Abstract
There is little doubt that nanoparticles offer real and new opportunities in many fields, such as biomedicine and materials science. Such particles are small enough to enter almost all areas of the body, including cells and organelles, potentially leading to new approaches in nanomedicine. Sensors for small molecules of biochemical interest are of critical importance. This review is an attempt to trace the use of nanomaterials in biochemical sensor design. The possibility of using nanoparticles functionalized with antibodies as markers for proteins will be elucidated. Moreover, capabilities and applications for nanoparticles based on gold, silver, magnetic, and semiconductor materials (quantum dots), used in optical (absorbance, luminescence, surface enhanced Raman spectroscopy, surface plasmon resonance), electrochemical, and mass-sensitive sensors will be highlighted. The unique ability of nanosensors to improve the analysis of biochemical fluids is discussed either through considering the use of nanoparticles for in vitro molecular diagnosis, or in the biological/biochemical analysis for in vivo interaction with the human body.
Introduction
There are well-developed methods to synthesize nanomaterials (eg, nanorod,Citation1 nanocube,Citation2 nanoshell,Citation3 which have found real applications in practice. The light-scattering properties of new nanomaterialsCitation4 in different composition, size, and shape have greatly attracted the attention of analysts, so the applications of nanomaterials for analytical purposes have grown dramatically. Because of their unique advantages, nanoparticles (NPs), with colorful light-scattering properties, are comparable to optical probes with various fluorescent dyes. For example, the light-scattering power of a single NP label is in order of magnitude greater than a single fluorescent label, and the light signals generated from these nanomaterials are not prone to photobleaching, even under illumination for a long time. What is more, in light-scattering detection, only a simple instrument is required, compared with a fluorescent system.Citation5
Metal NPs with large diameter (>30 nm) exhibit strong light-scattering in the visible region, which could be used directly for light-scattering labels in biochemical assay. However, small NPs, which apparently do not feature light-scattering, can also be used to sense chemical interactions (eg, antigen–antibody, avidin–biotin, DNA hybridization, and electrostatic attraction), since enhanced light-scattering signals would be produced if these NPs were to aggregate during the interactions. Because the enhanced light-scattering signals from the aggregated species are sufficiently sensitive to monitor NP aggregation, in a simple procedure, biochemical assay based on such light-scattering signals has been widely used in the determination of DNA, proteins, and drugs.Citation5
Immunoassay is important in basic research and clinical diagnostics. Sandwich-type immunoassay, using a primary antibody to capture the analyte, and a labeled, secondary antibody to detect antigen binding, is widely accepted procedure. The aggregation of nanomaterials, especially gold (Au), induced by the immunoreactions offers a new approach for immunoassay,Citation6 using light-scattering detection to obtain high sensitivity. The enhanced light-scattering signals from the AuNP aggregates, induced by the immunoreaction between the apolipoprotein and its AuNP-labeled antibody, have been successfully applied in clinical diagnostics.Citation7 There have been similar reports about light-scattering signals involving such different materials as silver nanoparticles (AgNP) and analytes (eg, fibrinogen and human immunoglobulin G [h-IgG]).Citation8 Interactions between proteins and nanomaterials have been applied in quantitative assays of proteins in biochemistry and clinical diagnostics. Electrostatic attraction between proteins and nanomaterials with opposite charge induces assembly of proteins or nanomaterials, resulting in enhanced light-scattering signals. Various nanomaterials (either metal or nonmetal), functionalized with the opposite charge to the protein, have been used to detect protein quantitatively with ng sensitivity.
This information stimulates our interest to review major research work related to the use of nanoparticles as biochemical sensors, and to highlight the advantages of using this strategy over the common analytical methods.
Nanoparticles in analytical biochemistry
One of the most important functions of nanoparticles is catalysis, especially with noble metal nanoparticles, which have high catalytic activity for many chemical reactions. Because nanomaterials also have good biocompatibility, they are used to immobilize biomolecules for the fabrication of biosensors.
