Abstract
Traumatic brain injury (TBI) has emerged as a leading cause of mortality and morbidity worldwide. Transplantation of bone mesenchymal stem cells (BMSCs) has emerged as a promising treatment for various central nervous system diseases. This study aims to evaluate the effect of BMSCs transplantation by intravenous injection on neurological function and angiogenesis of the TBI mice. C57BL/6 male mice were randomly divided into four groups: control, sham, TBI, and BMSC. Functional neurological evaluation was performed 1, 4, 7, 14, and 21 days after TBI using neurological severity scores. The impairment of learning and memory in mice was evaluated 14 days after TBI by Morris water maze experiment. Mice were sacrificed 14 days after TBI, and then brain sections were stained by terminal deoxyribonucleotidyl transferase (TDT)-mediated dUTP-digoxigenin nick end labeling staining to assess brain neuronal apoptosis. Immunohistochemistry was conducted to evaluate caspase-3 activity and identify vascular distribution and microvessel density. Protein and mRNA levels of vascular endothelial growth factor (VEGF) and angiogenin-1 (Ang-1) in brain tissues were analyzed by Western blot and quantitative real-time polymerase chain reaction, respectively. BMSCs transplantation promoted recovery of neurological function, ameliorated impairment of learning and memory, attenuated neuronal apoptosis, and diminished caspase-3 activation in mice after TBI. Also, BMSCs transplantation upregulated expressions of VEGF and Ang-1 and promoted the formation of microvessels in brain tissues after TBI. Our study demonstrated the important role of BMSCs transplantation in TBI mouse and indicated that the underlying mechanism was through promoting angiogenesis and improving neurological function. This provides a novel and effective strategy for cell-based therapy in the treatment of TBI.
Introduction
Traumatic brain injury (TBI) has emerged as a leading cause of mortality and morbidity worldwide.Citation1 It is defined as an alteration in brain function and/or other evidence of brain pathology caused by a sudden external mechanical force, such as rapid acceleration or deceleration, blast waves, crush, an impact, or penetration by a projectile.Citation1,Citation2 TBI survivors frequently suffer from temporary or permanent impairment of cognitive, physical, and psychosocial functions.Citation3–Citation5 It is widely acknowledged that the neurons in the human hippocampus, which are critical for learning and memory,Citation6 are extremely vulnerable to brain trauma. Once injured, the neurons will experience massive degeneration and necrosis, leading to dysfunction of learning and memory. Despite major progress in understanding the pathophysiology of TBI, promising neuroprotective drugs effective in animal TBI models, unfortunately, have all failed in Phase II or Phase III clinical trials.Citation1 Therefore, further investigation in pharmacological treatments, neural repair and regeneration, or other treatments is urgently needed to improve outcomes for TBI patients.Citation7
Bone mesenchymal stem cells (BMSCs) provide hematopoietic support and exhibit immunoregulation function, with multilineage differentiation potential (pluripotency) and self-renewal ability.Citation8 The transplanted BMSCs could be differentiated into neurons and glial cells.Citation9 Of note, BMSCs have been shown to be an excellent candidate for cell therapy.Citation10 They can be obtained from autologous donation, thus eliminating ethical disputes and other concerns associated with graft rejection.Citation11 In addition, clinical studies have shown that human BMSCs would not cause the activation of the host’s immune system,Citation12 suggesting that even allogeneic BMSCs can be used for transplantation therapy. Human trials have shown so far no adverse effects on allogeneic vs autologous BMSCs transplantation.Citation13 Transplantation of BMSCs has been shown to promote the repair and regeneration of nerve tissues in the central nervous system (CNS)Citation14 and has emerged as a promising treatment for various CNS diseases,Citation15 such as stroke, TBI, intracerebral hemorrhage, and spinal cord injury.
In our study, BMSCs were transplanted into TBI model to evaluate the effect of BMSCs on neurological function and angiogenesis of the host mice. Also, the related underlying mechanism was further explored. Our study provided supports for the clinical application of BMSCs in the treatment of TBI.
