Abstract
Objective
There is a relationship between non-rapid eye movement (NREM) sleep and Alzheimer’s disease (AD). The rostromedial tegmental nucleus (RMTg) is activated can enhance NREM. Therefore, our experiment was designed to investigate the effects of activation of RMTg by chemical genetic techniques on APP/PS1 mice learning and memory.
Materials and Methods
After the AAV-hSyn-hM3Dq-mCherry virus was injected into the RMTg nucleus, CNO solution was intraperitoneally injected to activate RMTg. The new object test and Morris water maze were used to determine the learning and memory level; T2-weighted imaging (T2WI) scanning was performed to analyze the volume of hippocampus and entorhinal cortex of each group; The virus transfection status was determined by laser confocal microscope and use immunohistochemical detection to observe the deposition of Beta Amyloid 1–42 (Aβ42).
Results
Activation of RMTg by chemical genetic techniques can reduce the escape latency and increase discrimination index (RI) and the number of crossing platform; Activation of RMTg by chemical genetic techniques reduced the atrophy of the entorhinal cortex. Aβ42 deposition in the brain was decreased after activation of RMTg.
Conclusion
Activation of the RMTg nucleus by chemogenetic techniques can improve the learning and memory impairment in APP/PS1 mice.
Introduction
Alzheimer’s disease (AD) is the most common neurodegenerative disease encountered in patients over 60 years.Citation1 Without effective prevention strategies or curative treatments, the number of AD patients will reach 42 million by 2020 and 81 million by 2040.Citation2 Owing to prevalence and the accelerating aging of the population, AD is a major public health problem.Citation3 AD is a highly complex and progressive neurodegenerative disease, characteristics of AD are extracellular aggregates of amyloid-β (Aβ) plaques and intracellular aggregations of neurofibrillary tangles.Citation4
Recent studies have shown a growing relationship between sleep and AD.Citation5–8 Sleep, particularly non-rapid eye movement (NREM) sleep, has specific electrophysiological hallmarks that have garnered extensive interest in the context of brain plasticity and relationship to human behavior. NREM sleep slow waves (SWA) and spindles are two oscillatory activities extensively involved in the processes of memory consolidation.Citation9 NREM sleep is essential to memory consolidation after memory formation.Citation10 Numerous experiments have established that NREM sleep disorder causes accumulation of Aβ in Cerebrospinal fluidCitation11,Citation12 and morbidity of AD.Citation13 Ju YS found a strong association between SWA and amyloid-β, where greater SWA disruption was associated with increased Aβ.Citation14 In APP/PS1 mice, NREM quality also decreased, and this phenomenon appeared before the deposition of Aβ.Citation15,Citation16 So, reduced NREM sleep is associated with AD neuropathology.
The rostromedial tegmental nucleus (RMTg) is a GABAergic structure.Citation17 Studies have shown that RMTg is involved in reward system, aversion to stimuli, drug addiction and motor suppression.Citation18,Citation19 Recently, a study indicated that activating the RMTg neurons by pharmacogenetics can increase and deepen NREM sleep.Citation20 In general, RMTg is a key nucleus that activates NREM sleep.
Yet effective pharmacological agents have not been found to provide symptomatic relief for AD that can restore quality of life. We believe that intervention to change the occurrence and development of AD from the perspective of sleep would be a good entry point. Since the activation of RMTg can improve NREM, we investigated that chemogenetic activation of RMTg can improve the learning and memory function of AD. In the present study, we investigated the effects of activation of RMTg by chemogenetic techniques on learning and memory function and Aβ deposition in APP/PS1 mice.
Materials and Methods
Animal and Groups
The SPF APP/PS1 mice and wild-type experimental mice C57BL/6J used in this study were purchased from the Institute of Model Animals of Nanjing University (SYXK2013-009). The experimental animals were reared and bred in the SPF Laboratory of the Laboratory Animal Center of Fujian University of Traditional Chinese Medicine with a temperature of 22°C and a humidity of 50% to 70%. The mice were genotyped by PCR using DNA isolated from tail tissues ().
