Abstract
This review summarizes the literature on mammalian toxicity of ZnO nanoparticles (NPs) published between 2009 and 2011. The toxic effects of ZnO NPs are due to the compound’s solubility. Whether the increased intracellular [Zn2+] is due to the NPs being taken up by cells or to NP dissolution in medium is still unclear. In vivo airway exposure poses an important hazard. Inhalation or instillation of the NPs results in lung inflammation and systemic toxicity. Reactive oxygen species (ROS) generation likely plays an important role in the inflammatory response. The NPs do not, or only to a minimal extent, cross the skin; this also holds for sunburned skin. Intraperitoneal administration induces neurological effects. The NPs show systemic distribution; target organs are liver, spleen, lung, and kidney and, in some cases, the heart. In vitro exposure of BEAS-2B bronchial epithelial cells and A549 alveolar adenocarcinoma cells results in cytotoxicity, increased oxidative stress, increased intracellular [Ca2+], decreased mitochondrial membrane potential, and interleukin (IL)-8 production. Decreased contractility of airway smooth muscle cells poses an additional hazard. In contrast to the results for BEAS-2B and A549 cells, in RKO colon carcinoma cells ZnO NPs and not Zn2+ induce cytotoxicity and mitochondrial dysfunction. Short-term exposure of skin cells results in apoptosis but not in an inflammatory response, while long-term exposure leads to increased ROS generation, decreased mitochondrial activity, and formation of tubular intercellular structures. Macrophages, monocytes, and dendritic cells are affected; exposure results in cytotoxicity, oxidative stress, intracellular Ca2+ flux, decreased mitochondrial membrane potential, and production of IL-1β and chemokine CXCL9. The NPs are phagocytosed by macrophages and dissolved in lysosomes. In vitro the Comet assay and the cytokinesis-blocked micronucleus assay show genotoxicity, whereas the Ames test does not. This is, however, not confirmed by in vivo genotoxicity assays. Protein binding results in increased stability.
Introduction
Zinc oxide nanoparticle (ZnO NP) powders are widely used in cosmetics (sunscreens, foot care, ointments, and over-the-counter topical products), pigments and coatings (ultraviolet [UV] protection, fungicide in paints), electronic devices, and catalysts. While skin exposure is predominantly consumer exposure to final products (sunscreens but also paints), airway exposure is predominantly workplace exposure to NP dusts (factories producing NPs but also paint factories).Citation1
Despite the widespread use of ZnO NPs, the safety of this compound for humans is still unclear. This review summarizes the literature published between 2009 and 2011 on the mammalian toxicity of ZnO NPs. The review discusses hazards resulting from exposure via inhalation and dermal exposure, effects on various toxicological endpoints in vivo, biodistribution, and effects in various cell lines in vitro. In addition, emerging knowledge on the mechanisms underlying the in vivo and in vitro effects is discussed.
In vivo studies
Effects of airway exposure in experimental animals
Theoretical modeling suggests preferential deposition of 10–100 nm NPs in the alveolar and tracheobronchial regions.Citation2 In case of alveolar deposition, alveolar macrophages generally phagocytose particles, and these cells migrate via the mucociliary escalator to the tracheobronchial region where they are coughed up and may be swallowed. If these mechanisms fail, particles can enter the interstitium where they are no longer available for clearance. This may result in chronic stimulation of cells, resulting in inflammation and fibrosis.Citation1
The acidic environment of the lung lining fluid may result in ZnO dissolution, leading to transient increases in the concentration of Zn2+ ionsCitation1 and local toxicity. Inhalation exposure of 3 μm ZnO microparticles (25 and 50 mg/m3) and instillation of 300 nm ZnO NPs (1 and 5 mg/kg body weight [bw]) of rats resulted in transient inflammation measured in the bronchoalveolar lavage (BAL) as increased lactate dehydrogenase (LDH) release, protein content, and neutrophil content.Citation3
Single intratracheal instillation of rats with 50–70 nm ZnO NPs and <1000 nm ZnO microparticles (1 and 5 mg/kg bw) resulted in potent but reversible inflammation (measured in the BAL by increased LDH release, cell number, and neutrophil content). This inflammation was resolved 1 month after instillation.Citation4 Single intratracheal instillation of rats with <10 nm ZnO NPs induced an eosinophilic/fibrotic/granulomatous inflammationCitation5 and recruitment of eosinophils and neutrophils in the BAL.Citation6
Rapid pH-dependent dissolution of ZnO NPs inside of phagosomes is the main cause of ZnO NP-induced lung injury.Citation7 A recently published model proposed that for high-solubility NPs, such as ZnO NPs, the protein corona is digested inside lysosomes due to the low pH and presence of lysosomal enzymes inside this organelle, after which the NPs quickly dissolve resulting in lysosomal destabilization by Zn2+.Citation8 In the mouse, iron doping of 20 nm ZnO NPs showed reduced lung toxicity (neutrophil counts and interleukin [IL]-6 expression) and reduced heme oxygenase (HO) expression compared to nondoped NPs.Citation9 Also in the rat reduced lung inflammation was seen after exposure to iron-doped NPs.
A recent study has shown that ZnO (and CuO) NPs form a separate group within the family of metal oxide NPs due to the compound’s high solubilityCitation10 Whereas for most metal oxide NPs tested in vivo and in vitro toxicity could be predicted by their band-gap energy ZnO (and CuO) NP toxicity is determined by its solubility. In fact, the first decision in the proposed strategy for toxicity prediction is based on whether the solubility of the metal oxide NP is above a certain threshold (as is the case for ZnO NPs); the second decision is based on whether the band-gap energy overlaps with the cellular redox potential.
