Abstract
Niacin, also known as nicotinic acid, is an organic compound that has several cardio-beneficial effects. However, its use is limited due to the induction of a variable flushing response in most individuals. Flushing occurs from a niacin receptor mediated generation of prostaglandins from arachidonic acid metabolism. This study examined the ability of docosahexaenoic acid, eicosapentaenoic acid, and omega-3 polyunsaturated fatty acids (PUFAs), to attenuate niacin-induced prostaglandins in THP-1 macrophages. Niacin induced both PGD2 and PGE2 generation in a dose-dependent manner. Niacin also caused an increase in cytosolic calcium and activation of cytosolic phospholipase A2. The increase in PGD2 and PGE2 was reduced by both docosahexaenoic acid and eicosapentaenoic acid, but not by oleic acid. Omega-3 PUFAs efficiently incorporated into cellular phospholipids at the expense of arachidonic acid, whereas oleic acid incorporated to a higher extent but had no effect on arachidonic acid levels. Omega-3 PUFAs also reduced surface expression of GPR109A, a human niacin receptor. Furthermore, omega-3 PUFAs also inhibited the niacin-induced increase in cytosolic calcium. Niacin and/or omega-3 PUFAs minimally affected cyclooxygenase-1 activity and had no effect on cyclooxygenase -2 activity. The effects of niacin on PGD2 generation were further confirmed using Langerhans dendritic cells. Results of the present study indicate that omega-3 PUFAs reduced niacin-induced prostaglandins formation by diminishing the availability of their substrate, as well as reducing the surface expression of niacin receptors. In conclusion, this study suggests that the regular use of omega-3 PUFAs along with niacin can potentially reduce the niacin-induced flushing response in sensitive patients.
Introduction
Nicotinic acid (niacin) is a water soluble vitamin that has been widely used in the prevention of cardiovascular disease.Citation1,Citation2 The Coronary Drug Project Study reported that niacin reduced nonfatal myocardial infarction by 24% and stroke by 22%.Citation3 Some of the general cardio-beneficial effects of niacin at a dose of 1.5 g/day include the reduction of total cholesterol, triglycerides, very-low-density lipoprotein, low density lipoprotein (LDL), and lipoprotein(a) by 20%.Citation4–Citation7 Niacin has also been shown to effectively increase high-density lipoprotein (HDL) by approximately 20%.Citation8,Citation9 These cardio-beneficial effects of niacin are mediated largely through a receptor-independent mechanism.
Alternative lipid-reducing medications are statins, best known for their LDL-cholesterol lowering effects. Niacin, in contrast, is the most effective, clinically available agent for increasing HDL-cholesterol. The combined use of niacin and statins is recommended for improved outcomes of cardiovascular events.Citation10–Citation14 For example, an HDL-Atherosclerosis Treatment StudyCitation15 (HATS) reported that a combined treatment of simvastatin plus niacin resulted in significant regression of angiographic coronary atherosclerosis and reductions in the rate of clinical events during 2.5 years of follow-up.Citation16 However, a very recent Atherothrombosis Intervention in Metabolic Syndrome with low HDL/High Triglyceride: Impact on Global Health Outcomes (AIM-HIGH) study reported no incremental clinical benefits from the addition of niacin to simvastatin therapy during a 36-month follow-up period, despite significant improvements in HDL cholesterol and triglyceride levels.Citation17,Citation18 The outcome of this study decreased enthusiasm for the combined use of niacin and statins. In addition to this, niacin use is limited by the majority of patients experiencing a “flushing response” that is characterized by severe reddening of the skin, itching, and tingling, which leads many patients to discontinue use.Citation19,Citation20 Several studies have shown that flushing occurs in response to the vasodilatory effects of prostaglandin D2 (PGD2) and prostaglandin E2 (PGE2), and their metabolites, which are elevated after treatment with niacin.Citation21–Citation23 Recently, it has been shown that the epidermal Langerhans cells are one of the cell types responsible for niacin-induced PGD2 release.Citation24,Citation25 The niacin-induced flushing appears to be initiated through a receptor-mediated process.Citation26–Citation28 For example, mice deficient in PUMA-G, a murine niacin receptor, failed to show a niacin-induced increase in ear blood flow (a measure of niacin flush).Citation29 These investigators have also demonstrated that mice lacking PGD2 and PGE2 receptors had reduced flushing responses.Citation29 Furthermore, other evidence also suggests that PGD2 acting through the DP1, a subtype of PGD2 receptor, and PGE2 acting via type 2 and type 4 PGE2 receptors, mediates the niacin-induced flushing response.Citation29,Citation30 Recent studies have demonstrated that Langerhans dendritic cells respond to niacin with a transient increase in the cytoplasmic Ca2 concentration and have suggested that Gi (GTP binding protein) is activated through the niacin receptor.Citation25 It is well known that an increase in the cytoplasmic Ca2+ concentration causes activation of phospholipase A2 (PLA2), which liberates arachidonic acid (AA) from membrane phospholipids. AA can be further metabolized to PGD2 and PGE2 by the ubiquitously expressed type 1 cyclooxygenase (COX) and both PGD2 and PGE2 synthases, which are present in Langerhans dendritic cells. Benyo et alCitation25 suggest that the release of PGD2 and PGE2 from niacin-activated cells then results in vasodilation in the dermal papillae of the upper dermis layer, where the Langerhans cells are localized. This sensitization of epidermis by PGD2 and PGE2 results in the characteristic flushing response.
Both epidemiologicalCitation31–Citation33 and prospective randomized clinical trialsCitation34–Citation36 have reported a decrease in morbidity and mortality from heart disease in patients with diets supplemented with omega-3 polyunsaturated fatty acids (PUFAs). Two particular omega-3 PUFAs are of interest: eicosapentaenoic acid (EPA; 20:5, omega-3) and docosahexaenoic acid (DHA; 22:6, omega-3).
Omega-3 PUFAs improve the plasma lipid profile. HarrisCitation37 concluded that omega-3 PUFAs generally lowered triglycerides (TG) by 25%–28% in an analysis of 72 human trials, where normal subjects or hypertriglyceridemic patients were given 7 g or less of omega-3 PUFAs/day for at least a 2 week period. HarrisCitation37 further noted that omega-3 PUFAs were able to lower lipoprotein cholesterol in animal studies, but there was only a minor impact on lipoprotein cholesterol levels in human studies. Another study by Mori et al also observed similar findings in mildly hypertriglyceridemic patients. Intake of omega-3 PUFAs (4 g/day for 6 weeks) reduced TG levels by 18%–20% but had a minimal impact on low-density lipoprotein cholesterol or high-density lipoprotein cholesterol (HDL-C).Citation38 In contrast to these studies, long-term treatment of hypertriglyceridemic patients with omega-3 PUFAs (4 g/day for 16 weeks) led to a significant reduction in TG by 47%, while TG levels rose by 16% with a placebo (corn oil). This omega-3 PUFA effect was associated with a decrease in ratios of total cholesterol to HDL (20%) and a modest increase in high-density lipoprotein cholesterol (13%).Citation39 Similar results were also reported in another study where hypertriglyceridemic patients were treated with omega-3 PUFAs (4 g/day) for 6 months.Citation40 It appears from different studiesCitation41 that higher levels of omega-3 PUFAs for longer durations have beneficial effects on the plasma lipid profile.