Figure 1 APP/PS1 PCR identification results. 1, 2, 3, 7, 8 were APP/PS1 double transgenic positive mice. 4, 5, 6, 9, 10 and 11 were wild control mice. N is negative control; P is positive control; W is the blank control.
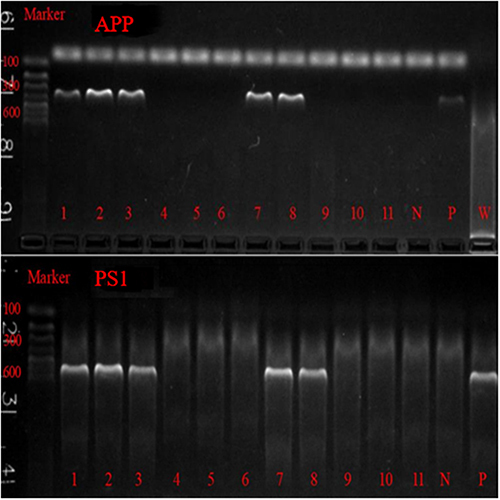
After genotyping, 30 APP/PS1 mice (4 months) were randomly divided into AD group, CNO group and SA group (n=10 in each group), WT mice (4 months) were randomly divided into WT group (n=10) (). This animal experiment followed the Ethical Review Form for Experimental Animal Welfare of Fujian University of Traditional Chinese Medicine (2019) and was approved by the Experimental Animal Ethics Committee of Fujian University of Traditional Chinese Medicine.
Activation of the RMTg
We used the parameters provided by the stereotaxic map of the mouse brain for localization. RMTg: (coordinates from bregma, anterior to posterior 3.75mm, medial to lateral 1.1mm, dorsal to ventral 4.4mm). A 200nl AAV-hSyn-hM3Dq-mCherry virus (Wuhan Shumi Technology, China) was injected into each side of RMTg nucleus. The speed of injection was 35 nl/min. After successful injection, stayed for 10 minutes and slowly pulled out to prevent the virus from overflowing. The mice were fully hemostasis, sutured, and placed in an incubator to wait for awakening. After virus injection, waiting 3 weeks for virus transfection.
After successful transfection, CNO solution (0.33 mg/mL) was intraperitoneally injected into the CNO group mice at 0.2mL/20g, and intervention was carried out five times a week, four weeks; SA group was injected with normal saline, and other injection conditions were the same as those of CNO group.
Morris Water Maze Test
The circular pool is divided into 4 quadrants, the platform is located in a quadrant, 2 cm below the water surface. In the first four days, the time it takes to get on the platform is their escape latency. If the mouse does not get on the platform in 90s, they were guided to the platform by the experimenter and kept there for 15s. Their escape latency were recorded as 90s. In the fifth day, the platform was removed. All mice were allowed to swim freely in the pool for 90s. The number of crossings over the original platform were recorded. The whole process was recorded using a video camera, which is transferred to the computer and recorded and stored, including incubation period, swimming distance, swimming speed, number of crossing platforms, time of first crossing platform, and swimming distance data of the quadrant where the platform is located.
New Object Recognition Task
The New Object Recognition experiment evaluates the cognitive memory ability of animals by the behavioral method of the length of exploration of familiar and new unfamiliar objects by experimental animals.Citation21 New object experiments take three days to complete. The first day was the adaptation period: mice were allowed to explore freely in the open field for 10 min to adapt to the open field environment. The day 2 was the learning period: two identical objects A and A were placed in the left and right corners under the open field. Then, placed into the mice to explore for 10 min. The day 3 is the test period: change object A in the right corner into object B, and object B is different from object A in shape and color. The mice were allowed to explore for 5 min and their trajectories were recorded. The video was recorded from the camera above the test box, and the recognition index (RI) was calculated according to the exploration time of the experimental mice for objects A and B.