Inhalation of 20 nm ZnO NPs (2.5 mg/kg bw) by rats twice daily for 3 days resulted in an increased Zn content in the liver after 12 hours and in the kidneys after 36 hours. Histopathology revealed damage in liver and lung tissues.Citation11
Zn2+, which is present due to the solubility of ZnO NPs, seems to be responsible for inducing inflammatory responses and necrosis.Citation12 A higher cytosolic [Zn2+] was found in BAL cells and white blood cells from rats after 38 nm ZnO NP inhalation.Citation13 The capacity of ZnO NPs to generate reactive oxygen species (ROS) in vitro seems to correlate with their potential to induce cellular inflammation in vivo.Citation12,Citation14
While generally metal oxide NPs are trapped in the lung mucus by adhesive interaction, a small fraction of <50 nm ZnO NPs could penetrate the airway mucus.Citation15 Inhalation and instillation of ZnO NPs result in lung inflammation and systemic toxicity. The solubility of ZnO NPs plays an important role in this inflammation. Dissolution in phagosomes is believed to be the main cause of inflammation. However, there are some indications that ZnO NPs may also show dissolution in lung lining fluid (surfactant). Airway exposure of ZnO NPs results in increased intracellular [Zn2+]. ROS generation is likely to play an important role in the inflammatory response. The lung mucus is able to trap most metal oxide NPs but not (completely) ZnO NPs.
Airway exposure to ZnO NPs poses an important hazard and there is a need for additional studies aimed at risk assessment and determination of no-observed-adverse-effect-levels.
Remaining questions are:
Can (some of) the effects seen be explained by acute high-dose exposure? Would chronic low-dose exposure provide more useful information, especially considering the transient effects seen in acute exposure studies?
Are instillation studies adequate to pinpoint effects specifically to the nano size of ZnO? The conditions of instillation exposure favor aggregate formation.
Are the effects of airway exposure to ZnO (be it nano- or micro-sized) different from Zn2+ itself?
Effects of dermal exposure of humans and experimental animals
The presence of ZnO (and TiO2) NPs in sunscreens implicates a major source of exposure since many people use sunscreens for many hours a day for weeks or months. Therefore, much attention is paid to the question of whether ZnO NPs in sunscreens are able to cross the skin barrier.
ZnO (and TiO2) have intrinsic UV-absorbing properties and are therefore used in sunscreens as UV blockers. In normal pigment size ranges (200–400 nm for ZnO), these particles reflect and scatter light, making the sunscreens appear white. However, 40–100 nm ZnO NPs absorb and scatter UV radiation and largely absorb visible wavelengths, making the sunscreens appear transparent on the skin.
ZnO (and TiO2) are semiconductors, and therefore part (~10%) of the absorbed UV radiation can generate free radicals on the surface of metal oxides in the presence of water. This photocatalytic activity increases with decreasing NP size. Coating or doping is used to reduce the metal oxide semiconductor activity when used in sunscreens.Citation1
Most studies on healthy human skin support the view that ZnO NPs in sunscreens do not penetrate beyond the stratum corneum.Citation16–Citation18 To distinguish Zn in ZnO particles from the body’s own Zn, 68Zn was used to prepare ZnO particles.Citation19 Two types of particles were used 19 nm NPs and > 100 nm micro-sized particles. After application of sunscreen containing one of these two types of particles on the skin of healthy human volunteers for 5 days, approximately 0.1% of all Zn in the blood was 68Zn. Application of the NPs resulted in a higher amount of 68Zn in blood and urine compared with the micro-sized particles.
Pigs were UV-irradiated resulting in moderate sunburn. ZnO NPs (140 nm) penetrated only one to two layers of the stratum corneum of sunburned pig skin.Citation20 ZnO NPs did pass the stratum corneum of the skin of hairless mice. This model is, however, considered to be of questionable relevance because the skin of mice is much more penetrable than human skin.Citation1 Moreover, application of sunscreen containing realistic doses of 20 nm ZnO NPs to rats for 28 days (five times a week) resulted in collagen loss.Citation21 This collagen loss was explained by the authors as being caused by skin passage of the ZnO NPs at the site of application due to the rat skin being thin.
It may be concluded that ZnO NPs do not cross the skin, or only do so to a small extent. This is also the case for sunburned skin. Rodents do not seem to be suitable animal models in the study of skin penetration.
Remaining questions are:
What is the risk in the case of damaged skin?Citation18,Citation22
Does long-term exposure result in skin penetration?Citation1 Studies have so far only addressed acute or short-term exposures.
Is (the small amount of) Zn that has been absorbed through the skin absorbed as ZnO NPs or as Zn2+ ions?Citation1,Citation19
Neurological effects in experimental animals
Few studies have investigated neurotoxicity and effects on cognition of ZnO NPs. Spatial learning and memory ability were attenuated by alteration of synaptic plasticity in rats after intraperitoneal administration of 20–80 nm ZnO NPs (4 mg/kg bw) twice weekly for 8 weeks.Citation23 This is a single study and should be complemented by other studies, also taking more relevant exposure routes into account.
Biodistribution in experimental animals
When using intravenous administration, absorption is bypassed and other aspects of toxicokinetics, such as tissue distribution and elimination, can be studied with more precision.
Because of the abundance of Zn in the body, radioactive ZnO NPs were used for biodistribution studies in mice.Citation24 Radioactive ZnO NPs did not differ from the original ZnO NPs in zeta potential and particulate form (40–100 nm). Intravenous injection resulted in primary retention in the lung (43.6% of the injected dose per g wet weight, 1 hour after administration) and subsequent translocation to the gastrointestinal tract for feces excretion.
Single intraperitoneal administration of 100 nm ZnO NPs (2.5 g/kg bw) resulted in accumulation in liver, spleen, lung, kidney, and heart.Citation25 The Zn concentration in the liver, spleen, and lung was higher after NP administration than after administration of similar amounts of 1 μm ZnO particles.
Oral administration of 100 nm ZnO NPs (2.5 g/kg bw) resulted in accumulation in the liver, spleen, lung, and kidney. In contrast to intraperitoneal administration, ZnO NPs did not accumulate in the heart.Citation25 The Zn concentration in the liver, spleen, and kidney was higher after NP administration than after administration of similar amounts of 1 μm ZnO particles. Oral NP administration resulted in transient histopathology of the liver that was not seen after administration of 1 μm ZnO particles. Nano- and micro-sized ZnO show systemic distribution, also after oral uptake, with a higher absorption and stronger toxic effect of the nano-sized particles. Liver, spleen, lung, and kidney and in some cases, the heart are target organs.
