Abstract
Purpose
This study was aimed to investigate the effects of heat-conduction dry needling therapy on chronic myofascial pain syndrome (CMPS) in rats and explore the analgesic mechanisms by measuring the mRNA expression of transient receptor potential cation channel subfamily V member 1 (TRPV1) in musculus gastrocnemius.
Methods
Seventy-two rats were randomly divided into five groups. Group A was the control group containing eight normal rats. Group B was the model group where 16 rats developed CMPS in musculus gastrocnemius but received no treatments. Groups C, D, and E containing 16 CMPS rats in each group were the trial groups receiving heat-conduction dry needling therapy. In detail, rats in Group C received dry needling treatment at the heating temperature of 44°C. Rats in Group D received intramuscular injection of capsazepine before receiving dry needling treatment with the heating temperature at 44°C. Rats in Group E received dry needling treatment with the heating temperature at 40°C. The mRNA expression of TRPV1, protein kinase C (PKC), and interleukin (IL)-6 in the needle insertion points of musculus gastrocnemius was measured at 24 hrs and 7 days after dry needling therapy.
Results
Compared with untreated CMPS rats, the mRNA expression of TRPV1, PKC, and interleukin-6 (IL-6) was increased in rats receiving dry needling therapy with the heating temperature at 44°C for 24 hrs. However, the mRNA expression of TRPV1, PKC, and IL-6 in CMPS rats receiving injection of TRPV1 antagonist capsazepine into musculus gastrocnemius followed by temperature-needling at 44°C was analogous to that in untreated CMPS rats.
Conclusion
Dry needling therapy with needles heated to 44°C could impact the activity of TRPV1/PKC/IL-6 pathway.
Introduction
Transient receptor potential (TRP) is a superfamily of non-selective cation channels that can be used as sensors for multiple physical and chemical signals, including cellular messengers (such as Ca2+, cyclic nucleotides, membrane lipids, and osmotic pressure) and environmental stimuli (such as thermal, light, mechanical, and chemical signals, including triggers of nociceptive and inflammatory pathways).Citation1 TRP ion channels are associated with a variety of pain, including inflammatory pain, neuropathic pain, visceral pain, and pain associated with certain pathological conditions, such as cancer and migraine.Citation2 TRP cation channel subfamily V member 1 (TRPV1), originally named as vanilloid receptor 1, was the first TRP channel cloned from rat dorsal root ganglion (DRG) by Caterina et al in 1997.Citation3 TRPV1 is widely distributed in central and peripheral nervous systems and highly expressed in algesia-related primary sensory neurons and spinal dorsal horns.Citation4 As the most widely studied member in TRP family, TRPV1 is of great importance to the perception of hazardous stimuli and the generation of idiopathic pain in afferent nociceptors. Under the stimulation of hazardous signals, TRPV1 in peripheral nociceptors will be sensitized and activated, then carry these signals to the central nervous system of the organism, and induce discomfort and pain to warn the organism of potential hazards.Citation5,Citation6 Capsaicin and temperatures above 43°C could effectively activate TRPV1, which could further change algesia.Citation7 TRPV1 knockout mice showed obvious insensitivity to thermal and pain stimuli.Citation8 TRPV1 inhibitors reduced pain associated with TRPV1 activation by impeding the excitation of TRPV1 channel,Citation9 which made these compounds potential candidates as preferred analgesics in several studies.Citation10 Intriguingly, several studies also showed that TRPV1 agonists could induce desensitization during continuous activation of TRPV1 channel to relieve pain in rodents and humans. Lee et al also confirmed the analgesic effect of RTX, a TRPV1 agonist, in rats based on an in vivo pharmacodynamics experiment.Citation11
However, there is a considerable disadvantage of TRPV1 agonists and inhibitors during their applications in pain relief. Systemic administration of capsaicin, a classical TRPV1 agonist, could not only decrease TRPV1 expression, improve the resistance of rats to noxious stimuli, but also induce temperature dysregulation.Citation12 Gavva et al found that administration of TRPV1 antagonists could increase body temperatures.Citation13 Thus, for now, TRPV1 channel antagonists and agonists have not been introduced to clinical practices due to the severe systematic adverse effects in spite of the great potential of TRPV1 channel in pain treatment and the exact analgesic effects of TRPV1 antagonists and agonists.
In previous studies, due to the difficulty of in vivo precision heating, in vitro cell heating was widely used as a simple alternative method for exploring the impacts of temperatures on TRPV1 channel.Citation14–Citation17 Nevertheless, the results obtained through the method above could not well match those obtained from living tissues. According to the study by Cheng et al in 2008, heating skin surface to 44.2°C via moxibustion could alter TRPV1 activity and affect blood pressures.Citation18 Notably, the heating method only worked on superficial tissues but not deep tissues.
