Abstract
Peripheral nerve injury is a worldwide clinical problem which could lead to loss of neuronal communication along sensory and motor nerves between the central nervous system (CNS) and the peripheral organs and impairs the quality of life of a patient. The primary requirement for the treatment of complete lesions is a tension-free, end-to-end repair. When end-to-end repair is not possible, peripheral nerve grafts or nerve conduits are used. The limited availability of autografts, and drawbacks of the allografts and xenografts like immunological reactions, forced the researchers to investigate and develop alternative approaches, mainly nerve conduits. In this review, recent information on the various types of conduit materials (made of biological and synthetic polymers) and designs (tubular, fibrous, and matrix type) are being presented.
Introduction
Peripheral nerve injury is a worldwide clinical problem that impairs the patient’s quality of life. The central nervous system (CNS) consists of the brain and the spinal cord. The peripheral nervous system, on the other hand, is located outside the CNS and includes the cranial, spinal and peripheral nerves that conduct impulses from and to the CNS. Injury in peripheral nerves could lead to loss of neuronal communication along sensory and motor nerves between the CNS and the peripheral organs. Peripheral nerve injuries often result in painful neuropathies via reduction in motor and sensory functions and can be catastrophic for patients, drastically affecting their daily activities.Citation1 Peripheral nerves are composed of bundles of nerve fibers and surrounding connective tissue sheaths including blood vessels (). Each individual nerve fiber and the supporting Schwann cells are surrounded by a loose connective tissue, the endoneurium. A bundle of nerve fibers is held by collagen fibrils in the connective tissue and form fascicles surrounded by a dense connective tissue called the perineurium. Entire nerve fascicles within a nerve trunk are completely ensheathed by a dense and irregular connective tissue called epineurium, which is the outermost layer of connective tissue sheaths.
In general, peripheral nerve injuries are caused by mechanical, thermal, chemical, or ischemic damages resulting mainly from traumatic accidents or some degenerative disorders. The severity of the injury determines the functional outcome. Peripheral nerve injury is commonly assessed according to the Seddon and the Sunderland classifications.Citation2–Citation4 SeddonCitation2 categorized the injuries in increasing severity as neurapraxia, axonotmesis, and neurotmesis.Citation2 A few years later, Sunderland classified them as 1–5 degree injuries.Citation3 In Sunderland’s first-degree injury that corresponds to neurapraxia, axons are anatomically intact and Wallerian degeneration is absent, but there is partial demyelination and impulses cannot be transmitted. These injuries recover with treatment within a few months. In Sunderland’s second-degree injury, which corresponds to axonotmesis in the Seddon classification, the endoneurium and Schwann cells are intact but the axon is severed. These injuries are regenerated by the aid of intact endoneurium. In the third-degree injury, even though the perineurium and fascicular arrangement is preserved, the endoneurium is disrupted. In these situations, fibrosis can take place within fascicles, and recovery of motor and sensory function can be significantly delayed. In fourth-degree injury, axons, endoneurium, and perineurium are disrupted, while only the outermost layer, the epineurium, is intact. There is a significantly higher degree of degeneration compared with lower-degree injuries; therefore, removal of scar tissue and surgical repair of the nerve may be needed to achieve regeneration. Sunderland’s fifth degree injury corresponds to neurotmesis, where the entire nerve trunk is transected completely and there is a scar formation. As a result, neuroma and Wallerian degeneration are formed at the proximal and distal ends, respectively. In such severe injuries, surgical repair is required.
In peripheral nerve injuries that lead to axonal disruption or nerve transection, degenerative changes such as axon and myelin breakdown are initiated at the proximal and distal end of the lesion site.Citation5 The distal end is disconnected from the neural body and undergoes Wallerian (anterograde) degeneration (). The axonal cytoskeleton components are disassembled and the axon is fragmented. These events lead to the breakdown of myelin sheath. On the other hand, a series of cellular and molecular changes, retrograde reactions, and chromatolysis occur in the damaged neuronal cell body, and associate with retrograde axonal degeneration in the proximal nerve stump. Schwann cells and infiltrating macrophages serve in the removal of axonal, myelin, and tissue debris. In addition, Schwann cells proliferate and are aligned along external lamina to form bands of Büngner which guide the regenerative axonal sprouts that originate from the severed proximal end of the axon. New axonal sprouts undergo myelination, and these regenerated axons reach their target to attain functional recovery. The ideal progress of axonal regrowth from the proximal end is 1 mm/day. However, if the axonal sprouts fail to cross the injury site, this leads to formation of neuroma and the denervated muscle fiber becomes atrophic.
Treatment strategies for nerve injuries
Although the peripheral nerves have a capacity to regenerate after injury, this spontaneous nerve repair may not be sufficient to achieve proper functional recovery. The length of the nerve gap, the time passed between the injury and repair, and the patient’s age are important parameters to be considered in the repair of peripheral nerve injuries. The primary medical therapy for complete lesions is a tension-free, end-to-end repair by suturing of nerve stumps via epineurial and/or group fascicular suturing. In the case of a significant nerve gap formation where end-to-end repair is not possible, peripheral nerve grafts or nerve conduits are required to serve as a bridge between the nerve stumps across the gap and to support axonal regrowth. presents a scheme that classifies the peripheral nerve grafts and the nerve conduit materials used.
Peripheral nerve grafts
Autografts
The most widely used nerve repair strategy, considered the “gold standard,” to bridge nerve gaps is the use of autografts, harvested from the patient’s own body but from another location. Nerve autografts have been studied extensively, and their superiority over epineurial suturing under tension has been reported.Citation6 Nerve autografts provide a structural guidance of the natural material for axonal progression from the proximal to the distal nerve stumps. Functionally less important nerves like sural nerves, superficial cutaneous nerve, or lateral and medial antebrachii cutaneous nerves are chosen as donor sites for autograft nerve tissue.Citation7 However, there are significant limitations in the use of nerve autografts, such as causing a second surgery site to harvest tissue from the donor site, which is associated with donor site morbidity and loss of function.Citation5 Therefore, the availability and the length of nerve that can be harvested are limited. Use of autografts is currently restricted to critical nerve gaps of nearly 5 cm length.Citation8 Mismatch of donor nerve size and fascicular inconsistency between the autograft and the proximal and the distal stumps of the recipient site are the main limitations in the use of nerve autografts. In fact, the type of nerve autografts chosen, like sensory nerves, motor nerves, or mixed nerves, is also important for a successful outcome, since a mismatch in axonal size, distribution, and alignment limits the regeneration capacity of the autografts. Nichols et alCitation9 reported that motor or mixed-nerve autografts are superior in axon regeneration than the sensory nerve autografts. Other important drawbacks of nerve autografts are the potential of infection and formation of painful neuroma. In addition, the recovery time for the patient can be prolonged, owing to the need for a second surgery.
The limitations of autografts forced the researchers to investigate and develop alternative approaches such as manufacturing novel nerve conduits for peripheral nerve injury.
Allografts
Nerve allograft, is a technique used to bridge a peripheral nerve lesion with tissues derived from a different individual of the same species. An allograft nerve tissue serves as a support for guidance and a source for viable donor-derived Schwann cells that would facilitate the connection of axons at the proximal and distal ends to achieve reinnervation of target tissue or organs.Citation7 However, the use of allografts presents limitations including especially immune rejection, risk of cross contamination, secondary infection, and limited supply. Therefore, the use of allografts requires systemic immunosuppressive therapy, but long-term immune suppression is not a desirable treatment due to increased risk of infection, decrease of healing rate, and it occasionally results in tumor formation and other systemic effects. In order to overcome some of these limitations, nerve allografts can be processed by repeated freeze–thaw cycles, irradiation, and decellularization with detergents.
Xenografts
A nerve xenograft is obtained from a member of a species other than that of the recipient.Citation10 In 1997, Hebebrand et alCitation11 transplanted 2 cm sciatic nerve xenografts obtained from Golden Syrian hamsters into a 0.5 cm gap in the sciatic nerve of Lewis rats. The performance of this grafting procedure was assessed by walking track analysis, by measuring somatosensory-evoked potentials, and by performing histology across the nerve xenografts. The test models were immunosuppressed with RS-61443 and FK-506, and non-immunosuppressed animals were used as controls. The functional recovery in the test animals was found to be not as good as those in the control isografts. Jia et al,Citation12 on the other hand, used acellular nerve xenografts and seeded them with bone marrow stromal cells (BMSCs). The xenografts were obtained from Sprague Dawley rats and New Zealand rabbits and introduced to 1 cm rat sciatic nerve gaps. When the allograft and the xenograft were compared with electro-physiological studies, it was observed that the xenografts were as effective as the allografts in regenerating the neurons. However, the risk of cross-species disease transmission would be considered for the use of xenografts.