Table 1 Performances of some NADH sensors
Glucose nanosensors
Glucose is a key metabolite for living organisms, especially in the case of patients suffering diabetes. Since the first enzyme electrode was reported in 1962 by Clark and Lyons.Citation9 There has been an increasing demand for the development of new methodologies for a rapid simple, reliable, reproducible, and sensitive determination of glucose. The detection of glucose by electrochemical biosensors is based on the electrochemical oxidation of hydrogen peroxide (H2O2) generated by enzyme-catalyzed oxidation of glucose at anodic potentials (N +0.6 V vs Ag/AgCl).Citation10 The immobilization of glucose oxidase (GOx) on the electrode surface, which is one of the main factors that affects the performance of a glucose biosensor, has received considerable attention in recent years.Citation11 A new amperometric glucose biosensor was constructed based on the immobilization of GOx with cross-linking in a matrix of chitosan (CHIT) on a glassy carbon electrode (GCE), which was modified by layer-by-layer-assembled carbon nanotube (CNT)/CHIT/gold nanoparticle (GNP) multilayer films. With the increasing of CNT/CHIT/GNP layers, the response current to H2O2 changed regularly, and the response current reached a maximum value when the number of CNT/CHIT/GNP layers was 8. The assembly process for the multilayer films was simple to operate. With GOx as an enzyme model, a new glucose biosensor was fabricated. The excellent electocatalytic activity and special structure of the enzyme electrode resulted in a detection limit of 3 × 10−6 M, estimated at a signal-to-noise ratio of 3, at a fast response time (less than 6 s). Moreover, it exhibited good reproducibility and stability. Human plasma samples were assayed in order to demonstrate the practical usage of the biosensor. Fresh plasma sample was first analyzed in the local hospital with the ASCA AGII Chemistry System (Landmark Scientific, Greensboro, NC). The samples were then re-assayed with the CNT/CHIT/GNP)8/GOx/GCE. A plasma sample (0.5 mL) was added into 2 mL of 0.067 M phosphate buffer saline (pH 7.0) and the response was obtained at +0.6 V The contents of glucose in blood could then be calculated from the calibration curve. The results are satisfactory and agree closely with those measured by the biochemical analyzer in the hospital.Citation11
Recently platinum nanoparticles (PtNPs) were deposited on mesoporous carbon material (CMK-3). GOx was immobilized in the resulting Pt nanoparticles/mesoporous carbon (Pt/CMK-3) matrix, and then the mixture was cast on a glassy carbon electrode using gelatin as a binder. The glucose biosensor exhibited excellent current response to glucose after cross-linking with glutaraldehyde. At 0.6 V (vs saturated calomel electrode) the response current was linear to glucose concentration in the range of 0.04–12.2 mM. The response time (time for achieving 95% of the maximum current) was 15 s and the detection limit (signal-to-noise ratio = 3) was 1 μM. The Michaelis–Menten constant (Kmapp) and the maximum current density were 10.8 mM and 908 μAcm−2 respectively. The activation energy of the enzymatic reaction was estimated to be 22.54 kJmol−1.12 The biosensor showed good stability. It achieved the maximum response current at about 52°C and retained 95.1% of its initial response current after being stored for 30 days. In addition, some fabrication and operational parameters for the biosensor were optimized in this work. The biosensor was used to monitor the glucose levels of serum samples after being covered with an extra Nafion film to improve its anti-interferent ability, and satisfactory results were obtained. The interference of some electroactive compounds to the glucose response was examined by Yu et al.Citation12 When the glucose concentration was 5.6 mM, the deviation of response was calculated according to (Ii-Ig)/Ig, where Ii and Ig were the steady-state currents recorded for solutions containing glucose and interferent, or glucose alone. At Pt/CMK-3-GOx-gelatin/GCE, the deviations caused by ascorbic acid (AA) (0.1 mM), p-acetaminophenol (0.05 mM) and uric acid (UA) (0.5 mM) were 0.44%, 4.69%, and 13.2% respectively. This indicates that the biosensor suffersed some influence from coexistents in serum samples. Therefore, 1 μM percentage by weight Nafion was cast on the surface of Pt/CMK-3-GOx-gelatin/GCE to prevent the interference. And then, at Nafion/Pt/CMK-3-GOx-gelatin/GCE, the deviations were in the acceptable range (±10%).Citation12
A silver hexacyanoferrate nanoparticle (AgHCFNP)/CNT modified GCE was fabricated and then successfully used for the simultaneous determination of AA, UA, and dopamine (DA) by cyclic voltammetry (CV). Meissam et alCitation13 reported for the first time a simultaneous determination of AA, DA, and UA using a glassy carbon electrode modified with a mixture of AgHCFNPs and multiwalled carbon nanotubes. Compared with the existing reports about simultaneous determination of AA, DA, and UA, the proposed method is much more convenient, as to preparation of the GCE/CNT-AgHCFNP-modified electrode.