In addition to their effects on the lipid profile, omega-3 PUFAs also exert many cardio-beneficial effects via their involvement in several cellular processes. The incorporation of omega-3 PUFAs into cellular membranes changes the nature of the substrate for phospholipases. PLA2 is a physiologically important enzyme. Its activity is stimulated by niacin through phospholipase C-mediated calcium mobilization.Citation42 Cytosolic PLA2 (cPLA2) catalyzes the hydrolysis of fatty acids from the sn-2 position of membrane phospholipids, resulting in the production of proinflammatory AA-derived eicosanoids and platelet-activating factors.Citation43 Not much is known about the direct regulatory effect of omega-3 PUFAs on cellular PLA2 activity. However, omega-3 PUFAs are easily incorporated into membrane phospholipids on the sn-2 position, where AA is usually present. Cytosolic PLA2 hydrolyzes phospholipids containing omega-3 PUFAs, and then generates free DHA or EPA. DHA, EPA, and AA all compete for COX and lipooxygenase enzymes; however, AA is the strongest substrate of the three.Citation44,Citation45 It is therefore possible that omega-3 PUFAs can effectively reduce the generation of AA-derived proinflammatory eicosanoids including PGD2 and PGE2. Furthermore, DHA is a unique fatty acid, because it significantly alters basic properties of cell membranes, including acyl chain order and fluidity, phase behavior, elastic compressibility, ion permeability, fusion, rapid flip-flop, and resident protein function.Citation46 Similarly, recent studies have demonstrated that several signaling proteins, including surface receptors, are enriched in lipid rafts and can be displaced from membrane rafts by both DHA and EPA.Citation47,Citation48 Various possible mechanisms for niacin-induced PGs formation are outlined in .
Figure 1 Proposed cellular mechanism for Niacin-induced prostaglandins formation.
Abbreviations: 5-lipooxygenase, 5-LOX; AA, arachidonic acid; COX-1 and-2, Cyclooxygenase-1 and-2; cPLA2, cytosolic phospholipase A2; DHA, docosahexaenoic acid; EPA, eicosapentaenoic acid; PG, prostaglandins; TXA, thromboxanes.
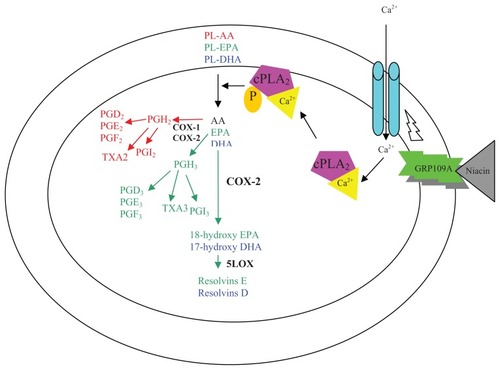
The objective of this study was to investigate if omega-3 PUFAs are capable of inhibiting the niacin-induced PGD2 and PGE2 generation. To test the “proof of the principle,” differentiated macrophages were used, as it has been shown that macrophages are capable of responding to niacin in a similar fashion to that of Langerhans cells.Citation49 Experiments on human cultured epidermal Langerhans cells were also performed to further confirm the results. Niacin treatment caused generation of both PGD2 and PGE2 in macrophages, but induced formation of only PGD2 in Langerhans cells. Omega-3 PUFAs reduced AA levels in the cellular membranes and also reduced surface expression of niacin receptors. This results in an attenuation of niacin-induced PGD2 and PGE2 synthesis.
Materials and methods
Materials
Niacin was obtained from Sigma-Aldrich (St Louis, MO). PGD2 and PGE2 monoclonal enzyme immunoassay (EIA) kits came from the Cayman Chemical Company (Ann Arbor, MI). COX Fluorescent Activity Assay Kits were also purchased from Cayman Chemical. Phycoerythrin-conjugated rat monoclonal anti-human HM74A/GPR109A and Rat IgG Isotype Control-PE were obtained from R&D Systems (Minneapolis, MN). THP-1 or Human Acute Monocytic Leukemia cells were purchased from American Type Culture Collection (Manassas, VA). Langerhans dendritic cells expressing CD1a, MHC class II, and Birbeck granules, were obtained from MatTek Corporation (Ashland, MA). Diisopropyl fluorophosphate and sodium fluoride were from Sigma Chemical Co (St Louis, MO). Phorbol-12-myristate-13- acetate was acquired from Calbiochem (San Diego, CA).
Cell culture and differentiation of THP-1 macrophages
THP-1 human monocytic cell line was maintained in RPMI 1640 media supplemented with L-glutamine, 25 mM HEPES (4-(2-hydroxyethyl)-1-piperazineethane-sulfonic acid), 10% fetal bovine serum (FBS), and 1% penicillin-streptomycin-antimycotic (100X; GIBCO, Grand Island, NY). Cells were maintained at 37°C and 5% CO2. To initiate differentiation, the cells were plated at near 90% confluence in serum-free RPMI 1640 (GIBCO) with 25 nM phorbol-12-myristate-13-acetate for 48 hours at 37°C and 5% CO2. Differentiation was verified via bright-field microscopy as well as Western blot analysis of CD36 expression.
Culturing of Langerhans cells
Freshly isolated human dendritic/Langerhans cells were obtained from MatTek Corporation (Ashland, MA) in a T-10 flask. Cell suspension was centrifuged at 800 xg; cells were re-suspended, and then plated at a density of 40,000 cells per well in a 96-well plate in dendritic-cells maintenance media (DC-MM) supplemented with 5% FBS and a proprietary mixture of cytokines to maintain the dendritic cell pheno-type as per the supplier’s instructions. Cells were allowed to attach overnight in an incubator maintained in 37°C and 5% CO2. Cells were then treated with niacin and/or fatty acids as described in the following sections.
Cell growth assay
Stock solutions (1 mM) of DHA, EPA, and oleic acids (OLA) were prepared by complexing with fatty acid-free bovine serum albumin (BSA).Citation4 Varying concentrations (0, 12.5, 25, 50, 75, and 100 μM) of fatty acids were used to treat the THP-1 derived macrophages. Cell viability was tested using the WST-1 assay. The assay was performed according to the manufacturer’s instructions (Roche, Indianapolis, IN).
