of interest in this work.
Acknowledgments
The authors acknowledge Françoise Truong Berthoz, MD, from Baxalta GmbH, a Takeda company, Zurich, Switzerland, who provided medical insights at the early stage of this project. The authors also acknowledge Alexander Bauer, Martin Wolfsegger (Baxalta Innovations GmbH, a Takeda company, Vienna, Austria), and Giovanni Smania (Pharmetheus AB, Uppsala, Sweden) for contributing population pharmacokinetic analyses of small, medium, and large/ultra-large molecular weight multimers following administration of rVWF; Ann-Katrin Holik, Michaela Schädler, Sylvia Peyrer-Heimstätt, Jutta Schreiner, and Manfred Billwein for VWF analytics; and Bettina Ploder (Baxalta Innovations GmbH, a Takeda company, Vienna, Austria) for contributing to the collection of the data. Medical writing support was provided by Nasser Malik, PhD, employee of Excel Medical Affairs (Fairfield, CT, USA) and was funded by Takeda Development Center Americas Inc., Lexington, MA, USA. This paper was presented at the International Society on Thrombosis and Haemostasis (ISTH) Virtual Congress, July 12–14, 2020, as an abstract presentation with interim findings. The poster’s abstract was published in “Poster Abstracts” in Research and Practice in Thrombosis and Haemostasis (RPTH). 2020; 4: Suppl 1 (https://abstracts.isth.org/abstract/recombinant-human-von-willebrand-factor-rvwf-differs-in-structure-and-function-from-plasma-derived-concentrates/).
Additional information
Funding
References
- Heijdra JM, Cnossen MH, Leebeek FWG. Current and emerging options for the management of inherited von Willebrand disease. Drugs. 2017;77(14):1531–1547. doi:10.1007/s40265-017-0793-2
- Furlan M. Von Willebrand factor: molecular size and functional activity. Ann Hematol. 1996;72(6):341–348. doi:10.1007/s002770050184
- Budde U, Metzner HJ, Müller HG. Comparative analysis and classification of von Willebrand factor/factor VIII concentrates: impact on treatment of patients with von Willebrand disease. Semin Thromb Hemost. 2006;32(6):626–635. doi:10.1055/s-2006-949668
- Furlan M, Robles R, Affolter D, Meyer D, Baillod P, Lämmle B. Triplet structure of von Willebrand factor reflects proteolytic degradation of high molecular weight multimers. Proc Natl Acad Sci U S A. 1993;90(16):7503–7507. doi:10.1073/pnas.90.16.7503
- Budde U, Pieconka A, Will K, Schneppenheim R. Laboratory testing for von Willebrand disease: contribution of multimer analysis to diagnosis and classification. Semin Thromb Hemost. 2006;32(5):514–521. doi:10.1055/s-2006-947866
- Goodeve AC. The genetic basis of von Willebrand disease. Blood Rev. 2010;24(3):123–134. doi:10.1016/j.blre.2010.03.003
- Stockschlaeder M, Schneppenheim R, Budde U. Update on von Willebrand factor multimers: focus on high-molecular-weight multimers and their role in hemostasis. Blood Coagul Fibrinolysis. 2014;25(3):206–216. doi:10.1097/MBC.0000000000000065
- Federici AB, Bader R, Pagani S, Colibretti ML, De Marco L, Mannucci PM. Binding of von Willebrand factor to glycoproteins Ib and IIb/IIIa complex: affinity is related to multimeric size. Br J Haematol. 1989;73(1):93–99. doi:10.1111/j.1365-2141.1989.tb00226.x
- Fischer BE, Kramer G, Mitterer A, et al. Effect of multimerization of human and recombinant von Willebrand factor on platelet aggregation, binding to collagen and binding of coagulation factor VIII. Thromb Res. 1996;84(1):55–66. doi:10.1016/0049-3848(96)00161-2
- Moake JL, Turner NA, Stathopoulos NA, Nolasco LH, Hellums JD. Involvement of large plasma von Willebrand factor (vWF) multimers and unusually large vWF forms derived from endothelial cells in shear stress-induced platelet aggregation. J Clin Invest. 1986;78(6):1456–1461. doi:10.1172/JCI112736
- Kragh T, Napoleone M, Fallah MA, Gritsch H, Schneider MF, Reininger AJ. High shear dependent von Willebrand factor self-assembly fostered by platelet interaction and controlled by ADAMTS13. Thromb Res. 2014;133(6):1079–1087. doi:10.1016/j.thromres.2014.03.024