Nerve conduits
Decellularized nerve conduits
Allografts and xenografts have the potential to induce immunogenic reactions in the host tissue. In order to suppress the immunogenic reactions, the grafts are used in combination with immunosuppressive drugs.Citation13 However, the use of these drugs may cause more susceptibility to infections and tumor formation.Citation14 Therefore, to eliminate the cellular constituents that cause immunogenic reactions but to preserve the native extracellular matrix (ECM) that is known to enhance the regenerative capacity, “decellularization methods” have been developed.Citation15 Preservation of the ECM, which is conserved among the various species together with the basal lamina of allografts or xenografts, creates a means for mechanical guidance for the regenerating axons. Decellularization processes include various physical and chemical methods as well as enzymatic methods. Widely used physical methods are lyophilization, direct pressure, sonication, and agitation.Citation16 Freezing nerve tissue causes disruption of cell membranes and results in cell lysis.Citation17 During the freezing step, disruption of the ECM caused by rapid freezing that produces ice crystals should be avoided. Application of direct pressure is another method that has been used to decellularize the grafts. Mechanical agitation and sonication have been used together with chemical treatment to disrupt cell membranes.Citation18,Citation19 Chemical methods used with or without physical treatments include processes with alkaline and acid solutions,Citation20,Citation21 non-ionic, ionic, and zwitter ionic detergents,Citation15,Citation22–Citation24 and hypotonic and hypertonic solutions.Citation25,Citation26 Treatment with acidic or alkaline solutions solubilizes cell components and disrupts nucleic acids. Ionic detergents like sodium dodecyl sulfate and Triton X-200 also solubilize cellular components and denature proteins. However, nonionic detergents like Triton X-100 leave protein–protein interactions intact, and this is not desirable. Hypotonic and hypertonic solutions like solutions of ethylenediamine tetraacetic acid (EDTA) result in osmotic shock and lead to cell lysis. Solutions like EDTA are generally used in conjunction with treatments involving enzymes such as exonucleases, endonucleases, and trypsin.Citation27–Citation29 The requirement for the removal of any remaining chemicals, which may cause cell damage in the host tissue after implantation, may be considered a disadvantage of decellularized graft materials. Trypsin proteolysis as an enzymatic degradation method has been widely used to decellularize dermis or heart valves, although the stability of ECM may be limited with the alteration of collagen content after trypsin treatment.Citation23,Citation30
Various studies have shown that decellularized grafts are able to promote regeneration in defects more than 1–2 cm long in a rat sciatic model.Citation31–Citation33 A study showed that axons do not always reach the distal segment when decellularized nerve conduits are used in the treatment of defects over 1–2 cm without the support of other components like chondroitinase.Citation34 In that study, it was found that 4 cm long lyophilized, decellularized nerve allografts with chondroitinase could support regeneration along their full length. AxoGen Inc. (Alachua, FL, USA) received US Food and Drug Administration (FDA) approval for this decellularized allograft (Avance®). Whitlock et alCitation35 compared Avance® with another FDA-approved commercial product Neuragen® which is a collagen-based device (discussed later in Section “Biological nerve conduits – proteins”). The performance of Avance® was evaluated in a 14 mm sciatic nerve-gap model, and it was found to be as good as Neuragen® and autografts demonstrated almost no difference after 12 weeks. In 2009, Avance® was evaluated in clinical studies, and grafts of 0.5–30 mm length were surgically implanted to two dorsal and eight digital sensory nerves of five men and two women. Evaluation based on infection, rejection, and graft extrusion revealed that nerve sensations were restored within 9 months. Sun et alCitation36 investigated the effects of a decellularized allogeneic artery conduit containing autologous, transdifferentiated, adipose-derived stem cells (dADSCs) on an 8 mm long facial nerve branch lesion in a rat model. After 8 weeks, functional evaluation of vibrissae movements and electrophysiological assessment, retrograde labeling of facial motor neurons, and morphological analysis of regenerated nerves were performed to assess regeneration. Use of decellularized allogeneic artery conduits with autologous dADSCs showed definite beneficial effects on nerve regeneration and functional restoration. Zhao et alCitation37 reported nerve regeneration achieved through the use of a decellularized nerve graft by supplementation with BMSCs in fibrin. In that study, mesenchymal stem cells (MSCs) injected around the graft helped improve nerve regeneration and functional recovery of peripheral nerve lesions as determined by functional analysis and histology.
Manufactured nerve conduits
Cylindrical nerve grafts have been used for nerve repair as early as 1879, with the first application of bone tube used as a nerve conduit.Citation38 Because of the scar formation, however, the experiments failed. The advantages of using nerve grafts are 1) limited myofibroblast infiltration, 2) reduced scar formation, and 3) guidance of regenerating nerves.Citation39 Because of their limitations such as the potential to cause donor site morbidity, researchers have started designing artificial nerve conduits by using synthetic and biological polymers as an alternative treatment. An ideal nerve conduit needs to have properties like biocompatibility, biodegradability, flexibility, high porosity, compliance, neuroinductivity, neuroconductivity with appropriate surface, and mechanical properties.Citation40 The nerve conduit may be designed in different ways; they could be cylindrical tubes with internal channels or matrix, porous walls, or cell incorporation, and the design may include bioactive agents (). Polymeric materials having the right properties have been studied and used as nerve conduits for peripheral nerve regeneration.Citation41,Citation42
Biological nerve conduits
Bioactive properties of the biological polymers used in tissue engineering and in other implants allow better interactions between the cells and the scaffolds which enhance proliferation of the cells and regeneration of tissues. Although high biocompatibility of the biological polymers makes them good candidates for use as nerve conduits, they have some limitations like batch-to-batch variation.Citation42 Among the biopolymers commonly used in nerve regeneration studies are polyesters (poly(3-hydroxybutyrate) [P3HB] and poly (3-hydroxybutyric acid-co-3-hydroxyvaleric acid)), proteins (silk, collagen, gelatin, fibrinogen, elastin, and keratin), and polysaccharides (hyaluronic acid, chiti, and alginate) constitute the majority. In , the structure of some biological polymers are presented.
Figure 5 Biological and synthetic polymers used in the structure of nerve conduits.
Abbreviations: P3HB, poly(3-hydroxybutyrate); PCL, poly(ε-caprolactone); PGA, poly(glycolic acid); PHBV, poly(3-hydroxybutyrate-co-3-hydroxyvalerate); PLA, poly(lactic acid); PU, polyurethane; PVA, polyvinyl alcohol.
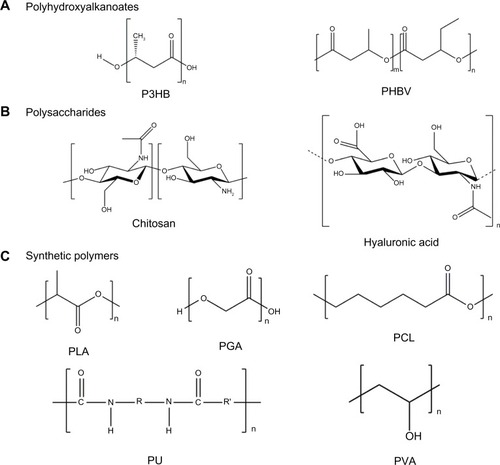
Polyesters
A variety of biological polyesters are obtained from microorganisms. Polyhydroxyalkonates (PHAs) are a class of biodegradable and biocompatible, synthetic, thermoplastic polyesters that are produced by various microorganisms, such as soil bacteria, blue-green algae, and also by some genetically modified plants ().Citation43 The natural role of PHAs in microorganisms is to serve as an intracellular energy and carbon storage product. The PHAs used in the tissue engineering applications are poly(4-hydroxy-butyrate), polyhydroxyvalerate, polyhydroxyhexanoate, polyhydroxyoctanoate, and P3HB. One of the most extensively studied PHAs in nerve regeneration is P3HB,Citation44 and another is poly(3-hydroxybutyrate-co-3-hydroxyvalerate) (PHBV).
P3HB
P3HB () is used as a bacterial storage product and can be commercially obtained as resorbable sheets, particles, and films. It has been used in peripheral nerve regeneration studies for more than 2 decades. Wrapping polyhydroxy-butyrate (PHB) sheets around nerve defects was shown to help regeneration of axons in cats.Citation45 Aberg et alCitation46 performed a clinical trial with P3HB as a wrap around the epineurial axons and compared it with epineural suturing. Tests of up to 18 months showed that P3HB yielded better results than epineurial suturing with no adverse effects. ECM proteins, Schwann cells, and nerve growth factors (NGFs) have been incorporated in order to improve the regenerative ability of the P3HB nerve conduits.Citation47,Citation48 In the study of Mohanna et al,Citation49 a P3HB conduit containing alginate hydrogel, or alginate and glial growth factor together, were used to bridge gaps 2–4 cm long in a rabbit common peroneal nerve model. PHB conduits served as a support in nerve regeneration for up to 63 days, bridging a 2 cm gap of axons. In another study, P3HB sheets together with a fibrin matrix were used to form a nerve conduit around an intravenous cannula.Citation50 These constructs seeded with adipose-derived stem cells (ADSCs) showed regenerative capacity. The regenerative role of the transplanted ADSCs was explained to be through the released growth factors and/or by the activity of the endogenous Schwann cells. In a more recent study, Masaeli et alCitation51 fabricated electrospun scaffolds by blending P3HB and PHBV in different proportions in order to investigate their potential for the regeneration of the myelinic membrane. Schwann cells grown on parallel PHB/PHBV/collagen fibers exhibited a bipolar morphology along the fiber direction, while Schwann cells grown on randomly oriented fibers had a multipolar morphology. It was concluded that aligned PHB/PHBV electrospun nanofibers could find potential use as scaffolds in nerve tissue engineering applications and presence of type I collagen in the nanofibers improves cell differentiation.
PHBV
Copolymers of 3-hydroxybutyrate and 3-hydroxyvalerate are the most widely used PHBV polymers due to our ability to tailor their physical characteristics according to our needs and their processability (). Incorporation of 3-hydroxyvalerate into the polymer chains leads to an increase in chain flexibility, and to a decrease in both the glass transition temperature and the melting temperature. This also improves the processability of the polymer. These properties make these polymers very suitable for use in nerve regeneration studies along with other tissue engineering applications. For example, a nanofibrous PHBV tube carrying Schwann cells was used as an artificial nerve graft for a 30 mm gap in a rat sciatic nerve model.Citation52 This polymeric conduit had sufficiently high mechanical properties to serve as a nerve guide. The results demonstrated that the sciatic nerve trunk had been reconstructed with restoration of nerve continuity and formatted nerve fibers with myelination.
Chitosan crosslinked nanofibrous PHBV nerve guides produced by electrospinning were tested in a rat sciatic nerve regeneration model across a 10 mm gap defect.Citation53 Prabhakaran et alCitation54 fabricated random and aligned nanofibers of PHBV and composite PHBV/collagen nanofibers. The diameters of the PHBV and composite fibers were in the range 386–472 nm and 205–266 nm, respectively. Aligned nanofibers of the composite showed contact guidance that led to the orientation of nerve cells along the direction of the fibers. This resulted in elongated cell morphology, with bipolar neurite extensions which were required for nerve regeneration.
Proteins
Scaffolds constructed of ECM proteins (collagen, fibrin, fibronectin, and hyaluronic acid) and using neurotropic factors have been extensively studied for peripheral nerve regeneration. In the preparation of nerve conduits, ECM proteins and other types of proteins like silk fibroin have been used alone or in combination with each other for more than 3 decades. Some of these studies involving various proteins are summarized below.
Collagen
Collagens are a family of 26 proteins, have a triple helical structure in the form of an extended rod, and they are the major components of the ECM. They have a high content of proline and hydroxyproline, and also a glycine at every third position.Citation55 Collagen, mainly type I, constitutes approximately 30% of the mammalian musculoskeletal tissues, and is one of the oldest natural polymers to be used as a biomaterial. Collagens exist as fibrils in the endoneurium or as the non-fibrillar component of the basal lamina and are appropriate materials to be used as the nerve conduits.Citation56 Fibrous constructs, hydrogels, particles, and foams are the different three-dimensional (3D) structures that can be produced from collagen Type 1.Citation57 Acid soluble collagen has been used to produce a hydrogel scaffold which was a very low density 3D lattice of nanofibrils produced by a combination of compression and blotting using layers of mesh and paper sheets.Citation58 The production method was used to obtain fibrils by unconfined plastic compression of hyperhydrated collagen gels. These scaffolds composed of aligned nanofibrils would have a significant potential for use in the regeneration of organized tissues like peripheral nerves. In a recent study, hydrogels of collagen Type 1 were used as the starting material, and after plastic compression, fibrillar collagen sheets, which were then rolled into 3D nerve conduits were formed.