Magnetic Resonance Imaging
Bruker Biospec 7.0T (70/20USR MRS scanner, Bruker Biospin, Germany) was used to scan. The specific operation was as follows: before the scanning, the mouse was anesthetized with 1.2–1.5% isoflurane and 30% oxygen mixed gas, then fixing the mouse head in the center of the special surface coil. During the scanning, the warm water circulation heater was used to control the body temperature of the mice at about 37 ° C, and the respiratory rate was monitored in real time. The T2-weighted imaging anatomical structure image scanning application fast spin echo (TubroRARE) sequence, the scan parameters are as follows: echotime (TE)/7 repetition time (TR) 65ms/16000ms, field of view (FOV) 20mm × 20mm, slices 75, slice thickness 0.2mm, slicegap0, matrix 256 × 256, flipangle (FA) 90°.
Immunohistochemical
Mice were anesthetized with 300–500 mL/min of inhaled isoflurane, we transcardially perfused with Normal saline 0.9% followed by 4°C paraformaldehyde. The brain tissue was dehydrated in gradient and embedded into wax blocks. Conventional dewaxing (xylene 15 min×2 times), alcohol hydration (100%, 95%, 75%, 50%, double steamingWater, 5 min each), citrate repair (pH 6.0, pressure cooker repair Compound 15min). The tissue sections were blocked with 5% BSA for 1 h at 37 °C. Then, the sections were incubated with primary antibodies (1:200; 25524-1-AP; Proteintech) overnight at 4 °C. The next day, the sections were washed with PBS and then incubated with appropriate secondary antibodies (SABC-POD (F), rabbit IgG, SA1028, Boster, China) at room temperature for 1 h. After being redyed with hematoxylin, they were dehydrated and mounted after transparence.
The Expression of hM3Dq Receptor
The whole brain tissues of the mice were perfused with 4% paraformaldehyde. After 24 hours, the cerebellums of the mice were cut off. Then, the brain tissue of the mice was stood upright on the base of the vibratome and fixed with glue for continuous coronary sectioning (30μm thickness). We selected two planes of RMTg for sectioning and observed the expression of hM3Dq receptor in RMTg ().
Statistical Analysis
Statistical analyses were performed using SPSS22.0. Normally distributed quantitative data are expressed as mean±standard deviation (x¯±s). A difference with P < 0.05 was considered statistically significant.
Results
Activation of the RMTg Could Ameliorate the Learning and Memory Impairment in APP/PS1 Transgenic Mice
In the new object recognition task, compared with WT group, RI was lower in AD group (P<0.01), CNO group (P<0.05), SA group (P<0.01). CNO group the RI was higher when compared with AD group (P<0.01) (). There was no significant difference in AD group and SA group (P>0.05). The Morris water maze is one of the commonly used behavioral measures of visuospatial memory and learning ability in animal models.Citation22 We tested the spatial learning and memory ability by Morris water maze. In the orientation navigation test, AD group had a longer escape latency when compared with WT group in the first four days (P<0.01). CNO group significantly reduced the escape latency compared with the AD group in the first four days (P<0.01). There was no difference between the AD group and the SA group in the first four days (P>0.05) ( and ). Furthermore, in the space exploration test, the AD group passed through the position of the platform fewer times than the WT group (P<0.01). Compared with AD group, CNO group traversed the platform more times (P<0.01). There was no significantly difference in AD group and SA group (P>0.05) (). In general, these data all suggest that activation of the RMTg could diminish the learning and memory impairment in APP/PS1 transgenic mice.
Figure 4 Effect of activation of RMTg nucleus on learning and memory in mice. (A) Discrimination index of each group (%). (B) Trends of the escape latency of each group in training trials. (C) Times across the platform of each group. (D) Typical route trajectory diagram on the fourth day. Compared with the WT group, #P<0.05, # #P<0.01; Compared with AD group, *P<0.05, **P<0.01; Compared with AD group, ^P>0.05.