- Gralnick HR, Williams SB, Morisato DK. Effect of multimeric structure of the factor VIII/von Willebrand factor protein on binding to platelets. Blood. 1981;58(2):387–397. doi:10.1182/blood.V58.2.387.387
- Leebeek FWG, Eikenboom JCJ. Von Willebrand’s disease. N Engl J Med. 2016;375(21):2067–2080. doi:10.1056/NEJMra1601561
- Mannucci PM. Treatment of von Willebrand’s disease. N Engl J Med. 2004;351(7):683–694. doi:10.1056/NEJMra040403
- Federici AB. The use of desmopressin in von Willebrand disease: the experience of the first 30 years (1977–2007). Haemophilia. 2008;14(Suppl1):5–14. doi:10.1111/j.1365-2516.2007.01610.x
- Ozgönenel B, Rajpurkar M, Lusher JM. How do you treat bleeding disorders with desmopressin?. Postgrad Med J. 2007;83(977):159–163. doi:10.1136/pgmj.2006.052118
- Batlle J, López-Fernández MF, Fraga EL, Trillo AR, Pérez-Rodríguez MA. Von Willebrand factor/factor VIII concentrates in the treatment of von Willebrand disease. Blood Coagul Fibrinolysis. 2009;20(2):89–100. doi:10.1097/MBC.0b013e3283254570
- López-Fernández MF, López-Berges C, Corral M, García-Talavera JR, López Borrasca A, Batlle J. Assessment of multimeric structure and ristocetin-induced binding to platelets of von Willebrand factor present in cryoprecipitate and different factor VIII concentrates. Vox Sang. 1987;52(1–2):15–19. doi:10.1111/j.1423-0410.1987.tb02981.x
- Baxalta US Inc. VONVENDI® (von Willebrand factor [recombinant]) lyophilized powder for solution for intravenous injection [prescribing information]; 2019. Available from: https://www.shirecontent.com/PI/PDFs/VONVENDI_USA_ENG.pdf. Accessed May 20, 2021.
- Baxalta Innovations GmbH. Veyvondi summary of product characteristics. Available from: https://www.ema.europa.eu/en/documents/product-information/veyvondi-epar-product-information_en.pdf. Accessed May 20, 2021.
- Franchini M, Mannucci PM. Von Willebrand factor (Vonvendi®): the first recombinant product licensed for the treatment of von Willebrand disease. Expert Rev Hematol. 2016;9(9):825–830. doi:10.1080/17474086.2016.1214070
- Turecek PL, Mitterer A, Matthiessen HP, et al. Development of a plasma- and albumin-free recombinant von Willebrand factor. Hamostaseologie. 2009;29(Suppl 1):S32–S38. doi:10.1055/s-0037-1617202
- Colling ME, Friedman KD, Dzik WH. In vitro assessment of von Willebrand factor in cryoprecipitate, antihemophilic factor/VWF complex (human), and recombinant von Willebrand factor. Clin Appl Thromb Hemost. 2019;25:1076029619873976. doi:10.1177/1076029619873976
- Mannucci PM, Kempton C, Millar C, et al. rVWF Ad Hoc Study Group. Pharmacokinetics and safety of a novel recombinant human von Willebrand factor manufactured with a plasma-free method: a prospective clinical trial. Blood. 2013;122(5):648–657. doi:10.1182/blood-2013-01-479527
- Turecek PL, Schrenk G, Rottensteiner H, et al. Structure and function of a recombinant von Willebrand factor drug candidate. Semin Thromb Hemost. 2010;36(5):510–521. doi:10.1055/s-0030-1255445
- Lethagen S, Carlson M, Hillarp A. A comparative in vitro evaluation of six von Willebrand factor concentrates. Haemophilia. 2004;10(3):243–249. doi:10.1111/j.1365-2516.2004.00893.x
- Reiter RA, Knöbl P, Varadi K, Turecek PL. Changes in von Willebrand factor-cleaving protease (ADAMTS13) activity after infusion of desmopressin. Blood. 2003;101(3):946–948. doi:10.1182/blood-2002-03-0814
- Turecek PL, Peck RC, Rangarajan S, et al. Recombinant ADAMTS13 reduces abnormally up-regulated von Willebrand factor in plasma from patients with severe COVID-19. Thromb Res. 2021;201:100–112. doi:10.1016/j.thromres.2021.02.012
- Hubbard AR, Hamill M, Beeharry M, Bevan SA, Heath AB. SSC sub-committee on von Willebrand factor of ISTH. Value assignment of the WHO 2nd International Standard von Willebrand factor, concentrate (09/182). J Thromb Haemost. 2011;9(8):1638–1640. doi:10.1111/j.1538-7836.2011.04365.x
- European Medicines Agency. Guideline on Reporting the Results of Population Pharmacokinetic Analyses; 2007. Available from: https://www.ema.europa.eu/en/documents/scientific-guideline/guideline-reporting-results-population-pharmacokinetic-analyses_en.pdf. Accessed November 4, 2022.