There are a number of FDA-approved collagen-based materials to be used as nerve grafts such as NeuraGen®, NeuroFlex™, NeuroMatrix™, NeuraWrap™, and Neuro-Mend™. summarizes the FDA-approved nerve conduits. The first Type 1 collagen nerve guide conduit approved by the FDA, in 2001, was NeuraGen (Integra LifeSciences Co, Plainsboro, NJ, USA). Manufacturing process of these nerve conduits includes the preservation of the natural fibrillar structure of the collagen and construction of a tubular matrix from those fibrillar sheets.Citation59,Citation60 The first trials on the efficacy of NeuraGen® were performed by Mackinnon et alCitation60 who observed the absence of compression neuropathy, which was generally seen with more rigid materials. Similar trials made by Archibald et alCitation59 using NeuraGen® entubulation approach showed repair of peripheral nerves in 4 weeks.
Table 1 Commercially available FDA-approved nerve conduits
Two different collagen Type 1 nerve guidance conduit devices, NeuroMatrix™ and Neuroflex™ (Collagen Matrix, Inc., Franklin Lakes, NJ, USA), received FDA approvals in 2001. Both conduits are resorbable, flexible, non-friable, semipermeable tubular matrices with a pore size in the range 0.1–0.5 μm to allow for nutrient transfer. Both conduits are resorbed in vivo within 4–8 months. The only difference between the two conduits is that NeuroMatrix™ does not have the kink resistant property of Neuroflex™. Both devices, however, were designed to be used in nerve-gap defects shorter than 2.5 cm.Citation61 The other Type 1 collagen based nerve conduit is NeuraWrap™ (Integra LifeSciences Co) having the same composition with NeuraGen®. It received FDA approval in 2004. NeuraWrap™ was designed to prevent neuromas during regeneration and to be used by wrapping around the injured nerves. The wall structure of the device consists of a longitudinal slit. According to the manufacturers, the advantages of the device are 1) minimal scar formation because the porous outer membrane mechanically resists compression by surrounding tissues, 2) minimal encapsulation and nerve entrapment, and 3) the ability to create an environment suitable for regeneration as a result of the semipermeable inner membrane allowing nutrient transport.Citation62
NeuroMend™ (Collagen Matrix, Inc.) is a recent device composed of Type 1 collagen, having the same structure as the others, and was approved by the FDA in 2006. Although NeuroMend™ is a semipermeable, collagen-based wrap, it was designed to be unrolled and self-curled to best match the dimensions of the injured tissue.Citation63 Moreover, the semipermeable membrane allows diffusion of the nutrients while preventing the migration of fibroblasts and inflammation.Citation64,Citation65
The approved Type 1 collagen devices are used in the clinics. Research on pure or blended collagen devices, however, is continuing. Collagen nerve conduits releasing neurotrophic factors, such as glial cell–derived neurotrophic factor (GDNF) and NGF, were prepared by electrospinning using the spinning mandrel approach.Citation66 In this study, delivery of synergistically acting GDNF and NGF from the collagen nerve conduit resulted in a more successful repair of peripheral nerve defects compared with commercial products NeuraGen® and Neurolac®. Yang and ChenCitation67 investigated composite scaffolds prepared by blending and crosslinking chitosan with collagen and icariin. These scaffolds proved to be suitable cell carriers for use in nerve regeneration applications. More recently, micropatterned tubular collagen matrices produced by spin casting were reported to have a regenerative capacity when used in a rat sciatic nerve model.Citation68
Gelatin
Gelatin, is denatured collagen, and has been widely used in tissue engineering applications after crosslinking with various chemicals.Citation69 Genipin is a crosslinking agent with low cytotoxicity.Citation70 Gamez et alCitation71 prepared photofabricated, gelatin-based nerve conduits and implanted them between the proximal and distal stumps of a 10 mm dissected right sciatic nerve of adult male Lewis rats for up to 1 year. As a result, functional, morphological, and electrophysiological response recovery were observed. In another study, genipin crosslinked gelatin conduits were evaluated as a guidance channel for peripheral nerve regeneration, and the successful functional recovery of a 10 mm gap was tested in a rat sciatic model.Citation72 LiuCitation73 fabricated a proanthocyanidin crosslinked gelatin conduit with a rough outer surface. The conduit was used in the regeneration of a 10 mm gap in a rat sciatic model, and regenerated nerve fibers crossing through and beyond the gap region were shown in 8 weeks. Nie et alCitation74 tested gelatin- and chitosan-based nerve conduits with transforming growth factor β1 in a 10 mm gap in the sciatic nerve of rats. After the implantation, the inner surface of the conduits remained intact during the regeneration time; thus, it could prevent the ingrowth of connective tissues. Functional recovery, electrophysiological test, retrograde labeling, and immunohistochemistry analysis showed similar nerve conduction velocity, regenerated myelin area, and myelinated axon count with those treated with an autograft.
Fibronectin
Fibronectin, one of the major ECM proteins, is a disulfide-linked glycoprotein. It has important roles in cell adhesion, morphology, migration, and differentiation, and interacts with collagen, heparin, fibrin, and cell surface receptors.Citation75 In the early 1990s, guides produced from fibronectin under unidirectional shear were seen to lead to the orientation of cells along fibronectin pattern.Citation76,Citation77 Fibronectin was also used as a source for the release of supportive materials in nerve guidance conduits.Citation75 In addition, the oriented strands of the cell adhesive fibronectin were prepared and used to bridge a 10 mm defect in the rat sciatic nerve. It was concluded that the oriented fibronectin was a material suitable for successful nerve repair and has potential for use in the clinics. In the 2000s, it was shown that fibronectin mats are successful guidance substrates for the outgrowth of neurites in injured rat sciatic nerve.Citation78 Addition of fibronectin to alginate matrices was shown to improve peripheral nerve regeneration in tissue engineered conduits.Citation79 Moshahebi et al studied how the addition of fibronectin to alginate would affect the outcome of nerve regeneration and showed that the presence of fibronectin supported Schwann cell viability and augmented its effect on axonal growth. Ding et alCitation80 reported that coating the collagen scaffold with laminin and fibronectin increased its effectiveness in axon regeneration when tested 40 days after surgery for functional properties, using recovery electro-physiology and sciatic nerve functional index evaluation.
Silk fibroin
Silk is made of fibrous proteins synthesized by members of the class Arachnida and in the specialized epithelial cells that line the glands in worms of mites, butterflies, and moths. Silk fibroin is composed of repetitive protein sequences.Citation81 It has hydrophobic domains of short side chain amino acids, and therefore, the general structure of silk fibroin is in the form of β-sheets. The assembly of silk and its strength originate from these hydrophobic regions interspaced with small hydrophilic segments.Citation82 When compared with other protein-based biomaterials, there are many advantages of using silk such as the risk of infection and possibility of rejection of the other materials. Another significant advantage is the high mechanical properties such as modulus, breaking strength, and elongation. Biocompatibility, water-based processing, and biodegradability are the other advantages of silk for it to be considered in tissue engineering applications. The supportive capacity of silk fibroin for dorsal root ganglia and Schwann cells was shown by Yang et al.Citation83 A scaffold composed of fibroin and spider silk was shown to be a good material to bridge a gap of up to 13 mm within 12 weeks.Citation84 In 2013, Gu et alCitation85 reported the use of scaffolds modified with ECM produced by Schwann cells. The conduit was prepared from chitosan and silk fibroin fibers and then seeded with Schwann cells for ECM deposition. The morphological and electrophysiological analyses indicated that regenerative outcomes achieved by the developed scaffold were similar to those obtained with an acellular nerve graft but superior to those prepared by a plain chitosan–silk fibroin scaffold. In a recent study, spider silk proteins were also recommended for use in nerve regeneration applications because they support cell proliferation and regeneration.Citation86
Keratin
Keratin is a protein produced by keratinocytes. Cysteine in the structure is rich in sulfur, and it plays a role in the cohesion of the hair.Citation87 The hair fibers are mainly made up of cross-linked keratins, and these crosslinks can be broken and the free keratin molecules could be used in the construction of biomaterials.Citation88,Citation89 Sierpinski et alCitation90 oxidized keratin crosslinks to form hydrogels. These keratin hydrogel nerve conduits were compared with sensory nerve autografts, and it was concluded that the keratin-based biomaterials also had neuroinductive capacity. Lin et alCitation88 filled a conduit with keratin gel and studied its performance in a rat 15 mm sciatic nerve defect model. They observed a high density of Schwann cells and axons. Recently, a human hair keratin hydrogel was used as nerve conduit luminal filler following a median nerve transection injury model in Macaca fascicularis primates.Citation89 The results obtained after 12 months by electrophysiology showed that the keratin-based scaffolds were able to support nerve regeneration.
Polysaccharides
Polysaccharides are a class of biopolymers constituted by mono- or disaccharides and have roles in membranes and in intracellular communication; they also have roles in storage (). Differences in the monosaccharide composition, chain organization, and molecular weight determine their physical properties including solubility, gelation, and surface properties. Heparin and other polysaccharides are structurally similar. They are highly biocompatible which makes them useful in use in tissue engineering applications.Citation91,Citation92 Some of the most commonly used saccharides in nerve regeneration constructs are given below.