The voltametric behaviors of a mixture of AA, DA, and UA on bare GCE, GCE/CNT, and GCE/CNT-AgHCFNP elctrodes were studied, and a comparison of the CV results is shown in . DA showed broad oxidation peak and could not be discriminated from AA and UA when a bare GC electrode was used (). For DA, the anodic and cathodic peaks appear at 0.482 V and 0.552 V, respectively, and the separation of peak potential is about 0.070 V. Moreover, the cathodic peak current is much smaller than the anodic peak current. It is clear that the electrochemical reaction of DA at the bare GCE is irreversible, indicating the sluggish electron transfer kinetic of DA at the bare GCE, which may be related to electrode fouling caused by the deposition of this compound and its oxidation product on the electrode surface.Citation14 When GCE/CNT or GCE/CNT-AgHCFNPs was used as the working electrode, the detection sensitivity was improved significantly, and effective separation of the anodic peaks of AA, DA, and UA was obtained.Citation13
Figure 1 Proposed mechanism for Pd (core)-Au (shell) NPs catalyzed oxidation of NADH to NAD.Citation24
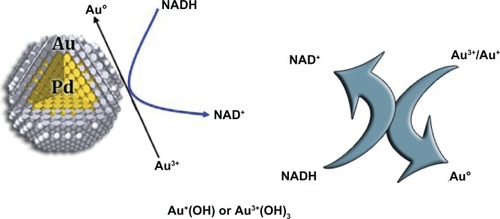
It could be suggested that the modification of the GCE surface by CNT-AgHCFNPs not only improves the electrochemical catalytic activities towards the oxidation of AA, DA, and UA, but also resolves the overlapped oxidation peaks of AA, DA, and UA into three well-defined peaks at potentials 0.193 V, 0.444 V, and 0.620 V in CV, respectively. Comparison between GCE/CNT and GCE/CNT-AgHCFNPs demonstrates that GCE/CNT-AgHCFNPs better facilitates the simultaneous determination of AA, DA, and UA with good stability, sensitivity, and selectivity. In comparison with other electroanalytical methods that report for the simultaneous determination of ascorbic acid, dopamine, and uric acid, this method has better figures of merit. The proposed method can be applied to the simultaneous determination of AA, DA, and UA concentrations in real samples with satisfactory results.Citation13
Choline nanosensors
Choline is distributed in the central and peripheral nervous systems of mammals.Citation15 It is also an important component of phospholipids (lecithin and sphingomyelin), which are required for the synthesis of the neurotransmitter precursor acetylcholine.Citation16 Classified as vitamin-like, choline is generally uptaken by the human body from both dietary and endogenous sources. Determination of choline is carried out by a number of techniques nowadays. As the most promising alternative, enzyme-based biosensors have emerged in the past few years for direct monitoring of choline. A number of enzyme electrodes, with immobilized ChOx, were reported for choline determination based on the detection of liberated H2O2.Citation17,Citation18 The manganese dioxide (MnO2) nanoparticle-modified electrodes show a bidirectional electrocatalytic ability toward the reduction/oxidation of H2O2. Based on this property, a choline biosensor was fabricated via direct and facile electrochemical deposition of a biocomposite that was made of chitosan hydrogel, ChOx, and MnO2 nanoparticles onto a glassy carbon electrode. The biocomposite is homogeneous and easily prepared, and provides a shelter for the enzyme to retain its bioactivity. The results of square wave voltammetry showed that the electrocatalytic reduction currents increased linearly with the increase of choline chloride concentration in the range 1.0 × 10−5 M–2.1 × 10−3 M, and no obvious interference from AA and UA was observed. Good reproducibility and stability were obtained.