Lipid extraction analysis
THP-1 monocytes (5 × 106) were differentiated in a T-75 flask for 48 hours. The resulting macrophages were treated with 0, 12.5, 25, 50, 75, and 100 μM DHA, EPA, or OLA for 24 hours in RPMI 1640 supplemented with L-glutamine, 25 mM HEPES, 5% FBS, and 1% penicillin-streptomycin-antimycotic at 37°C and 5% CO2. The cells were harvested by trypsinization and centrifuged at 800 xg for 5 minutes and then washed in phosphate buffered saline (PBS) containing 1% fatty-acid free BSA. The cells were re-suspended and then lysed in PBS by sonicating on ice. An internal standard (C23:0) was added to 500 μl of cell lysate and a portion of the remaining 100 μl was used to establish a protein concentration in order to normalize fatty acid content to the amount of protein present. Lipids were extracted with chloroform: methanol (2:1) using the Folch methodCitation50 and fatty acids were converted into methyl esters at room temperature for 24 hours as described previously.Citation51 The fatty acids were separated on a gas chromatography system equipped with an auto sampler, flame ionizing detector (GC2010; Shimadzu Corporation, Kyoto, Japan), and a Zebron ZB-WAX plus column (100 m, 0.25 mm ID, 0.25 m; Phenomenex, Torrance CA). The oven temperature increased from 30°C (2 minute hold) to 180°C at 20°C/minute (2 minute hold) to 207°C at 4°C/minute (3 minute hold) to 220°C at 2°C/minute (2 minute hold) to 240°C at 2°C/minute (2 minute hold). The flame ionization detector was used at 250°C to detect the resolved fatty acids peaks, which were identified using authentic standards (Restek Corp, Bellefonte, PA). Data was analyzed with Shimadzu’s GC solutions software (v2.30.00).
Prostaglandin D2 and E2 EIA assay
Prostaglandin D2 and E2 content in the culture media were measured using the competitive EIA kit from Cayman Chemical (Ann Arbor, MI). The assay was carried out according to the manufacturer’s protocol. Briefly, THP-1 differentiated macrophages (3 × 104) or Langerhans cells (4 × 104) were treated with DHA, EPA, and OLA in 96 well plates in duplicate for 24 hours in RPMI-1640 containing 5% FBS and 1% penicillin-streptomycin-antimycotic (100X) at 37°C and 5% CO2. After washing with PBS containing 0.1% bovine serum albumin, the cells were treated with varying concentrations of niacin for 30 minutes. The supernatant (50 μl) was then analyzed for the presence of prostaglandins.
Niacin receptor expression
THP-1 differentiated macrophages (1 × 106) were treated with DHA, EPA, or OLA for 24 hours. The cells were scraped with a rubber policeman and washed in PBS containing 0.5% bovine serum albumin and finally resuspended in 50 μl of this labeling buffer. Cells were then labeled with 0.5 μg phycoerythrin-conjugated GPR109A antibody for 45 minutes. The cells were washed twice with PBS containing 0.5% bovine serum albumin. To ensure specificity of the antibody, an isotype control was established for each sample. Analysis was performed on a FACSCalibur flow cytometer (Becton Dickenson, San Jose, CA) at a 488 nm wavelength. The results indicate the mean fluorescent intensity of the THP-1 cells.
Determination of calcium mobilization
THP-1 macrophages, treated with fatty acids for 24 hours, were loaded with fura-2 acetoxy-methyl ester (fura 2-AM). Briefly, cells (1 × 107) were incubated in Hanks’ balanced salt solution (HBSS) in the presence of 5 μM fura-2 acetoxymethyl ester for 15 minutes at 37°C. After incubation, cells were washed twice with modified (Ca2+ and Mg2+ free) HEPES-buffered (1 mg/ml) HBSS (pH 7.2) and finally resuspended in the same buffer (prewarmed at 37°C). Cells (1 × 106) were preincubated for 1 minute in modified HEPES-buffered HBSS in a temperature-controlled Perkin-Elmer LS50B luminescence spectrometer (Perkin-Elmer Ltd, Beaconfield, England) to determine baseline excitation fluorescence 340/380 nm ratios (R) at 510 nm emission. Cells were stimulated with niacin (3 mM) to determine relative alterations in R due to intracellular release of calcium by continuously monitoring (5 minutes) at 340 and 380 nm excitation.
Western blot analysis of phosphorylated-cPLA2
Lysates of THP-1 macrophages treated with varying concentrations of niacin were prepared in radioimmunoprecipitation assay lysis buffer (Millipore, Temecula, CA) containing 2.5 mM diisopropyl fluorophosphate, 100 mM sodium fluoride and protease inhibitors cocktail (Roche, Indianapolis, IN). Proteins in the samples were separated by sodium dodecyl sulfate page electrophoresis and transferred onto nitrocellulose membranes. The membranes were blocked with 10% western blocking reagent (Roche, Indianapolis, IN) in 1× Tris-Buffered Saline-Tween at room temperature for 1 hour. The membranes were then incubated overnight at 4°C with the phospho-cPLA2 primary antibody (Cell Signaling Technologies, Danvers, MA) at 1:1000 in 1× Tris-Buffered Saline-Tween. Blots were developed using biotinylated secondary antibodies linked to horse radish peroxidase (GE Healthcare, Little Chalfont, UK) and the signal was detected using enhanced chemi-luminescence plus western blocking detection reagents (Amersham, Little Chalfont, UK).
COX activity EIA assay
The total COX activity and COX-1 and COX-2 content in the culture media were measured using the fluorescent activity assay kit from Cayman Chemical. THP-1 differentiated macrophages (1.5 × 106) were treated with 50 μM DHA, EPA, or OLA for 24 hours at 37°C and 5% CO2. Cells were scraped using a rubber policeman. The cells were sonicated in 100 mM Tris HCl (pH 7.5) containing protease inhibitors (Sigma). The lysate was centrifuged at 10,000 ×g for 15 minutes at 4°C and the supernatant was transferred to another micro-centrifuge tube. 10 μl of each sample was transferred to a 96 well plate and the assay was carried out according to the manufacturer’s protocol. The plate was analyzed using a fluorescent well plate reader (Perkin-Elmer). The total COX, COX-1 and COX-2 activities were determined against a standard curve and using the COX-1 inhibitor SC-560 and the COX-2 inhibitor DuP-697.
Results
Fatty acid treatment and cell growth
To ensure cell survival during experiments, THP-1 macrophages were exposed to 0–100 μM DHA, EPA, and OLA. DHA treatment at concentrations below 50 μM did not induce any substantial effect on cell viability; however, concentrations at 75–100 μM showed a reduction in cellular viability by 20%–25% (P > 0.05) when compared to the non-treated control (data not shown). EPA-treatments showed less impact on THP-1 viability, with less than a 10% decrease (non-significant) at 100 μM. The OLA-treatment resulted in only <2% decrease in cell viability at the highest concentration of 100 μM. Based on these results, the authors performed most subsequent experiments at 50 and 75 μM fatty acids.