In this study, we postulated that with dry needles heated to 44°C, heat-conduction dry needling therapy could affect the mRNA abundance of TRPV1, protein kinase C (PKC), and interleukin (IL)-6 in rats, which, however, could be abrogated by a TRPV1 antagonist. Internal heated dry needles that could heat deep living tissues were applied for precision heating of musculus gastrocnemius in rats for the first time and the mRNA abundance of TRPV1 was measured. Our results illustrated the potential role of topical physical heating in regulating TRPV1 activity and analgesic therapy.
Materials And Methods
Instruments
Internal heated dry needles with 13.5 cm in total length were purchased from Tianjin Boshuobei Biotechnology Co., Ltd., Tianjin, China. The needle handles were 4.5 cm in length. The needle bodies were 9 cm in length and 1.0 mm in diameter, of which the heating zones were 8 cm in length. An internal heated dry needle heater with a precision of 1°C was purchased from Tianjin Boshuobei Biotechnology Co., Ltd., Tianjin, China.
Animals And Experimental Procedures
All experimental procedures were approved by the Ethics Committee on the Care and Use of Animals of First Medical Center of Chinese PLA General Hospital (Beijing, China, ID: 2015FC-TSYS-1033) and all animals received human care in strict accordance with the National Institutes of Health Guidelines. Seventy-two adult male Wistar rats weighing 180–220 g were used for the study. All rats grew in a specific pathogen-free clean room with a condition of 22–24°C and a 12-hr dark/light cycle.
Establishment Of Chronic Myofascial Pain Syndrome (CMPS) Model In Rats
Seventy-two rats were randomly divided into five groups (A, B, C, D, and E), of which Group A contained eight rats and the remaining four groups contained 16 rats in each group. Rats in Groups B, C, D, and E were weighted and intraperitoneally injected with 10% chloral hydrate (3 mL/kg) for anesthesia. Anesthetized rats were fixed and musculus gastrocnemius were exposed. Muscle belly of musculus gastrocnemius was marked and injured by a 1200-g dropping object from a height of 20 cm. Then, rats were fed under normal conditions. All rats undertook a 90-mins downhill running program with the gradient of −16° at a speed of 16 m/min the next day. In this program, rats were driven by sound and electrical stimuli. The program was performed once a week for four consecutive weeks.Citation19
Interventions
Group A was the control group containing normal rats. Group B was the model group in which CMPS rats naturally recovered without any interventions. Group C was a trial group in which CMPS rats received heat-conduction dry needling therapy. Herein, dry needles were heated to 44°C that was slightly higher than the target temperature 43°C due to the fluctuation of heating temperatures. Group D was a trial group in which rats were administrated with capsazepine (25 mg/kg) by local injection into musculus gastrocnemius and then treated with heat-conduction dry needling therapy at the heating temperature of 44°C 1 hr later. Group E was a trial group in which rats were inserted with dry needles heated to 40°C.
For heat-conduction dry needling therapy, rats in Groups C, D, and E were anesthetized by intraperitoneal injection of 10% chloral hydrate (3 mL/kg). Then, the positions of myofascial trigger points (MTrPs) in musculus gastrocnemius were located and marked. After sterilization, dry needles with 1.0 mm in diameter were injected into indicated MTrPs at a 30-degree angle with skin. The needle tails were connected to the heating device and heated for 20 mins. The temperature in Groups C and D was set at 44°C, while the temperature in Group E was set at 40°C (). Needles were withdrawn when interventions finished. Wound surfaces were covered with gauze for 3 mins for hemostasis. All procedures were performed at a temperature of 24°C.
Experimental Evaluation
Eight rats per group were selected from Groups B, C, D, and E and sacrificed at 24 hrs after the treatment. The remaining rats were sacrificed at 7 days after the treatment. The changes in transcription levels of target genes in rat gastrocnemius tissues were detected by real-time polymerase chain reaction (RT-PCR) analysis. Total RNA was isolated from muscle tissues using TRIzol reagent (Tiangen Biotech Co. Ltd., Beijing, China). The concentration of RNA was 2600.12 ng/μL. The quality of extracted RNA was evaluated using NanoDrop 2000 spectrophotometer (Thermo Fisher Scientific, Waltham, MA, USA), and total RNA content was 49.4 µg. Extracted RNA was reversely transcribed into cDNA using PrimeScript RT reagent kit (TaKaRa, Dalian, Liaoning, China). Two microliters of the cDNA were added into an 18-μL RT-PCR reaction system. The DNA amplification was carried out by the following procedure: 95°C for 30 s, 40 cycles (95°C for 5 s, 60°C for 40 s). Primer sequences for RT-PCR are summarized in . Glyceraldehyde phosphate dehydrogenase (GAPDH) was used as the invariant control, and the relative mRNA abundance of TRPV1, PKC, and IL-6 was expressed as fold changes after normalization to GAPDH. Amplified PCR products were electrophoresed on 1% agarose gel for verification.