Materials and methods
BMSCs culture
BMSCs were harvested as previously described by Wei et al.Citation16 In brief, mice were anesthetized and sacrificed by cervical dislocation. BMSCs were rinsed out from the femurs and tibias of mice by a 20-gauge syringe. The cells were suspended in DMEM containing 10% fetal bovine serum and incubated at 37°C with 5% CO2 for 24 hours. The medium containing the nonadherent cells was removed, and then fresh medium was added to allow for selection by plastic adhesion. After BMSCs isolation, the medium was replaced every 3 days, and the cultures were passaged when the BMSCs reached 80% confluence. To confirm cell characteristics of cultured cells, BMSCs were subjected to fluorescence-activated cell sorting using CD90, CD34, and CD45 markers, and BMSCs were identified as CD90 positive and CD34/CD45 negative cells.
Animals
C57BL/6 male mice weighing 20–25 g were purchased from Laboratory Animal Center of Shanghai Institutes for Biological Science. This study was approved by the Ethics Committee of the First Affiliated Hospital of Zhengzhou University. All animal procedures were in compliance with the National Institutes of Health (NIH) Guidelines for the Care and Use of Laboratory Animals. Mice (N=60) were randomly divided into four groups with 15 for each: control, sham (received sham operation without cortical impact), TBI (received TBI injury), and BMSC (received intravenous injection of 2×106/μL BMSCs at tail vein after TBI).
Establishment of mice TBI model
Establishment of mice TBI model was performed as described by Hunt et al,Citation17 with some alterations. Briefly, mice were anesthetized with 4% chloral hydrate (0.4 mg/g) and positioned in a stereotaxic apparatus. A midline incision was made to expose the skull. A 4-mm craniotomy was performed lateral to the sagittal suture and centered between bregma and lambda using a dental drill. The skull cap was carefully removed without disruption of the exposed underlying dura, and mice were then subjected to TBI using a controlled cortical impact device. A standardized parietal contusion was performed by letting a 20 mg steel rod with a flat end and a diameter of 2 mm drop on a piston resting on the dura from a height of 50 cm. The piston was allowed to compress the cortex to a depth of 1.0 mm, with the same duration. After injury, the incision was sutured, and mice were carefully observed and nursed. Each group guaranteed at least five animals for the final surviving behavior, water maze, and morphological examination.
Neurological assessment
A standardized neurological severity score (NSS) was performed to assess the extent of neurological impairment in a blinded fashion by two different investigators at defined time intervals after trauma (t=1, 4, 7, 14, and 21 days), as described by Leinhase et al and Stahel et al.Citation18,Citation19 NSS consists of 10 individual parameters, including tasks on motor function, alertness, and physiological behavior, where one point indicates failure of the task and no point indicates success. The maximum NSS score (10 points) reflects the severest neurological impairment with failure of all tasks.
Morris water maze
To evaluate the impairment of learning and memory in mice, Morris water maze (MWM) experiment was performed 14 days after TBI experiment, as described previouslyCitation20,Citation21 with some alterations. The apparatus includes a circular pool (180 cm in diameter, 45 cm in depth) filled with water (30 cm in depth) and plexiglass platform (10 cm in diameter). The pool is virtually subdivided into four equal quadrants. The platform was placed 1 cm beneath the surface of the water. Animal behaviors were monitored by the EthoVision system. Mice which were able to find the platform within 20–40 seconds in the training session were selected for MWM experiment. Spatial navigation (invisible platform) and probe trials were performed. In brief, the platform was placed at the center of a fixed quadrant. The mice were placed in the water at the opposite side of the platform and the time they spent reaching the underwater platform is the escape latency. After the completion of spatial navigation trial, original platform was removed and the mice were placed in the water at opposite site of the original platform, and the swimming tracks of mice within 2 minutes were recorded. The percentage of target zone entries, the percentage of time spent finding the target zone, and the number of times crossing the site (original platform) were calculated.