In vitro studies
General observations
In vitro studies using ZnO NPs often show a small concentration range in which cell viability is reduced from almost 100% to almost zero, ie, a steep concentration-viability curve. It is assumed that this is caused by the solubility of ZnO, resulting in free intracellular Zn2+. Zn is a component of many enzymes (eg, alcohol dehydrogenase, matrix metalloproteinase) and transcription factors (eg, zinc finger protein transcription factors). Disruption of cellular Zn homeostasis in in vitro systems has been linked to loss of viability, oxidative stress, and mitochondrial dysfunction.Citation1,Citation13 Similarly, Deng et al concluded from their study on ZnO NP exposure of neural stem cells that toxicity results from dissolved Zn2+ in the culture medium or inside cells.Citation26 Furthermore, Müller et al showed that pH-triggered intracellular release of ionic Zn2+ is responsible for the toxicity of ZnO nanowires.Citation27
The toxic effects of ZnO NPs are due to their solubility, resulting in increased intracellular [Zn2+]. This results in cytotoxicity, oxidative stress, and mitochondrial dysfunction. An overview of the in vitro studies discussed below is provided in .
Table 1 Overview of in vitro studies using zinc oxide nanoparticles
In vitro studies using the human bronchial epithelial cell line BEAS-2B and the human alveolar adenocarcinoma cell line A549
From the in vivo section of this review we concluded that airway exposure to ZnO NPs poses an important hazard. In line with this, a large part of the in vitro studies reported use cell lines and exposure models derived from the airways. The human bronchial epithelial cell line (BEAS-2B) and human alveolar adenocarcinoma cell line (A549) are the two human cell lines representing the airways that are used most often. They represent two important cell types, bronchial epithelial cells and alveolar epithelial cells.
A cytotoxicity screening approach using four dyes (Hoechst 33342, JC-1, Fluo-4, and propidium iodide), showed induction of the intracellular Ca2+ flux, lowering of the mitochondrial membrane potential, and loss of membrane integrity after exposure of BEAS-2B cells and RAW264.7 murine macrophages to 20 nm ZnO NPs.Citation28 Dissolution of these NPs occurred both in culture medium and in endosomes.Citation29 When these NPs did not dissolve, in BEAS-2B cells they entered caveolae, whereas in RAW264.7 cells they entered lysosomes.Citation29 Iron doping of the NPs reduced the rate of Zn2+ dissolution and thereby reduced cytotoxicity.Citation28
Along a similar line, coating 32–95 nm ZnO NP cores with a TiO2 shell reduced mitochondrial activity (reduction of 3-(4,5-dimethylthiazol-2-yl)-2,5-diphenyltetrazolium bromide [MTT]), membrane damage (LDH release), IL-8 production, and ROS generation in A549 lung epithelial cells.Citation30 Thicker shell NPs were less cytotoxic. The reduced toxicity resulting from the TiO2 coating is presumably because of less interaction of ZnO NPs with the cells and a lower rate of Zn2+ release by the ZnO NPs.
In BEAS-2B cells 20 nm ZnO NPs induced a concentration-and time-dependent cytotoxicity as well as increased LDH release, increased oxidative stress, and increased intracellular [Ca2+].Citation31 The expression of four genes involved in apoptosis and oxidative stress were increased (BNIP, PRDX3, PRNP, and TXRND1).
In primary human bronchial epithelial cells and BEAS-2B cells, IL-8 messenger (m)RNA expression and production was induced by 24–70 nm ZnO NPs.Citation32 This induction was mediated by p65 phosphorylation and IκBα phosphorylation and degradation. The p53 pathway was activated by 22.5 nm ZnO NPs in BEAS-2B cells.Citation33
Aqueous extracts from < 10 nm ZnO NPs affected cytotoxicity, IL-8 production, and activation of the transcription factors AP-1 and nuclear factor (NF)-κB in A549 cells.Citation6 These findings again suggest that Zn2+ is an important mediator of the in vitro effects of ZnO NPs.
ZnO nanorods (6 × 8 nm and 7 × 19 nm) were more toxic to A549 cells than spherical ones (6, 25, and 38 nm) and smaller ones were more toxic to these cells than larger ones.Citation34 The contact area between a single NP and a single cell was more important for toxicity than the total surface area of an NP.
Exposure of A549 cells to 50 × 140 nm ZnO nanorods induced cytotoxicity, ROS generation, oxidative stress, and activation of caspase-3 and caspase-9 in a dose- and time-dependent wayCitation35 Heat-shock protein 70 and p53 were induced. The pro-apoptotic protein bax was upregulated while the antiapoptotic proteins survivin and bcl-2 were downregulated. In conclusion, ZnO nanorods induced apoptosis involving p53, survivin, bax/bcl-2, and caspase pathways.
mRNA expression of IL-8 and HO-1 were different when 24–71 nm ZnO NP-exposed submerged A549 cells were compared with 24–71 nm ZnO NP-exposed air-liquid interface (ALI) A549 cells.Citation36
Xie et al compared 25 nm ZnO NP exposure of submerged and ALI cells but used C10 mouse alveolar type II cells.Citation37 The NPs induced cytotoxicity at a similar dose range in the two exposure systems. The time course of oxidative stress was, however, different, peaking at 2–6 hours in the case of the submerged cells and at 6 hours in the case of the ALI cells.
Apical exposure of a rat alveolar epithelial cell monolayer to 20 nm ZnO NPs resulted in a dose- and time-dependent decrease in transepithelial electrical resistance.Citation38 Mitochondrial activity was decreased, while LDH release and intracellular ROS were increased. Tight junctions were disrupted. The effect was, at least in part, mediated by Zn2+ released from ZnO NPs. Pretreatment with the antioxidant N-acetyl-L-cysteine attenuated injury related to intracellular ROS.
Exposure of BEAS-2B cells results in cytotoxicity, ROS generation, apoptosis, and increased oxidative stress and increased intracellular [Ca2+]. The mitochondrial membrane potential is decreased, and production of IL-8 is induced. The effects on A549 cells seem to be comparable to the effects on BEAS-2B cells. ZnO nanorods appear to be more toxic than spherical ZnO NPs. ZnO nanorods induce apoptosis, but whether spherical ZnO NPs also induce apoptosis was not investigated. ZnO NP toxicity can be reduced by lowering its solubility by doping or coating, resulting in a lower release of Zn2+. The response of A549 cells in ALI differs from the response when these cells are submerged in medium.
In vitro studies using dermal cell lines
ZnO NPs are present in products that are used on the skin, such as sunscreens. For this reason, in vitro studies have investigated exposure effects on skin cells.