Table 1 Primer Sequences
Statistical Analysis
All experimental data were presented as mean ± standard deviation (SD) and results were analyzed using SPSS 19.0 software. The significance of difference was determined by analysis of variance or by Rank-Sum tests when equal variances were not assumed. Values of P < 0.05 were considered to be statistically significant.
Results
As shown in and , compared with normal control rats, the mRNA expression of TRPV1 in untreated CMPS rats, CMPS rats receiving 44°C heating-conducting dry needling therapy and CMPS rats administrated with 40°C heating-conducting dry needling treatment was significantly increased (P<0.05). Compared with the untreated model group, TRPV1 mRNA abundance significantly increased by 44°C heating-conducting dry needling treatment group (P<0.05). Results also showed obvious higher TRPV1 mRNA expression in CMPS rats receiving sole 44°C heating-conducting dry needling treatment than in CMPS rats receiving capsazepine injection and 44°C heating-conducting dry needling treatment (P<0.05). Similar changes in TRPV1 expression were observed in rats at 7 days after interventions ( and ).
Figure 2 The effects of heat-conduction dry needling therapy on the expression of TRPV1 mRNA in musculus gastrocnemius lesions of CMPS rats. (A) The mRNA expression of TRPV1 at 1 day after the treatment. (B) The mRNA expression of TRPV1 at 7 days after the treatment.
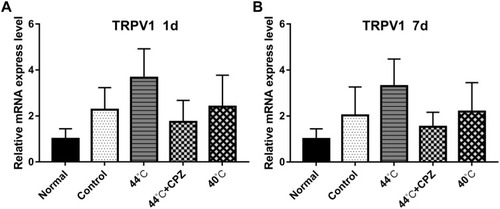
Table 2 The Comparison Of TRPV1 mRNA Expression In Rats Of Five Groups
and showed a significant increase in PKC mRNA expression in CMPS rats administrated with 44°C heating-conducting dry needling therapy, compared with healthy control rats or untreated CMPS rats (P<0.05). However, pre-injection of capsazepine impaired the induction of 44°C heating-conducting dry needling treatment on PKC mRNA expression (P<0.05). The results obtained at 7 days after treatments were in analog to these collected at 1 day after treatments ( and ).
Figure 3 The effects of heat-conduction dry needling therapy on the expression of PKC mRNA in musculus gastrocnemius lesions of CMPS rats. (A) The mRNA expression of PKC at 1 day after the treatment. (B) The mRNA expression of PKC at 7 days after the treatment.
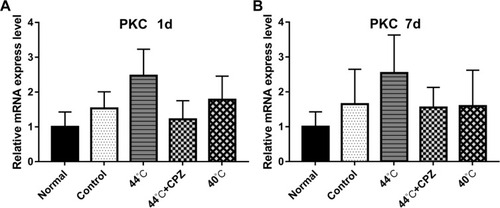
Table 3 The Comparison Of PKC mRNA Expression In Rats Of Five Groups
As seen in and , results showed that compared with healthy control rats, rats in the other four groups gained significant higher IL-6 mRNA expression (P<0.05). Compared with the untreated model group, 44°C heating-conducting dry needling treatment group distinctly enhanced the mRNA expression of IL-6 (P<0.05). The expression of IL-6 mRNA was higher in 44°C heating-conducting dry needling treatment group than that in 44°C heating-conducting dry needling treatment group combined with capsazepine injection and in 40°C heating-conducting dry needling treatment group (P<0.05). Different therapeutic periods gave similar results ( and ).
Figure 4 The effects of heat-conduction dry needling therapy on the expression of IL-6 mRNA in musculus gastrocnemius lesions of CMPS rats. (A) The mRNA expression of IL-6 at 1 day after the treatment. (B) The mRNA expression of IL-6 at 7 days after the treatment.