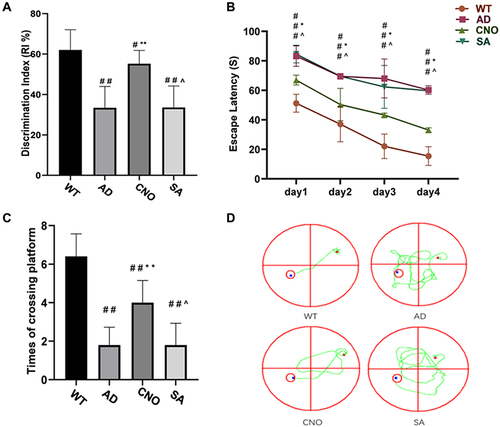
Activation of the RMTg Could Reduce Aβ Deposition in APP/PS1 Transgenic Mice
The effect of activation of RMTg nucleus on Aβ42 deposition in the brain of APP/PS1 mice was investigated (). As demonstrated in , the AD group exhibited a significant increase in Aβ42 deposition (P<0.01 versus the WT group). While, when we activate the RMTg (CNO group), Aβ deposition reduces obviously (P<0.05 versus the AD group). There was no significant difference between AD group and SA group (P>0.05). These results demonstrate that activation of RMTg in APP/PS1 mice effectively decreases the deposition of Aβ42 in the brain.
Figure 5 Effect of activation of RMTg nucleus on Aβ deposition (A–D) Aβ42 deposition in the hippocampus (20×) a: WT group, b: AD group, c: CNO group, d: SA group. (E) The average optical density of Aβ-42 in the brain. Compared with the WT group, #P<0.05, # #P<0.01; Compared with AD group, *P<0.05, **P<0.01; Compared with AD group, ^P>0.05.
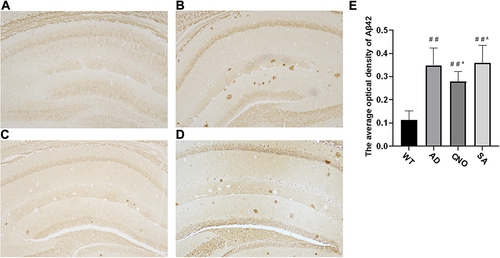
Activation of the RMTg Could Reduce Entorhinal Cortex Volume Atrophy in APP/PS1 Transgenic Mice
The consensus is that hippocampal formation has long been implicated in learning and memory.Citation9 However, the entorhinal cortex also plays an important role. Decreased hippocampal and entorhinal volumes are closely associated with decreased declarative memory.Citation23 We used MRI scans to investigate changes in the volume of the hippocampus () and entorhinal cortex (). We found that there is no difference (P>0.05) on the volume of hippocampus between the four groups (). As for the volume of entorhinal cortex, compared with WT group, the volume of the entorhinal cortex decreased in AD group (P<0.01). The volume of the entorhinal cortex of the CNO group was larger than AD group (P<0.05). AD group was not different from SA group (P>0.05) ().
Figure 6 Effect of activation of RMTg nucleus on the volume of the hippocampus and entorhinal cortex. (A) The hippocampus of experimental mice in WT group. (B) The hippocampus of experimental mice in AD group. (C) The hippocampus of experimental mice in CNO group. (D) The hippocampus of experimental mice in SA group. (E) The entorhinal cortex of experimental mice in WT group. (F) The entorhinal cortex of experimental mice in the AD group. (G) The entorhinal cortex of experimental mice in the CNO group. (H) The entorhinal cortex of experimental mice in the SA group. (I) The volume hippocampus of each group. (J) The volume of entorhinal cortex of each group. Compared with the WT group, #P<0.05,# #P<0.01; Compared with AD group, *P<0.05, **P<0.01; Compared with AD group, ^P>0.05.
