Abstract
Purpose
This study was aimed to investigate the effect of localized vibration on sensory thresholds in mice and humans using a novel quantitative method.
Participants and methods
The sensory thresholds of 7-week-old male C57BL/6J mice were measured with four sine-wave electrostimulation frequencies (5, 50, 250, and 2,000 Hz) before and after applying 2-minute vibration to the plantar side of the foot in mice. In human participants (16 males and 16 females; mean age, 21.0±0.8 years), the sensory threshold was measured at 50 Hz before and after applying 2-minute and 5-minute vibrations to the dorsal side of the foot.
Results
Application of a 2-minute vibration at either the ipsilateral or contralateral side modulated the sensory thresholds elicited by a 5- or 50-Hz right electrostimulation in mice. In human participants, application of a 5-minute vibration at either the ipsilateral or contralateral side modulated the sensory threshold elicited by 50-Hz right electrostimulation, but had no effect on local skin temperature. These results suggest that the right side of pain-related Aδ fibers (50 Hz) or C fibers (5 Hz) was modulated by the localized ipsilateral or contralateral side of vibratory stimuli, respectively, in mice and humans.
Conclusion
The ability of contralateral vibration to modify the right sensory thresholds suggests possible involvement of the central nervous system in vibratory modulation.
Introduction
Sensation can be categorized as either superficial or deep. In superficial sensation, tactile input mediated by Aβ fibers is transmitted to deep-layer neurons in the spinal cord dorsal horn.Citation1 In contrast, superficial or noxious sensation mediated by fast (Aδ fiber) and slow (C fibers) pain fibers synapse with neurons of the substantia gelatinosa in the superficial dorsal horn.Citation1 Aδ or C fiber-mediated pain is involved in numerous chronic pain states that can limit activities of daily living and cause disuse syndrome.
Although many scales for assessing clinical pain, such as the Visual Analog Scale,Citation2 Faces Pain Scale,Citation3 Verbal Numerical Rating Scale,Citation4 Verbal Descriptor Scale,Citation5 and McGill Pain Index,Citation6,Citation7 are available, none of them provide an objective measurement of pain. In contrast, several quantitative scales are used to assess pain in rodents, including the Von Frey filament test,Citation8 tail flick test,Citation9 and hot plate test.Citation10 The Neurometer is used for electrostimulation-induced quantitation of sensory thresholds in both human subjects and rodents.Citation11,Citation12 The benefits of the Neurometer include the ability to represent the sensory threshold as current and the ability to stimulate Aβ, Aδ, and C fibers selectively.Citation13–Citation15 However, the Neurometer is potentially a stand-alone system and cannot alter the stimulation protocol.Citation16 More recently, other specialized electrostimulation-based equipment was developed for quantifying pain, such as PainVision PS-2100 (Nipro Inc., Osaka, Japan) and the STG-4000 series (Multichannel Systems Inc., Reutlingen, Germany). PainVision has been used to quantitatively determine pain intensity as the “degree of pain” calculated from the current production of electrical threshold perception and the current production of a comparable pain sensation.Citation17,Citation18 The STG-4000 series generate stimuli for both current- and voltage-driven stimulations. Any arbitrary analog waveform can be designed as a stimulation signal for every single channel. The programmed stimulation is controlled by the PC-based software.
Numerous treatment strategies have been developed for chronic pain, including medications,Citation19 massage,Citation20 acupuncture,Citation21 stretching,Citation22 physical medicine,Citation23 cognitive-behavioral therapy,Citation24 and alternative therapies.Citation25 In these treatments, especially, the mechanical stimulation (eg, thermal stimulation, pinch, or electrostimulation), which is contained in either the physical medicine or the alternative therapies, has already been reported to have an inhibitory effect on pain.Citation26–Citation28 For example, in 1982, Fitzgerald published a paper on “contralateral” pinch and heat stimulation inducing an inhibitory effect on neuronal activity in the spinal dorsal horn.Citation28 Further, Le Bars et al reported that the activity of convergent dorsal neurons, which receive both low and high threshold afferent inputs, was inhibited by the noxious stimuli applied to various parts of the body.Citation26,Citation27 They proposed this phenomenon as a diffuse noxious inhibitory control (DNIC) hypothesis.Citation26,Citation27
Vibratory stimulation is another form of mechanical stimulation. Previous reports have indicated that whole-body vibration can suppress chronic low back pain,Citation29 knee osteoarthritis,Citation30 and peripheral neuropathy.Citation31,Citation32 Further, it has been reported that local vibratory stimulation also reduces pain in both animalCitation33,Citation34 and human subjects,Citation35,Citation36 increases skin temperature,Citation37 has a positive effect on blood flow at both the exposed and unexposed sides,Citation38 and decreases finger blood flow.Citation39 Moreover, reports have been published on the inhibition of prolonged capsaicin-induced hyperalgesia, caused by daily vibratory stimulation,Citation40 on the suppressive effect of the left vibration for vibrotactile thresholds at the left foot sole.Citation41 It has also been reported that vibration-induced inhibition contains factors of both temporal and spatial summation, which is associated with the amplitude,Citation42 frequency,Citation43,Citation44 threshold,Citation45 duration,Citation45 masking stimulus,Citation46 stimulated mechanoreceptors,Citation46,Citation47 and location and area.Citation48–Citation50
Thus, although numerous studies on the inhibitory effects of noxious mechanical stimulation have already been published, the effects of the contralateral vibratory stimulus for the pain-related sensory threshold have still not been established with measurement of the quantitative sensory threshold. Furthermore, each previous study involved either animal or human experiments, and a comparative inter-species research using both animals and humans has not been reported so far. Therefore, the first purpose of this study was to examine the localized, vibration-induced, bilateral temporal and spatial inhibition of the sensory threshold. The second purpose was to investigate the differences of the response between mouse and human subjects.