Neuronal apoptosis assay and evaluation of caspase-3 activity
Fourteen days after TBI experiment, four mice in each group were randomly selected and sacrificed under anesthesia. Brain tissues were isolated and paraffin sections were prepared. To evaluate the apoptosis of brain neurons, the sections were subjected to terminal deoxyribonucleotidyl transferase (TDT)-mediated dUTP-digoxigenin nick end labeling (TUNEL) staining. Briefly, the sections (4 μm) were dewaxed, hydrated, and washed. After that, proteinase K (20 μL/mL) and Triton X-100 in 0.1% sodium citrate were added successively. Then the sections were incubated in 0.3% H2O2/methanol, followed by incubation with TUNEL reaction mixture (50 g; Boehringer Co., Mannheim, Germany) at 37°C in a humidified chamber. Finally, the sections were stained with diaminobenzidine (DAB). For evaluation of caspase-3 activity, immunohistochemical staining was conducted to observe the expression of caspase-3. Images were acquired by an IX81 Olympus microscope (Olympus, Tokyo, Japan).
Western blot
Fourteen days after TBI experiment, four mice in each group were randomly selected and sacrificed under anesthesia. Brain tissues were isolated and subjected to Western blot. In brief, tissues were lysed in in 200 μL lysis buffer. Then equal amount of protein from cell lysates was separated by 10% sodium dodecyl sulfate-polyacrylamide gel electrophoresis gels and transferred to polyvinylidene difluoride membrane (EMD Millipore, Billerica, MA, USA). After that, primary antibodies against vascular endothelial growth factor (VEGF) or angiogenin-1 (Ang-1) (Santa Cruz Biotechnology Inc., Dallas, TX, USA) were added, followed by horseradish peroxidase (HRP)-conjugated goat anti-rabbit IgG secondary antibodies. GAPDH was used as an internal control. The protein analysis was visualized by Quantity One software (Bio-Rad Laboratories Inc., Hercules, CA, USA).
RNA extraction and quantitative real-time polymerase chain reaction
Fourteen days after TBI experiment, four mice in each group were randomly selected and sacrificed under anesthesia. Brain tissues were isolated and subjected to Western blot. Total RNA was extracted from tissues and cells by using Trizol reagent (Thermo Fisher Scientific, Waltham, MA, USA) and was reverse transcribed into cDNAs using the reverse transcription kit (Takara, Shiga, Japan). The cDNA template was synthesized through quantitative real-time polymerase chain reaction (qRT-PCR) using SYBR Premix Dimmer Eraser kit (Takara) by the ABI7900 system (Thermo Fisher Scientific). The relative expressions of VEGF and Ang-1 were calculated by the 2−ΔΔCt method and normalized to the internal control GAPDH.
Immunohistochemistry and microvessel counting
Vascular distribution and microvessel density (MVD) in mice brain tissues was identified immunohistochemically by monoclonal antibodies against CD34, as previously described.Citation22 Briefly, paraffin-embedded brain tissues (4 μm) were dewaxed, hydrated, and washed. Then 0.3% H2O2 was added to quench the endogenous peroxidase activity. After blocking nonspecific staining by incubation with 5% bovine serum albumin, sections were incubated with the primary specific biotinylated antibodies against CD34 (Cell signaling Technology, Danvers, MA, USA), followed by incubation with HRP-conjugated streptavidin. Staining was visualized with DAB, and sections were counterstained with Mayer’s hematoxylin. Images were acquired by a fluorescence microscope. Quantification of MVD was conducted as described by Weidner et al.Citation23 The mean value of microvessel counts from five randomly selected fields was recorded as MVD of the section.
Statistical analysis
All statistical analyses were performed using SPSS 18.0. Differences between two groups were evaluated by Student’s t-test. Data are presented as the mean ± SD from three independent experiments. Values of P<0.05 were considered statistically significant.