ZnO NPs (23.5 nm) induced apoptosis and upregulation of p53 and phospho-p38 in human dermal fibroblasts.Citation39 p53 was phosphorylated at the SerCitation33 and SerCitation46 sites, which are known to be phosphorylated by p38. Together this suggests a role of the p53–p38 pathway in apoptosis induction.
HaCaT human keratinocytes and SK Mel-28 human melanoma cells were exposed to 8–10 nm ZnO NPs and <44 μm ZnO particles.Citation40 Gene profiling revealed changes in metal metabolism, chaperonin proteins, and protein folding genes but did not show a proinflammatory signature.
Exposure of NCTC2544 human keratinocytes to <100 nm ZnO NPs (10 μg/mL) for 3 months induced tubular intercellular structures.Citation41 The NPs were only found inside vesicles within the cytoplasm, particularly in early and late endosomes and amphisomes. Moreover, exposure resulted in ROS generation, decreased mitochondrial activity, loss of normal cell morphology, and a disturbed cell-cycle distribution. ZnO NPs (15 nm) did not activate the inflammasome in primary human keratinocytes.Citation42
The p53 pathway was activated by 22.5 nm ZnO NPs in BJ human neonatal foreskin cells.Citation33 After p53 knock-down, the cells became more resistant to NP-induced cell death, and they increased cell-cycle progression.
ZnO NPs do not (or only slightly) penetrate the human skin, but they do affect skin cells in vitro. Short-term exposure results in apoptosis but not in an inflammatory response, while long-term exposure shows increased ROS generation, decreased mitochondrial activity, and the formation of tubular intercellular structures.
In vitro studies using colon cell lines
ZnO NPs are present in products that may be ingested, such as tooth paste. For this reason, in vitro studies have investigated exposure effects on colon cell lines as representative of the digestive tract.
Cytotoxicity of 8–10 nm ZnO NPs and <44 μm ZnO microparticles to RKO human colon carcinoma cells was dependent on direct particle-cell contact and independent of the Zn2+ concentration in cell culture medium.Citation43 The NPs showed a higher cytotoxic potency per unit mass. ZnO particles induced annexin V staining. ZnO particles but not Zn2+-induced mitochondrial dysfunction (measured by JC-1 staining).
RKO and Caco-2 human colon carcinoma cells were exposed to 8–10 nm ZnO NPs and <44 μm ZnO microparticles.Citation40 Similar to the results described above for HaCaT and SK Mel-28 cells, gene profiling revealed changes in metal metabolism, chaperonin proteins, and protein folding genes but did not show a pro-inflammatory signature.
Exposure of the LoVo human colon carcinoma cell line to 50–70 nm ZnO NPs resulted in decreased viability, increased H2O2/OH·, decreased O2−· and glutathione, depolarization of the inner mitochondrial membrane, apoptosis, and IL-8 release.Citation44 Together these results show that oxidative stress is a key route in inducing cytotoxicity.
The results for RKO cells seem to contradict the abovementioned results for BEAS-2B and A549 cells in that, for RKO cells, ZnO NPs but not Zn2+ induced cytotoxicity and mitochondrial dysfunction. Moreover, for RKO cells, gene profiling did not suggest an inflammatory response, a response that was observed for BEAS-2B and A549 cells.
In vitro studies using cells of the immune system
Particulate matter, such as pathogens but also NPs, are readily ingested by monocytes/macrophages and dendritic cells. This may pose a hazard for these cell types, thereby reducing the capacity to mount a (functional) immune response.
Monocytes are more sensitive to 4–20 nm ZnO NP cytotoxicity than lymphocytes.Citation45 In both cell types, cytotoxicity is mediated by oxidative stress. Cytotoxicity and ROS generation increased with decreasing NP size. Interferon (IFN)-γ, tumor necrosis factor (TNF)-α, and IL-12 production were induced by these NPs in peripheral blood mononuclear cells.
Expression of IL-1 β and chemokine CXCL9 was induced by 20 nm ZnO NPs in murine bone marrow-derived dendritic cells and RAW264.7 murine macrophages.Citation46 In RAW264.7 cells, 20 nm ZnO NPs resulted in induction of the intracellular Ca2+ flux, lowering of the mitochondrial membrane potential, and loss of membrane integrityCitation28 In addition, in these cells, 22.5 nm ZnO NPs induced the p53 pathway.Citation33
ZnO NPs (10–30, 30, and 100 nm) but also <1 μm ZnO microparticles induced cytotoxicity in Ana-1 murine macrophages.Citation47 The [Zn2+] that induced 50% viability in cells exposed to nano- and micro-sized ZnO was close to the [Zn2+] that induced 50% viability in ZnCl2-treated cells. Moreover, the [Zn2+] in supernatants from cells exposed to nano- and micro-sized ZnO correlated with viability and LDH release. Together this suggests an important role of Zn2+ in the cytotoxicity induced by nano- and micro-sized ZnO.
ZnO NPs (15 nm) do not activate the inflammasome in THP-1 human macrophages.Citation42 ZnO NPs clearly affect macrophages, monocytes, and dendritic cells. Exposure results in LDH release, oxidative stress, intracellular Ca2+ flux, lowering of the mitochondrial membrane potential, and production of IL-1ß and CXCL9. It is likely that the p53 pathway plays a role in (some of) these processes. ZnO nanowires were taken up by macrophages through phagocytosis and dissolved in lysosomes.Citation27 Again, the effects of ZnO NPs are likely caused by Zn2+.
In vitro studies using other cell lines
In Jurkat human leukemia cells and H1355 human lung carcinoma cells, <50 nm ZnO NP exposure resulted in a rise in cytosolic and mitochondrial [Zn2+].Citation13 In H1355 cells, these NPs induced LDH release, depolarization of the mitochondrial membrane potential, and caspase-3 activation.
Exposure of Jurkat cells to 15.5 nm ZnO NPs resulted in caspase-independent apoptosis that was also independent of ROS formation.Citation48 This effect was caused by Zn2+ that was formed by extracellular dissolution of ZnO NPs. Coating the NP with mercaptopropyl trimethoxysilane-SiO2 or methoxyl clearly reduced cell death.