Chitin and chitosan
Chitin, a component of the cytoskeleton in the outer skeleton of insects and in the exoskeleton of crustaceans, is the main source of chitosan, and after starch, it is the second most abundant polysaccharide on earth.Citation93 Chitosan is a linear polysaccharide composed of glucosamine and N-acetyl glucosamine units linked by β(1–4) glycosidic bonds (). Chitosan is the deacetylated form of chitin and is soluble in slightly acidic medium. Chitosan-based scaffolds are attractive for tissue engineering applications because of their ability to form interconnected porous structures (sponges), their cationic nature, and reasonable level of mechanical properties. Zheng and CuiCitation94 used chitosan conduits combined with bone marrow MSCs to promote peripheral nerve regeneration. They found that BMSCs could differentiate into neural stem cells (NSCs) in vivo in rats and they could bridge an 8 mm long gap upon differentiation.Citation95 Haastert-Talini et alCitation95 constructed chitosan tubes to regenerate 10 mm nerve defects in adult rats. The chitosan tubes could be made of chitosan with low, medium, or high degrees of deacetylation, and therefore, present different levels and rates of degradation and microenvironments for the regenerating nerve tissue. Chitosan tubes, however, suffered from certain limitations such as a high rate of degradation and low mechanical stability. In another study conducted with chitosan nanofibers for peripheral nerve repair, it was concluded that the nontoxic nature of the chitosan fibers is appealing for peripheral nerve regeneration applications.Citation96 Hsu et alCitation97 combined laminin-coated chitosan conduits and stem cells to bridge a 10 mm long gap in the sciatic nerve of Sprague Dawley rats. The rats implanted with the conduit carrying BMSCs showed the best results when judged by the extent of nerve regrowth, muscle mass of gastrocnemius, functional recovery, and tract tracing.
Hyaluronic acid
Hyaluronic acid is an immunoneutral polysaccharide that is ubiquitous in the human body, and it has been in clinical use for over 30 years ().Citation98 Hyaluronic acid can be processed into many physical forms such as viscoelastic solutions, hydrogels, electrospun fibers, non-woven meshes, macroporous and fibrillar sponges, flexible sheets, and nanoparticulates. Suri and SchmidtCitation99 produced hydrogel constructs of hyaluronic acid, collagen, and laminin for neural tissue engineering. Encapsulation of Schwann cells in 3D hydrogel constructs did not affect cell viability, and cells were viable for 2 weeks in all hydrogel samples. They also revealed that in the co-culture of dissociated neurons with Schwann cells, neurons were able to extend neurites, and some neurites were observed to follow Schwann cells. A study conducted on chitosan-gelatin porous scaffolds containing hyaluronic acid and heparan sulfate fabricated via lyophilization showed that presence of both chemicals in the scaffolds significantly promoted adhesion of NSCs and progenitor cells and supported growth in a 3D environment for long durations.Citation100
Synthetic nerve conduits
Biodegradable synthetic polymers are attractive alternatives to the natural origin biopolymers due to the following advantages: 1) some biodegradable synthetic polymers are biocompatible and they do not initiate any immunological responses, 2) their mechanical properties and degradation rates can be controlled by changing the process conditions and components without changing the bulk features of the polymer, and 3) they can be processed in various forms to enhance the tissue ingrowth.Citation101 Over time, the material choice for nerve conduits shifted towards the use of more biocompatible synthetic polymers. Biodegradable polyesters, such as poly(lactic acid) (PLA), poly(glycolic acid) (PGA), poly(lactic acid-co-glycolic acid) (PLGA), poly(ε-caprolactone) (PCL), polyurethanes (PUs), tri-methylene carbonate-co-ε-caprolactone, poly(D,L-lactide-co-ε-caprolactone), and nonbiodegradable polymers such as methacrylate-based hydrogels, polystyrene, silicone, and poly(tetrafluoroethylene), were used as nerve conduit materials. In , the structure of some synthetic polymers are presented. Various fabrication techniques such as electrospinning, injection molding, photolithography, and extrusion were used in the processing of these materials.
Polyesters
Polyesters have an ester functional group in their main backbone. PLA, poly(L-lactic acid) (PLLA), PGA, PLGA, and PCL are polyesters most commonly used in the fabrication of nerve conduits.
PLA
PLA can be made from lactic acid obtained from corn, sugar beet, or wheat, and is biocompatible (). PLA was used as a nerve conduit material in a number of studies. In one such study, a multilayer PLA nerve conduit was fabricated by microbraiding to obtain adequate mechanical strength at the injury site.Citation102 In the applications on rats, successful regeneration through the gap was observed at 8 weeks after operation. In another study, a PLA nerve conduit was made by immersion precipitation to bridge a 20 mm long gap in a rabbit sciatic nerve transection model.Citation103 These conduits had macropores on the outside, and interconnected micropores in the inner layer to provide a higher outflow rate than inflow rate. It was reported that the functional recovery after 18 months was about 80%, based on electrophysiology and behavior analysis. A nonwoven fabric nerve conduit was made by a melt-blow process, in which the fabric was wrapped around a stainless steel core bar and heated at 90°C for 20 minutes.Citation104 In this study, nerve conduits made of a PLA non-woven fabric, a silicone tube filled with type I collagen gel, and an autologous nerve were implanted in the buccal branch of a 7 mm facial nerve defect, and their nerve regeneration performances were evaluated 13 weeks after the surgery. The number of myelinated neural fibers in the middle portion of the regenerated nerve and the axon diameter were the highest for PLA tubes. It was concluded that PLA non-woven fabric tube, composed of randomly connected PLA fibers, provided better nerve regeneration compared with a silicone control. In a different study, alignment in a nerve conduit was provided with microporous-micropatterned PLA by photolithography.Citation105 PLA conduits grafted with chitosan–nano Au and the fibroblast growth factor 1 (FGF1) after plasma activation showed the greatest regeneration capacity and functional recovery when they were tested for their ability to bridge a 15 mm critical gap defect in a rat sciatic nerve injury model. Addition of NSCs on the micropatterned surfaces further enhanced efficacy of the conduits compared with autografts.
PLLA
PLLA is a stereoregular and highly crystalline form of PLA. It is also widely used in tissue engineering applications. In a study, a PLLA nerve conduit was fabricated by extrusion and was used in a 10 mm sciatic nerve defect model in rats.Citation106 The PLLA conduits were highly porous with an interconnected pore structure (83.5% porosity and 12.1 μm mean pore size). The nerve fiber density in the distal sciatic nerve for the PLLA conduits was similar to that for the control isografts at 16 weeks. Increased axon number and nerve fiber density was found in the PLLA mid-conduit compared with control isograft at 16 weeks. In more recent years, importance of the alignment in nerve guide conduits was understood, and the designers of the conduits started to take this into account. In a later study, a porous, micropatterned poly(D,L-lactic acid) (PDLLA) conduit was seeded with Schwann cells to provide additional trophic and physical support in a 10 mm rat sciatic nerve defect.Citation107 The results showed that time of recovery and the sciatic function indices were significantly enhanced when the Schwann cell–seeded conduits were used, and it was concluded that biodegradable, micropatterned conduits seeded with Schwann cells which provide a combination of physical, chemical, and biological guidance cues for regenerating axons at the cellular level offered a better alternative in the repair of sciatic nerve compared with conventional biodegradable conduits. Li et alCitation108 prepared multi-walled PLLA conduits using solvent casting, physical imprinting, and rolling-fusing methods to obtain multiple intraluminal walls and precise topography along the longitudinal axis to provide the alignment along the conduits. It was demonstrated that when cultured on these patterned films, PC12 cells and chick sympathetic neurites aligned predominantly in the direction of the underlying grooves.
In recent years, besides photolithographic techniques, electrospinning has become a powerful technique to create aligned structures needed to improve the growth of the neurons within the conduits. To illustrate, PLLA was combined with polycaprolactone and NGF to promote neurite outgrowth using core–shell structured biodegradable nanofibers fabricated by coaxial electrospinning.Citation109 Here, poly(L-lactide-co-ε-caprolactone) (P(LLA-CL)) was used for the shell, and bovine serum albumin (BSA) or BSA/NGF for the core, and implanted at a 10 mm long rat sciatic nerve defect. The functional and histological observations revealed that the number and arrangement of regenerated nerve fibers, myelination, and restoration of nerve function in the P(LLA-CL)/NGF conduit group and the nerve autograft group were similar, but they were significantly greater than the empty P(LLA-CL) or the NGF-injected P(LLA-CL) conduit groups. It was, therefore, concluded that incorporation of NGF in the nerve conduit had a positive contribution on both myelination and functional recovery of the nerves. In addition to the contribution of alignment in the conduits, conductivity is another parameter in obtaining physiologically active, properly healed nerves. In a recent study, PDLLA was blended with polypyrrole (PPy) to produce a conducting nerve conduit.Citation110 Increasing degrees of conductivity (5.65, 10.40, and 15.56 mS/cm) were obtained with increasing PPy content (5%, 10%, and 15%, respectively). It was found that there was a significant increase in both the percentage of neurite bearing cells and the median neurite length as the PPy content was increased when PC12 cells, seeded on these conduits, were stimulated with 100 mV for 2 hours. It was reported that the PPy/PDLLA nerve conduit performed similarly to the “gold standard” autologous nerve graft in the repair of a 10 mm rat sciatic nerve defect.
PGA
PGA is another biodegradable, rigid, thermoplastic, and highly crystalline polyester which exhibits a high tensile modulus with very low solubility in organic solvents ().Citation111 In an earlier study, PGA-based crimped tube device (Neurotube®; Synovis Micro Companies Alliance, Birmingham, AL, USA) was described for the repair of peripheral nerve injuries.Citation112 It is the first synthetic, highly porous, bioresorbable nerve guide conduit to receive approval by the FDA (1999). In a more recent study, bone marrow stem cells (BMSCs) were combined with PGA tube (PGAt) (Neurotube®) in autografted rat facial nerves.Citation113 After cutting of the mandibular branch of the rat facial nerve, surgical repair consisted of autologous graft in a PGAt filled with basement membrane matrix with undifferentiated BMSCs or Schwann-like cells that had differentiated from BMSCs. After 6 weeks of surgery, animals from either cell-containing group had compound muscle action potential amplitudes significantly higher than the control groups. Facial nerves with Schwann-like cell implants had axonal densities within the range of reference values, and had the highest axonal diameter in distal segments. It was concluded that regeneration of the facial nerve was improved by BMSCs within PGAt in rats; however, Schwann-like cells yielded superior effects. PGA is also commonly combined with natural polymers such as collagen (will be explained in the “Hybrid nerve conduits” section).