Omega-3 PUFAs reduced niacin-induced PGD2 and PGE2 production
To test the effect of the omega-3 PUFAs on niacin induced PGD2 and PGE2 release in macrophages, THP-1 cells were treated with DHA, EPA, and OLA prior to exposure to increasing concentrations of niacin. Niacin increased both respectively). In contrast, OLA treatment resulted in further enhancement of basal, as well as niacin-induced PGD2 and PGE2 formation (–).
Figure 2 Effect of fatty acids on niacin induced PGD2 secretion in THP-1 macrophages.
Abbreviations: DHA, docosahexaenoic acid; EIA, enzyme immunoassay; EPA, eicosapentaenoic acid; PGD2, prostaglandin D2; OLA, oleic acid.
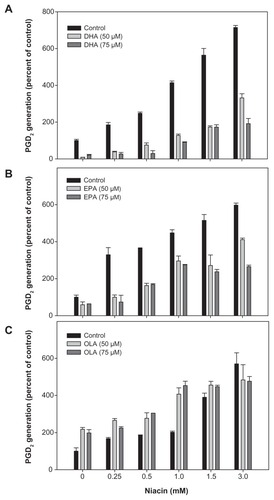
Figure 3 Effect of fatty acids on niacin induced PGE2 secretion in THP-1 macrophages.
Abbreviations: DHA, docosahexaenoic acid; EIA, enzyme immunoassay; EPA, eicosapentaenoic acid; PGD2, prostaglandin D2; OLA, oleic acid.
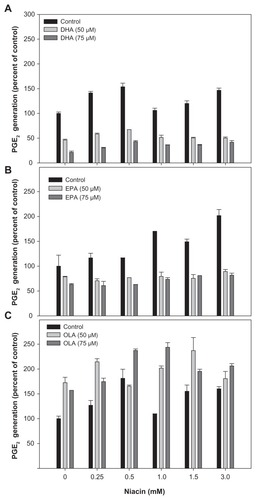
Omega-3 PUFAs alter FA profile
The authors next examined the incorporation of fatty acid in THP-1 cells. An analysis of membrane fatty acid composition suggested that DHA treatment increased the incorporation of DHA in the phospholipids in a dose dependent manner (2 to 168 μg/mg protein). Furthermore, the increased DHA levels in phospholipids occurred at the expense of AA, whose levels decreased from 24 to 13 μg AA/mg protein (). EPA-treatment subsequently increased EPA incorporation in phospholipids in a dose dependent manner (2 to 190 μg/mg protein) (). EPA incorporation into membrane phospholipids also occurred at the expense of AA and resulted in its reduction from 25 to 9 μg AA/mg protein (). Although basal levels of OLA were substantially greater than DHA or EPA, OLA amounts significantly increased in a concentration dependent manner (51 to 391 μg/mg protein); however, OLA incorporation had a minimal effect on AA displacement ().
Figure 4 Fatty acid incorporation into phospholipids of THP-1 macrophages.
Notes: Values are the mean ± the standard deviation of triplicates. Results are analyzed using Student’s t test. *P < 0.01 compared to non-supplemented cells.
Abbreviations: AA, arachidonic acid; DHA, docosahexaenoic acid; EPA, eicosapentaenoic acid; PGD2, prostaglandin D2; OLA, oleic acid.
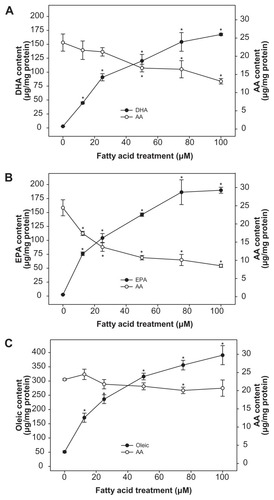
Figure 5 Effect of fatty acids on GPR109A niacin receptor expression in THP-1 macrophages.
Abbreviations: AA, arachidonic acid; DHA, docosahexaenoic acid; EPA, eicosapentaenoic acid; PGD2, prostaglandin D2; OLA, oleic acid.
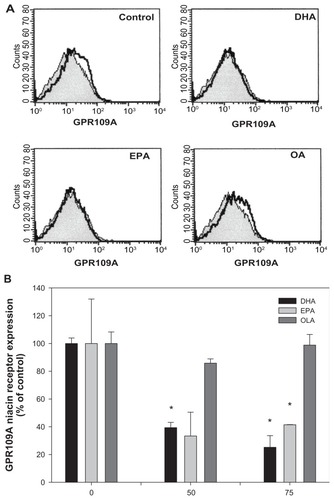
Omega-3 PUFAs down regulate the GPR109A receptor
The authors analyzed the effects of DHA, EPA, and OLA on the expression of the niacin receptor, GPR109A, using flow cytometry. Data in indicate that DHA-treatment at 50 μM showed a 60% decrease in GPR109A expression, while 75 μM DHA further decreased the expression to 75% fewer receptors than compared to the control. 50 μM EPA reduced GPR109A receptor expression by 67%; however, 75 μM EPA did not further reduce its expression. In contrast, OLA treatment did not result in a significant reduction of niacin receptor expression.
Effect of omega-3 PUFAs on cellular calcium release
The authors further analyzed the effect of niacin on down-stream calcium mobilization (). In untreated cells niacin increased intracellular calcium (red line) in a time dependent manner. However, niacin-induced intracellular calcium increases were not observed when cells were pretreated with DHA (blue line), EPA (green line), or OLA (pink line).
Figure 6 Effect of fatty acids on calcium mobilization in THP-1 macrophages.
Abbreviations: DHA, docosahexaenoic acid; EPA, eicosapentaenoic acid; OLA, oleic acid.
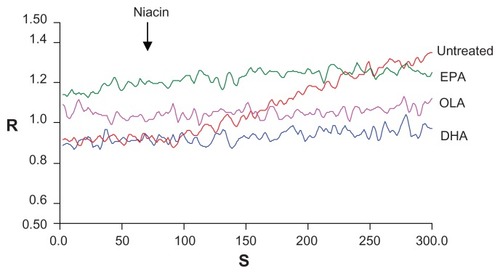
Niacin induces cPLA2 activation
One of the downstream targets for Ca2+ is cPLA2, whose activity causes the release of AA, a substrate for PGD2 and PGE2 synthesis. Therefore, the effect of niacin treatment on cPLA2 activity was measured. Earlier experiments () indicate that DHA and EPA incorporated into phospholipids at the expense of AA. As a result, it was realized that measuring AA release by niacin in DHA and EPA-treated cells is not an appropriate assay for PLA2 activity. Hence, the phosphorylation of PLA2 by niacin was used as a measure of PLA2 activity. Results shown in indicate that niacin at 1.5 mM caused a maximal 2-fold increase in cPLA2 phosphorylation at 30 minutes of incubation. Higher concentration of niacin at 3 mM also induced a 2-fold increase in phosphorylation of cPLA2 at 10 minutes of incubation which did not change on a longer incubation (30 minutes).