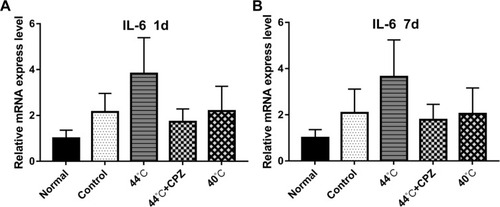
Table 4 The Comparison Of IL-6 mRNA Expression In Rats Of Five Groups
Discussion
TRPV1 channel, which is widely expressed in peripheral nerve fibers of rats and humans, is a critical part of the pain path that carries hazardous messages to the central nervous system.Citation5 TRPV1 is a multimodal channel that can respond to a variety of physical and chemical stimuli, such as heat, acidic pH, and mechanical stimuli.Citation20 Suppression of TRPV1 channel can relieve or even remove pain, while activation of TRPV1 can induce pain. However, desensitization will occur after consecutive activation of TRPV1, which also can reduce or even remove pain. TRPV1 not only plays a key role in physiological pain but also in inflammatory pain, such as thermal hyperalgesia, spontaneous pain, and mechanical pain.Citation21 The most core mechanism underlying the role of TRPV1 channel in chronic pain is to increase the hyperexcitability of primary nociceptive nerves and cause central sensitization and chronic pain.Citation22 Genetic and pharmacological studies have shown that TRPV1 channel was an essential part of cellular signal transductions and also a vital medium for induction of thermal hyperalgesia and allodynia by injuries.Citation23–Citation25 It was found that TRPV1 expression was up-regulated in multiple clinical pain-related diseases.Citation26
Persistent pain has a high clinical morbidity. Thus, it is of great essentiality to develop novel analgesic reagents. Previous studies have identified TRP channels as potential targets for pharmacological analgesia.Citation27 However, TRPV1 antagonists or agonists are unable to be applied in clinical pain relief due to the severe side effects, such as loss of thermal sensation, risk of burns, and hyperthermia, even though TRPV1 agonist capsaicin has been found effective in reducing the pain of patients with neurological diseases.Citation28 Systemic administration of AMG9810, a TRPV1 antagonist, triggered hyperpyrexia, a common side effect of TRPV1 antagonists, in trial animals.Citation29 A clinical study also showed that TRPV1 antagonist AMG517 induced prolonged fervescence in subjects, among which the highest body temperature reached above 40°C.Citation30 Herein, a majority of prior research on TRPV1 antagonists have been suspended due to the considerable adverse effects.Citation21 For example, AMG 517 did not meet its endpoint in Phase I clinical trials in human toothache due to hyperpyrexia occurring after TRPV1 inhibition.Citation31 Several studies tried to develop a promising drug for relieving pain without affecting basic thermosensitivity. Yet these potential drugs are far from clinical practices for now.Citation32,Citation33
In addition to agonists and antagonists, temperatures above 43°C can activate TRPV1 pathway as well. Intriguingly, TRPV1 will desensitize quickly within a few seconds or even milliseconds if thermal stimulus continuously exists, which is the main mechanism underlying the analgesic effect of high temperatures (above 43°C).Citation34 Thus, this study was aimed to explore a satisfactory method for relieving pain without inducing systematic thermoregulation disorder. For this purpose, regional heating was applied to regulate the activity of TRPV1 pathway.
Thermotherapy is a frequently used method for treating multiple pain diseases. Common thermotherapies include hot compress, thermomagnet, infrared radiation, microwave, spa, kerotherapy, moxibustion, etc. The mechanisms underlying the analgesic effects of thermotherapies mainly include accelerated bloodstream, enhanced metabolism, increased nutrition and oxygen supplies, accelerated tissue repair, increased collagenous tissue viscoelasticity and expanded range of motion.Citation35 Based on prior clinical experiences, heat-conduction dry needling therapy with needles heated to 44°C was applied in this study for treating CMPS and results showed promising therapeutic effects of the therapeutic strategy.Citation36 Originally, only the dry needle tails retained in vitro were heated due to technical limitations, which further heated the remaining needle bodies by heat conduction. However, this type of device could not heat deep tissues, causing distinct heating efficiencies in different tissues and organisms. Recently, internal-heated dry needles have drawn considerable attention due to the excellent performance in treating skeletal muscle injury-associated diseases like CMPS. This type of internal-heated dry needles adopts a multilayer structure with temperature feedback elements, with the outermost layer made of metal, the middle layer made of insulating materials, and the innermost layer made of resistance wires. The needle tails are linked to the heater via wires. The structure of the device could eliminate the impact of penetration depths and ensure the homogeneity of heating inside the needles. The temperatures can be precisely set with a fluctuation range of 1°C, which ensures the stability and controllability of heating in different study subjects. The well-designed device provided a solid technical basis for this study.
CMPS is a common musculoskeletal pain disease that can involve all skeletal muscles. The most distinct clinical feature of CMPS is MTrPs, hyper-reactive taut bands or nodules found in skeletal muscles.Citation37 In addition to causing local pain in head, trunk, limbs, and other local muscles, CMPS also leads to muscle rigidity, limited joint movement, myasthenia and balance disorder.Citation38 The morbidity of CMPS varies among different departments, such as 21% in department of orthopedics, 30% in department of internal medicine and 85–93% in the department of pain management.Citation39 Currently, CMPS models have been well established in a variety of animals for medical research. MTrPs also contribute to the determination of dry needling therapeutic sites. Thus, the CMPS rat model was used in this study for testifying our hypothesis.
This study showed that compared with healthy control rats, TRPV1 expression was significantly increased in CMPS rats. Compared with CMPS rats, TRPV1 expression was increased with no statistical significance in CMPS rats receiving dry needling therapy with needles heated to 40°C. Notably, TRPV1 expression was significantly higher in CMPS rats undergoing dry needling therapy with needles heated to 44°C than that in control rats and CMPS rats, which suggested that the therapy could modulate the activity of TRPV1 pathway. However, there was no statistical difference in TRPV1 mRNA abundance between untreated CMPS rats and CMPS rats injected with capsazepine and then treated with dry needling therapy at the heating temperature of 44°C, which suggested that TRPV1 activation could be a potential basis for heat-conduction dry needling therapy to work. The mRNA expression of PKC and IL-6 was also measured and similar varies to TRPV1 were observed, highlighting the correlation between PKC, IL-6, and TRPV1.