Participants and methods
Animals
Male C57BL/6J mice (7 weeks old, 20–23 g, n=26) were purchased from Kyudo, Inc. (Kumamoto, Japan) and housed in an environment with controlled temperature (24°C±1°C) and humidity (55%±10%) on a 12-hour light–dark cycle with ad libitum access to food and water. All animal protocols were approved by the Animal Care Committees of Kumamoto Health Science University (approval no. 14-015) and were conducted in accordance with the National Institute of Health guide for the care and use of laboratory animals (NIH publications No. 80-23, revised 1996).
Vibratory stimuli and sensory threshold measurement in mice
Briefly, a mouse was immobilized in a plastic tube while awake, which was further clamped with an adjusting magnetic base and stand (A-2, Shinwa Rules Co, Sanjyo, Niigata, Japan) and lab clamp (NC-3, Kenis, Osaka, Japan). A vibration device (HB-M01-A, Electric Inc., Tokyo, Japan), which is commercially available at low cost and used for humans, was clamped to the examiner’s hand. The device was then attached to the distal and plantar sides of the right hind paw. The vibration was delivered at the foot sole of either the right (ipsilateral) or left (contralateral) side for 2 minutes (at a frequency of 4,900 times/min; ).
Figure 1 Measurement of the sensory threshold.
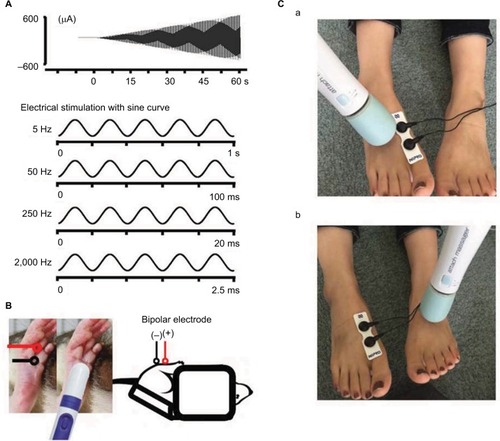
Sensory thresholds were measured with 5, 50, 250, and 2,000 Hz sine electrostimulation (STG-4002, Multichannel Systems Inc.; ). The four different electrostimulation frequencies that we used in this study can stimulate Aβ fibers (2,000 Hz), Aδ fibers (50 and 250 Hz), and C fibers (5 Hz).Citation13–Citation15 Therefore, by using these four electrostimulation frequencies, we were able to evaluate the threshold of each of Aβ, Aδ, and C fibers. Under the immobilized condition of the mice, ball-type bipolar electrodes were placed on the plantar side of the right hind paw, and electrostimulation was applied to the plantar side because the knee joint was flexed maximally and the ankle joint was also flexed dorsally under the supine position. Therefore, since the dorsal side of the foot was hidden, we could not stimulate the dorsal side of the hind paw (). The electrostimulation-induced withdrawal reflex of the mouse’s hind limb resulted in loss of contact with the electrode. The time from the onset of electrostimulation to the appearance of the withdrawal reflex was measured, and the intensity at which the withdrawal reflex occurred was calculated (μA). Before the vibration was delivered, as control, we measured the sensory thresholds 15 times (five times/set and three sets) for each of the four electrostimulation frequencies (5, 50, 250, and 2,000 Hz). After the vibration was delivered at the foot sole of either the right (ipsilateral) or left (contralateral) side for 2 minutes (at a frequency of 4,900 times/min; ), the sensory thresholds were remeasured at the point of X-min (X=0, 2, 4, 6, 8, 10, 12, 15) after exposure to the vibration stimulus (). We remeasured the sensory thresholds three times for each point, and the averaged threshold was adopted for the value.
Figure 2 The measurement of the sensory threshold (test stimulus) and vibratory stimulus (conditioning stimulus).
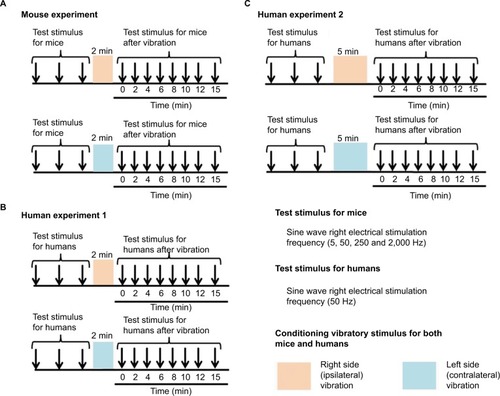
Vibratory stimuli and sensory threshold measurement in human participants
A total of 32 healthy participants (16 males, 16 females; mean age, 21.0±0.8 years) were recruited for study participation. First, we investigated the effect of a 2-minute vibration applied to either the ipsilateral or contralateral dorsal foot for the sensory threshold. Then, we examined the effect of a 5-minute vibration applied to either the ipsilateral or contralateral dorsal foot for the sensory threshold. The sensory thresholds of human participants were measured only with 50-Hz electrostimulation based on a program for clinical evaluation (Pain vision, Nipro Inc.) because the equipment producing 5, 50, 250, and 2,000 Hz electrostimulation (STG-4002, Multichannel Systems Inc.), which was used with the mice, was not entirely suitable for use with human participants in this study; therefore, we evaluated the threshold of only Aδ fibers in human participants. Further, although we initially attempted to measure the sensory threshold using the plantar side of the foot, the sensory threshold in each participant fluctuated to a great extent under the control condition before the vibratory stimuli were applied. Therefore, the dorsal side of the foot was selected in human participants. After the dorsal side of the foot was exposed, bipolar electrodes were placed proximal to the metacarpophalangeal joint and on the dorsal side of the right first finger. Then, human participants held the push button, which could release the electrostimulation, in their right hand. After the electrostimulation was administered to the participants, when they experienced maximum pain, they could push the button. Then, the intensity (mA) was automatically measured, and the value was stored in a personal computer.