PLGA
PLGA is a copolyester that has been used extensively as a nerve guide material due to its ease of fabrication, approval by the FDA, and low inflammatory response it created. In an earlier study, porous, PLGA conduits with longitudinally aligned channels were produced by using a combined injection molding and thermally induced phase transition technique.Citation114 In this approach, PLGA, dissolved in acetic acid, was injected into a cold mold which induced solidification of the polymer solution and led to solid–liquid phase separation. The foam obtained had a macrostructure with high anisotropy due to the removal of acetic acid by sublimation. Macropores were organized into bundles of channels up to 20 μm wide in the PLGA matrix, which then was used as a nerve conduit. In another study, NGF was incorporated into the PLGA nerve conduits (single or multiple lumens) which were fabricated from a mixture of PLGA microspheres and porogen (NaCl) that was loaded into a mold and processed by gas foaming.Citation115 After 13 days of implantation, the conduits were observed to have sufficient mechanical properties, which could be controlled by porosity. Porosity was needed to create open channels that allow tissue ingrowth. It was concluded that PLGA conduits with controllable lumen diameters and protein release might enhance nerve regeneration by guiding and stimulating neurite outgrowth. Wen and TrescoCitation116 fabricated PLGA hollow fiber membranes (HFMs) using a wet phase inversion technique to create nerve tract guidance channels. HFMs with different size, inner and outer surface morphologies, porosity, and permeability were produced and under simulated in vitro physiological conditions, PLGA HFMs exhibited a degradation profile to accommodate nervous system regeneration and axonal outgrowth. In another study, PLGA was combined with pluronic F127, which is a nonionic, surfactant polyol.Citation117 Asymmetrically porous PLGA-F127 tubes were produced to serve as nerve guide conduit using a modified immersion-precipitation method. By making the scaffold asymmetric, the inner surface of the tube with nanosize pores (~50 nm) could effectively prevent fibrous tissue infiltration but allow permeation of nutrients and retain neurotrophic factors. Meanwhile, the outer surface had microsize pores (~50 μm) that could allow vascular ingrowth to supply nutrients inside the tube. It was reported that after implantation into a 10 mm left sciatic nerve defect in rats, immunohistochemical and electrophysiological evaluations showed that PLGA-F127 tubes were better in nerve regeneration than the control silicone or hydrophobic PLGA tubes. In another study, PPy-coated, electrically conductive, electrospun PLGA nanofibers were fabricated to investigate the combined effect of nanofiber structures and electrical stimulation.Citation118 PPy-PLGA electrospun meshes enhanced the growth and differentiation of rat PC12 cells and hippocam-pal neurons. Electrical stimulation showed that PC12 cells stimulated on PPy-PLGA scaffolds exhibited 40%–50% longer neurites and 40%–90% more neurite formation compared with unstimulated cells on the same scaffolds. In addition, stimulation of the cells on aligned PPy-PLGA fibers resulted in longer neurites and more neurite-bearing cells than stimulation on random PPy-PLGA fibers. As expected, combined effect of electrical stimulation and topographical guidance provided better nerve guide conduits. In another study, PLGA nerve conduits, made of cylindrically woven PLGA filaments, were treated with pulsed oxygen plasma and coated with ciliary neurotrophic factor (CNTF) and chitosan, and were used in the repair of 25 mm long canine tibial nerve defects in crossbred dogs.Citation119 After 3 months, histological results showed that PLGA/chitosan-CNTF conduits were better than the PLGA/chitosan conduits, and were similar to autologous nerve grafts. In a recent study, nerve tubes of PLGA, poly(caprolactone-fumarate) (PCLF), a neutral oligo[(polyethylene glycol) fumarate] hydrogel and a positively charged oligo[(polyethylene glycol)fumarate] hydrogel with a PCLF sleeve, were implanted in a rat sciatic nerve model.Citation120 The PCLF tube significantly improved nerve regeneration and recovery compared with the other conduits.
PCL
PCL another polyester, has high solubility in organic solvents, low melting temperature (55°C–60°C) and glass transition temperatures (−60°C) (). When its in vivo performance was compared with those of PHB conduits, PCL conduits were better in bridging up a 10 mm gap in rat sciatic nerve in 2 weeks.Citation121 Oliveira et alCitation122 incorporated MSCs into a PCL nerve conduit to improve median nerve regeneration after transection. MSC-treated animals showed significantly larger numbers of myelinated and unmyelinated nerve fibers and blood vessels compared with DMEM (Dulbecco’s Modified Eagle’s medium)-treated animals. This system also prevented decrease of creatine phosphokinase levels, which might be an indicator of tissue activity in muscle, and improved functional recovery in mice. In one study, PCLF was blended with a conductive polymer, PPy, and electrical stimulation was applied.Citation123 In vitro studies with PC12 cells showed that there was a significant increase in the percentage of neurite bearing cells, number of neurites per cell, and neurite length when electrical stimulation was applied. Extending neurites were observed to align in the direction of the current applied. In another study, controlled release of vascular endothelial growth factor (VEGF) from PCLF nerve conduits was studied.Citation124 VEGF was added into PLGA microspheres by double emulsion solvent evaporation technique, placed in the PCLF guide, and robust angiogenesis and nerve regeneration were observed. Another group filled biodegradable PCL tubes with MSCs and used these tubes in the treatment of the left sciatic nerve of mice.Citation125 There was a significant increase in the number of myelinated fibers and the number of neurons in the dorsal root ganglion for the PCL conduits with MSCs. Higher values of trophic factor expression, especially that of NGF, and improvement in the sciatic functional index were observed in the MSC-treated groups. Moreover, there was an increase in the weight of the gastrocnemius muscle and creatine phosphokinase enzyme, suggesting an improvement of reinnervation and activity in animals that received MSCs.
Effect of the type of surface topography and mechanical properties on cell morphology and alignment were investigated by using smooth, pitted, and grooved structures of ultrathin PCL-PLA solvent cast films.Citation126 The PCL-PLA conduits were made by rolling the films around a mandrel and using a thermal welding technique to join the edges. It was concluded that sloped channels and their angles were the most important parameters in guiding nerve cell arrangement. Besides, the grooved structure reduced the mechanical strength of PCL-PLA films, so the mechanical properties became closer to those of the healthy nerve.
Dual stimulation, with NGF acting as a biological stimulus and low intensity pulse ultrasound (US) as a physical stimulus, was applied to enhance nerve regeneration within asymmetrically porous PCL-pluronic F127 conduits.Citation127 These conduits were implanted into a 10 mm left sciatic nerve defect in rats. After 12 and 24 weeks of implantation, it was reported that each stimulation (NGF or US) had a positive effect in promoting the peripheral nerve regeneration through the nerve guide conduit. However, the US-stimulated nerve guide conduit group achieved faster nerve regeneration compared with the NGF-stimulated group. Dual stimulation (NGF and US) was more effective on nerve regeneration than the single stimulation.
Poly(D,L-lactide-co-ε-caprolactone)
Poly(D,L-lactide-co-ε-caprolactone) is a copolymer of lactic acid and caprolactone monomers. In a study, cylindrical poly(D,L-lactide-co-ε-caprolactone) 80/20 copolymer nerve conduits were fabricated by using an ink-jet system.Citation128 In Radulescu et al’s previous study, it was found that hNGF-EcR-293 cells could be genetically modified to deliver NGF in vitro and in vivo to support the local neuroreparative factor delivery via a tightly controlled system.Citation129 Their findings demonstrated that these cells could attach and survive for more than 8 weeks when cultured on the 80/20 PLA-PCL copolymer but failed to attach and died on 25/75 and 40/60 PLA-PCL copolymer used. The level of bioactive NGF was higher compared with control on 80/20 PLA-PCL scaffolds.
In another study, Neurolac™, a commercial poly(D,L-lactide-co-ε-caprolactone) nerve guide, was used on patients.Citation130 Neurolac™ was tested on 28 nerve lesions on various sites: arm, elbow, forearm, wrist, palm, and fingers. Defects were about 11 mm long. After an average of 21.9 months follow-up (3–45 months), subjective criteria (pain and cold intolerance) and objective criteria (strength) were compared with the contralateral side. Average pain score was 2.17/10, and cold intolerance was reported in 15 cases. Grip strength averaged 64.62% of the contralateral side. Eight complications were observed, the most serious being two fistulizations of the Neurolac™ device close to a joint and one neuroma. It was concluded that use of Neurolac™ in repairing hand nerve defects was not very effective.
PUs
PU is a polymer with a backbone containing urethane linkages and is used in the fabrication of many biomedical devices including the nerve guides (). PU conduits prepared by uniform coating on a rotating mandrel were implanted for the repair of a 12 mm femoral nerve-gap model in rabbits, and myelinated axon regeneration was observed after 4 weeks of implantation.Citation131 In a more recent study, nerve guides were prepared from block PUs (abbreviated as PUCL-ran-EG) which were based on poly(3-caprolactone) (PCL-diol) and poly(ethylene glycol), without any additional compounds like growth factors or proteins, by using particle leaching method.Citation132 They had pore sizes in the range 1–5 μm and porosity 88%. Four types of guides, PUCL-ran-EG, autograft, PCL, and silicone tubes, were compared in the rat model. Bridging of a 10 mm defect gap by the regenerated nerve was observed in all rats after 14 weeks. Results revealed that polyurethane nerve guides exhibited much better regeneration behavior than the others and were comparable to the autograft.
Polyols
Polyvinyl alcohol (PVA) is a water soluble, nondegradable, synthetic polymer and was used as a nerve conduit material in addition to its many other uses in the biomedical field (). Currently there is a commercially available nonresorbable PVA hydrogel (SaluBridge; SaluTunnel; SaluMedica LCC, Atlanta, GA, USA). In a study, a tubular PVA conduit was produced by using a single screw extruder, and preseeded with Schwann cells.Citation133 Porosity, wall thickness, and the Schwann cell seeding density were tested for their effect on axon growth using rat dorsal root ganglia. It was found that high wall thickness and low porosity have a detrimental effect on the growth of the axons. Maximum growth rate was observed at a porosity of 75% for Schwann cell–seeded conduits, but not with unseeded ones. In more recent studies,Citation134,Citation135 PVA was combined with chitosan. (This will be further explained in the “Polysaccharides with synthetic biomaterials” section).
Hybrid nerve conduits
The surface characteristics and charge densities of the nerve conduits have an important role in cell adhesion.Citation136,Citation137 Most of the synthetic materials used for this purpose are hydrophobic and they are not very suitable for cell adhesion. As a result, researchers started to coat the guide surfaces with ECM proteins or design hybrid nerve conduits with different architectures to overcome this problem.Citation138–Citation143 In recent years, use of such hybrids in nerve guide production has increased due to the improved properties achieved as a result of using a combination of natural and synthetic polymers. Some of those studies are summarized below.