Figure 7 Niacin induces cPLA2 activation in THP-1 macrophages.
Abbreviations: cPLA2, cytosolic phospholipase A2.
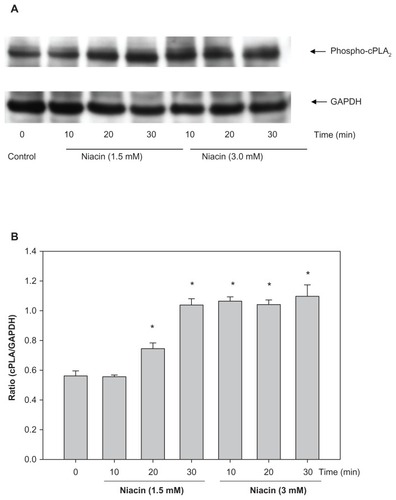
Effect of omega-3 PUFAs on COX activity
The authors observed that niacin itself has no effect on COX-1 activity (). DHA treatment alone slightly reduced COX-1 activity, which was further decreased when DHA-treated cells were stimulated with niacin. EPA treatment alone had no effect COX-1 activity; however, activity was decreased when EPA-treated cells were stimulated with niacin. OLA also had no effect on COX-1 activities, and this activity was also decreased when OLA-treated cells were stimulated with niacin. THP-1 cells also showed very little COX-2 activity that was not affected by niacin or fatty acid treatment ().
Table 1 Effect of fatty acids on COX-1 activity in THP-1 macrophages
Omega-3 PUFAs also regulate niacin-induced PGD2 and PGE2 production in Langerhans cells
After the initial completion of this study of THP-1 cells, the authors evaluated the effects of omega-3 PUFAs in the more disease relevant epidermal Langerhans cells. Data in show that untreated Langerhans cells exhibited a dose-dependent increase in PGD2 production with increasing concentrations of niacin. When treated with 50 μM DHA, the PGD2 production decreased to 71% and 42% at 1.5 and 3 mM concentrations of niacin, respectively. EPA decreased PGD2 production to 53% and 85% at 1.5 and 3 mM concentrations of niacin, respectively. PGE2 production showed very little change with both concentrations of niacin (data not shown).
Figure 8 Effect of fatty acids on niacin-induced PGD2 secretion in Langerhans cells.
Abbreviations: EIA, enzyme immunoassay.
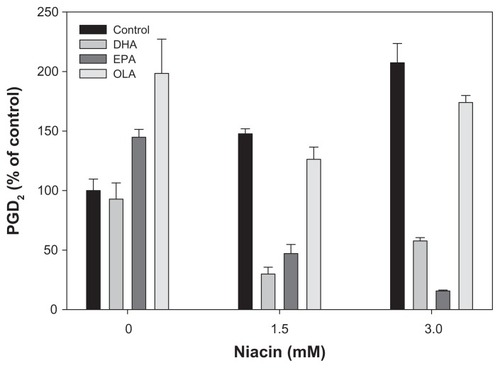
Discussion
Several studies have reported that niacin’s receptor-mediated enhanced release of PGD2 from tissue macrophages causes flushing, particularly by epidermal Langerhans dendritic cells.Citation22,Citation24,Citation25,Citation30,Citation49,Citation52 Several mechanisms have been proposed to reduce flushing in patients on niacin treatment, including reducing absorption of niacin, blocking DP1 receptors, and preventing production of prostaglandins.Citation53 Initial attempts to make slow release niacin, which effectively reduced flushing, failed because of its hepatotoxicity.Citation54,Citation55 Conversely, an extended- release niacin (rates between immediate release and slow release niacin) formulation (Niaspan, Abbott Park, IL) improved CHD outcome and had no hepatotoxic effects, but continued to cause flushing in patients when given higher doses (1–2 g/day) in combination with statins.Citation56–Citation58 A selective DP1 antagonist, laropiprant, was developed to further mitigate the niacin-induced flushing and has shown promise to allow 1–2 g/day dosing regimen of niacin.Citation59 Although laropiprant is a potent inhibitor of DP1, it does not eliminate flushing in all patients,Citation9 suggesting that other pathways may be involved in the residual flushing. Inhibition of prostaglandin synthesis by blocking the activities of COX enzymes with aspirin,Citation60–Citation62 indomethacin,Citation63 ibuprofen,Citation53 and naproxenCitation23 has also been shown to decrease niacin-induced flushing. In the present study, another approach was used to prevent production of prostaglandins. Since prostaglandins are synthesized directly from AA, an omega-6 PUFA, it was hypothesized that replacing cellular content of AA by omega- 3 PUFAs (DHA and EPA) would reduce niacin-induced PGD2 synthesis. The effects of omega-3 PUFAs were tested in differentiated THP-1 macrophages, as these were easy to maintain in culture and also reported to respond in a similar fashion as that of the epidermal Langerhans cells.Citation49 OLA, an omega-9 monounsaturated fatty acid, was also included to demonstrate the specificity of omega-3 PUFA effects.
This study’s data has shown that EPA and OLA did not significantly impact THP-1 macrophage growth; however, DHA did exhibit modest growth inhibition of these cells at higher concentrations (≥75 μM). As previously reported by several investigators, the data also clearly demonstrated that niacin induced PGD2 synthesis in a dose-dependent manner. Furthermore, both DHA and EPA effectively reduced basal, as well as niacin-induced PGD2 synthesis. In contrast, OLA alone stimulated PGD2 release, which was further enhanced in the presence of niacin. The effect of niacin on PGE2 synthesis was also measured. The extent of PGE2 synthesis by niacin was substantially lower than that of PGD2 synthesis. However, both DHA and EPA were able to reduce niacin-induced PGE2 synthesis, whereas OLA had a stimulatory effect. Production of eicosanoids can be regulated by the availability of their substrate, AA, in phospholipids.Therefore the effect of fatty acids on AA content in membrane phospholipids was measured. The data clearly indicated that both DHA and EPA incorporated into cellular phospholipids at the expense of AA. This result is consistent with the authorsCitation46 previous reports that omega-3 PUFAs effectively decrease cellular AA content. However, it is notable that OLA, despite its substantial incorporation into phospholipids, had no effect on AA levels and exhibited a stimulatory effect on PGD2 and PGE2 synthesis. The data indicates (not shown) that OLA mostly incorporated at the expense of stearic and palmitic acid (18:0 and 16:0, respectively), and therefore has no impact over AA incorporation. Furthermore, OLA is not a substrate for the COX pathway and, therefore, it does not compete with AA for eicosanoid generation. It is not clear from the present investigation why OLA had a stimulatory effect on PGD2 and PGE2 synthesis. It is possible that the released stearic and/or palmitic acid induced COX-1 or -2 activities. Clearly, further investigation is needed to understand the effect of OLA on PGD2 and PGE2, which was beyond the scope of this study.