After we measured the sensory thresholds 15 times (five times/set and three sets) as control condition, a vibration device (YCM-721, Daito Electric Co, Osaka, Japan) was attached to the dorsal side of the foot at either the ipsilateral or contralateral side to provide a vibratory stimulus for 2 minutes or 5 minutes (at a frequency of 5,230 times/min). Thereafter, the sensory thresholds were remeasured at the point of X min (X=0, 2, 4, 6, 8, 10, 12, 15) after exposure to the vibratory stimulus. We remeasured the sensory thresholds three times for each point, and the averaged threshold was adopted for the value ( and ).
Measurement of skin temperature in human participants
Before and after applying the vibratory stimulus on the dorsal foot of either the ipsilateral (five male and four female participants; mean age, 21.0±0.0 years) or contra-lateral side (seven male and six female participants; mean age, 21.0±1.3 years), we simultaneously measured the skin temperature using a digital thermometer (CT-450WR, CUSTOM, Tokyo, Japan) where the sensory threshold on the dorsal foot was measured. The time of the vibratory stimulus was 5 minutes, and the measurement of the skin temperature was performed before vibration, and at 0 and 15 minutes after the vibration. Then, the skin temperature was normalized.
All the participants provided written informed consent according to the Declaration of Helsinki before the start of the experiments, and all human experiments were approved by the Life Science Committees of Kumamoto Health Science University (approval no. 25-29 and 2016-02).
Statistical analysis
Experimental data are expressed as the mean ± SD. Within-group comparisons (before vibration: control vs 0 minute, after vibration: “0”; before vibration: control vs 15 minutes, after vibration: “15”; –) were performed using Wilcoxon signed rank tests. P<0.05 was the threshold for statistical significance. The average values of all three baseline tests were used for the statistical analysis. Furthermore, in human experiments, the averaged line graphs of either the sensory threshold or the skin temperature are shown. Further, the histograms of the sensory threshold are shown. The histograms present an increase in sensory threshold value (above 100% of the normalized sensory threshold) and a decrease in the sensory threshold value (below 100% of the normalized sensory threshold). Moreover, 100% of the normalized sensory threshold indicates no change in the sensory threshold value. All statistical analyses were performed with EZR (Saitama Medical Center, Jichi Medical University, Saitama, Japan), which is a graphical user interface for R (The R Foundation for Statistical Computing, Vienna, Austria). More precisely, EZR is a modified version of R commander designed to add statistical functions frequently used in biostatistics.Citation51
Figure 4 Averaged values of the sensory thresholds for the right four electrostimulation frequencies in mice (**P<0.001).
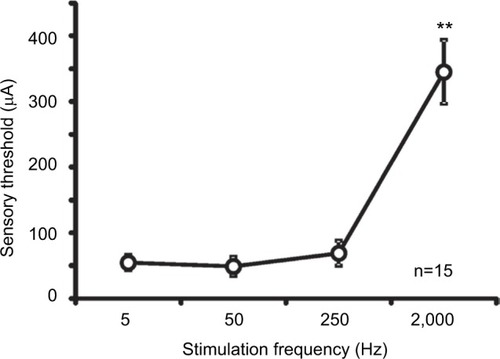
Figure 5 Measurement of the sensory thresholds for the right four electrostimulation frequencies after ipsilateral vibration in mice.
Abbreviation: ns, nonsignificant.
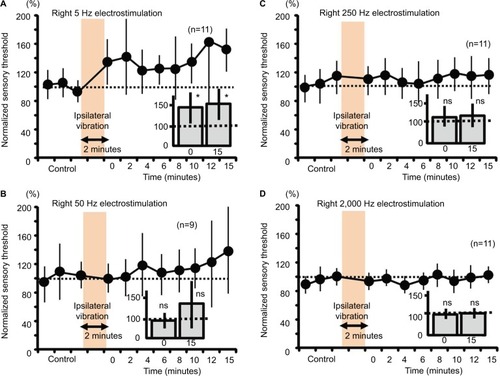
Figure 6 Measurement of the sensory thresholds for the four electrostimulation frequencies after the contralateral vibration in mice.
Abbreviation: ns, nonsignificant.
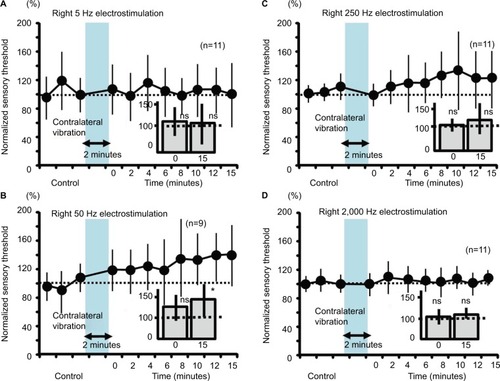
Figure 7 Measurement of the sensory thresholds for the 50-Hz electrostimulation after either ipsilateral or contralateral vibration (2 minutes) in human subjects.
Abbreviation: ns, nonsignificant.
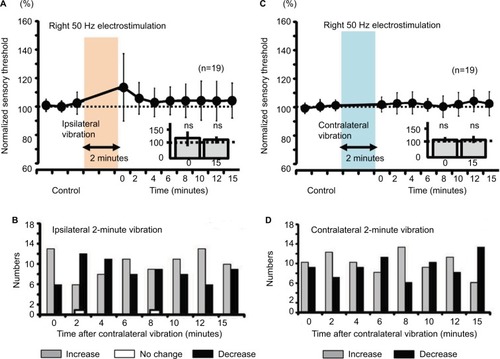
Figure 8 Measurement of the sensory thresholds for the right 50-Hz electrostimulation after either ipsilateral or contralateral vibration (5 minutes) in human subjects.