Carbon nanotube-modified electrodes have shown excellent electrocatalytic activity towards some electromotive substances, such as H2O2, due to the fast electron transfer ability of CNTs.Citation19
Nicotinamide adenine dinucleotide nanosensors
Nicotinamide adenine dinucleotide (NAD+) and dihydronicotinamide adenine dinucleotide (NADH) are the key central charge carriers in living cells. NAD+ is a ubiquitous cofactor utilized by more than 300 dehydrogenase enzymes. The nicotinamide region is the site of reversible redox processes in living cells. NAD+ and phosphorylated NAD+ (NADP+) are the two forms of coenzyme. NADH and NADPH are reduced forms of NAD+, NADP+ respectively. While NAD+ and NADP+ accept electrons from other molecules, NADH and NADPH donate electrons. NADH plays a key role in the multi-enzyme redox system in the microchrondrial transport chain.Citation20 A large amount of byproduct is often produced during the direct electrode process. Also, the adsorbed NAD+ could cause electrode fouling. Further, the high potentials used for the oxidation of NADH could also cause simultaneous oxidation of many interfering substrates, such as AA.Citation21
The sensitivity of detection of NADH was determined from the plot of absorbance (at 340 nm) vs CNADH. Typical calibration plots were deduced from ultraviolet visible spectroscopy (UV-vis) recorded at 10 min and 90 min. The sensitivity of NADH was determined to be 0.45 mM per unit of absorbance. The detection limit of NADH was estimated to be 0.45 μM. The spectrophotometer can measure absorbance with an accuracy of 0.001. Because the sensitivity of detection for NADH is 0.45 mM for unit absorbance change, the detection limit for NADH could be 0.45 μM. Nanoparticle-NADH sensors performances are different depending on the composition of the sensor, showing different detection limits and linearity.Citation22,Citation23
A plausible mechanism for Palladium (Pd) (core)-Au (shell) NP-catalyzed conversion of NADH is proposed by Gopalan et al (2009).Citation24 It is clear that the position of surface plasmon resonance (SPR) bands of AuNPs showed significant shift during the catalytic transformation of NADH to NAD+. It is therefore envisioned that the surface of the Au layer catalyzes the reaction. They presume that oxygen molecules adsorbed on the surface of the Au layer may induce oxidation of NADH to NAD+. It is known that reactions such as low temperature oxidation of carbon monoxide or propylene that involve oxygen as oxidant are catalyzed by AuNPs.Citation25 In the Gopalan model,Citation24 Au+ or Au3+ species are expected to present in the shell layer (Au) of the Pd (core)-Au (shell) catalyst. NADH oxidation was facilitated by the conversion of Au3+/Au+ to Au0 ().