Enrichment of omega-3 PUFAs in cellular membranes often results in changes in the surface expression of membrane proteins, including receptors.Citation46,Citation48 Therefore, the surface expression of GPR109A, a niacin receptor, was examined. The authors found that both DHA and EPA reduced surface expression of the niacin receptor, whereas OLA was ineffective. Diminished GPR109A expression could result in a reduction in niacin-induced flushing. The present study did not investigate if the reduced expression of GPR109A is due to reduced synthesis at the transcriptional or translational level, or if it is due to reduced translocation to the surface from intracellular storage. It is likely that omega-3 PUFAs reorganize lipid raftsCitation48 and therefore affected the translocation of the receptor. The downstream events to niacin receptor were then examined. As previously reported,Citation25 niacin induced an increase in cytosolic calcium, which was effectively blocked by both DHA and EPA as well as by OLA. Stimulation of cells with niacin caused a gradual rise in calcium in the absence of a rapid initial rise. This suggests that the rise in calcium was due to influx from extracellular sources rather than release from intracellular stores. L-type voltage gated calcium channels that regulate influx of calcium from extracellular sources have previously been shown to be inhibited by omega-3 PUFAs.Citation64–Citation66 It is therefore likely that omega-3 PUFAs inhibited calcium influx through inhibiting L-type calcium channels. Other unsaturated fatty acids have been shown to act on these channelsCitation67 and it is therefore possible that OLA also inhibited L-type calcium channels in the present study. One of the targets for rising cytosolic calcium is activation of cPLA2, which releases AA for PGD2 and PGE2 synthesis. The effect of niacin on cPLA2 activity was accessed by measuring its phosphorylation. The results clearly indicate that niacin-treatment of THP-1 cells resulted in cPLA2 phosphorylation, indicating that niacin induces PGD2 and PGE2 formation by liberating AA from phospholipids. However, when AA is replaced by DHA or EPA, niacin-induced cPLA2 activity releases DHA or EPA. Both DHA and EPA compete with AA for downstream COX-1 activity.Citation68–Citation71 During the present investigation, none of the fatty acids had any significant effect on COX-1 activity. It is therefore possible that inhibition of PGD2 and PDE2 synthesis by DHA or EPA is, to some extent, due to a reduction of their substrate, AA. However, the effect of EPA and DHA on GPR109A expression, and inhibition of niacin-induced calcium release also play a role in the reduction of niacin-induced flushing response by omega-3 PUFA. Furthermore, it is also possible that DHA and EPA directly induced anti-inflammatory effects through GPR120, a specific omega-3 fatty acids receptor expressed on macrophages;Citation72 however, it is not known if EPA and DHA mediated effects through GPR120 regulate pro-inflammatory prostaglandin synthesis.
In order to correlate these studies to relevant primary cell line, the effect of DHA, EPA, and OLA on niacin-induced PGD2 and PGE2 synthesis was also measured in Langerhans dendritic cells. Both DHA and EPA were able to inhibit niacin-induced PGD2 synthesis, whereas OLA was not effective. The authors also noticed that EPA and OLA caused a basal increase in PGD2 synthesis. The reason for this increase is not clear. One possibility is variation in the incorporation and metabolism of EPA in the different cell types. In contrast, niacin did not increase PGE2 synthesis and fatty acid-treatments had variable responses. However, this study’s results are consistent with other studies where niacin enhanced only PGD2 production in Langerhans dendritic cells.Citation25,Citation26 These data suggest that PGD2 plays key a role in mediating niacin-induced flushing responses. It is unclear why PGE2 production is stimulated by niacin in THP-1 cells but not in Langerhans cells. Perhaps a second co-factor is required for niacin stimulation that is not present in the Langerhans cells. Further investigation is required to determine this.
It is important to note that the outcome from the recent AIM HIGH study reduces enthusiasm for the use of niacin and statin combination therapy; however, it is possible that use of omega-3 fatty acids with a combination therapy of niacin and statins may be advantageous in improving cardiac events. As mentioned above, long term use of omega-3 fatty acids would have added benefits on improving LDL/HDL ratios and reducing triglycerides. Several pharmaceutical companies are aggressively marketing omega-3 fatty acids preparations for cardiac benef its. For example, preparations such as Crystal EPA-TG (Equateq, Scotland), Omevital TG, Omevital TG-Gold, Omevital –TG-ultra and Omevital-90%TG (Cognis, UK), Incromega (Croda, Goole, UK), and algal-DHA (Martek, Columbia, MD) have been developed that have substantially improved contents of omega-3 fatty acids than that of commercially available menhaden fish oils. In addition to this prescription grade omega-3, supplements containing ethyl esters of DHA + EPA (Lovaza, GlaxoSmithKline, London, UK) are also available. More recently pure ethyl ester preparations of EPA (AMR101, Amarin Corporation, Bedminster, NJ) were developed to treat patients for high or very high triglyceride levels. These preparations supply enriched quantities of omega-3 fatty acids higher than typically present in over-the-counter fish oil supplements.
Conclusions
The data indicated that omega-3 PUFAs can effectively inhibit niacin-induced synthesis of PGD2 and PGE2, the key mediators of flushing caused by niacin intake. The possible inhibitory mechanisms appear to be diminished niacin receptor expression and reduced availability of AA, a substrate for PGD2 and PGE2 synthesis. These effects are mediated within cellular membranes and are based on the enhanced incorporation of omega-3 PUFAs into membrane phospholipids. Therefore, this study suggests that regular intake of fish, fish oil supplements, prescription grade DHA and EPA formulation (GlaxoSmithKline) or other commercially available DHA-, and EPA-enriched preparations, will not only be beneficial in reducing the flushing response of niacin, but also provide several known cardio-beneficial effects.Citation73
Acknowledgments
The research was supported by a research grant from Abbott Laboratories, Abbott Park, IL 60064.
Disclosure
The authors report no conflict of interest.