Abbreviation: ns, nonsignificant.
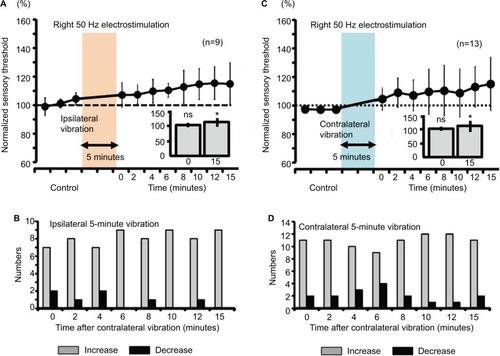
Results
Sensory thresholds of four electrostimulation frequencies
The mean sensory thresholds of the right four electrostimulation frequencies were 56.7±11.6, 48.9±14.3, 69.0±19.0, and 345.1±48.7 μA, respectively (). The threshold was significantly higher in response to a 2,000-Hz electrostimulation than in response to the other electrostimulation frequencies (P<0.001).
Right sensory thresholds and ipsilateral vibration in mice
Two-minute vibration applied to the ipsilateral plantar sole produced significant increases in the sensory threshold elicited by the right 5-Hz electrostimulation at both 0 minutes (fast response) and 15 minutes (slow response) (, P<0.05). Although ipsilateral 2-minute vibration gradually elevated the averaged value of the sensory threshold at 50-Hz electrostimulation (, 140% as an average value at 15 minutes after stimulation), the sensory threshold did not significantly change (, not significant). Further, ipsilateral 2-minute vibration did not change the sensory threshold by the 250- and 2000-Hz electrostimulation ( and , not significant).
Right sensory thresholds and contralateral vibration in mice
Contralateral 2-minute vibration produced significant increases in the sensory threshold of the right hind limb by the 50-Hz electrostimulation, whose modulation was at 15 minutes after vibratory stimulation (slow response; , P<0.05). However, contralateral 2-minute vibration did not change the sensory threshold by the 5-, 250- and 2000-Hz electrostimulation (, not significant).
Right sensory thresholds and either side vibration in humans
Neither ipsilateral nor contralateral 2-minute vibration had any effect on the right sensory thresholds in human participants (, not significant).
Right sensory thresholds and persistent either side vibration in humans
Both ipsilateral and contralateral vibrations for 5 minutes produced significant increase in right sensory thresholds, whose modulation involved a slow response (, P<0.05). Furthermore, the right sensory threshold of all the nine subjects increased at 6, 10, and 15 minutes after the right vibration (), and the right sensory threshold of 12 of 13 subjects increased at 10 and 12 minutes after the left vibration ().
Vibration-induced skin temperature in humans
In both ipsilateral and contralateral vibratory stimuli, vibration-induced fluctuation of skin temperature was seen in a few instances. However, there were no significant differences in skin temperature between before and after vibratory stimulation (99.44%±3.76% at 0 minute after the ipsilateral vibration, 100.00%±4.78% at 15 minutes after the ipsilateral vibration, 100.93%±3.49% at 0 minute after the contralateral vibration, 100.70%±5.63% at 15 minutes after the ipsilateral vibration; ).
Figure 9 Changes in skin temperature after ipsilateral or contralateral 5-minute vibration.
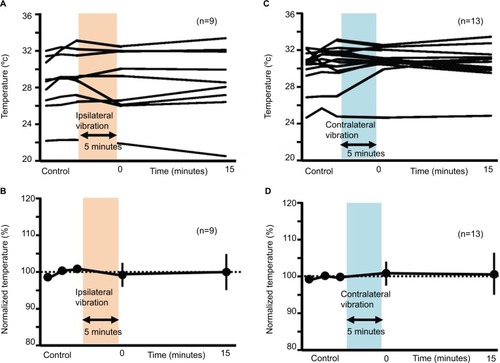
Discussion
The present study found that in mice, ipsilateral 2-minute vibration modulated the sensory threshold in response to right 5-Hz electrostimulation, and contralateral 2-minute vibration modulated in response to right 50-Hz electrostimulation. Further, persistent 5-minute vibration, but not 2-minute vibration, of both the ipsilateral and the contralateral sides modulated the sensory threshold in response to right 50-Hz electrostimulation in human subjects, whose modulation was independent of skin temperature. Moreover, except for the modulation of the right sensory threshold caused by the ipsilateral vibratory stimulus in mice, other sensory modulation was observed for 50-Hz electrostmulation in both mice and humans.
In accordance with previous studies, the four different electrostimulation frequencies that we used in this study could stimulate Aβ fibers (2,000 Hz), Aδ fibers (50 and 250 Hz), and C fibers (5 Hz).Citation13–Citation15 However, Koga et al, reported that a 2,000-Hz sine wave mainly activates Aβ fibers (and partially activates Aδ fibers), while a 250-Hz sine wave activates both Aβ and Aδ fibers, and a 5-Hz sine wave stimulates all Aβ fibers, Aδ fibers, and C fibers.Citation52 In experiments on mice, therefore, the ipsilateral vibratory modulation of the sensory threshold in response to right 5-Hz electrostimulation potentially results from C fibers, as the results were not significant at 50 and 250 Hz (Aβ and Aδ fibers), and 2,000 Hz (Aβ fibers; ). The contralateral vibratory modulation of the sensory threshold in response to right 50-Hz electrostimulation potentially results from Aδ fibers as the results were not significant at 2,000 Hz (). In human experiments, both the ipsilateral and contralateral vibratory threshold modulations in response to right 50-Hz electrostimulation derives from Aβ and Aδ fibers, presumably the Aδ fibers.