Lactate nanosensors
L-lactate is constantly produced from pyruvate by lactate dehydrogenase (LDH) in a process of fermentation during normal metabolism and exercise. L-lactate concentration plays an important role in clinical diagnostics, medicine validation, and food analysis. For example, the concentration of L-lactate in blood is a fundamental parameter for the prevention and diagnosis of a number of clinical disorders such as hypoxiaCitation26,Citation27 some acute heart diseases, and drug toxicity tests. Over the past few years, various approaches based on LDH or lactate oxidase have been developed for the detection of lactate. They employ different methods to attach enzymes on the sensing layer, including adsorption,Citation28 cross-linking,Citation29 covalent attachment,Citation30,Citation31 conducting polymer entrapment,Citation32,Citation33 and confinement in sol-gel matrix.Citation34 Flow injection analysisCitation35,Citation36 and mediator-based lactate biosensorsCitation37 have also been developed. In the LDH-based amperometric biosensor, LDH catalyzes the oxidation of lactate to pyruvate in the presence of the oxidized form of NAD+. However, the LDH-based biosensors have some common drawbacks, including the fact that they are unstable and that the electrochemical oxidation of the reduced form of NAD commonly occurs at high potentials, which can allow interference from other electroactive compounds usually present in real samples. Moreover, the intermediates produced during the oxidation reaction can lead to electrode fouling. Therefore, there is a demand for the development of a biosensor that is stable, reproducible, and sensitive for lactate detection in real samples. Recently, Rahman et alCitation38 developed a lactate biosensor based on a conducting polymer, poly-5, 20-50,200-terthiophene-30-carboxylic acid (pTTCA), and multiwall carbon nanotube (MWNT) composite on a gold electrode. LDH and the oxidized NAD+ were subsequently immobilized onto the pTTCA/MWNT composite film. Analytical performances and dynamic ranges of the sensor were determined and the results showed that the sensitivity, stability, and reproducibility of the sensor improved significantly using the pTTCA/MWNT composite film. The calibration plot was linear (coefficient of determination = 0.9995) over the range of 5 to 90 μM. The sensitivity was approximately 0.0106 μA/μM, with a detection limit of 1 μM, based on a signal-to-noise ratio of 3. The applicability of the sensor for the analysis of L-lactate concentration in commercial milk and human serum samples was demonstrated successfully.
Triglyceride nanosensors
Estimation of triglycerides (triacylglycerols) is extremely important, since its high concentration (normal range: 40–160 mg/dL in men, and 35–135 mg/dL in women) can cause hyperlipidemia.Citation39–Citation41 Apart from coronary diseases, hyperlipidemia is associated with several disorders, including diabetes mellitus, nephrosis, liver obstruction, and endocrine impairment. Moreover, estimation of triglyceride content in food has become important due to increased health awareness and stringent regulatory laws.Citation42 The conventional methods for triglycerides detection, such as colorimetric or fluorometric techniques, are based on enzymatic hydrolysis of triglycerides to glycerol and free fatty acids.Citation43–Citation45 Most triglyceride biosensors reported to date are based on multi-enzymes, wherein a biochemical reaction depends upon the enzyme kinetics of another enzymatic reaction. McGowan et alCitation46 have described an enzymatic method for serum triglyceride detection that involves simultaneous catalysis of four bio-enzymes (lipase, glycerol kinase, L-a-glycerophosphate oxidase, and peroxidase).Citation46 These methods are, however, time-consuming, complicated, and expensive. Recently, lipase has been immobilized onto a sol-gel-derived nanostuctured cerium oxide (Nano-CeO2, 35 nm) film deposited onto indium-tin-oxide-(ITO) coated glass plate for tributyrin detection.Citation47 The Nano-CeO2/ITO electrode and lipase/nano-CeO2/ITO bioelectrode have been characterized using scanning electron microscopy (SEM) and CV. The electrochemical response of the lipase/nano-CeO2/ITO bioelectrode towards tributyrin, investigated using CV studies, exhibits linearity, detection limit, and shelf life as 50–500 mg/dl, 32.8 mg/dl, and 12 weeks respectively. The value of the apparent Michaelis–Menten constant obtained as 22.27 mg/dl (0.736 mM) for the lipase/nano-CeO2/ITO bioelectrode, indicating a high affinity of lipase with tributyrin samples ().Citation47
Figure 2 Electrochemical reaction at lipase/nano-CeO2/ITO bioelectrode.Citation47
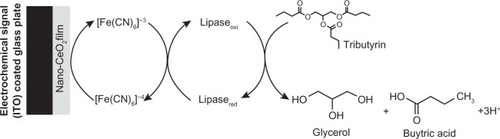
Ochratoxin A detection
Among the various metal oxides, nanostructured zinc oxide (ZnO) has been used for immunosensor applications.Citation48–Citation51 Its unique properties, such as high isoelectric point (IEP ≈ 9.5) and biocompatibility, facilitate immobilization of an enzyme and protein having low IEP via electrostatic interactions. Besides this, the positively charged ZnO nanoparticles not only provide a friendly microenvironment for immobilizing negatively charged rabbit antibodies (r-IgGs; IEP ≈ 5.5) and retain bioactivity, but also accelerate electron transfer communication between the protein and the electrode to a large extent.Citation48,Citation49 Moreover, nontoxicity, high chemical stability, and high electron transfer capability make Nano-ZnO a promising material for immobilization of desired biomolecules for fabrication of an immunosensor. The sol-gel derived Nano-ZnO has recently emerged as an attractive material due to its ease of preparation under ambient conditions, tunable porosity, high thermal stability, chemical inertness, and negligible swelling in aqueous and nonaqueous solutions for immobilization of desired biomolecules (enzymes, proteins, and antibodies)Citation52 Liu et alCitation51 have prepared stable, nanosized, flower-like ZnO film by a hydrothermal method for a H2O2 sensor. ZnO nanocomb has been fabricated in bulk quantity, by the vapor phase transport method, for glucose detection.Citation48 Wei et alCitation48 have grown ZnO nanorods on Au electrodes for the development of an enzymatic glucose biosensor.