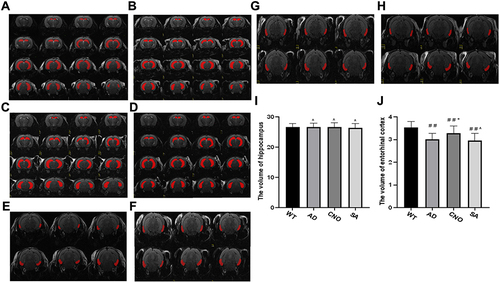
Discussion
In this study, we identified activation of the RMTg nucleus by chemogenetic techniques alleviates the learning and memory impairment in APP/PS1 transgenic mice. First, our data show that APP/PS1 mice in the CNO group performed well relative to the model control group in the behavioral tests (Morris water maze experiment and new object recognition experiment). Second, Aβ is the main pathological sign of AD patients. We found that the Aβ deposition in the CNO group was significantly less than control group. Third, the area of entorhinal cortex of APP/PS1 mice in the CNO group was larger than that of the control group, which is consistent with previous research.Citation24,Citation25 Our experiment found that RMTg activation did not reduce hippocampal volume atrophy, which we thought might be due to the younger age of APP/PS1 mice. A study has shown that APP/PS1 mice show signs of hippocampal atrophy only at 7 months of age.Citation26 Therefore, we plan to next observe the effect of RMTg activation on the hippocampal volume of APP/PS1 mice of different months.
Previous studiesCitation20,Citation27,Citation28 have indicated that NREM is related to the function of the brain to clear Aβ and activated RMTg correlates with NREM sleep quality. Patients with AD experienced a more rapid NREM (especially SWA) decline compared with a normal age group.Citation29 The decrease and interruption of NREM were correlated with Aβ deposition in entorhinal cortex, hippocampus and medial prefrontal cortex.Citation30 In mice, sleep disruption increases amyloid generation and deposition.Citation31 Dopamine is required for normal electrocortical sleep–wake states.Citation32,Citation33 RMTg, a brake of the dopamine system, sending inhibitory projections to multiple arousal-promoting nuclei.Citation34 RMTg is central to sleep regulation. Activation of RMTg by some effective means can prolong NREM sleep time, reduce the accumulation of toxic substances in the brain, and improve brain homeostasis health.Citation20
Most of the researches on the RMTg nucleus focus on drug abuse addiction and aversive behavior,Citation18,Citation35 but there are no reports on the improvement of cognitive function. Our study confirms the role of RMTg in improving cognitive function. We can infer that the activation of RMTg by chemogenetic techniques improves the quality of NREM sleep, thereby reducing the Aβ deposition in the brains of APP/PS1 mice, alleviating the atrophy of the entorhinal cortex of these mice, and finally improving cognitive function of the mice. We provide important evidence for targeted RMTg therapy. Therefore, finding effective intervention methods to activate RMTg becomes a potential therapeutic target for improving AD function.
In the following study, we will focus on discussing the effect of activating RMTg nucleus on improving NREM in APP/PS1 mice. Quantitative assessment was performed using electroencephalogram (EEG) and electromyography (EMG). In AD patients, finding safe and feasible activation modes of the RMTg nucleus is also the focus of our research.
Disclosure
The authors declare that there are no conflicts of interest in this work.
Acknowledgments
This study was funded by Natural Science Foundation of Fujian Province (NO.2021J01954), Medical Innovation Project of Fujian Province (NO. 2021CXA040) and Fujian Province Health and Family Planning Scientific Research talents Training Project youth scientific research project (NO.2018-2-75).
References
- Jedidi H, Jedidi Z, Salmon E. [Alzheimer’s disease]. Rev Med Liege. 2010;65:163–168. Danish.
- Ferri CP, Prince M, Brayne C, et al. Global prevalence of dementia: a Delphi consensus study. Lancet. 2005;366:2112–2117. doi:10.1016/S0140-6736(05)67889-0
- Steel K. Alzheimer’s disease. N Engl J Med. 2010;362(1844):1844–1845.