Exposure of human cardiac microvascular endothelial cells to 45 nm ZnO NPs induced a concentration- and time-dependent cytotoxicity, measured by MTT reduction and LDH leakage.Citation49 In addition, cell permeability was affected (measured by the flux through endothelial monolayers of bovine serum albumin [BSA]-fluorescein isothiocyanate conjugate), and an inflammatory response was induced (measured by ROS generation and increased mRNA expression of vascular cell adhesion molecule 1, intercellular adhesion molecule 1, chemokine CCL2, and IL-8).
Zn2+ released from 90 × 25 nm ZnO nanorods induced cytotoxicity in NIH 3T3 murine fibroblasts.Citation50 ZnO NPs (47–106 nm) induced cytotoxicity and ROS generation in HepG2 human hepatocellular carcinoma cells.Citation51 ZnO NPs (uncoated 100 nm and triethoxycaprylylsilane-coated 130 nm) were 50% soluble, which could account for their cytotoxicity.Citation52 Moreover, both ZnO NPs (but not other NPs tested) decreased albumin production by C3A human hepatoblastoma cells, suggesting effects on hepatic function.
Nano- and micro-sized ZnO induced cytotoxicity at similar concentrations in IP15 human glomerular mesangial cells.Citation53 The NPs (75 nm) induced ROS generation and reduced glutathione levels in IP15 cells and HK-2 human proximal epithelial cells. In addition, these NPs induced NF-κB translocation in HK-2 cells.
In human embryonic lung fibroblasts, 20, 30, and 40 nm ZnO NPs were cytotoxic.Citation54 In primary mouse embryo fibroblasts, 20 nm ZnO NPs were cytotoxic (measured by MTT reduction, LDH leakage, and the water-soluble tetrazolium [WST] assay) and induced oxidative stress (measured by glutathione depletion, malondialdehyde production, and superoxide dismutase inhibition) as well as ROS generation.Citation55 Exposure of neuro-2A mouse neuroblastoma cells to 50–70 nm ZnO NPs induced cytotoxicity (measured by MTT reduction and LDH release).Citation56 Both 40–100 nm ZnO NPs and 44 μm micro-sized ZnO particles decreased viability and cell contractility of human airway smooth muscle cells.Citation57 It may be speculated that ZnO NPs mediate UV phototoxicity. However, at least in vitro there was no difference in cytotoxicity between UV-irradiated and nonirradiated ZnO NPs.Citation18
Similar to the observations reported above in sections on in vitro studies, cytotoxicity, oxidative stress, mitochondrial dysfunction, apoptosis, and an inflammatory response were reported. ZnO NPs decrease cell contractility of airway smooth muscle cells, posing an additional adverse effect on airway cells. This single study should be complemented with additional ones. ZnO NPs do not mediate UV phototoxicity.
Genotoxicity studies in vivo and in vitro
In vivo studies
Triethoxycaprylylsilane-coated ZnO NPs (30–200 nm) did not induce genotoxicity in lung cells from rats exposed by inhalation, as determined by the mouse bone marrow micronucleus test.Citation58 Although ZnO NPs (60–200 nm) showed some clastogenic activity in vitro in mammalian cells, there was no indication for clastogenic potential or aneugenic activity in vivo.Citation20
In vitro studies
Dimethoxydiphenylsilane/triethoxycaprylylsilane crosspolymer-coated ZnO NPs (30–200 nm) did not induce genotoxicity in vitro, established by the Salmonella reverse mutation (Ames) test.Citation58 ZnO NPs (<100 nm) were negative in the Ames test up to 1000 μg/mL in the absence of S9 metabolic activation and induced only marginal mutagenesis in Escherichia coli WP2 trp uvrA in the presence of 9% S9 fraction.Citation59 Tetramethylammonium hydroxide-coated ZnO NPs (size before coating was reported to be 5.4 nm) were negative in the Ames test using Salmonella typhimurium strains TA98, TA100, TA1535, and TA1537 and E. coli strain WP2uvrA(−), with and without metabolic activation using S9 pre-incubation.Citation60
ZnO NPs (<100 nm) induced genotoxicity in HEp-2 human cervix carcinoma cells, using the Comet assay and the cytokinesis-blocked micronucleus assay.Citation61 Genotoxicity was associated with increased tyrosine phosphorylation.
ZnO NPs (<100 nm) were internalized in primary human epidermal keratinocytes after 6 hours exposure.Citation62 Inhibition of mitochondrial activity was seen as well as induction of DNA damage as evident from the comet assay; in the A431 human epidermal cell line, these NPs induced DNA damage.Citation63 In the latter study, oxidative stress was also found to be induced by these NPs, as evidenced by glutathione depletion and reduction of catalase and superoxide dismutase activity.
In Caco-2 cells, 10 nm ZnO NPs induced cytotoxicity (measured by the WST assay and LDH release) and DNA strand breakage and oxidative DNA damage (established using the Fpg-comet assay).Citation64
Repetitive exposure to 76 × 53 nm ZnO NPs (5 μg/mL) of human nasal mucosa mini-organ cultures induced DNA damage, established using the comet assay.Citation65 This damage was further increased after a 24-hour regeneration period. The NPs were distributed in the cytoplasm and the nucleus.
Coating 30 nm ZnO NPs with poly methyl acrylic acid (PMAA) reduced cytotoxicity and ROS generation in WIL2-NS human lymphoblastoid cells.Citation66 PMAA coating did, however, significantly increase genotoxicity compared to the uncoated NPs, established by the cytokinesis-blocked micronucleus assay.Citation67
In vivo genotoxicity assays did not show any effects. In vitro, the Ames test failed to show genotoxicity, but the relevance of the bacterial mutation assay for NPs may be limited. However, in vitro both the Comet assay and the cytokinesis-blocked micronucleus assay showed induction of DNA damage and genotoxicity. This may suggest that the type of assay and whether testing was done in vitro or in vivo affects the outcome of the test.
Protein binding
NPs are covered by proteins in vitro in cell culture medium and in cells but also in vivo. The identity of the proteins that bind to the NPs depends, next to the NP itself, on the environment (ie, cell culture medium, blood) and on the time in which the NPs are present in that specific environment.
Ultrasonication is the most effective way for disaggregating NPs in water. ZnO NPs (20 nm) could not remain stable in suspensions in water, which is presumably due to the high Zn2+ concentration resulting in enhanced aggregation.Citation68 Adsorption of BSA to 20 nm ZnO NPs led to deagglomeration.Citation69 The presence of BSA results in enhanced stability.