Cross-species differences in both spatial and temporal summation
For mice, 2-minute vibration may be sufficient to modulate the sensory threshold. However, 2-minute vibration in humans did not affect the threshold (). These results suggest that the 2-minute vibration itself did not reach “the threshold” to modulate the sensory threshold in human experiments. Except for the ipsilateral vibratory C fiber modulation and fast response in mice, other sensory modulation was observed for Aδ fibers and slow response in both mice and humans. In mouse experiments, we utilized the vibration device that is used for humans. According to a previous report, the sensory threshold for the vibration decreased with increase of the vibration area,Citation48 suggesting that the intensity of the vibration as a stimulator positively correlates with the area factor. Therefore, the ipsilateral vibratory modulation in mice may have involved both slow and fast responses because the vibratory stimulus for humans had a strong intensity for mice. Although ipsilateral 50-Hz vibration-induced threshold modulation of the sensory threshold did not change significantly, the averaged value of the sensory threshold was gradually elevated (, 140% as slow response), suggesting that ipsilateral vibratory modulation in mice may affect both C and Aδ fibers because of the ipsilateral strong vibration. Either ipsilateral or contra-lateral persistent (5-minute) vibration in humans modulated the sensory thresholds (), suggesting that temporal summation may have compensated for the limited spatial (area) summation, and the persistent vibratory stimulus finally reached “the threshold” to modulate the sensory threshold.
We cannot fully explain the relationship among the foot location of the sensory threshold, the vibratory modulation, and the inter-species differences because there is no research on the comparison of the sensory threshold between the dorsal and plantar sides of the foot. However, the dorsal side of the hand appears to be more sensitive to two-point discrimination than the volar side of the hand,Citation53 suggesting that the dorsal side of the foot is also more sensitive to sensation than the plantar side of the foot. If this is true, the 2-minute experiments in humans should have a more drastic effect than that in mice. However, the results were the opposite (–). A few studies have examined age-dependent changes in sensation, such as in warm, cold, and vibration.Citation54,Citation55 In this study, although the age of the mice was less than that of the humans, we do not believe that age is the main factor for the difference in the response, since the age itself cannot be simply compared between these two species.
Cutaneous mechanoreceptors and stimulus frequency in mice
The peak sensitivity of the Pacinian corpuscle-mediated system is at approximately a 250-Hz vibration.Citation56,Citation57 The non-Pacinian, Meissner’s corpuscles-mediated, rapid-adapting system appears to be activated at up to a 50-Hz vibration.Citation56,Citation57 Although we cannot be certain, either 50- or 250-Hz sine waves may also activate different types of cutaneous mechanoreceptors. Therefore, a persistent (5-minute) 50-Hz electrostimulation may strongly activate the specific cutaneous mechanoreceptor system to modulate the sensory threshold resulting from the Aδ fibers.
Vibratory stimulus and skin temperature in humans
A previous study reported that local vibratory stimulation increases skin temperature.Citation37 In that study, Oliveri et al used 100-Hz vibration and a 15-minute stimulus to measure skin temperature at the vibrated small spot, directly.Citation37 We believe that there are two reasons why the 5-minute vibration in our study did not increase skin temperature. One involves the timing of the stimulation. The other involves the location at which the skin temperature was measured. If we had measured the skin temperature at the vibrated small spot, the increase of temperature would have perhaps been detectable. Another study reported that increase in skin temperature reduced the vibration threshold on the forearm.Citation58 Therefore, if the vibration increased the skin temperature of the surrounding area, the sensory threshold may have been reduced. The opposite may be true for the elevation of the sensory threshold.
Contralateral vibratory modulation and central mechanisms
Two alternative regions were potentially responsible for contralateral vibration-induced changes in the right sensory threshold. First is the brainstem descending inhibitory system,Citation59,Citation60 and the second is the cortical region.Citation61,Citation62 Descending serotonergic projections originate in the raphe nuclei,Citation59 while the noradrenergic system constitutes A5, A6, and A7 cell groups.Citation60 These descending inhibitory systems project to spinal cord dorsal horn neurons via the dorsolateral funiculus.Citation60,Citation63,Citation64 The vibrotactile stimulation-induced medial lemniscus (ML) ascending pathway terminates in the nucleus gracilis, nucleus cuneatus, posterior lateral nucleus of the thalamus, and para-brachial nuclei (PV).Citation65,Citation66 Further, some Aβ fibers, which are activated by vibrotactile stimulation, terminate in a deep layer of the dorsal horn to relay ascending information via the anterior spinothalamic (ST) pathway.Citation67 A previous study reported that the ST ascending pathway targets not only the thalamus but also the caudal ventrolateral medulla (VLM), lateral PV, and periaqueductal gray matter (PAG).Citation68,Citation69 For example, PAG neurons project to the A5, A6, and A7 cells to modulate nociception.Citation70 The PAG and VLM form synapses with the raphe nuclei,Citation71,Citation72 and the raphe neurons project to the A7.Citation73,Citation74 Thus, vibrotactile stimulation may have activated both the ML and ST ascending pathways and indirectly affected the raphe nuclei and A5, A6, and A7 cells, which are components of the serotonergic and noradrenergic descending inhibitory systems.