- Masters CL, Bateman R, Blennow K, Rowe CC, Sperling RA, Cummings JL. Alzheimer’s disease. Nat Rev Dis Primers. 2015;1:15056. doi:10.1038/nrdp.2015.56
- Mander BA, Marks SM, Vogel JW, et al. β-amyloid disrupts human NREM slow waves and related hippocampus-dependent memory consolidation. Nat Neurosci. 2015;18:1051–1057. doi:10.1038/nn.4035
- Lucey BP, McCullough A, Landsness EC, et al. Reduced non-rapid eye movement sleep is associated with tau pathology in early Alzheimer’s disease. Sci Transl Med. 2019;11:1.
- López-García S, Lage C, Pozueta A, et al. Sleep time estimated by an actigraphy watch correlates with CSF Tau in cognitively unimpaired elders: the modulatory role of APOE. Front Aging Neurosci. 2021;13:663446. doi:10.3389/fnagi.2021.663446
- Winer JR, Deters KD, Kennedy G, et al. Association of short and long sleep duration with amyloid-β burden and cognition in aging. JAMA Neurol. 2021;78(10):1187. doi:10.1001/jamaneurol.2021.2876
- Garcia AD, Buffalo EA. Anatomy and function of the primate entorhinal cortex. Annu Rev Vis Sci. 2020;6:411–432. doi:10.1146/annurev-vision-030320-041115
- Marshall L, Helgadóttir H, Mölle M, Born J. Boosting slow oscillations during sleep potentiates memory. Nature. 2006;444:610–613. doi:10.1038/nature05278
- Ju YE, McLeland JS, Toedebusch CD, et al. Sleep quality and preclinical Alzheimer disease. JAMA Neurol. 2013;70:587–593. doi:10.1001/jamaneurol.2013.2334
- Holth JK, Fritschi SK, Wang C, et al. The sleep-wake cycle regulates brain interstitial fluid tau in mice and CSF tau in humans. Science. 2019;363:880–884. doi:10.1126/science.aav2546
- Hahn EA, Wang HX, Andel R, Fratiglioni L. A change in sleep pattern may predict Alzheimer disease. Am J Geriatr Psychiatry. 2014;22:1262–1271. doi:10.1016/j.jagp.2013.04.015
- Ju YS, Ooms SJ, Sutphen C, et al. Slow wave sleep disruption increases cerebrospinal fluid amyloid-β levels. Brain. 2017;140:2104–2111. doi:10.1093/brain/awx148
- Zhang F, Zhong R, Li S, et al. Alteration in sleep architecture and electroencephalogram as an early sign of Alzheimer’s disease preceding the disease pathology and cognitive decline. Alzheimers Dement. 2019;15:590–597. doi:10.1016/j.jalz.2018.12.004
- Kent BA, Michalik M, Marchant EG, et al. Delayed daily activity and reduced NREM slow-wave power in the APPswe/PS1dE9 mouse model of Alzheimer’s disease. Neurobiol Aging. 2019;78:74–86. doi:10.1016/j.neurobiolaging.2019.01.010
- Smith RJ, Vento PJ, Chao YS, Good CH, Jhou TC. Gene expression and neurochemical characterization of the rostromedial tegmental nucleus (RMTg) in rats and mice. Brain Struct Funct. 2019;224:219–238. doi:10.1007/s00429-018-1761-7
- Jhou TC, Fields HL, Baxter MG, Saper CB, Holland PC. The rostromedial tegmental nucleus (RMTg), a GABAergic afferent to midbrain dopamine neurons, encodes aversive stimuli and inhibits motor responses. Neuron. 2009;61:786–800. doi:10.1016/j.neuron.2009.02.001
- Hong S, Jhou TC, Smith M, Saleem KS, Hikosaka O. Negative reward signals from the lateral habenula to dopamine neurons are mediated by rostromedial tegmental nucleus in primates. J Neurosci. 2011;31:11457–11471. doi:10.1523/JNEUROSCI.1384-11.2011