References
- MeyersCDKamannaVSKashyapMLNiacin therapy in atherosclerosisCurr Opin Lipidol200415665966515529025
- CarlsonLANicotinic acid: the broad-spectrum lipid drug. A 50th anniversary reviewJ Intern Med200525829411416018787
- The Coronary Drug Project Research GroupClofibrate and niacin in coronary heart diseaseJAMA197523143603811088963
- van GreevenbroekMMVoorhoutWFErkelensDWvan MeerGde BruinTWPalmitic acid and linoleic acid metabolism in Caco-2 cells: different triglyceride synthesis and lipoprotein secretionJ Lipid Res199536113247706938
- WahlbergGWalldiusGOlssonAGKirsteinPEffects of nicotinic acid on serum cholesterol concentrations of high density lipoprotein subfractions HDL2 and HDL3 in hyperlipoproteinaemiaJ Intern Med199022821511572394966
- GanjiSHZhangL-HKamannaVSKashyapMLEffect of niacin on lipoproteins and atherosclerosisFuture Lipidol200615549
- CapuzziDMGuytonJRMorganJMEfficacy and safety of an extended-release niacin (Niaspan): a long-term studyAm J Cardiol19988212A74U81U discussion 85U–86U
- BackesJMGibsonCAEffect of lipid-lowering drug therapy on small-dense low-density lipoproteinAnn Pharmacother200539352352615671087
- ParhoferKGReview of extended-release niacin/laropiprant f ixed combination in the treatment of mixed dyslipidemia and primary hypercholesterolemiaVasc Health Risk Manag2009590190820016845
- VogtAKassnerUHostalekUSteinhagen-ThiessenEEvaluation of the safety and tolerability of prolonged-release nicotinic acid in a usual care setting: the NAUTILUS studyCurr Med Res Opin200622241742516466614
- BallantyneCMDavidsonMHMcKenneyJMKellerLHBajorunasDRKarasRHComparison of the efficacy and safety of a combination tablet of niacin extended-release and simvastatin with simvastatin 80 mg monotherapy: the SEACOAST II (high-dose) studyJ Clin Lipidol200822799021291724
- BallantyneCMDavidsonMHMcKenneyJKellerLHBajorunasDRKarasRHComparison of the safety and efficacy of a combination tablet of niacin extended release and simvastatin vs simvastatin monotherapy in patients with increased non-HDL cholesterol (from the SEACOAST I study)Am J Cardiol2008101101428143618471454
- KnoppRHDrug treatment of lipid disordersN Engl J Med1999341749851110441607
- ShepherdJPackardCJPatschJRGottoAMJrTauntonODEffects of nicotinic acid therapy on plasma high density lipoprotein subfraction distribution and composition and on apolipoprotein A metabolismJ Clin Invest1979635858867221531
- National Heart, Lung, and Blood InstituteHDL-atherosclerosis treatment study (HATS)ClinicalTrialsgov [website on the Internet]Bethesda, MDUS National Library of Medicine1999 [updated June 23, 2005]. Available from: http://clinicaltrials.gov/ct2/show/NCT00000553?term=NCT00000553&rank=1. NLM identifier: NCT00000553Accessed February 10, 2012
- BrownBGZhaoXQChaitASimvastatin and niacin, antioxidant vitamins, or the combination for the prevention of coronary diseaseN Engl J Med2001345221583159211757504
- BodenWEProbstfieldJLAndersonTNiacin in patients with low HDL cholesterol levels receiving intensive statin therapyN Engl J Med2011365242255226722085343
- National Heart, Lung, and Blood InstituteNiacin plus statin to prevent vascular eventsClinicalTrialsgov [website on the Internet]Bethesda, MDUS National Library of Medicine2005 [updated June 3, 2011]. Available from: http://clinicaltrials.gov/ct2/show?term=NCT00120289&rank=1. NLM identifier: NCT00120289Accessed February 12, 2012
- GrayDRMorganTChretienSDKashyapMLEfficacy and safety of controlled-release niacin in dyslipoproteinemic veteransAnn Intern Med199412142522587741833
- SternRHSpenceJDFreemanDJParbtaniATolerance to nicotinic acid flushingClin Pharmacol Ther199150166701855354
- NozakiSKiharaSKuboMKamedaKMatsuzawaYTaruiSIncreased compliance of niceritrol treatment by addition of aspirin: relationship between changes in prostaglandins and skin flushingInt J Clin Pharmacol Ther Toxicol198725126436473481359
- MorrowJDParsonsWGIIIRobertsLJIIRelease of markedly increased quantities of prostaglandin D2 in vivo in humans following the administration of nicotinic acidProstaglandins19893822632742475889
- EklundBKaijserLNowakJWennmalmAProstaglandins contribute to the vasodilation induced by nicotinic acidProstaglandins1979176821830504690
- Maciejewski-LenoirDRichmanJGHakakYGaidarovIBehanDPConnollyDTLangerhans cells release prostaglandin D2 in response to nicotinic acidJ Invest Dermatol2006126122637264617008871
- BenyoZGilleABennettCLClausenBEOffermannsSNicotinic acid-induced flushing is mediated by activation of epidermal langerhans cellsMol Pharmacol20067061844184917008386
- LorenzenAStannekCBurmeisterAKalvinshISchwabeUG protein-coupled receptor for nicotinic acid in mouse macrophagesBiochem Pharmacol200264464564812167483
- SogaTKamoharaMTakasakiJMolecular identification of nicotinic acid receptorBiochem Biophys Res Commun2003303136436912646212
- WiseAFoordSMFraserNJMolecular identification of high and low affinity receptors for nicotinic acidJ Biol Chem2003278119869987412522134
- BenyoZGilleAKeroJGPR109A (PUMA-G/HM74A) mediates nicotinic acid-induced flushingJ Clin Invest2005115123634364016322797
- ChengKWuT-JWuKKAntagonism of the prostaglandin D2 receptor 1 suppresses nicotinic acid-induced vasodilation in mice and humansProc Natl Acad Sci U S A2006103176682668716617107
- DaviglusMLStamlerJOrenciaAJFish consumption and the 30-year risk of fatal myocardial infarctionN Engl J Med199733615104610539091800
- AlbertCMHennekensCHO’DonnellCJFish consumption and risk of sudden cardiac deathJAMA1998279123289424039
- BangHODyerbergJHjoorneNThe composition of food consumed by Greenland EskimosActa Med Scand19762001–26973961471
- Dietary supplementation with n-3 polyunsaturated fatty acids and vitamin E after myocardial infarction: results of the GISSI-Prevenzione trialGruppo Italiano per lo Studio della Sopravvivenza nell’Infarto miocardicoLancet1999354917744745510465168
- de LorgerilMRenaudSMamelleNMediterranean alpha-linolenic acid-rich diet in secondary prevention of coronary heart diseaseLancet19943438911145414597911176
- LeafAAlbertCMJosephsonMPrevention of fatal arrhythmias in high-risk subjects by fish oil n-3 fatty acid intakeCirculation2005112182762276816267249
- HarrisWSn-3 fatty acids and lipoproteins: comparison of results from human and animal studiesLipids19963132432528900453