As for the second alternative region, we propose cortical modulation. Pain stimuli activate the contralateral thalamus,Citation75–Citation77 primary somatosensory cortex (SI),Citation61 contralateral anterior cingulate cortex,Citation61 and bilateral secondary somatosensory cortex (SII).Citation61,Citation62 In one study, focal pain sensation changed regional cerebral blood flow (rCBF) in the contralateral SI, contralateral SII, contralateral insula, and others.Citation78 The ST ascending pathway sends more fibers to the primary motor cortex (M1) and SI than to the pre-motor cortex or somatosensory association cortex.Citation79,Citation80 Torquati et alCitation81 reported that activation of the bilateral posterior SII was associated with pain stimulation. In contrast, vibrotactile stimulation activated the bilateral thalamus, contralateral SI, bilateral SII, and others.Citation82 Vibrotactile stimulation also changed rCBF in the contralateral SI and SII.Citation78 The ML ascending pathway sends more fibers to the premotor cortex and M1 than to the SI or SII.Citation79,Citation80 Moreover, the bilateral anterior SII has been associated with somatosensory stimulation.Citation81 Thus, both pain sensation and vibrotactile stimulation overlap in the activation of the contralateral thalamus, contra-lateral SI, and the bilateral SII.
Our study has the following limitations. “The central mechanisms” in response to the contralateral vibratory stimulation which we proposed in this study remain a hypothesis. Therefore, in animal experiments, using whole animal live imaging or electrophysiological techniques, such as in vivo cortical, spinal imaging, or in vivo intracellular recording under an anesthetized condition, and in human experiments using electroencephalography or functional magnetic resonance imaging, it is necessary to demonstrate the involvement of the central nervous system in the contralateral vibratory stimulation-induced sensory modulation.
Clinical implication
DNIC represents inhibitory modulation, usually performed by a “pain inhibits pain” test paradigm.Citation83 Additionally, DNIC is a technique for reducing pain, which is inhibited by the noxious stimuli applied to various parts of the body.Citation26,Citation27 In fact, the DNIC technique has been used in clinical medicine.Citation84 In this study, we used vibration as a conditioning stimulus instead of using noxious stimuli. Our vibratory conditioning stimulus, which is applied to the contralateral side of body, potentially shares commonalities with the DNIC. Further, the mechanisms of DNIC are thought of as “central mechanisms,” which may be in line with our “central hypothesis” for the contralateral vibratory modulation. Therefore, our animal and human study, which used precise and quantitative evaluation may be useful for the elucidation of the “DNIC and central mechanisms”.
Conclusion
In conclusion, we used a novel quantitative method to show that local bilateral vibration elevates the right sensory thresholds in mice and in human subjects. The contralateral vibratory modulation of the right sensory threshold suggests the involvement of the central nervous system.
Disclosure
The authors report no conflicts of interest in this work.
References
- AbrairaVEGintyDDThe sensory neurons of touchNeuron201379461863923972592
- StaufferMETaylorSDWatsonDJPelosoPMMorrisonADefinition of nonresponse to analgesic treatment of arthritic pain: an analytical literature review of the smallest detectable difference, the minimal detectable change, and the minimal clinically important difference on the pain visual analog scaleInt J Inflam2011201123192621755025
- LeeJYMeasurement of Trigeminal Neuralgia Pain: Penn Facial Pain ScaleNeurosurg Clin N Am201627332733627324999
- FarrarJTPritchettYLRobinsonMPrakashAChappellAThe clinical importance of changes in the 0 to 10 numeric rating scale for worst, least, and average pain intensity: analyses of data from clinical trials of duloxetine in pain disordersJ Pain201011210911819665938
- WyshamNGMiriovskyBJCurrowDCPractical Dyspnea Assessment: Relationship Between the 0-10 Numerical Rating Scale and the Four-Level Categorical Verbal Descriptor Scale of Dyspnea IntensityJ Pain Symptom Manage201550448048726004401
- MainCJPain assessment in context: a state of the science review of the McGill pain questionnaire 40 years onPain201615771387139926713423
- DworkinRHTurkDCRevickiDADevelopment and initial validation of an expanded and revised version of the Short-form McGill Pain Questionnaire (SF-MPQ-2Pain20091441-2354219356853
- BradmanMJFerriniFSalioCMerighiAPractical mechanical threshold estimation in rodents using von Frey hairs/Semmes-Weinstein monofilaments: Towards a rational methodJ Neurosci Methods20152559210326296284
- HoleKTjølsenAThe tail-flick and formalin tests in rodents: changes in skin temperature as a confounding factorPain19935332472548351154
- VilelaFCVieiraJSGiusti-PaivaASilvaMLDExperiencing early life maternal separation increases pain sensitivity in adult offspringInt J Dev Neurosci20176281428751239
- TsuiBCShakespeareTJLeungDHTsuiJHCorryGNReproducibility of current perception threshold with the Neurometer(®) vs the Stimpod NMS450 peripheral nerve stimulator in healthy volunteers: an observational studyCan J Anaesth201360875376023690134
- KisoTNagakuraYToyaTNeurometer measurement of current stimulus threshold in ratsJ Pharmacol Exp Ther2001297135235611259562
- MassonEAVevesAFernandoDBoultonAJCurrent perception thresholds: a new, quick, and reproducible method for the assessment of peripheral neuropathy in diabetes mellitusDiabetologia198932107247282591640