Results
BMSCs transplantation promoted recovery of neurological function in mice after TBI
A standardized NSS was performed to assess the extent of neurological impairment in a blinded fashion at defined time intervals after TBI. The control and sham mice showed relatively normal behavior, as reflected by the low NSS scores. In contrast, a significantly increased NSS was observed at all time intervals after trauma in the TBI mice. However, after treated by transplantation of BMSCs, mice displayed markedly reduced NSS scores (). These results implied that transplantation of BMSCs promoted recovery of neurological function.
Figure 1 BMSCs transplantation promoted recovery of neurological function in mice after TBI. The extent of neurological impairment in control, sham, TBI, and BMSC groups was assessed by a standardized neurological severity score (NSS) at defined time intervals after trauma (t=1, 4, 7, 14, and 21 days). *P<0.05: Sham vs TBI, #P<0.05: BMSC vs TBI.
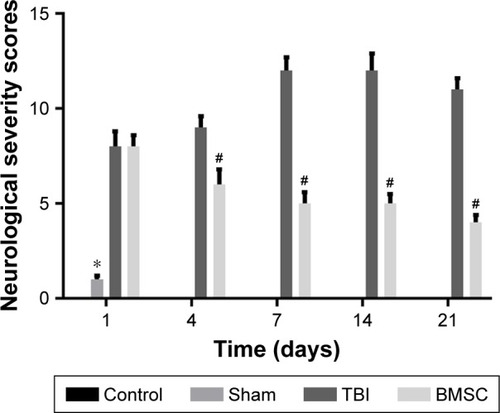
BMSCs transplantation ameliorated the impairment of learning and memory in mice after TBI
To evaluate the impairment of learning and memory in mice, MWM experiment was performed 14 days after TBI experiment. For spatial navigation trial, TBI mice greatly prolonged the escape latency, whereas BMSCs-transplanted mice showed reduced latency, close to the level in control and sham groups (). For spatial probe trial, compared to control and sham groups, a reduction in target zone entries, time in target zone, and number of times crossing the site was observed in TBI mice. However, transplantation of BMSCs induced an increase in the previously mentioned parameters (). Our results indicated that BMSCs transplantation attenuated learning and memory impairment in mice after TBI.
Figure 2 Spatial navigation and probe trials in Morris water maze. (A) The time of latency, (B) the percentage of target zone entries, (C) the percentage of time spent finding the target zone, and (D) the number of times crossing the site (original platform) were calculated. *P<0.05: Sham vs TBI, #P<0.05: BMSC vs TBI.
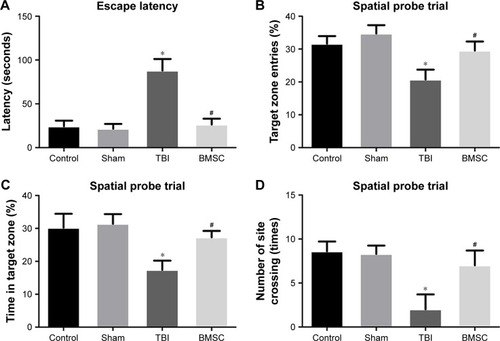
BMSCs transplantation attenuated neuronal apoptosis and diminished caspase-3 activation
Fourteen days after TBI experiment, four mice in each group were randomly selected and sacrificed under anesthesia. Brain tissues were isolated and paraffin sections were prepared. To evaluate the apoptosis of brain neurons, the sections were subjected to TUNEL staining. Data revealed that, compared to control and sham groups, increased TUNEL-positive cells exhibiting typical apoptotic morphology including shrunken nuclei, condensed chromatin, and apoptotic bodies were observed in TBI mice. Meanwhile, mice treated with BMSCs transplantation showed reduced TUNEL-positive cells (). Furthermore, caspase-3 expression was evaluated to confirm the ongoing apoptosis (via the extrinsic pathway) by cleaved caspase-3 immunohistochemical staining. Data demonstrated that, compared to TBI group, the activity of caspase-3 was reduced in BMSCs-transplanted mice (). All these results suggested that BMSCs transplantation reduced apoptotic damage induced by TBI in mice.
Figure 3 BMSCs transplantation attenuated neuronal apoptosis and diminished caspase-3 activation.