- MoriTABurkeVPuddeyIBPurified eicosapentaenoic and docosahexaenoic acids have differential effects on serum lipids and lipoproteins, LDL particle size, glucose, and insulin in mildly hyperlipidemic menAm J Clin Nutr20007151085109410799369
- HarrisWSGinsbergHNArunakulNSafety and efficacy of Omacor in severe hypertriglyceridemiaJ Cardiovasc Risk199745–63853919865671
- AbeYEl-MasriBKimballKTSoluble cell adhesion molecules in hypertriglyceridemia and potential significance on monocyte adhesionArterioscler Thromb Vasc Biol19981857237319598830
- McKenneyJMSicaDPrescription omega-3 fatty acids for the treatment of hypertriglyceridemiaAm J Health Syst Pharm200764659560517353568
- RheeSGRegulation of phosphoinositide-specific phospholipase CAnn Rev Biochem20017028131211395409
- LibertyIFRaichelLHazan-EitanZCytosolic phospholipase A2 is responsible for prostaglandin E2 and leukotriene B4 formation in phagocyte-like PLB-985 cells: studies of differentiated cPLA2-deficient PLB-985 cellsJ Leukoc Biol200476117618415123778
- CapdevilaJHFalckJRHarrisRCCytochrome P450 and arachidonic acid bioactivation. Molecular and functional properties of the arachidonate monooxygenaseJ Lipid Res200041216318110681399
- SmithWLGaravitoRMDeWittDLProstaglandin endoperoxide H synthases (cyclooxygenases)-1 and -2J Biol Chem19962715233157331608969167
- StillwellWShaikhSRZerougaMSiddiquiRWassallSRDocosahexaenoic acid affects cell signaling by altering lipid raftsReprod Nutr Dev200545555957916188208
- HorejsiVDrbalKCebecauerMGPI-microdomains: a role in signalling via immunoreceptorsImmunol Today199920835636110431155
- SiddiquiRAHarveyKAZalogaGPStillwellWModulation of lipid rafts by omega-3 fatty acids in inflammation and cancer: implications for use of lipids during nutrition supportNutr Clin Pract2007221748817242459
- MeyersCDLiuPKamannaVSKashyapMLNicotinic acid induces secretion of prostaglandin D2 in human macrophages: an in vitro model of the niacin flushAtherosclerosis2007192225325816945375
- FolchJLeesMStanleyGHSA simple method for the isolation and purification of total lipids from animal tissueJ Biol Chem195722649750913428781
- XuZHarveyKAPavlinaTDutotGZalogaGPSiddiquiRAAn improved method for determining medium- and long-chain FAMEs using gas chromatographyLipids20104519920820082149
- PapaliodisDBoucherWKempurajDNiacin-induced “flush” involves release of prostaglandin D2 from mast cells and serotonin from platelets: evidence from human cells in vitro and an animal modelJ Pharmacol Exp Ther2008327366567218784348
- KamannaVSGanjiSHKashyapMLThe mechanism and mitigation of niacin-induced flushingInt J Clin Prac200963913691377
- ChristensenNAAchorRWBergeKGMasonHLNicotinic acid treatment of hypercholesteremia. Comparison of plain and sustained-action preparations and report of two cases of jaundiceJAMA196117754655013693364
- McKenneyJMProctorJDHarrisSChinchiliVMA comparison of the efficacy and toxic effects of sustained- vs immediate-release niacin in hypercholesterolemic patientsJAMA199427196726778309029
- McKenneyJMJonesPHBaysHEComparative effects on lipid levels of combination therapy with a statin and extended-release niacin or ezetimibe versus a statin alone (the COMPELL study)Atherosclerosis2007192243243717239888
- KashyapMLMcGovernMEBerraKLong-term safety and efficacy of a once-daily niacin/lovastatin formulation for patients with dyslipidemiaAm J Cardiol200289667267811897208
- HunninghakeDBMcGovernMEKorenMA dose-ranging study of a new, once-daily, dual-component drug product containing niacin extended-release and lovastatinClin Cardiol200326311211812685616
- BaysHEBallantyneCWhat’s the deal with niacin development: is laropiprant add-on therapy a winning strategy to beat a straight flush?Curr Opin Lipidol200920646747619779335
- CefaliEASimmonsPDStanekEJMcGovernMEKisslingCJAspirin reduces cutaneous flushing after administration of an optimized extended-release niacin formulationInt J Clin Pharmacol Ther2007452788817323787
- DunnRTFordMARindoneJPKwiecinskiFALow-dose aspirin and ibuprofen reduce the cutaneous reactions following niacin administrationAm J Ther19952747848011850694
- ThakkarRBKashyapMLLewinAJKrauseSLJiangPPadleyRJAcetylsalicylic acid reduces niacin extended-release-induced flushing in patients with dyslipidemiaAm J Cardiovasc Drugs200992697919331435
- KaijserLEklundBOlssonAGCarlsonLADissociation of the effects of nicotinic acid on vasodilatation and lipolysis by a prostaglandin synthesis inhibitor, indomethacin, in manMed Biol1979572114117376964
- HirafujiMEbiharaTKawaharaFHamaueNEndoTMinamiMInhibition by docosahexaenoic acid of receptor-mediated Ca(2+) influx in rat vascular smooth muscle cells stimulated with 5-hydroxytryptamineEur J Pharmacol2001427319520111567649
- RinaldiBDi PierroPVitelliMREffects of docosahexaenoic acid on calcium pathway in adult rat cardiomyocytesLife Sci2002719993100412088759
- HallaqHSmithTWLeafAModulation of dihydropyridine-sensitive calcium channels in heart cells by fish oil fatty acidsProc Natl Acad Sci U S A1992895176017641371883
- XiaoYFKeQWangSYSingle point mutations affect fatty acid block of human myocardial sodium channel alpha subunit Na+ channelsProc Natl Acad Sci U S A20019863606361111248125
- SmithWLOmega-3 and omega-6 essential fatty acids and cyclooxygenase pathwaysPaper presented at: Experimental BiologyApr 2006San Fransico, CA
- LaneuvilleOBreuerDKXuNFatty acid substrate specificities of human prostaglandin-endoperoxide H synthase-1 and -2. Formation of 12-hydroxy-(9Z, 13E/Z, 15Z)-octadecatrienoic acids from alpha-linolenic acidJ Biol Chem19952703319330193367642610
- HwangDFatty acids and immune responses – a new perspective in searching for clues to mechanismAnnu Rev Nutr20002043145610940341
- CoreyEJShihCCashmanJRDocosahexaenoic acid is a strong inhibitor of prostaglandin but not leukotriene biosynthesisProc Natl Acad Sci U S A19838012358135846304720
- OhDYTalukdarSBaeEJGPR120 is an omega-3 fatty acid receptor mediating potent anti-inflammatory and insulin-sensitizing effectsCell2011142568769820813258
- SiddiquiRAHarveyKAZalogaGPModulation of enzymatic activities by n-3 polyunsaturated fatty acids to support cardiovascular healthJ Nutr Biochem20081941744317904342