ZnO NPs (30 nm) are able to bind proteins with important biological functions, including immunoglobulins, lipoproteins, albumin, α-1-antichymotrypsin, α-2-macroglobulin, and transferrin.Citation70 Polymethylsilsesquioxane-coated ZnO NPs did not bind any plasma proteins.
Proteins adhering to ZnO NPs result in enhanced stability of the NPs. The proteins that are bound by ZnO NPs are physiologically important.
General conclusions and recommendations
The in vivo results indicate that ZnO NP exposure via inhalation poses the most important hazard, while for skin exposure hazard can be considered rather minimal in view of the limited uptake via the skin and the absence of local effects. Risk assessment (exposure assessment, dose response relationships) of ZnO NP exposure is almost lacking; risk assessment of inhalation exposure is needed urgently. Exposure of rats to 2.4 mg/m3 35 nm ZnO NPs for 6 hours resulted in an increased neutrophil number in the BAL 24 hours later.Citation71 Moreover, the number of white blood cells was increased. Earlier studies have shown that exposure of rats to 1 mg/m3 60 nm ZnO NPs for 3 hours resulted in a fivefold increase in HO mRNA expression; exposure to 2.5 and 5 mg/m3 resulted in increased mRNA expression of HO and metallothionein.Citation72 Exposure of guinea pigs to 2.3 mg/m3 50 nm ZnO NPs for 2 days (3 hours/day) resulted in an increased neutrophil number, LDH release, and alkaline phosphatase activity in the BAL, while exposure to 1.1 mg/m3 resulted in an increased neutrophil number and acid phosphatase activity in the BAL.Citation73,Citation74 Exposure of guinea pigs to 4.6 mg/m3 50 nm ZnO NPs for 6 days (3 hours/day) resulted in functional and morphological changes in the lung.Citation75 Importantly, metal fume fever developed in workers exposed to 2.5 mg/m3 for 2 hours.Citation76 Together these data suggest that the current exposure standard of 5 mg/m3 may not be adequate to protect workers from acute lung inflammation.
Various cell types (representing, among others, airways, skin, intestines, and the immune system) are affected in vitro by ZnO NPs. Most studies suggest that these effects are caused by Zn2+ that results from NP dissolution outside the cell. One study suggests that NPs are taken up by the cell, after which dissolution takes place inside the cell. The in vitro results indicate that induction of oxidative stress is the most important or most likely mechanism underlying ZnO NP toxicity. The putative mechanisms underlying the in vitro effects of ZnO NPs are outlined in .
Figure 1 Putative mechanisms underlying the in vitro effects of ZnO NPs.
Abbreviations: L, lysosome; N, nucleus; NPs, nanoparticles; ROS, reactive oxygen species.
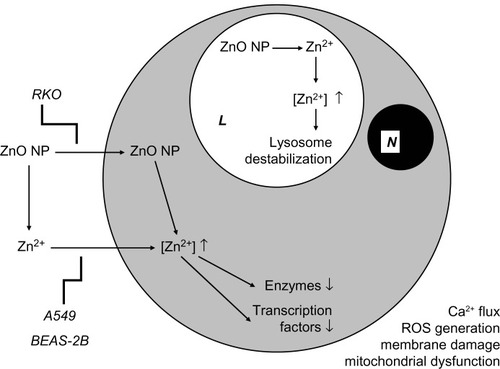
After systemic exposure neurological effects may occur. Additional studies are needed regarding potential neurological responses after systemic ZnO NP exposure.
So far, genotoxicity was only observed in in vitro studies, while in vivo studies were negative. Additional studies are needed before definitive conclusions on the genotoxicity of ZnO NPs can be made.
Acknowledgments
Prof Henk van Loveren and Dr Margriet Park are acknowledged for critical review of the manuscript. This review was supported by The Netherlands Food and Consumer Product Safety Authority (V340370).
Disclosure
The authors report no conflicts of interest in this work.
References
- OsmondMJMcCallMJZinc oxide nanoparticles in modern sunscreens: an analysis of potential exposure and hazardNanotoxicology201041154120795900
- WitschgerOFabrièsJFParticules ultra-fines et santé au travail: 1 – Caractéristiques et effets potentiels sur la santé [Ultrafine particles and occupational health: 1 – Characteristics and potential health effects]Cahiers de notes documentaires20051992135 French
- WarheitDBSayesCMReedKLNanoscale and fine zinc oxide particles: can in vitro assays accurately forecast lung hazards following inhalation exposures?Environ Sci Technol200943207939794519921917
- SayesCMReedKLWarheitDBAssessing toxicity of fine and nanoparticles: comparing in vitro measurements to in vivo pulmonary toxicity profilesToxicol Sci200797116318017301066
- ChoWSDuffinRPolandCAMetal oxide nanoparticles induce unique inflammatory footprints in the lung: important implications for nanoparticle testingEnviron Health Perspect2010118121699170620729176
- ChoWSDuffinRPolandCADifferential pro-inflammatory effects of metal oxide nanoparticles and their soluble ions in vitro and in vivo; zinc and copper nanoparticles, but not their ions, recruit eosinophils to the lungsNanotoxicology201261223521332300
- ChoWSDuffinRHowieSEProgressive severe lung injury by zinc oxide nanoparticles; the role of Zn2+ dissolution inside lysosomesPart Fibre Toxicol201182721896169
- ChoWSDuffinRThielbeerFZeta potential and solubility to toxic ions as mechanisms of lung inflammation caused by metal/metal oxide nanoparticlesToxicol Sci2012126246947722240982
- XiaTZhaoYSagerTDecreased dissolution of ZnO by iron doping yields nanoparticles with reduced toxicity in the rodent lung and zebrafsh embryosACS Nano2011521223123521250651
- ZhangHJiZXiaTUse of metal oxide nanoparticle band gap to develop a predictive paradigm for oxidative stress and acute pulmonary inflammationACS Nano2012654349436822502734
- WangLWangLDingWZhangFAcute toxicity of ferric oxide and zinc oxide nanoparticles in ratsJ Nanosci Nanotechnol201010128617862421121374
- LandsiedelRMa-HockLKrollATesting metal-oxide nanomaterials for human safetyAdv Mater201022242601262720512811