- Yang SR, Hu ZZ, Luo YJ, et al. The rostromedial tegmental nucleus is essential for non-rapid eye movement sleep. Plos Biol. 2018;16:e2002909. doi:10.1371/journal.pbio.2002909
- Salimzade A, Hosseini-Sharifabad A, Rabbani M. Comparative effects of chronic administrations of gabapentin, pregabalin and baclofen on rat memory using object recognition test. Res Pharm Sci. 2017;12:204–210. doi:10.4103/1735-5362.207201
- D’Hooge R, De Deyn PP. Applications of the Morris water maze in the study of learning and memory. Brain Res Brain Res Rev. 2001;36:60–90. doi:10.1016/s0165-0173(01)00067-4
- Stoub TR, Rogalski EJ, Leurgans S, Bennett DA, DeToledo-Morrell L. Rate of entorhinal and hippocampal atrophy in incipient and mild AD: relation to memory function. Neurobiol Aging. 2010;31:1089–1098. doi:10.1016/j.neurobiolaging.2008.08.003
- Becker JA, Hedden T, Carmasin J, et al. Amyloid-β associated cortical thinning in clinically normal elderly. Ann Neurol. 2011;69:1032–1042. doi:10.1002/ana.22333
- Jack CJ, Lowe VJ, Senjem ML, et al. 11C PiB and structural MRI provide complementary information in imaging of Alzheimer’s disease and amnestic mild cognitive impairment. Brain. 2008;131:665–680. doi:10.1093/brain/awm336
- Gengler S, Hamilton A, Hölscher C. Synaptic plasticity in the hippocampus of a APP/PS1 mouse model of Alzheimer’s disease is impaired in old but not young mice. PLoS One. 2010;5:e9764. doi:10.1371/journal.pone.0009764
- Xie L, Kang H, Xu Q, et al. Sleep drives metabolite clearance from the adult brain. Science. 2013;342:373–377. doi:10.1126/science.1241224
- Macedo AC, Balouch S, Tabet N. Is sleep disruption a risk factor for Alzheimer’s disease? J Alzheimers Dis. 2017;58:993–1002. doi:10.3233/JAD-161287
- Prinz PN, Vitaliano PP, Vitiello MV, et al. Sleep, EEG and mental function changes in senile dementia of the Alzheimer’s type. Neurobiol Aging. 1982;3:361–370. doi:10.1016/0197-4580(82)90024-0
- Sprecher KE, Bendlin BB, Racine AM, et al. Amyloid burden is associated with self-reported sleep in nondemented late middle-aged adults. Neurobiol Aging. 2015;36:2568–2576. doi:10.1016/j.neurobiolaging.2015.05.004
- Kang JE, Lim MM, Bateman RJ, et al. Amyloid-beta dynamics are regulated by orexin and the sleep-wake cycle. Science. 2009;326:1005–1007. doi:10.1126/science.1180962
- Holst SC, Bersagliere A, Bachmann V, Berger W, Achermann P, Landolt HP. Dopaminergic role in regulating neurophysiological markers of sleep homeostasis in humans. J Neurosci. 2014;34:566–573. doi:10.1523/JNEUROSCI.4128-13.2014
- Eban-Rothschild A, Rothschild G, Giardino WJ, Jones JR, de Lecea L. VTA dopaminergic neurons regulate ethologically relevant sleep-wake behaviors. Nat Neurosci. 2016;19:1356–1366. doi:10.1038/nn.4377
- Zhao YN, Yan YD, Wang CY, et al. The rostromedial tegmental nucleus: anatomical studies and roles in sleep and substance addictions in rats and mice. Nat Sci Sleep. 2020;12:1215–1223. doi:10.2147/NSS.S278026
- Sun Y, Cao J, Xu C, Liu X, Wang Z, Zhao H. Rostromedial tegmental nucleus-substantia nigra pars compacta circuit mediates aversive and despair behavior in mice. Exp Neurol. 2020;333:113433. doi:10.1016/j.expneurol.2020.113433