Ochratoxin-A (7-[L-β-phenylalanylcarbonyl]-carboxyl-5-chloro-8-hydroxy-3,4-dihydro-3R-methylisocumarin, OTA) is one of the most abundant food-contaminating mycotoxins.Citation53–Citation57 OTA is found in tissues and organs of animals, including human blood and breast milk, and is known to produce nephrotoxic, tetratogenic, carcinogenic, and immune toxic activity in several animal species. OTA contamination has been reported in cereals, coffee, wines, dried fruits, and animal feeds, as well as in tissues and blood of animals and human beings.Citation55,Citation56,Citation58 It affects humans mainly through consumption of improperly stored food products, and causes carcinogenicity. The International Agency of Research on Cancer has classified OTA as a possible carcinogenic compound (Group 2B, possibly by induction of oxidative DNA damage) for humans, since it causes immunosuppression and immunotoxicity.Citation55,Citation56
Nano-ZnO film has been deposited onto ITO glass plate for co-immobilization of r-IgGs and bovine serum albumin (BSA) for OTA detection. The results of x-ray diffraction studies reveal the formation of Nano-ZnO with average particle size ≈ 5.0 nm. Fourier transform infrared spectroscopy, SEM, and electrochemical impedance spectroscopy techniques have been used to characterize the Nano-ZnO/ITO electrode and the BSA/r-IgGs/Nano-ZnO/ITO immunoelectrode. The electrochemical impedimetric response of the BSA/r-IgGs/Nano-ZnO/ITO immunoelectrode, obtained as a function of OTA concentration, exhibits linearity as 0.006–0.01 nM/dm3, detection limit of 0.006 nM/dm3, response time of 25 s, and sensitivity of 189 Ω/nM/dm3cm−2, with a regression coefficient of 0.997.Citation55
Urea nanosensors
Urea is widely distributed in nature, and its analysis is of considerable interest in clinical and agricultural chemistryCitation60,Citation61 It is known to be an important marker for evaluating uremic toxin levels. The normal level of urea in serum is from 15 to 40 mg/dL (2.5–7.5 mM/l). In patients suffering from renal insufficiency urea concentrations in serum vary from 180 to 480 mg/dL and at elevated levels above 180 mg/dL, hemodialysis is required. Apart from clinical applications, there is a growing demand for robust, reliable instrumentation toward the estimation of urea in other fields (eg, food science and environmental monitoring). As the principal component of nonprotein nitrogen in cow milk, urea is utilized as an indicator of protein-feeding efficiencyCitation62 Nanopatterned porous alumina, prepared by electrical anodization in acid solution, has been used as an enzyme electrode and pH sensor for urea detection. The biocompatibility of nano-size porous alumina as an immobilization matrix for biocolloidal systems has stimulated interest for improved sensor sensitivity. The sensor was tailored to enlarge the active surface area, which in turn increased the sensitivity of the sensor. Some other supports used for urea biosensor fabrication include bilayer lipid membranes,Citation63 carbon paste electrodes,Citation64 composite hydrogel membranes,Citation65 gelatin membranes,Citation66 inorganic matrices (eg, clays, laponite, and zinc-aluminum-layered double hydroxide matrices),Citation67 silicon dioxide (SiO2) films, glass beads, diatomite, silica, porous glass, hornblende, and molecular sieves.Citation68