- TayBWallaceMSIrvingGQuantitative assessment of differential sensory blockade after lumbar epidural lidocaineAnesth Analg1997845107110759141933
- LiuSKopaczDJCarpenterRLQuantitative assessment of differential sensory nerve block after lidocaine spinal anesthesiaAnesthesiology199582160637832336
- OhDYunTKimJThe Measurement of the Sensory Recovery Period in Zygoma and Blow-Out Fractures with Neurometer Current Perception ThresholdArch Plast Surg201643541141727689047
- OhtoriSKawaguchiHTakebayashiTPainVision Apparatus Is Effective for Assessing Low Back PainAsian Spine J20148679379825558322
- WangDZhangKHanSYuLPainVision® Apparatus for Assessment of Efficacy of Pulsed Radiofrequency Combined with Pharmacological Therapy in the Treatment of Postherpetic Neuralgia and Correlations with MeasurementsBiomed Res Int20172017567021928357404
- CollocaLLudmanTBouhassiraDNeuropathic painNat Rev Dis Primers201731700228205574
- Gok MetinZArikanDonmezAIzguNOzdemirLArslanIEAromatherapy Massage for Neuropathic Pain and Quality of Life in Diabetic PatientsJ Nurs Scholarsh201749437938828605119
- CabýogluMTErgeneNTanUThe mechanism of acupuncture and clinical applicationsInt J Neurosci2006116211512516393878
- Mata DizJBde SouzaJRLeopoldinoAAOliveiraVCExercise, especially combined stretching and strengthening exercise, reduces myofascial pain: a systematic reviewJ Physiother2017631172227989732
- Espí-LópezGVArnal-GómezABalasch-BernatMInglésMEffectiveness of Manual Therapy Combined With Physical Therapy in Treatment of Patellofemoral Pain Syndrome: Systematic ReviewJ Chiropr Med201716213914628559754
- KnoerlRLavoie SmithEMWeisbergJChronic Pain and Cognitive Behavioral Therapy: An Integrative ReviewWest J Nurs Res201638559662826604219
- SinghHBhushanSAroraRSingh ButtarHAroraSSinghBAlternative treatment strategies for neuropathic pain: Role of Indian medicinal plants and compounds of plant origin-A reviewBiomed Pharmacother20179263465028578258
- Le BarsDDickensonAHBessonJMDiffuse noxious inhibitory controls (DNIC). I. Effects on dorsal horn convergent neurones in the ratPain197963283304460935
- Le BarsDDickensonAHBessonJMDiffuse noxious inhibitory controls (DNIC). II. Lack of effect on non-convergent neurones, supraspinal involvement and theoretical implicationsPain197963305327460936
- FitzgeraldMThe contralateral input to the dorsal horn of the spinal cord in the decerebrate spinal ratBrain Res198223622752877066689
- WangXQPiYLChenPJWhole body vibration exercise for chronic low back pain: study protocol for a single-blind randomized controlled trialTrials20141510424693945
- AnwerSAlghadirAZafarHAl-EisaEEffect of whole body vibration training on quadriceps muscle strength in individuals with knee osteoarthritis: a systematic review and meta-analysisPhysiotherapy2016102214515126619822
- KesslerNJHongJWhole body vibration therapy for painful diabetic peripheral neuropathy: a pilot studyJ Bodyw Mov Ther201317451852224139013
- HongJBarnesMKesslerNCase study: use of vibration therapy in the treatment of diabetic peripheral small fiber neuropathyJ Bodyw Mov Ther201317223523823561872
- LundebergTAbrahamssonPBondessonLHakerEVibratory stimulation compared to placebo in alleviation of painScand J Rehabil Med19871941531583438712
- LundebergTNordemarROttosonDPain alleviation by vibratory stimulationPain198420125446333660
- YarnitskyDKuninMBrikRSprecherEVibration reduces thermal pain in adjacent dermatomesPain1997691-275779060015
- KakigiRShibasakiHMechanisms of pain relief by vibration and movementJ Neurol Neurosurg Psychiatry19925542822861583512
- OliveriDJLynnKHongCZIncreased skin temperature after vibratory stimulationAm J Phys Med Rehabil198968281852930643
- FurutaMSakakibaraHMiyaoMKondoTYamadaSEffect of vibration frequency on finger blood flowInt Arch Occup Environ Health19916332212241917073
- YeYGriffinMJReductions in finger blood flow induced by 125-Hz vibration: effect of area of contact with vibrationEur J Appl Physiol201311341017102623064872
- KimHKSchattschneiderJLeeIChungKBaronRChungJMProlonged maintenance of capsaicin-induced hyperalgesia by brief daily vibration stimuliPain20071291-29310117134833
- GuCGriffinMJVibrotactile thresholds at the sole of the foot: Effect of vibration frequency and contact locationSomatosens Mot Res2011283-4869322115026
- GescheiderGAZwislockiJJRasmussenAEffects of stimulus duration on the amplitude difference limen for vibrotactionJ Acoust Soc Am19961004 Pt 1231223198865638
- GreenBGVibrotactile temporal summation: effect of frequencySens Processes1976121381491029083
- HämäläinenHPertovaaraASoininenKJärvilehtoTIs there low frequency vibrotactile temporal summation?Scand J Psychol19812232032067330616
- van DorenCLTemporal summation by Pacinian corpuscles precludes entrainment at the detection thresholdJ Acoust Soc Am1985776218821894019902
- GescheiderGAHoffmanKEHarrisonMATravisMLBolanowskiSJThe effects of masking on vibrotactile temporal summation in the detection of sinusoidal and noise signalsJ Acoust Soc Am1994952100610168132895
- LamoréPJKeeminkCJEvidence for different types of mechanoreceptors from measurements of the psychophysical threshold for vibrations under different stimulation conditionsJ Acoust Soc Am1988836233923513411025
- GuCGriffinMJSpatial summation of vibrotactile sensations at the footMed Eng Phys20133581221122723357339
- MoriokaMGriffinMJThresholds for the perception of hand-transmitted vibration: dependence on contact area and contact locationSomatosens Mot Res200522428129716503581
- WhitehouseDJMoriokaMGriffinMJEffect of contact location on vibrotactile thresholds at the fingertipSomatosens Mot Res2006231-2738116846962