- US Food and Drug Administration. Population pharmacokinetics: guidance for industry; 2019. Available from: http://www.fda.gov/downloads/Drugs/GuidanceComplianceRegulatoryInformation/Guidances/ucm072137.pdf. Accessed November 4, 2022.
- Budde U, Schneppenheim R, Eikenboom J, et al. Detailed von Willebrand factor multimer analysis in patients with von Willebrand disease in the European study, molecular and clinical markers for the diagnosis and management of type 1 von Willebrand disease (MCMDM-1VWD). J Thromb Haemost. 2008;6(5):762–771. doi:10.1111/j.1538-7836.2008.02945.x
- Gill JC, Castaman G, Windyga J, et al. Hemostatic efficacy, safety, and pharmacokinetics of a recombinant von Willebrand factor in severe von Willebrand disease. Blood. 2015;126(17):2038–2046. doi:10.1182/blood-2015-02-629873
- Bauer A, Ploder B, Li Z, Mellgård B, Friberg-Hietala S, Wolfsegger M. Population pharmacokinetics and pharmacodynamics of recombinant von Willebrand factor [abstract PB1541]. Res Pract Thromb Haemost. 2020;4(Suppl 1):776–777.
- Peyvandi F, Mamaev A, Wang JD, et al. Phase 3 study of recombinant von Willebrand factor in patients with severe von Willebrand disease who are undergoing elective surgery. J Thromb Haemost. 2019;17(1):52–62. doi:10.1111/jth.14313
- Mannucci PM. New therapies for von Willebrand disease. Blood Adv. 2019;3(21):3481–3487. doi:10.1182/bloodadvances.2019000368
- Patzke J, Budde U, Huber A, et al. Performance evaluation and multicentre study of a von Willebrand factor activity assay based on GPIb binding in the absence of ristocetin. Blood Coagul Fibrinolysis. 2014;25(8):860–870. doi:10.1097/MBC.0000000000000169
- Siedlecki CA, Lestini BJ, Kottke-Marchant KK, Eppell SJ, Wilson DL, Marchant RE. Shear-dependent changes in the three-dimensional structure of human von Willebrand factor. Blood. 1996;88(8):2939–2950. doi:10.1182/blood.V88.8.2939.bloodjournal8882939
- Reininger AJ. The function of ultra-large von Willebrand factor multimers in high shear flow controlled by ADAMTS13. Hamostaseologie. 2015;35(3):225–233. doi:10.5482/HAMO-14-12-0077
- Ledford-Kraemer MR. Analysis of von Willebrand factor structure by multimer analysis. Am J Hematol. 2010;85(7):510–514. doi:10.1002/ajh.21739
- Dent JA, Galbusera M, Ruggeri ZM. Heterogeneity of plasma von Willebrand factor multimers resulting from proteolysis of the constituent subunit. J Clin Invest. 1991;88(3):774–782. doi:10.1172/JCI115376
- Wagner DD, Saffaripour S, Bonfanti R, et al. Induction of specific storage organelles by von Willebrand factor propolypeptide. Cell. 1991;64(2):403–413. doi:10.1016/0092-8674(91)90648-i
- Berber E. The molecular genetics of von Willebrand disease. Turk J Haematol. 2012;29(4):313–324. doi:10.5505/tjh.2012.39205