Srivastava et alCitation66 have described a urea biosensor that utilizes urease immobilized on gelatin beads. The system was successful in achieving a long storage stability, with a half-life of 240 days. The characteristics of this sensor include a detection limit from 0.8 to 23 mM and a response time of 6 min. Another sensing system has been proposed for urease immobilization on porous glass beads.Citation69 The sensor setup proposed by Seki’s groupCitation70 used urease immobilized on SiO2 films as the pH-sensitive layer in light-addressable potentiometric sensors, with a detection range from 5–15 mM. However, more studies must be done in this field to fabricate a cost-effective, commercial sensor using these novel materials. The use of enzyme mixtures can provide improved performance in urea sensors. Seo et alCitation71 determined ammonia via amperometric methods, using the immobilization of L-glutamate dehydrogenase on the immobilon-AV affinity membrane. Incorporation of the enzyme into a carbon paste was performed by Yang et alCitation64 for the construction of an amperometric enzyme electrode. The enzyme-modified carbon paste electrode resulted in improved specificity and sensitivity. This system made use of bi-enzyme systems involving glutamate dehydrogenase and urease for urea estimation ().
Figure 3 Schematic representation of a urea biosensor.Citation66
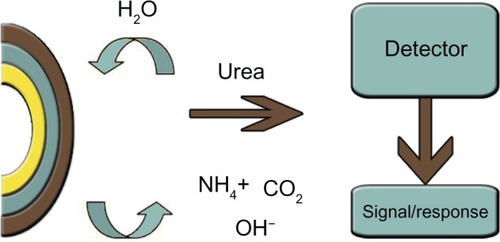
Nanoparticles in bioassays
Human immunoglobulin G
Bioassays are widely used in medical applications for sensitive and specific detection of biomolecules such as antibodies and antigens. The enzyme-linked immunosorbent assay (ELISA) is a well-known and commonly used tool for this purpose.Citation72 However, in typical ELISA systems, several washing steps are performed that lead to an increased risk of, for example, residual contamination or loss in reagent, thereby reducing the apparent sensitivity, accuracy, and/or reliability. Moreover, ELISA measurements give a static picture of the sample in terms of concentration of analyte, but provide no information about the binding kinetics or the dynamics of the reactions. A new approach for bioassays based on frequency-and time-domain measurements of magnetic nanoparticles (MNP) was recently introduced by Oisjoen et al.Citation73 They demonstrate a one-step wash-free bioassay measurement system capable of tracking biochemical binding events. They combine the high resolution of frequency- and high speed of time-domain measurement in a single device in combination with a fast one-step bioassay. The one-step nature of their magnetic nanoparticle-based assay reduces the time between sample extraction and quantitative results, while mitigating the risks of contamination related to washing steps. The biofunctionalized MNPs they use for their experiment are multicore particles containing single domains of cobalt ferrous oxide. According to the manufacturer, the MNP system has a median hydrodynamic radius of 50 nm. The size distribution is a critical factor in determining the sensitivity of this system. Larger MNPs have smaller relative change in hydrodynamic radii associated with molecules binding to their surfaces. The particles are functionalized with streptavidin, a biomolecule that has high affinity for biotin. According to specification from the provider, the MNPs can bind 80 pmol of biotinylated protein per mg of MNPs, which corresponds roughly to 30 poly(sebacic anhydride) (PSA) molecules per MNP. This system has an advantage in that it does not require a separation step, which is usually employed for PSA detection through Brownian relaxation measurements of functionalized MNPs.Citation73 There is no potential loss of signal associated with competitive binding of free analyte. This system has a sensitivity to 2 μL samples, which means that less sample volume from a patient is needed, which provides the capability to perform additional parallel screening-type measurement on a single sample.