- KandaYInvestigation of the freely available easy-to-use software ‘EZR’ for medical statisticsBone Marrow Transplant201348345245823208313
- KogaKFurueHRashidMHTakakiAKatafuchiTYoshimuraMSelective activation of primary afferent fibers evaluated by sine-wave electrical stimulationMol Pain200511315813963
- GellisMPoolRTwo-point discrimination distances in the normal hand and forearm: application to various methods of fingertip reconstructionPlast Reconstr Surg19775915763318754
- LautenbacherSKunzMStratePNielsenJArendt-NielsenLAge effects on pain thresholds, temporal summation and spatial summation of heat and pressure painPain2005115341041815876494
- VerrilloRTAge related changes in the sensitivity to vibrationJ Gerontol19803521851937410775
- BensmaïaSJLeungYYHsiaoSSJohnsonKOVibratory adaptation of cutaneous mechanoreceptive afferentsJ Neurophysiol20059453023303616014802
- MakousJCFriedmanRMVierckCJA critical band filter in touchJ Neurosci1995154280828187722630
- VerrilloRTBolanowskiSJThe effects of skin temperature on the psychophysical responses to vibration on glabrous and hairy skinJ Acoust Soc Am19868025285323745684
- BowkerRMWestlundKNSullivanMCCoulterJDOrganization of descending serotonergic projections to the spinal cordProg Brain Res1982572392656296918
- WestlundKNBowkerRMZieglerMGCoulterJDNoradrenergic projections to the spinal cord of the ratBrain Res1983263115316839168
- TalbotJDMarrettSEvansACMeyerEBushnellMCDuncanGHMultiple representations of pain in human cerebral cortexScience19912514999135513582003220
- SimõesCHariRRelationship between responses to contra- and ipsilateral stimuli in the human second somatosensory cortex SIINeuroimage199910440841610493899
- WestlundKNCoulterJDDescending projections of the locus coeruleus and subcoeruleus/medial parabrachial nuclei in monkey: axonal transport studies and dopamine-beta-hydroxylase immunocytochemistryBrain Res1980232352647470856
- WestlundKNCarltonSMZhangDWillisWDDirect catecholaminergic innervation of primate spinothalamic tract neuronsJ Comp Neurol199029921781862229478
- MassopustLCHaugeDHFernedingJCDoubekWGTaylorJJProjection systems and terminal localization of dorsal column afferents: an autoradiographic and horseradish peroxidase study in the ratJ Comp Neurol198523745335442413085
- WildJMAvian somatosensory system: II. Ascending projections of the dorsal column and external cuneate nuclei in the pigeonJ Comp Neurol198928711182794122
- AbrairaVEKuehnEDChirilaAMThe Cellular and Synaptic Architecture of the Mechanosensory Dorsal HornCell20171681-229531028041852
- ToddAJMcgillMMShehabSANeurokinin 1 receptor expression by neurons in laminae I, III and IV of the rat spinal dorsal horn that project to the brainstemEur J Neurosci200012268970010712649
- ToddAJNeuronal circuitry for pain processing in the dorsal hornNat Rev Neurosci2010111282383621068766
- BajicDProudfitHKProjections of neurons in the periaqueductal gray to pontine and medullary catecholamine cell groups involved in the modulation of nociceptionJ Comp Neurol1999405335937910076931
- SnowballRKDampneyRALumbBMResponses of neurones in the medullary raphe nuclei to inputs from visceral nociceptors and the ventrolateral periaqueductal grey in the ratExp Physiol19978234855009179568
- ZagonAInnervation of serotonergic medullary raphe neurons from cells of the rostral ventrolateral medulla in ratsNeuroscience19935538498677692351
- ClarkFMProudfitHKThe projection of noradrenergic neurons in the A7 catecholamine cell group to the spinal cord in the rat demonstrated by anterograde tracing combined with immunocytochemistryBrain Res199154722792881884202
- ClarkFMProudfitHKThe projection of locus coeruleus neurons to the spinal cord in the rat determined by anterograde tracing combined with immunocytochemistryBrain Res199153822312452012966
- CraigADBushnellMCZhangETBlomqvistAA thalamic nucleus specific for pain and temperature sensationNature199437265087707737695716
- ApkarianAVShiTSquirrel monkey lateral thalamus. I. Somatic nociresponsive neurons and their relation to spinothalamic terminalsJ Neurosci19941411 Pt 2677967957965079
- GingoldSIGreenspanJDApkarianAVAnatomic evidence of nociceptive inputs to primary somatosensory cortex: relationship between spinothalamic terminals and thalamocortical cells in squirrel monkeysJ Comp Neurol199130834674901865012
- CoghillRCTalbotJDEvansACDistributed processing of pain and vibration by the human brainJ Neurosci1994147409541088027764
- JangSHSeoJPDifferences of the medial lemniscus and spinothalamic tract according to the cortical termination areas: A diffusion tensor tractography studySomatosens Mot Res2015322677125365478
- JangSHKwonYHLeeMYLeeDYHongJHTermination differences in the primary sensorimotor cortex between the medial lemniscus and spinothalamic pathways in the human brainNeurosci Lett20125161505322480695
- TorquatiKPizzellaVBabiloniCNociceptive and non-nociceptive sub-regions in the human secondary somatosensory cortex: an MEG study using fMRI constraintsNeuroimage2005261485615862204
- GolaszewskiSMSiedentopfCMKoppelstaetterFHuman brain structures related to plantar vibrotactile stimulation: a functional magnetic resonance imaging studyNeuroimage200629392392916253525
- YarnitskyDConditioned pain modulation (the diffuse noxious inhibitory control-like effect): its relevance for acute and chronic pain statesCurr Opin Anaesthesiol201023561161520543676
- StaudRRobinsonMEVierckCJPriceDDDiffuse noxious inhibitory controls (DNIC) attenuate temporal summation of second pain in normal males but not in normal females or fibromyalgia patientsPain20031011-216717412507711