Polyesters with synthetic biomaterials
Biological polyesters like PHBV can be combined with synthetic polymers. Yucel et alCitation144 constructed a biodegradable, tubular nerve conduit by wrapping a porous micropatterned film (PHBV–P(L-D,L)LA–PLGA) around aligned electrospun fibers (PHBV–PLGA). It was revealed that this two-component nerve conduit would have a good potential to serve as a nerve guide with its porosity and mechanical properties. The polymer blends were chosen so that the protective tube cover, film part, would erode slower than the fibrous mat to achieve complete healing before the tube eroded. Characterization with scanning electron microscopy showed that the fibers were aligned parallel to the groove axis of the micropatterned films, making the conduit desirable for peripheral nerve regeneration applications with its oriented architecture. In further studies, Yucel et alCitation145 constructed a guided tissue engineered nerve tube which consisted of aligned, electrospun fibers (PHBV–PLGA) seeded with NSCs wrapped in a porous, micropatterned film (PHBV– P(L-D,L)LA–PLGA) with supportive cells aligned along the microgrooves to support the NSCs. The investigations of cell behavior on the scaffolds showed that both cells, undifferentiated NSCs, and supportive cells were oriented along the guiding and support elements, the microgrooves, and the aligned fibers. In addition, in vitro studies showed that the cells were able to survive and maintained their alignment in the 3D tissue engineered nerve tube.
Polysaccharides with synthetic biomaterials
Polysaccharides like chitosan are widely combined with synthetic polymers to prepare neural conduits. Cooper et alCitation146 fabricated parallel chitosan–PCL fiber bundles or meshes for nerve tissue regeneration and investigated how the fiber orientation influenced nerve cell organization and function in comparison with randomly oriented fibrous scaffolds or cast films of the same material. Schwann cells grown on the parallel chitosan–PCL fibers exhibited a bipolar morphology that aligned with the fiber direction. The chitosan-PCL material supported neuron-like PC12 cell adhesion, and the parallel fibers regulated the growth of PC12 cells along the fiber orientation. In another study, chitosan-PVA nanofibers produced by electrospinning were combined with single-walled carbon nanotube (SWCNT), and results have shown that SWCNTs as reinforcing phase can augment the morphology, porosity, and structural properties of chitosan-PVA nanofiber composites and thus benefit the proliferation rate of both human brain-derived cells and astocytoma cells (U373).Citation134 Alhosseini et alCitation135 produced electrospun PVA-chitosan nanofibrous scaffolds with large pore sizes for nervous tissue repair. The chitosan-containing scaffolds were used for in vitro cell culture in contact with PC12 nerve cells, and it was found that addition of chitosan to the PVA scaffolds enhances viability and proliferation of nerve cells.
Proteins with synthetic biomaterials
Proteins are natural polymers, and therefore, their blends with synthetic polymers could be considered as hybrid structures. The most commonly used protein in hybrid preparation is collagen. In a study, a PGA fibrous mesh was coated with collagen, filled with neurotrophic factors, and implanted across a 25 mm long left sciatic nerve gap in cats.Citation147 Regeneration of both myelinated and unmyelinated axons was confirmed by electron microscopy after 5 months of surgery. After 4–16 months of implantation, histology revealed the regeneration of well vascularized nerve tissue. Electrophysiological examinations demonstrated restoration of evoked electromyograms and sensory-evoked potentials, which were recorded from the cerebral cortex as well as from the spinal cord. In another study, PGA-collagen tubes filled with laminin-coated collagen fibers were implanted into an 80 mm gap in the left peroneal nerve of dogs.Citation148 The outer tube was made of cylindrically woven PGA mesh; its outer and inner surfaces were coated with amorphous collagen coated with laminin. Histological observations showed that numerous unmyelinated and myelinated nerve fibers regrew through and beyond the gap after 12 months of implantation. Compound muscle action potentials, motor-evoked potentials, and somatosensory-evoked potentials gave peak amplitudes, and latencies were recovered gradually after 3 months of implantation, indicating the functional establishment of the nerve connection with the target organs. This sample was later compared with another guide, PGA-collagen tubes filled with laminin-soaked collagen spongesCitation149 by implanting into 80 mm gaps of canine peroneal nerve defects. After 12 months, both morphometrically and electrophysiologically effective nerve regeneration was observed in the sponge group, although these differences were statistically insignificant. In another study, a PGA-collagen tube was implanted across a 15 mm gap in the left peroneal nerves of 24 beagle dogs.Citation150 As a control, the right peroneal nerve was reconstructed with the autograft harvested from the left side. PGA tube was prepared with a tubular braiding machine, and it was coated with collagen layers. Results showed that the myelinated axons on the PGA side were larger in diameter than those on the autograft side. This PGA-collagen nerve conduit led to superior functional recovery in comparison to the autograft.
Future perspectives and conclusion remarks
As can be seen in this review, the nerve guide field is developing in a variety of directions such as the choice of different types of nerve and support cells (Schwann cells), use of fibers or channels for guidance, use of bioactive agents (mainly growth factors) to enhance the response of the seeded cells or the cells in the vicinity of the defect site, and the choice of polymers to be used as the guide material. The advances made are significant, as shown by the number of commercially available nerve guides. summarizes the types and the performance of a variety of conduit materials. The limitation appears to be in the length of the defect that we can treat (which is not longer than 2 cm) with our sophisticated materials and designs. The target should now be to increase the length of the defect that we can treat through the use of the current knowledge that has been accumulated mainly over the last decade.
Table 2 Conduit materials used in the regeneration of the peripheral nerves and their success rates
Disclosure
The authors report no conflicts of interest in this work.
References
- DalyWYaoLZeugolisDA biomaterials approach to peripheral regeneration: bridging the peripheral nerve gap and enhancing functional recoveryJ R Soc Interface2012920222122090283
- SeddonHThree types of nerve injuryBrain194366237288
- SunderlandSA classification of peripheral nerve injuries producing loss of functionBrain19517449151614895767
- CampbellWWEvaluation and management of peripheral nerve injuryClin Neurophysıol20081191951196518482862
- DeumensRBozkurtAMeekMFRepairing injured peripheral nerves: bridging the gapProg Neurobıol20109224527620950667
- MillessiHTechniques for nerve graftingHand Clin200016739110696578
- KehoeSZhangXFBoydDFDA approved guidance conduits and wraps for peripheral nerve injury: a review of materials and efficacyInjury20124355357221269624
- SiemionowMBrzezickiGChapter 8: current, techniques and concepts in peripheral nerve repairStefanoGPierluigiTBrunoBInternational Review of NeurobiologySan Diego, CAAcademic Press2009141172
- NicholsCMBrennerMJFoxIKEffects of motor versus sensory nerve grafts on peripheral nerve regenerationExp Neurol200419034735515530874
- SiemionowMSonmezENerve Allograft transplantation: a reviewJ Reconstr Microsurg200723851152018189213
- HebebrandDZohmanGJonesNFNerve xenograft transplantation: immunosuppression with FK-506 and RS-61443Biomaterials1997223304307
- JiaHJWangYTongXJSciatic nerve repair by acellular nerve xenografts ımplanted with BMSCs in rats xenograft combined with BMSCsSynapse20126625626922127791
- MackinnonSHudsonAFalkRBilbaoJKlineDHunterDNerve allograft response: a quantitative immunological studyNeurosurgery19821061697035993
- MackinnonSEDoolabhVBNovakCBTrulockEPClinical outcome following nerve allograft transplantationPlast Reconstr Surg20011071419142911335811
- HudsonTWLiuSYSchmidtCEEngineering an improved acellular nerve graft via optimized chemical processingTissue Eng2004101346135815588395
- GilbertTWSellaroTLBadylakSFDecellularization of tissues and organsBiomaterials200627193675368316519932
- GulatiAKEvaluation of acellular and cellular nerve grafts in repair of rat peripheral nerveJ Neurosurg1988681171233335896
- FreytesDOBadylakSFWebsterTJBiaxial strength of multilaminated extracellular matrix scaffoldsBiomaterials2004252353236114741600
- LinPChanWCBadylakSFAssessing porcine liver-derived biomatrix for hepatic tissue engineeringTissue Eng2004101046105315363162
- YooJJMengJOberpenningFBladder augmentation using allogenic bladder submucosa seeded with cellsUrology1998512212259495701
- De FilippoREYooJJAtalaAUrethral replacement using cell seeded tubularized collagen matricesJ Urol20021681789179212352360
- DahlSLKohJPrabhakarVDecellularized native and engineered arterial scaffolds for transplantationCell Transplant20031265966614579934
- ChenRNHoHOTsaiYTProcess development of an acellular dermal matrix (ADM) for biomedical applicationsBiomaterials200425132679268614751754
- WoodsTGratzerPFEffectiveness of three extraction techniques in the development of a decellularized bone–anterior cruciate ligament–bone graftBiomaterials2005267339734916023194
- VyavahareNHirschDLernerEPrevention of bioprosthetic heart valve calcification by ethanol preincubation. Efficacy and mechanismsCirculation1997954794889008467
- GoissisGSuziganSParreiraDRPreparation and characterization of collagen–elastin matrices from blood vessels intended as small diameter vascular graftsArtif Organs20002421722310759645
- CourtmanDWPereiraCAKashefVDevelopment of a pericardial acellular matrix biomaterial: biochemical and mechanical effects of cell extractionJ Biomed Mater Res1994286556668071376