- KaoYYChenYCChengTJChiungYMLiuPSZinc oxide nanoparticles interfere with zinc ion homeostasis to cause cytotoxicityToxicol Sci2012125246247222112499
- AyresJGBormPCasseeFREvaluating the toxicity of airborne particulate matter and nanoparticles by measuring oxidative stress potential – a workshop report and consensus statementInhal Toxicol2008201759918236225
- JachakALaiSKHidaKTransport of metal oxide nanoparticles and single-walled carbon nanotubes in human mucusNanotoxicology7292011 Epub ahead of print
- CrossSEInnesBRobertsMSTsuzukiTRobertsonTAMcCormickPHuman skin penetration of sunscreen nanoparticles: in-vitro assessment of a novel micronized zinc oxide formulationSkin Pharmacol Physiol200720314815417230054
- FilipePSilvaJNSilvaRStratum corneum is an effective barrier to TiO2 and ZnO nanoparticles percutaneous absorptionSkin Pharmacol Physiol200922526627519690452
- SchillingKBradfordBCastelliDHuman safety review of “nano” titanium dioxide and zinc oxidePhotochem Photobiol Sci20109449550920354643
- GulsonBMcCallMKorschMSmall amounts of zinc from zinc oxide particles in sunscreens applied outdoors are absorbed through human skinToxicol Sci2010118114014920705894
- Monteiro-RiviereNAWienchKLandsiedelRSchulteSInmanAORiviereJESafety evaluation of sunscreen formulations containing titanium dioxide and zinc oxide nanoparticles in UVB sunburned skin: an in vitro and in vivo studyToxicol Sci2011123126428021642632
- SurekhaPKishoreASSrinivasARepeated dose dermal toxicity study of nano zinc oxide with Sprague-Dawley ratsCutan Ocul Toxicol2012311263221830917
- NewmanMDStotlandMEllisJIThe safety of nanosized particles in titanium dioxide- and zinc oxide-based sunscreensJ Am Acad Dermatol200961468569219646780
- HanDTianYZhangTRenGYangZNano-zinc oxide damages spatial cognition capability via over-enhanced long-term potentiation in hippocampus of Wistar ratsInt J Nanomedicine201161453146121796247
- ChenJKShihMHPeirJJThe use of radioactive zinc oxide nanoparticles in determination of their tissue concentrations following intravenous administration in miceAnalyst201013571742174620505857
- LiCHShenCCChengYWOrgan biodistribution, clearance, and genotoxicity of orally administered zinc oxide nanoparticles in miceNanotoxicology9272011 Epub ahead of print
- DengXLuanQChenWNanosized zinc oxide particles induce neural stem cell apoptosisNanotechnology2009201111510119420431
- MüllerKHKulkarniJMotskinMpH-dependent toxicity of high aspect ratio ZnO nanowires in macrophages due to intracellular dissolutionACS Nano20104116767677920949917
- GeorgeSPokhrelSXiaTUse of a rapid cytotoxicity screening approach to engineer a safer zinc oxide nanoparticle through iron dopingACS Nano201041152920043640
- XiaTKovochichMLiongMComparison of the mechanism of toxicity of zinc oxide and cerium oxide nanoparticles based on dissolution and oxidative stress propertiesACS Nano20082102121213419206459
- HsiaoILHuangYJTitanium oxide shell coatings decrease the cytotoxicity of ZnO nanoparticlesChem Res Toxicol201124330331321341804
- HuangCCAronstamRSChenDRHuangYWOxidative stress, calcium homeostasis, and altered gene expression in human lung epithelial cells exposed to ZnO nanoparticlesToxicol In Vitro2010241455519755143
- WuWSametJMPedenDBBrombergPAPhosphorylation of p65 is required for zinc oxide nanoparticle-induced interleukin 8 expression in human bronchial epithelial cellsEnviron Health Perspect2010118798298720194077
- NgKWKhooSPHengBCThe role of the tumor suppressor p53 pathway in the cellular DNA damage response to zinc oxide nanoparticlesBiomaterials201132328218822521807406
- HsiaoILHuangYJEffects of various physicochemical characteristics on the toxicities of ZnO and TiO(2) nanoparticles toward human lung epithelial cellsSci Total Environ201140971219122821255821
- AhamedMAkhtarMJRajaMZnO nanorod-induced apoptosis in human alveolar adenocarcinoma cells via p53, survivin and bax/bcl-2 pathways: role of oxidative stressNanomedicine20117690491321664489
- LenzAGKargELentnerBA dose-controlled system for air-liquid interface cell exposure and application to zinc oxide nanoparticlesPart Fibre Toxicol200963220015351
- XieYWilliamsNGTolicAAerosolized ZnO nanoparticles induce toxicity in alveolar type II epithelial cells at the air-liquid interfaceToxicol Sci2012125245046121964423
- KimYHFazlollahiFKennedyIMAlveolar epithelial cell injury due to zinc oxide nanoparticle exposureAm J Respir Crit Care Med2010182111398140920639441
- MeyerKRajanahalliPAhamedMRoweJJHongYZnO nanoparticles induce apoptosis in human dermal fibroblasts via p53 and p38 pathwaysToxicol In Vitro20112581721172621903158
- MoosPJOlszewskiKHoneggarMResponses of human cells to ZnO nanoparticles: a gene transcription studyMetallomics20113111199121121769377
- KocbekPTeskacKKreftMEKristlJToxicological aspects of long-term treatment of keratinocytes with ZnO and TiO2 nanoparticlesSmall20106171908191720677183
- YazdiASGuardaGRiteauNNanoparticles activate the NLR pyrin domain containing 3 (Nlrp3) inflammasome and cause pulmonary inflammation through release of IL-1α and IL-1βProc Natl Acad Sci U S A201010745194491945420974980
- MoosPJChungKWoessnerDHoneggarMCutlerNSVeranthJMZnO particulate matter requires cell contact for toxicity in human colon cancer cellsChem Res Toxicol201023473373920155942
- De BerardisBCivitelliGCondelloMExposure to ZnO nanoparticles induces oxidative stress and cytotoxicity in human colon carcinoma cellsToxicol Appl Pharmacol20102463116127