PKC, widely distributed in many tissues, organs, and cells, participates in neuropathic pain management in unphosphorylated and phosphorylated forms.Citation40 It was reported that PKC/NF-κB pathway in rat DRG was involved in inflammatory neuropathic pain,Citation41 where PKC activated NF-κB and cause persistent inflammatory pain.Citation42 TRPV1 is the main Ca2+ channel for activating PKC in sensory axons.Citation43 Intracellular PKC could be activated by TRPV1 and calmodulin signaling pathway after being cultured at 42°C for 30 mins.Citation16
This study also indicated that PKC mRNA expression was increased in CMPS rats when compared with that in control rats. Dry needling therapy with needles heated to 44°C significantly increased PKC mRNA abundance in CMPS rats, while capsazepine injection counteracted the effect, which was consistent with previous studies that TRPV1 could be a critical regulator of PKC.
Cytokines are key participants in the pathophysiological activities of inflammation, such as IL-6, IL-11, leukemia suppressor factor, oncostatin M, etc.Citation44,Citation45 Among these cytokines, IL-6 is a pleiotropic cytokine with diverse key functions in pain management. IL-6 expresses at a low level in healthy individuals but is highly expressed in patients with inflammatory pain diseases.Citation46,Citation47 Likewise, IL-6 mRNA expression was higher in CMPS than that in control rats in this study. As a classical inflammatory cytokine, IL-6 is also involved in anti-inflammation, immunoreaction, and metabolism.Citation48 Injection of IL-6 could induce hypersensitivity to thermal and mechanical stimuli,Citation49 while inhibition of IL-6 could relieve pain.Citation15 Besides, capsaicin could activate the TRPV1 channel and induce IL-6 expression at both transcriptional and translational levels in human upper respiratory tract epithelial cells.Citation50 In 2005, Zheng et al found that activation of PKC and its downstream molecules was essential for IL-6 to induce cell proliferation.Citation51 In 2006, Kovacs et al found that heating could affect the expression of IL-6 by modulating the TRPV1 channel.Citation52 In 2017, Obi et al investigated the interaction between TRPV1, PKC, and IL-6 and concluded the TRPV1/PKC/CREB/IL-6 pathway. Heating promoted the phosphorylation of TRPV1 channel, which consequently triggered an increase in intracellular calcium flow, PKC phosphorylation, CREB activation and then IL-6 up-regulation.Citation15
Our results revealed that the expression of IL-6 was remarkably increased in CMPS rats after the 44°C dry needling therapy, whereas the therapeutic action was eliminated when rats were pretreated with the TRPV1 antagonist capsazepine. These results suggested that IL-6 could be modulated by TRPV1, which was consistent with the results of previous studies.
Except for potential TRPV1 regulation, thermotherapies can also promote local blood circulation, accelerate metabolism, etc. Dry needling therapy is well recognized as an effective physical therapeutic strategy in Western countries. Thus, dry needling therapy with needles heated to 40°C was designed as a trial therapeutic strategy in this study, which was postulated to exhibit certain therapeutic effects like common dry needling therapy and thermotherapy. However, theoretically, TRPV1 could not be activated in this trial group because the temperature was below 43°C. Notably, our results showed that the mRNA expression of TRPV1 was not altered by 40°C dry needling therapy.
This study further validated the theory of the impacts of temperatures on the activity of TRPV1 pathway. According to our results, compared with heating dry needles to 40°C, heating dry needles to a higher temperature like 44°C significantly enhanced the expression of TRPV1 mRNA in rat local musculus gastrocnemius, which was consistent with the previous finding that temperatures higher than 43°C could effectively activate TRPV1 pathway. Moreover, patients who receive oral administration or injection of TRPV1 agonists or antagonists generally develop multiple systematic adverse effects, whereas the topical treatment in this study may prevent subjects from the side effects generated by extensive TRPV1 activation or inhibition. Hence, the current study provided a possibility of applying TRPV1 as a core target for clinical pain management. In our future studies, the alternations of pain-related behaviors of CMPS rats before and after the topical therapy will be investigated, including pressure pain threshold, thermoresistance, body temperatures, etc.
In this study, heat-conduction dry needling therapy, a kind of physical thermotherapy, was used to heating lesions to 44°C for treating CMPS and determining the role of TRPV1 pathway. Our results demonstrated that heat-conduction dry needling therapy altered the expression of TRPV1 mRNA and modulated its activity. These results provided a novel insight into the roles of temperature control and TRPV1 regulation in pain management and highlighted the potential of TRPV1 channel as a valuable therapeutic target in pain relief. Further studies would be focused on the application of heat-conduction dry needling therapy in other tissues or diseases.