Abbreviations: BMSC, bone mesenchymal stem cell; TBI, traumatic brain injury; TUNEL, terminal deoxyribonucleotidyl transferase (TDT)-mediated dUTP-digoxigenin nick end labeling.
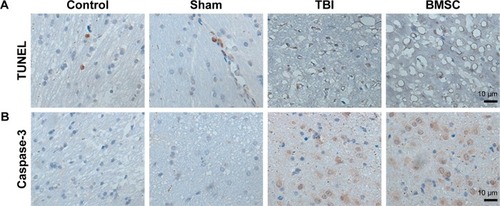
BMSCs transplantation upregulated expressions of VEGF and Ang-1 in brain tissues after TBI
Brain tissues from randomly selected mice in each group were isolated 14 days after TBI experiment. To evaluate the effect of BMSCs transplantation on angiogenesis of TBI-treated mice, expressions of VEGF and Ang-1 at mRNA and protein levels in brain tissues were analyzed by qRT-PCR and Western blot, respectively. Data revealed that TBI mice showed reduced levels of VEGF and Ang-1 compared to control and sham groups. Interestingly, we found that BMSCs transplantation upregulated VEGF and Ang-1 to levels close to those found in control or sham group ().
Figure 4 BMSCs transplantation upregulated expression of VEGF and Ang-1 in brain tissues of TBI mice. The protein and mRNA levels of (A) VEGF and (B) Ang-1 were detected by Western blot and qRT-PCR, respectively. GAPDH served as the control. *P<0.05: Sham vs TBI, #P<0.05: BMSC vs TBI.
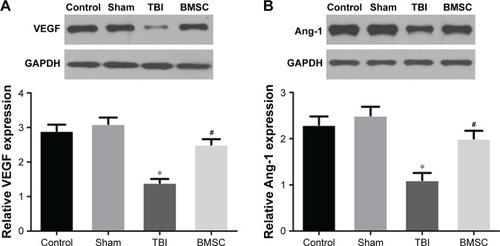
BMSCs transplantation promoted the formation of microvessels in brain tissues after TBI
MVD is regarded as a surrogate marker for angiogenesis.Citation22 To further evaluate the effect of BMSCs transplantation on the formation of microvessels of TBI-treated mice, brain tissues from randomly selected mice in each group were isolated 14 days after TBI experiment, then vascular distribution and MVD in brain tissues was identified immunohistochemically by using monoclonal antibodies against CD34. Data revealed that microvessels within the brain tissues of control and sham mice were strongly stained by CD34. However, microvessels in the brain tissues of TBI mice expressed a minimal level of CD34. Interestingly, mice receiving BMSCs transplantation showed more microvessels stained by CD34 than TBI mice (). Furthermore, MVD determined by CD34 was calculated and showed no significant difference between control and sham groups. MVD in the TBI group was markedly lower than in the control or sham group. BMSC group displayed higher MVD than TBI group but a little lower MVD than control and sham groups (). These results indicated that BMSCs transplantation promoted the formation of microvessels in brain tissues after TBI.
Figure 5 BMSCs transplantation promoted the formation of microvessels in brain tissues after TBI. (A) Microvessels stained by CD34 in brain tissues from mice in the four groups. Scale bar = 10 μm, magnification ×100 and (B) MVD determined by CD34-positive microvessels in the four groups. *P<0.05: Sham vs TBI, #P<0.05: BMSC vs TBI.
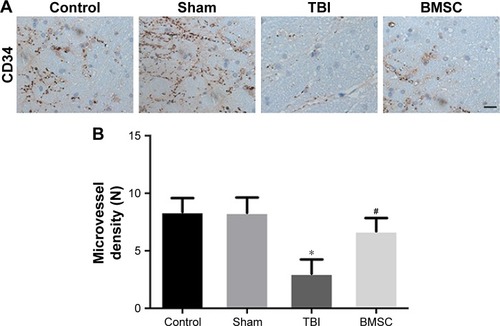
Discussion
TBI presents as a significant health concern and an enormous socioeconomic burden. Unfortunately, to date, there are no pharmacologic agents demonstrated to improve TBI outcomes.Citation4 Thus, there is a compelling need to develop treatments for TBI. The safety and feasibility of BMSCs transplantation was confirmed in animal modelsCitation24,Citation25 and human trials.Citation26,Citation27 Our study found that cell therapies using BMSCs transplantation intravenously promoted angiogenesis and improved neurological function in TBI mice, thus provided supports for the treatment of TBI.