- HanleyCThurberAHannaCPunnooseAZhangJWingettDGThe influences of cell type and ZnO nanoparticle size on immune cell cytotoxicity and cytokine inductionNanoscale Res Lett20094121409142020652105
- PalomäkiJKarisolaPPylkkänenLSavolainenKAleniusHEngineered nanomaterials cause cytotoxicity and activation on mouse antigen presenting cellsToxicology20102671–312513119897006
- SongWZhangJGuoJRole of the dissolved zinc ion and reactive oxygen species in cytotoxicity of ZnO nanoparticlesToxicol Lett2010199338939720934491
- Buerki-ThurnherrTXiaoLDienerLIn vitro mechanistic study towards a better understanding of ZnO nanoparticle toxicityNanotoxicology3202012 Epub ahead of print
- SunJWangSZhaoDHunFHWengLLiuHCytotoxicity, permeability, and inflammation of metal oxide nanoparticles in human cardiac microvascular endothelial cells: cytotoxicity, permeability, and inflammation of metal oxide nanoparticlesCell Biol Toxicol201127533334221681618
- YangSTLiuJHWangJCytotoxicity of zinc oxide nanoparticles: importance of microenvironmentJ Nanosci Nanotechnol201010128638864521121377
- WangYAkerWGHwangHMYedjouCGYuHTchounwouPBA study of the mechanism of in vitro cytotoxicity of metal oxide nanoparticles using catfish primary hepatocytes and human HepG2 cellsSci Total Environ2011409224753476221851965
- KermanizadehAPojanaGGaiserBKIn vitro assessment of engineered nanomaterials using a hepatocyte cell line: cytotoxicity, proinflammatory cytokines and functional markersNanotoxicology1202012 Epub ahead of print
- PujaltéIPassagneIBrouillaudBCytotoxicity and oxidative stress induced by different metallic nanoparticles on human kidney cellsPart Fibre Toxicol201181021371295
- YuanJHChenYZhaHXDetermination, characterization and cytotoxicity on HELF cells of ZnO nanoparticlesColloids Surf B Biointerfaces201076114515019926459
- YangHLiuCYangDZhangHXiZComparative study of cytotoxicity, oxidative stress and genotoxicity induced by four typical nanomaterials: the role of particle size, shape and compositionJ Appl Toxicol2009291697818756589
- JengHASwansonJToxicity of metal oxide nanoparticles in mammalian cellsJ Environ Sci Health A Tox Hazard Subst Environ Eng200641122699271117114101
- BerntsenPParkCYRothen-RutishauserBBiomechanical effects of environmental and engineered particles on human airway smooth muscle cellsJ R Soc Interface20107Suppl 3S331S34020356875
- LandsiedelRMa-HockLVan RavenzwaayBGene toxicity studies on titanium dioxide and zinc oxide nanomaterials used for UV-protection in cosmetic formulationsNanotoxicology2010436438120925445
- PanXReddingJEWileyPAWenLMcConnellJSZhangBMutagenicity evaluation of metal oxide nanoparticles by the bacterial reverse mutation assayChemosphere201079111311620106502
- YoshidaRKitamuraDMaenosonoSMutagenicity of water-soluble ZnO nanoparticles in Ames testJ Toxicol Sci200934111912219182441
- OsmanIFBaumgartnerACemeliEFletcherJNAndersonDGenotoxicity and cytotoxicity of zinc oxide and titanium dioxide in HEp-2 cellsNanomedicine (Lond)2010581193120321039197
- SharmaVSinghSKAndersonDTobinDJDhawanAZinc oxide nanoparticles induced genotoxicity in primary human epidermal keratinocytesJ Nanosci Nanotechnol20111153782378821780369
- SharmaVShuklaRKSaxenaNParmarDDasMDhawanADNA damaging potential of zinc oxide nanoparticles in human epidermal cellsToxicol Lett2009185321121819382294
- GerloffKAlbrechtCBootsAWFörsterISchinsRPCytotoxicity and oxidative DNA damage by nanoparticles in human intestinal Caco-2 cellsNanotoxicology200934355364
- HackenbergSZimmermannFZScherzedARepetitive exposure to zinc oxide nanoparticles induces DNA damage in human nasal mucosa mini organ culturesEnviron Mol Mutagen201152758258921786336
- YinHCaseyPSMcCallMJSurface modifications of ZnO nanoparticles and their cytotoxicityJ Nanosci Nanotechnol201010117565757021137983
- YinHCaseyPSMcCallMJFenechMEffects of surface chemistry on cytotoxicity, genotoxicity, and the generation of reactive oxygen species induced by ZnO nanoparticlesLangmuir20102619153991540820809599
- TsoCPZhungCMShihYHTsengYMWuSCDoongRAStability of metal oxide nanoparticles in aqueous solutionsWater Sci Technol201061112713320057098
- TantraRTompkinsJQuinceyPCharacterisation of the deagglomeration effects of bovine serum albumin on nanoparticles in aqueous suspensionColloids Surf B Biointerfaces201075127528119775871
- DengZJMortimerGSchillerTMusumeciAMartinDMinchinRFDifferential plasma protein binding to metal oxide nanoparticlesNanotechnology2009204545510119822937
- HoMWuKYCheinHMChenLCChengTJPulmonary toxicity of inhaled nanoscale and fine zinc oxide particles: mass and surface area as an exposure metricInhal Toxicol2011231494795622122307
- CosmaGFultonHDeFeoTGordonTRat lung metallothionein and heme oxygenase gene expression following ozone and zinc oxide exposureToxicol Appl Pharmacol1992117175801440616
- ConnerMWFloodWHRogersAEAmdurMOLung injury in guinea pigs caused by multiple exposures to ultrafine zinc oxide: changes in pulmonary lavage fluidJ Toxicol Environ Health198825157693418745
- ConnerMWFloodWHRogersAEAmdurMOChanges in pulmonary lavage fluid of guinea pigs exposed to ultrafine zinc oxide with adsorbed sulfuric acidJ Toxicol Environ Health19892622232342921780
- LamHFConnerMWRogersAEFitzgeraldSAmdurMOFunctional and morphologic changes in the lungs of guinea pigs exposed to freshly generated ultrafine zinc oxideToxicol Appl Pharmacol198578129384035670
- FineJMGordonTChenLCKinneyPFalconeGBeckettWSMetal fume fever: characterization of clinical and plasma IL-6 responses in controlled human exposures to zinc oxide fume at and below the threshold limit valueJ Occup Environ Med19973987227269273875