There are several limitations to this study. Firstly, the results need to be verified by further experiments with a larger sample size due to limited samples of this study. Secondly, it remains unclear whether the results obtained from rats could be consistent with clinical trial results. Thirdly, the correlation between TRPV1 mRNA expression and therapeutic effects remained unclear.
Conclusion
Compared with control rats, the protein expression of TRPV1, PKC and IL-6 was increased in CMPS rats, which was further enhanced at 1 day and 7 days after 44°C dry needling therapy but not 40°C dry needling therapy. These results verified the feasibility of the application of heating-conducting dry needling therapy in regulating the TRPV1 pathway based on the theory of the regulatory function of temperatures above 43°C on TRPV1 activity. However, the increases in TRPV1, PKC and IL-6 mRNA abundance in CMPS rats receiving dry needling therapy with needles heated to 44°C were not observed in CMPS rats pretreated with TRPV1 antagonist capsazepine. These results suggested that dry needling therapy with needles heated to 44°C could regulate TRPV1 activity, but the regulatory effect could be blocked by capsazepine.
Disclosure
The authors report no conflicts of interest in this work.
References
- Bais B, Greenberg RM. TRP channels as potential targets for antischistosomals. Int J Parasitol. 2018;8(3):511–517. doi:10.1016/j.ijpddr.2018.08.003
- Mickle AD, Shepherd AJ, Mohapatra DP. Nociceptive TRP channels: sensory detectors and transducers in multiple pain pathologies. Pharmaceuticals (Basel). 2016;9(4):1–26. doi:10.3390/ph9040072
- Caterina MJ, Schumacher MA, Tominaga M, Rosen TA, Levine JD, Julius D. The capsaicin receptor: a heat-activated ion channel in the pain pathway. Nature. 1997;389(6653):816–824. doi:10.1038/398079349813
- Marwaha L, Bansal Y, Singh R, Saroj P, Bhandari R, Kuhad A. TRP channels: potential drug target for neuropathic pain. Inflammopharmacology. 2016;24(6):305–317. doi:10.1007/s10787-016-0288-x27757589
- Julius D. TRP channels and pain. Annu Rev Cell Dev Biol. 2013;29:355–384. doi:10.1146/annurev-cellbio-101011-15583324099085
- Patapoutian A, Tate S, Woolf CJ. Transient receptor potential channels: targeting pain at the source. Nat Rev Drug Discov. 2009;8(1):55–68. doi:10.1038/nrd275719116627
- Chugunov AO, Volynsky PE, Krylov NA, Nolde DE, Efremov RG. Temperature-sensitive gating of TRPV1 channel as probed by atomistic simulations of its trans- and juxtamembrane domains. Sci Rep. 2016;6:1–16. doi:10.1038/s41598-016-0001-828442746
- Grace MS, Dubuis E, Birrell MA, Belvisi MG. Pre-clinical studies in cough research: role of Transient Receptor Potential (TRP) channels. Pulm Pharmacol Ther. 2013;26(5):498–507. doi:10.1016/j.pupt.2013.02.00723474212
- Srebro D, Vuckovic S, Prostran M. Participation of peripheral TRPV1, TRPV4, TRPA1 and ASIC in a magnesium sulfate-induced local pain model in rat. Neuroscience. 2016;339:1–11. doi:10.1016/j.neuroscience.2016.09.03227687800
- Sui F, Zhou HY, Meng J, et al. A chinese herbal decoction, shaoyao-gancao tang, exerts analgesic effect by down-regulating the TRPV1 channel in a rat model of arthritic pain. Am J Chin Med. 2016;44(7):1363–1378. doi:10.1142/S0192415X1650076227785943
- MG LD L, Huh BK, Choi SS, et al. The effect of clonidine pretreatment on epidural resiniferatoxin in a neuropathic pain rat model. Acta Med Okayama. 2015;69(2):95–103. doi:10.18926/AMO/5333825899631
- Jeong KY, Seong J. Neonatal capsaicin treatment in rats affects TRPV1-related noxious heat sensation and circadian body temperature rhythm. J Neurol Sci. 2014;341(1–2):58–63. doi:10.1016/j.jns.2014.03.05424746025
- Gavva NR, Bannon AW, Hovland DN Jr., et al. Repeated administration of vanilloid receptor TRPV1 antagonists attenuates hyperthermia elicited by TRPV1 blockade. J Pharmacol Exp Ther. 2007;323(1):128–137. doi:10.1124/jpet.107.12567417652633
- Sugimoto N, Matsuzaki K, Katakura M, et al. Heat attenuates sensitivity of mammalian cells to capsaicin. J Biochem Mol Toxicol. 2019;33(5):1–7. doi:10.1002/jbt.22288
- Obi S, Nakajima T, Hasegawa T, et al. Heat induces interleukin-6 in skeletal muscle cells via TRPV1/PKC/CREB pathways. J Appl Physiol (1985). 2017;122(3):683–694. doi:10.1152/japplphysiol.00139.201627979980
- Obi S, Nakajima T, Hasegawa T, et al. Heat induces myogenic transcription factors of myoblast cells via transient receptor potential vanilloid 1 (Trpv1). FEBS Open Bio. 2019;9(1):101–113. doi:10.1002/2211-5463.12550
- Luo L, Wang Y, Li B, et al. Molecular basis for heat desensitization of TRPV1 ion channels. Nat Commun. 2019;10(1):1–12. doi:10.1038/s41467-018-07882-830602773
- Cheng L, Li P, Patel Y, et al. Moxibustion modulates sympathoexcitatory cardiovascular reflex responses through paraventricular nucleus. Front Neurosci. 2018;12:1–10. doi:10.3389/fnins.2018.0000129403346
- Beil H, Qiangmin H, Shushen T, Xiaoqiang Z. Spontaneous myoelectric phenomenon and histopathology of myofascial trigger points in rats. Chin J Sports Med. 2011;30(6):532–535.