Misra et alCitation28 indicated that the stem cell therapies are an emerging paradigm in strokes. Brenneman et alCitation29 indicated that autologous bone marrow mononuclear cells can be safely harvested from rodents after stroke, and they are documented to migrate to the peri-infarct area, enhance recovery, and modulate the post-ischemic inflammatory response. Yang et alCitation30 has well characterized the dose response, therapeutic time window, and biodistribiton of mononuclear cells from bone marrow in animal stroke models. Sharma et alCitation31 demonstrated the potential of the intrathecal transplantation of autologous bone marrow mononuclear cells in improving the prognosis of functional recovery in chronic stage of stroke.
Bakhtiary et alCitation32 stated that bromodeoxyuridine (Brdu)-labeled BMSCs were present in the lesion boundary zone by immunohistochemistry analysis after intravenous injection of BMSCs in TBI rats, indicating BMSCs can survive, migrate, and differentiate into neurons in vivo. Also, Chen et alCitation33 proposed that intraventricular injection of BMSCs increased the brain concentration of nerve growth factor and promoted neurological functional recovery in TBI mice. In accordance with this, in our study, we found that BMSCs transplantation by intravenous injection promoted recovery of neurological function in TBI mice, providing new evidence for the improvement of neurological function by BMSCs.
The cognitive dysfunction which TBI patients suffer from is mainly reflected by the impairment of learning and memory, attention, language and communication skills, the ability of identifying time and space, as well as logical reasoning. Mahmood et alCitation34 reported that TBI rats treated with combination therapy of BMSCs and atorvastatin showed attenuated dysfunction of learning and memory reflected by the MWM test. Consistent with this, our study revealed that, compared to the TBI group, a reduction in escape latency and an increase in target zone entries, time in target zone, and number of times crossing the site were observed in the BMSC group, indicating intravenous transplantation of BMSCs effectively improved the learning and memory of TBI mice. In addition, our study also found that BMSCs transplantation ameliorated neuronal apoptosis and diminished activation of caspase-3, an important apoptotic executor in apoptotic cascades. Collectively, we can conclude that BMSCs improve neurological function in TBI mice.
Recent animal study has linked enhanced angiogenesis to improved performance in neurological and behavioral tests.Citation35 Angiogenesis, the growth of new blood vessels, can provide tissue with oxygen and nutrition, ameliorate tissue ischemia, and promote the structural remodeling of damaged brain tissue, thus accelerating the repair of nerve function. Promoting angiogenesis may be a new approach to treat ischemic brain after TBI.Citation4,Citation36–Citation39 Angiogenesis is regulated by the expression of various vascular growth factors and modulators, the most widely expressed and critical regulator of which is VEGF. This protein is secreted by tissues in response to ischemic and inflammatory stimuli and leads to endothelial migration, proliferation, and increased vascular permeability.Citation40 In this study, levels of VEGF in brain gradually reduced 14 days after TBI, and similar results were observed with Ang-1, another stimulator of angiogenesis. It is noteworthy that BMSCs transplantation upregulated expressions of VEGF and Ang-1 to levels close to those found in control or sham group. These findings suggested that BMSCs transplantation may promote angiogenesis through upregulating VEGF and Ang-1, which was then confirmed by the formation of microvessels using immunohistochemistry and microvessel counting. Current investigations of the changes in molecular expression are increasing our understanding of the important role of BMSCs transplantation in TBI therapies; however, clinical studies should be performed to further validate our results. Molecular imaging has been emerging as a novel technology for visualizing metabolism and signal transduction to gene expression,Citation41 which could be used in our further study to explore the signal transduction to VEGF and Ang-1 expression. In conclusion, our study demonstrated the important role of BMSCs transplantation in TBI mouse and indicated that the underlying mechanism was through promoting angiogenesis and improving neurological function. This provides a novel and effective strategy for cell-based therapy in the treatment of TBI.