- RiederEKasimirMTSilberhumerGDecellularization protocols of porcine heart valves differ importantly in efficiency of cell removal and susceptibility of the matrix to recellularization with human vascular cellsJ Thorac Cardiovasc Surg200412739940514762347
- GambaPGConconiMTLo PiccoloRExperimental abdominal wall defect repaired with acellular matrixPediatr Surg Int20021832733112415348
- ClarkJNOgleMFAshworthPPrevention of calcification of bioprosthetic heart valve cusp and aortic wall with ethanol and aluminium chlorideAnn Thorac Surg200579389790415734402
- FrerichsOFansaHSchichtCWolfGSchneiderWKeilhoffGReconstruction of peripheral nerves using acellular nerve grafts with implanted cultured Schwann cellsMicrosurgery200222731131512404350
- KrekoskiCANeubauerDGrahamJBMetalloproteinase-dependent predegeneration in vitro enhances axonal regeneration within acellular peripheral nerve graftsJ Neurosci20022223104081041512451140
- KimBSYooJJAtalaAPeripheral nerve regeneration using acellular nerve graftsJ Biomed Mater Res A200468220120914704961
- NeubauerDGrahamJBMuirDChondroitinase treatment increases the effective length of acellular nerve graftsExp Neurol200720716317017669401
- WhitlockELTuffahaSHLucianoJPProcessed allografts and type I collagen conduits for repair of peripheral nerve gapsMuscle Nerve20093978779919291791
- SunFZhouKMiWJCombined use of decellularized allogeneic artery conduits with autologous transdifferentiated adipose-derived stem cells for facial nerve regeneration in ratsBiomaterials201132328118812821816463
- ZhaoZWnagYPengJImprovement in nerve regeneration through a decellularized nerve graft by supplementation with bone marrow stromal cells in fibrinCell Transplant20142319711023128095
- GluckTUeber Transplantation, Regeneration und entzündliche NeubildungKlin Wochenschr188118554557
- LundborgGA 25-year perspective of peripheral nerve surgery: evolving neuroscientific concepts and clinical significanceJ Hand Surg200025391414
- VerreckGChunILiYPreparation and physico-chemical characterization of biodegradable nerve guides containing the nerve growth agent sabeluzoleBiomaterials2005261307131515475061
- FreedLEVunjak-NovakovicGBironRJBiodegradable polymer scaffolds for tissue engineeringNat Biotechnol199412689693
- SchmidtCELeachJBNeural tissue engineering: strategies for repair and regenerationAnnu Rev Biomed Eng2003529334714527315
- HrabakOIndustrial production of poly-3-hydroxybutyrateFEMS Microbiol Rev1992103251256
- WilliamsSFMartinDPHorowitzDMPeoplesOPPHA applications: addressing the price performance issue: I. Tissue engineeringInt J Biol Macromol19992511112110416657
- HazariAJohansson-RudénGJunemo-BostromKA new resorbable wrap-around implant as an alternative nerve repair techniqueJ Hand Surg Br19992429129510433438
- AbergMLjungbergCEdinEClinical evaluation of a resorbable wrap-around implant as an alternative to nerve repair: a prospective, assessor-blinded, randomised clinical study of sensory, motor and functional recovery after peripheral nerve repairJ Plast Reconstr Aesthet Surg2009621503150918938119
- TerenghiGMosahebiAThe interface between peripheral axons, Schwann cells and biosynthetic nerve guidesAldskogiusHFraherJGlial Interfaces in the Nervous System: Role in Repair and PlasticityAmsterdamIOS Press20021320
- ArmstrongSJWibergMTerenghiGECM molecules mediate both Schwann cell proliferation and activation to enhance neurite outgrowthTissue Eng200713122863287017727337
- MohannaPNYoungRCWibergMA composite poly-hydroxybutyrate–glial growth factor conduit for long nerve gap repairsJ Anat200320355356514686691
- ErbaPMantovaniCKalbermattenDFRegeneration potential and survival of transplanted undifferentiated adipose tissue-derived stem cells in peripheral nerve conduitsJ Plast Reconstr Aes20106312e811e817
- MasaeliEMorshedMNasr-EsfahaniMHFabrication, characterization and cellular compatibility of poly(hydroxy alkanoate) composite nanofibrous scaffolds for nerve tissue engineeringPloS One201382e5715723468923
- BiazarEHeidariKHA nanofibrous phbv tube with Schwann cell as artificial nerve graft contributing to rat sciatic nerve regeneration across a 30-mm defect bridgeCell Commun Adhes2013201–2414923461795
- BiazarEKeshelSHChitosan–cross-linked nanofibrous PHBV nerve guide for rat sciatic nerve regeneration across a defect bridgeASAIO J201356665165924172271
- PrabhakaranMPAtankhanERamakrishnaSElectrospun aligned PHBV/collagen nanofibers as substrates for nerve tissue engineeringBiotechnol Bioeng2013110102775278423613155
- KadlerKEHolmesDETrotterJACollagen fibril formationBiochem J19963161118645190
- EvansDGBaserMEMcGaughranJSharifSHowardEMoranAMalignant peripheral nerve sheath tumours in neurofibromatosis 1J Med Genet20023931131412011145
- BrownRAPhillipsJBCell responses to biomimetic protein scaffolds used in tissue repair and engineeringInt Rev Cytol20072627515017631187
- BrownRAWisemanMChuoC-BCheemaUNazhatSNUltrarapid engineering of biomimetic materials and tissues: fabrication of nano- and microstructures by plastic compressionAdv Funct Mater20051517621770
- ArchibaldSKrarupCShefnerJA collagen-based nerve guide conduit for peripheral nerve repair: an electrophysiological study of nerve regeneration in rodents and nonhuman primatesJ Comp Neurol19913066856962071700
- MackinnonSEHudsonARBojanowskiVHunterDAMaraghiEPeripheral-nerve injection injury with purified bovine collagen – an experimental-model in the ratAnn Plast Surg1985144284364083699
- MeekMFCoertJHUS Food and Drug Administration/Conformit Europe–approved absorbable nerve conduits for clinical repair of peripheral and cranial nervesAnn Plast Surg20086046647218437784
- KramerBAKaderAgKlarkRNUse of the Neuro-Wrap system for severe post-electroconvulsive therapy headachesJ ECT200824215215518580561
- LiSTYuenDinventorsCollagen Matrix, Inc., assigneeImplant devices for nerve repair United States patent US6716225 B2462004
- LiSTArchibaldSJKrarupCMadisonRDPeripheral nerve repair with collagen conduitsClin Mater1992919520010149970
- LohmeyerJASiemersFMachensHGThe clinical use of artificial nerve conduits for digital nerve repair: a prospective cohort study and literature reviewJ Reconstr Mıcrosurg200925556119037847
- MadduriSFeldmanKTervoortTCollagen nerve conduits releasing the neurotrophic factors GDNF and NGFJ Controlled Release2010143168174
- YangCRChenJDPreparation and biological evaluation of chitosan–collagen–icariin composite scaffolds for neuronal regenerationNeurol Sci20133494194722833221
- SalvatoreLMadaghieleMParisiCGattiFSanninoACrosslinking of micropatterned collagen based nerve guides to modulate the expected half lifeJ Biomed Mater Res A Epub2142014
- LiuBSYaoCHHsuSHA novel use of genipin-fixed gelatin as extracellular matrix for peripheral nerve regenerationJ Biomater Appl200419213415245641
- CenniECiapettiGSteaSBiocompatibility and performance in vitro of a hemostatic gelatin spongeJ Biomater Sci Polym Ed20001168569911011767
- GamezEGotoYNagataKIwakiTSasakiTMatsudaPhotofabricated gelatin-based nerve conduits: nerve tissue regeneration potentialCell Transplant200413554956415565867
- ChenYSChangJYChenCYAn in vivo evaluation of a biodegradable genipin-cross-linked gelatin peripheral nerve guide conduit materialBiomaterials2005263911391815626438
- LiuBSFabrication and evaluation of a biodegradable proanthocyanidin-crosslinked gelatin conduit in peripheral nerve repairJ Biomed Mater Res A2008158741092110218428983
- NieXDengMYangMLiuLZhangYWenXAxonal regeneration and remyelination evaluation of chitosan/gelatin based nerve guide combined with transforming growth factor-β1 and Schwann cellsCell Biochen Biophys201468163172
- WhitworthIHTerenghiGGreenCJTargeted delivery of nerve growth factor via fibronectin conduits assists nerve regeneration in control and diabetic ratsEur J Neurosci19957222022258563971
- WhitworthIHBrownRADoreCJNerve growth factor enhances nerve regeneration through fibronectin graftsJ Hand Surg199621B514522
- AhmedZBrownRAAdhesion, alignment and migration of cultured Schwann cells on ulrathin fibronectin fibresCell Motıl Cytoskel199942331343
- PhillipsJBBuntingSCJWardZHallSMBrownRAFibronectin tubes as tissue engineering devices for peripheral nerve repair. Molecular, Cellular and Tissue Engineering, 2002Proceedings of the IEEE-EMBS Special Topic Conference
- MosahebiAWibergMTerenghiGAddition of fibronectin to alginate matrix improves peripheral nerve regeneration in tissue-engineered conduitsTissue Eng20099220921812740084
- DingTLuWWZhengYZyLiHbPanLuoZRapid repair of rat sciatic nerve injury using a nanosilver-embedded collagen scaffold coated with laminin and fibronectinRegen Med20116443744721749202
- KaplanDLMelloSMArcidiaconoSMcGrathKKDProtein Based MaterialsBostonBirkhauser1998103131
- BiniEKnightDPKaplanDLMapping domain structures in silks from insects and spiders related to protein assemblyJ Mol Biol20043351274014659737
- YangYChenXDingFBiocompatibility evaluation of silk fibroin with peripheral nerve tissues and cells in vitroBiomaterials2007281643165217188747
- HuangWBegumRBarberTRegenerative potential of silk conduits in repair of peripheral nerve injury in adult ratsBiomaterials201233597122005069
- GuYZhuJXueCChitosan/silk fibroin-based, Schwann cell-derived extracellular matrix-modified scaffolds for bridging rat sciatic nerve gapsBiomaterials20143572253226324360577
- SchachtKScheibelTProcessing of recombinant spider silk proteins into tailor-made materials for biomaterials applicayionsCurr Opin Biotech201429C626924657706
- CrewtherWGFraserRDBLennoxFGLindleyHThe chemistry of keratinsAnfinsenCBJrAnsonMLEdsallJTRichardsFMAdvances in Protein ChemistryNew YorkAcademic Press1965191346
- LinYCRamadanMVan DykeMKeratin gel filler for peripheral nerve repair in a rodent sciatic nerve injury modelPlast Reconstr Surg20121291677822186500
- PaceLAPlateJFMannavaSA human hair keratin hydrogel scaffold enhances median nerve regeneration in nonhuman primates: an electrophysiological and histological studyTissue Eng Part A2014203–450751724083825
- SierpinskiPGarrettJMaJThe use of keratin biomaterials derived from human hair for the promotion of rapid regeneration of peripheral nervesBiomaterials200829111812817919720