- Jardin I, Lopez JJ, Diez R, et al. TRPs in pain sensation. Front Physiol. 2017;8:392. doi:10.3389/fphys.2017.0039228649203
- Hung CY, Tan CH. TRP channels in nociception and pathological pain. Adv Exp Med Biol. 2018;1099:13–27. doi:10.1007/978-981-13-1756-9_230306511
- Berta T, Qadri Y, Tan PH, Ji RR. Targeting dorsal root ganglia and primary sensory neurons for the treatment of chronic pain. Expert Opin Ther Targets. 2017;21(7):695–703. doi:10.1080/14728222.2017.132805728480765
- Basbaum AI, Bautista DM, Scherrer G, Julius D. Cellular and molecular mechanisms of pain. Cell. 2009;139(2):267–284. doi:10.1016/j.cell.2009.09.02819837031
- Caterina MJ, Leffler A, Malmberg AB, et al. Impaired nociception and pain sensation in mice lacking the capsaicin receptor. Science. 2000;288(5464):306–313. doi:10.1126/science.288.5464.30610764638
- Davis JB, Gray J, Gunthorpe MJ, et al. Vanilloid receptor-1 is essential for inflammatory thermal hyperalgesia. Nature. 2000;405(6783):183–187. doi:10.1038/3501207610821274
- Chen X, Duan Y, Riley AM, et al. Long-term diabetic microenvironment augments the decay rate of capsaicin-induced currents in mouse dorsal root ganglion neurons. Molecules. 2019;24(4):1–13.
- Weyer-Menkhoff I, Lötsch J. Human pharmacological approaches to TRP-ion-channel-based analgesic drug development. Drug Discov Today. 2018;23(12):2003–2012. doi:10.1016/j.drudis.2018.06.02029969684
- Szolcsanyi J, Sandor Z. Multisteric TRPV1 nocisensor: a target for analgesics. Trends Pharmacol Sci. 2012;33(12):646–655. doi:10.1016/j.tips.2012.09.00223068431
- Gavva NR, Bannon AW, Surapaneni S, et al. The vanilloid receptor TRPV1 is tonically activated in vivo and involved in body temperature regulation. J Neurosci. 2007;27(13):3366–3374. doi:10.1523/JNEUROSCI.4833-06.200717392452
- Gavva NR, Treanor JJ, Garami A, et al. Pharmacological blockade of the vanilloid receptor TRPV1 elicits marked hyperthermia in humans. Pain. 2008;136(1–2):202–210. doi:10.1016/j.pain.2008.01.02418337008
- Chizh BA, O’Donnell MB, Napolitano A, et al. The effects of the TRPV1 antagonist SB-705498 on TRPV1 receptor-mediated activity and inflammatory hyperalgesia in humans. Pain. 2007;132(1–2):132–141. doi:10.1016/j.pain.2007.06.00617659837
- Lehto SG, Tamir R, Deng H, et al. Antihyperalgesic effects of (R, E)-N-(2-hydroxy-2,3-dihydro-1H-inden-4-yl)-3-(2-(piperidin-1-yl)-4-(trifluorom ethyl)phenyl)-acrylamide (AMG8562), a novel transient receptor potential vanilloid type 1 modulator that does not cause hyperthermia in rats. J Pharmacol Exp Ther. 2008;326(1):218–229. doi:10.1124/jpet.107.13223318420600
- Reilly RM, McDonald HA, Puttfarcken PS, et al. Pharmacology of modality-specific transient receptor potential vanilloid-1 antagonists that do not alter body temperature. J Pharmacol Exp Ther. 2012;342(2):416–428. doi:10.1124/jpet.111.19031422570364
- Cui Y, Yang F, Cao X, Yarov-Yarovoy V, Wang K, Zheng J. Selective disruption of high sensitivity heat activation but not capsaicin activation of TRPV1 channels by pore turret mutations. J Gen Physiol. 2012;139(4):273–283. doi:10.1085/jgp.20111072422412190
- Malanga GA, Yan N, Stark J. Mechanisms and efficacy of heat and cold therapies for musculoskeletal injury. Postgrad Med. 2015;127(1):57–65. doi:10.1080/00325481.2015.99271925526231
- Wang G, Gao Q, Hou J, Li J. Effects of temperature on chronic trapezius myofascial pain syndrome during dry needling therapy. Evid Based Complement Alternat Med. 2014;2014:1–9.