Disclosure
The authors report no conflicts of interest in this work.
References
- XiongYMahmoodAChoppMAnimal models of traumatic brain injuryNat Rev Neurosci201314212814223329160
- MenonDKSchwabKWrightDWMaasAIPosition statement: definition of traumatic brain injuryArch Phys Med Rehabil201091111637164021044706
- WalkerPAShahSKHartingMTCoxCSJrProgenitor cell therapies for traumatic brain injury: barriers and opportunities in translationDis Model Mech200921–2233819132123
- XiongYZhangYMahmoodAChoppMInvestigational agents for treatment of traumatic brain injuryExpert Opin Investig Drugs2015246743760
- MaasAIStocchettiNBullockRModerate and severe traumatic brain injury in adultsLancet Neurol20087872874118635021
- XuBGaoYZhanSQuantitative protein profiling of hippocampus during human agingNeurobiol Aging201639465626923401
- RosenfeldJVMaasAIBraggePMorganti-KossmannMCManleyGTGruenRLEarly management of severe traumatic brain injuryLancet201238098471088109822998718
- JamesSFoxJAfsariFMultiparameter analysis of human bone marrow stromal cells identifies distinct immunomodulatory and differentiation-competent subtypesStem Cell Reports2015461004101526070611
- BaoCWangYMinHCombination of ginsenoside Rg1 and bone marrow mesenchymal stem cell transplantation in the treatment of cerebral ischemia reperfusion injury in ratsCell Physiol Biochem201537390191026384017
- ParekkadanBMilwidJMMesenchymal stem cells as therapeuticsAnnu Rev Biomed Eng2010128711720415588
- MalgieriAKantzariEPatriziMPGambardellaSBone marrow and umbilical cord blood human mesenchymal stem cells: state of the artInt J Clin Exp Medicine201034248269
- TseWTPendletonJDBeyerWMEgalkaMCGuinanECSuppression of allogeneic T-cell proliferation by human marrow stromal cells: implications in transplantationTransplantation200375338939712589164
- FouillardLChapelABoriesDInfusion of allogeneic-related HLA mismatched mesenchymal stem cells for the treatment of incomplete engraftment following autologous haematopoietic stem cell transplantationLeukemia200721356857017252011
- Nandoe TewarieRDHurtadoALeviADGrotenhuisJAOudegaMBone marrow stromal cells for repair of the spinal cord: towards clinical applicationCell Transplant200615756357717176609
- YalvacMEYilmazAMercanDDifferentiation and neuro-protective properties of immortalized human tooth germ stem cellsNeurochem Res201136122227223521785835
- WeiLFraserJLLuZYHuXYuSPTransplantation of hypoxia preconditioned bone marrow mesenchymal stem cells enhances angiogenesis and neurogenesis after cerebral ischemia in ratsNeurobiol Dis201246363564522426403
- HuntRFScheffSWSmithBNSynaptic reorganization of inhibitory hilar interneuron circuitry after traumatic brain injury in miceJ Neurosci201131186880689021543618
- LeinhaseIRozanskiMHarhausenDInhibition of the alternative complement activation pathway in traumatic brain injury by a monoclonal anti-factor B antibody: a randomized placebo-controlled study in miceJ Neuroinflammation200741317474994
- StahelPFShohamiEYounisFMExperimental closed head injury: analysis of neurological outcome, blood-brain barrier dysfunction, intracranial neutrophil infiltration, and neuronal cell death in mice deficient in genes for pro-inflammatory cytokinesJ Cereb Blood Flow Metab200020236938010698075
- InostrozaMCidEBrotons-MasJHippocampal-dependent spatial memory in the water maze is preserved in an experimental model of temporal lobe epilepsy in ratsPloS one201167e2237221829459
- SunYXDaiDKLiuRTherapeutic effect of SN50, an inhibitor of nuclear factor-kappaB, in treatment of TBI in miceNeurol Sci201334334535522437493