- NishinariKTakahashiRInteraction in polysaccharide solutions and gelsCurr Opin Colloid Interface Sci20038396400
- YuLDeanKLiLPolymer blends and composites from renewable resourcesProg Polym Sci200631576602
- EugeneKLeeYLImplantable applications of chitin and chitosanBiomaterials2003242339234912699672
- ZhengLCuiHFUse of chitosan conduit combined with bone marrow mesenchymal stem cells for promoting peripheral nerve regenerationJ Mater Sci Mater Med20102151713172020101439
- Haastert-TaliniKGeunaSDahlinLBChitosan tubes of varying degrees of acetylation for bridging peripheral nerve defectsBiomaterials201334389886990424050875
- CarvalhoCRCorreiaMAOliveireJMReisRLChitosan nanofibers as scaffolds for peripheral nerve regenerationJ Tissue Eng Reg Med20137Supp 1652
- HsuSHKuoWCChenYTNew nerve regeneration strategy combining laminin-coated chitosan conduits and stem cell therapyActa Biomater2013956606661523376237
- BurdickAJPrestwichGDHyaluronic acid hydrogels for biomedical applicationsAdv Mater20112312H41H5621394792
- SuriSSchmidtCRCell-laden hydrogel constructs of hyaluronic acid, collagen, and laminin for neural tissue engineeringTissue Eng Part A201016517071713
- GuanSZhangXLLinXMLiuTQMaXHCuiZFChitosan/gelatin porous scaffolds containing hyaluronic acid and heparan sulfate for neural tissue engineeringJ Biomater Sci Polym Ed2013248999101423647254
- TianBLiuJDvirTMacroporous nanowire nanoelectronic scaffolds for synthetic tissuesNat Mater20121198699422922448
- LuMCHuangYTLinJHEvaluation of a multi-layer micro-braided polylactic acid fiber-reinforced conduit for peripheral nerve regenerationJ Mater Sci Mater Med2009201175118019115095
- HsuSHChanSHChiangCMPeripheral nerve regeneration using a microporous polylactic acid asymmetric conduit in a rabbit long-gap sciatic nerve transection modelBiomaterials2011323764377521396706
- MatsumineHSasakiRYamatoMOkanoTSakuraiHA polylactic acid non-woven nerve conduit for facial nerve regeneration in ratsJ Tissue Eng Regen Med20148645446222689468
- NiHCTsengTCChenJRHsuSHChiuIMFabrication of bioactive conduits containing the fibroblast growth factor 1 and neural stem cells for peripheral nerve regeneration across a 15 mm critical gapBiofabrication2013503501023880639
- EvansGRDBrandtKWidmerMSIn vivo evaluation of poly(L-lactic acid) porous conduits for peripheral nerve regenerationBiomaterials1999201109111510382826
- RutkowskiGEMillerCAJeftinijaSMallapragadaSKSynergistic effects of micropatterned biodegradable conduits and Schwann cells on sciatic nerve regenerationJ Neural Eng2004115115715876634
- LiJMcNallyHShiREnhanced neurite alignment on micro-patterned poly-L-lactic acid filmsJ Biomed Mater Res Part A200887392404
- LiuJJWangCYWangJGPeripheral nerve regeneration using composite poly(lactic acid-caprolactone)/nerve growth factor conduits prepared by coaxial electrospinningJ Biomed Mater Res Part A201196120
- XuHHolzwarthJMYanYConductive PPY/PDLLA conduit for peripheral nerve regenerationBiomaterials20143522523524138830
- TabeshHAmoabedinyGNikNSThe role of biodegradable engineered scaffolds seeded with Schwann cells for spinal cord regenerationNeurochem Int200954738319084565
- DellonALChangBWAn alternative incision for approaching recurrent median nerve compression at the wristPlast Reconstr Surg1992895765781535853
- CostaHJZRBentoRFSalomoneRMesenchymal bone marrow stem cells within polyglycolic acid tube observed in vivo after six weeks enhance facial nerve regenerationBraın Res20131510102123542586
- SundbackCHadlockTCheneyMManufacture of porous polymer nerve conduits by a novel low-pressure injection molding processBiomaterials20032481983012485800
- YangYDe LaporteLRivesCBNeurotrophin releasing single and multiple lumen nerve conduitsJ Control Release200510443344615911044
- WenXTrescoPAFabrication and characterization of permeable degradable poly(DL-lactide-co-glycolide) (PLGA) hollow fiber phase inversion membranes for use as nerve tract guidance channelsBiomaterials2006273800380916564567
- OhSHKimJHSongKSPeripheral nerve regeneration within an asymmetrically porous PLGA/Pluronic F127 nerve guide conduitBiomaterials2008291601160918155135
- LeeJYBashurCAGoldsteinASPolypyrrole-coated electro-spun PLGA nanofibers for neural tissue applicationsBiomaterials2009304325433519501901
- ShenHShenZLZhangPHCiliary neurotrophic factor-coated polylactic-polyglycolic acid chitosan nerve conduit promotes peripheral nerve regeneration in canine tibial nerve defect repairJ Biomed Mater Res B Appl Biomater20109516117020737557
- DalyWTKnightAMWangHComparison and characterization of multiple biomaterial conduits for peripheral nerve repairBiomaterials2013348630863923937914
- SunMKinghamPJReidAJIn vitro and in vivo testing of novel ultra thin PCL and PCL/PLA blend films as peripheral nerve conduitJ Biomed Mater Res Part A20109314701481
- OliveiraJTAlmeidaFMBiancalanaAMesenchymal stem cells in a polycaprolactone conduit enhance median-nerve regeneration, prevent decrease of creatine phosphokinase levels ın muscle, and improve functional recovery in miceNeuroscience20101701295130320800664
- MoroderPRungeMBWangHMaterial properties and electrical stimulation regimens of polycaprolactone fumarate-polypyrrole scaffolds as potential conductive nerve conduitsActa Biomater2011794495320965280
- RuiJDadsetanMRungeMBControlled release of vascular endothelial growth factor using poly-lactic-co-glycolic acid microspheres: in vitro characterization and application in polycaprolactone fumarate nerve conduitsActa Biomater2012851151822019759
- FrattiniFLopesFRPAlmeidaFMMesenchymal stem cells in a polycaprolactone conduit promote sciatic nerve regeneration and sensory neuron survival after nerve injuryTıssue Eng Part A2012182030203922646222
- MobasseriSATerenghiGDownesSMicro-structural geometry of thin films intended for the inner lumen of nerve conduits affects nerve repairJ Mater Sci Mater Med20132471639164723572143
- KimJROhSHKwonGBAcceleration of peripheral nerve regeneration through asymmetrically porous nerve guide conduit applied with biological/physical stimulationTissue Eng Part A2013192674268523859225
- RadulescuDDharSYoungCMTissue engineering scaffolds for nerve regeneration manufactured by ink-jet technologyMater Scı Eng C200727534539
- McConnellMPDharSNguyenTNerve growth factor expression response to induction agent booster dosing in transfected human embryonic kidney cellsPlast Reconstr Surg2005115250651415692357
- ChiriacSFaccaSDiaconuMExperience of using the bioresorbable copolyester poly(DL-lactide-ε-caprolactone) nerve conduit guide Neurolac™ for nerve repair in peripheral nerve defects: report on a series of 28 lesionsJ Hand Surg Eur201137342349
- YinDWangXYanYZhangRPreliminary studies on peripheral nerve regeneration using a new polyurethane conduitJ Bioact Compat Polym200722143159
- NiuYChenKCHeTScaffolds from block polyurethanes based on poly(3-caprolactone) (PCL) and poly(ethylene glycol) (PEG) for peripheral nerve regenerationBiomaterials2014354266427724582378
- RutkowskiGEHeathCADevelopment of a bioartificial nerve graft. II. Nerve regeneration in vitroBiotechnol Prog20021837337911934309
- ShokrgozarMAMottaghitalabFMottaghitalabVFabrication of porous chitosan/poly(vinyl alcohol) reinforced single-walled carbon nanotube nanocomposites for neural tissue engineeringJ Bıomed Nanotechnol201171921626892
- AlhosseiniSNMoztarzadehFMozafariMSynthesis and characterization of electrospun polyvinyl alcohol nanofibrous scaffolds modified by blending with chitosan for neural tissue engineeringInt J Nanomed201272534
- BergethonPRTrinkaus-RandallVFranzblauCModified hydroxyethylmethacrylate hydrogels as a modelling tool for the study of cell-substratum interactionsJ Cell Sci1989921111212777911
- LeeJCuddihyMJKotovNAThree-dimensional cell culture matrices: state of the artTıssue Eng Part B2008146186
- DhootNOTobiasCAFischerIPeptide-modified alginate surfaces as a growth permissive substrate for neurite outgrowthJ Biomed Mater Res200471A191200
- YuTTShoichetMSGuided cell adhesion and outgrowth in peptide-modified channels for neural tissue engineeringBiomaterials2005261507151415522752
- GuptaDTatorCHShoichetMSFast-gelling injectable blend of hyaluronan and methylcellulosefor intrathecal, localized delivery to the injured spinal cordBiomaterials2006272370237916325904
- WangXCuiTYanYZhangRPeroneal nerve regeneration using a unique bilayer polyurethane-collagen guide conduitJ Bioact Compat Polym2009242109127
- XuHYanYLiSPDLLA/chondroitin sulfate/chitosan/NGF conduits for peripheral nerve regenerationBiomaterials2011324506451521397324
- LeeBKJuYMChoJGEnd-to-side neurorrhaphy using an electrospun PCL/collagen nerve conduit for complex peripheral motor nerve regenerationBiomaterials2012339027903622998812
- YucelDTorun KoseGHasircıVPolyester based nerve guidance conduit designBiomaterials20103171596160319932504
- YucelDTorun KoseGHasircıVTissue engineered, guided nerve tube consisting of aligned neural stem cells and astrocytesBiomacromolecules2010113584359121070042
- CooperABhattaraiNZhangMFabrication and cellular compatibility of aligned chitosan–PCL fibers for nerve tissue regenerationCarbohydr Polym2011851149156
- KiyotaniTTeramachiMTakimotoYNerve regeneration across a 25-mm gap bridged by a polyglycolic acid-collagen tube: a histological and electrophysiological evaluation of regenerated nervesBrain Res199674066748973799
- MatsumatoKOhnishiKKiyotaniTPeripheral nerve regeneration across an 80-mm gap bridged by a polyglycolic acid (PGA)-collagen tube filled with laminin-coated collagen fibers: a histological and electrophysiological evaluation of regenerated nervesBrain Res200086831532810854584
- TobaTNakamuraTShimizuYRegeneration of canine peroneal nerve with the use of a polyglycolic acid-collagen tube filled with laminin-soaked collagen sponge: a comparative study of collagen sponge and collagen fibers as filling materials for nerve conduitsJ Biomed Mater Res20015862263011745513
- NakamuraTInadaYFukudaSExperimental study on the regeneration of peripheral nerve gaps through a polyglycolic acid-collagen (PGA-collagen) tubeBrain Res20041027182915494153