- Yildirim MA, Ones K, Goksenoglu G. Effectiveness of ultrasound therapy on myofascial pain syndrome of the upper trapezius: randomized, single-blind, placebo-controlled study. Arch Rheumatol. 2018;33(4):418–423. doi:10.5606/ArchRheumatol.2018.653830874250
- Saxena A, Chansoria M, Tomar G, Kumar A. Myofascial pain syndrome: an overview. J Pain Palliat Care Pharmacother. 2015;29(1):16–21. doi:10.3109/15360288.2014.99785325558924
- Parthasarathy S, Sundar S, Mishra G. Assessment of predisposing factors in myofascial pain syndrome and the analgesic effect of trigger point injections – a primary therapeutic interventional clinical trial. Indian J Anaesth. 2019;63(4):300–303. doi:10.4103/ija.IJA_6_1931000895
- Xie JD, Chen SR, Chen H, Pan HL. Bortezomib induces neuropathic pain through protein kinase C-mediated activation of presynaptic NMDA receptors in the spinal cord. Neuropharmacology. 2017;123:477–487. doi:10.1016/j.neuropharm.2017.06.02728663117
- Zhao R, Pei GX, Cong R, Zhang H, Zang CW, Tian T. PKC-NF-kappaB are involved in CCL2-induced Nav1.8 expression and channel function in dorsal root ganglion neurons. Biosci Rep. 2014;34(3):1–9. doi:10.1042/BSR20140005
- Souza GR, Cunha TM, Silva RL, et al. Involvement of nuclear factor kappa B in the maintenance of persistent inflammatory hypernociception. Pharmacol Biochem Behav. 2015;134:49–56. doi:10.1016/j.pbb.2015.04.00525902407
- Johnstone AD, de Leon A, Unsain N, Gibon J, Barker PA. Developmental axon degeneration requires TRPV1-dependent Ca(2+) influx. eNeuro. 2019;6(1):1–15. doi:10.1523/ENEURO.0019-19.2019
- Yoshida K, Taga T, Saito M, et al. Targeted disruption of gpl30, a common signal transducer for the interleukin 6 family of cytokines, leads to myocardial and hematological disorders. Proc Natl Acad Sci U S A. 1996;93(1):407–411. doi:10.1073/pnas.93.1.4078552649
- Betz UA, Bloch W, van den Broek M, et al. Postnatally induced inactivation of gp130 in mice results in neurological, cardiac, hematopoietic, immunological, hepatic, and pulmonary defects. J Exp Med. 1998;188(10):1955–1965. doi:10.1084/jem.188.10.19559815272
- Kiefer R, Kieseier B, Stoll G, Hartung HP. The role of macrophages in immune-mediated damage to the peripheral nervous system. Prog Neurobiol. 2001;64(2):109–127.11240209
- Smith PC, Hobisch A, Lin DL, Culig Z, Keller ET. Interleukin-6 and prostate cancer progression. Cytokine Growth Factor Rev. 2001;12(1):33–40.11312117
- Pedersen BK. Muscle as a secretory organ. Compr Physiol. 2013;3(3):1337–1362. doi:10.1002/cphy.c12003323897689
- Brenn D, Richter F, Schaible HG. Sensitization of unmyelinated sensory fibers of the joint nerve to mechanical stimuli by interleukin-6 in the rat: an inflammatory mechanism of joint pain. Arthritis Rheum. 2007;56(1):351–359. doi:10.1002/art.2228217195239
- Seki N, Shirasaki H, Kikuchi M, Himi T. Capsaicin induces the production of IL-6 in human upper respiratory epithelial cells. Life Sci. 2007;80(17):1592–1597. doi:10.1016/j.lfs.2007.01.03717306835
- Zheng X, Li AS, Zheng H, Zhao D, Guan D, Zou H. Different associations of CD45 isoforms with STAT3, PKC and ERK regulate IL-6-induced proliferation in myeloma. PLoS One. 2015;10(3):1–18.
- Kovacs D, Eszlari N, Petschner P, et al. Interleukin-6 promoter polymorphism interacts with pain and life stress influencing depression phenotypes. J Neural Transm (Vienna). 2016;123(5):541–548. doi:10.1007/s00702-016-1506-926821321