- DingSLiCLinSComparative evaluation of microvessel density determined by CD34 or CD105 in benign and malignant gastric lesionsHum Pathol200637786186616784986
- WeidnerNSempleJPWelchWRFolkmanJTumor angiogenesis and metastasis – correlation in invasive breast carcinomaN Eng J Med1991324118
- LuDMahmoodAWangLLiYLuMChoppMAdult bone marrow stromal cells administered intravenously to rats after traumatic brain injury migrate into brain and improve neurological outcomeNeuroreport200112355956311234763
- MahmoodALuDQuCGoussevAChoppMHuman marrow stromal cell treatment provides long-lasting benefit after traumatic brain injury in ratsNeurosurgery200557510261031 discussion 1026–103116284572
- ZhangZXGuanLXZhangKZhangQDaiLJA combined procedure to deliver autologous mesenchymal stromal cells to patients with traumatic brain injuryCytotherapy200810213413918368592
- TianCWangXWangXAutologous bone marrow mesenchymal stem cell therapy in the subacute stage of traumatic brain injury by lumbar punctureExp Clin Transplant201311217618122891928
- MisraVYangBSharmaSSavitzSCell-Based Therapy for StrokeTotowa, NJHumana Press2011143161
- BrennemanMSharmaSHartingMAutologous bone marrow mononuclear cells enhance recovery after acute ischemic stroke in young and middle-aged ratsJ Cereb Blood Flow Metab201030114014919773802
- YangBStrongRSharmaSTherapeutic time window and dose response of autologous bone marrow mononuclear cells for ischemic strokeJ Neurosci Res201189683383921412816
- SharmaASaneHGokulchandranNAutologous bone marrow mononuclear cells intrathecal transplantation in chronic strokeStroke Res Treat20142014201423409525126443
- BakhtiaryMMarzbanMMehdizadehMComparison of transplantation of bone marrow stromal cells (BMSC) and stem cell mobilization by granulocyte colony stimulating factor after traumatic brain injury in ratIran Biomed J201014414214921283256
- ChenQLongYYuanXProtective effects of bone marrow stromal cell transplantation in injured rodent brain: synthesis of neurotrophic factorsJ Neurosci Res200580561161915880454
- MahmoodALuDQuCGoussevAChoppMTreatment of traumatic brain injury with a combination therapy of marrow stromal cells and atorvastatin in ratsNeurosurgery2007603546553 discussion 553–55417327800
- CaplanAIDennisJEMesenchymal stem cells as trophic mediatorsJ Cell Biochem20069851076108416619257
- XiongYQuCMahmoodADelayed transplantation of human marrow stromal cell-seeded scaffolds increases transcallosal neural fiber length, angiogenesis, and hippocampal neuronal survival and improves functional outcome after traumatic brain injury in ratsBrain Res2009126318319119368838
- XiongYMahmoodAMengYDelayed administration of erythropoietin reducing hippocampal cell loss, enhancing angiogenesis and neurogenesis, and improving functional outcome following traumatic brain injury in rats: comparison of treatment with single and triple doseJ Neurosurg2010113359860819817538
- LuDGoussevAChenJAtorvastatin reduces neurological deficit and increases synaptogenesis, angiogenesis, and neuronal survival in rats subjected to traumatic brain injuryJ Neurotrauma2004211213214987462
- XiongYMahmoodAChoppMAngiogenesis, neurogenesis and brain recovery of function following injuryCurr Opin Investig Drugs2010113298308
- PandyaNMDhallaNSSantaniDDAngiogenesis – a new target for future therapyVascul Pharmacol200644526527416545987
- SanduNSchallerBStem cell transplantation in brain tumors: a new field for molecular imaging?Mol Med201016943343720593112