Many immunoassay techniques have been developed. Some substances such as radioisotopes, enzymes, chemiluminescent, and fluorescent compounds have been used as labels in immunoassay. However, water-soluble cadmium tellurium (CdTe) nanocrystals have attracted considerable attention as novel biological luminescent labels, compared to the traditional labels, in recent years. They have some unique physical and chemical properties, such as excellent brightness, narrow and precise, tunable emission, negligible photobleaching, fairly high quantum yields, and good chemical stability. Lately, some luminescent nanoparticles are also becoming very attractive for immunoassays. Crystalline europium oxide nanoparticles,Citation74 water-soluble poly (acrylic acid) grafted luminescent silicon nanoparticlesCitation75 and cadmium/selenium-zinc/sulphur core-shell quantum dotsCitation76 have been successfully applied as fluorescence labels in fluoroimmunoassays. The experimental procedure for determination of h-IgG using CdTe is very simple, involving the mixing of ten microliters of an analyte h-IgG with 0.8 ml of iron oxide (Fe3O4) and primary antibody solution. The mixture was incubated for 30 min at 37°C. The Fe3O4/primary antibody/h-IgG conjugate was formed, and then 2.0 mL of CdTe-secondary antibody conjugate was added into the tube to form the sandwich-type complex. Li et alCitation77 were able to use this technique and show that the sandwich-type complex in an immunoassay can be sensitively detected by colloidal CdTe nanoparticles as labels. The CdTe label has better sensitivity and reproducibility than the traditional fluorescent labels. In addition, the CdTe nanoparticle labeling procedure is very simple, and the biochemical activity of the labeled compound is almost unaffected. Dextran–Fe3O4 magnetic nanoparticles were used in the immunoassays, so the separation procedure is simple and rapid. This new technique may be applied in many types of antibody–antigen system.
Surface-enhanced Raman scattering (SERS)-based immunoassay, a new technique with high sensitivity, showing the ability to detect picomole to femtomole amounts, is known as a potent detection means for protein determination. Raman reporter-labeled immuno-gold nanoparticles are utilized, and the biologic proteins are finally assayed qualitatively or quantitatively by the characteristics SERS peaks of the reporter.Citation78,Citation79 It is well known that the response ability of the SERS-based immunoassay is critically related to the size of the gold nanoparticles, as well as the aggregation degree of the immune aggregates.Citation80,Citation81 Beermann et alCitation82 have investigated the surface enhancement ability of an isolated gold nanoparticle and found that a single nanoparticle with an average dimension of 25 nm was insufficient to enhance the Raman signals of the reporter. Similarly, it was discovered that no signal could be detected using small gold nanoparticles as a substrate.Citation77 Conversely, bigger gold nanoparticles will result in greater SERS signals. But their stability may decrease significantly with the increasing sizes when they are modified with Raman reporters.Citation82 Thus, taking into account the higher stability, many researchers have to carry out the SERS-based immunoassay with small gold nanoparticles. Meanwhile, an additional treatment (eg, silver stain) for SERS activation must be executed to ensure immunoassay with higher sensitivity.Citation83–Citation85
A highly sensitive immunoassay based on SERS has been developed with a novel immune marker, a Raman reporter-labeled immuno-gold aggregate on a SERS-active immune substrate. The features of those immune aggregates were characterized by UV-vis extinction spectra, transmission electron microscope images, SEM pictures, and SERS spectra. It is found that stable gold aggregates in appropriate morphologies can be induced by mixing proper amounts of reporter molecules with gold nanoparticles. Based on those reporter-labeled Au aggregates, immune aggregates with high stability can be prepared successfully by immobilizing antibody to the surface of the aggregates. Using this proposed immunoassay structure, the concentration detection of h-IgG was performed, and a calibration curve was obtained in the range from 100 ngmL−1 to 100 fgmL−1. This opens a new avenue for sensitive immunoassay and other biochemical analysis based on SERS.
Steroids
Steroids are lipid compounds. With the exception of cholesterol, steroids are natural hormones or hormone precursors. The determination of the levels of steroid hormones is an important issue for the inspection of endocrinological disorders related to adrenal or gonadal function. Among analytical methods used to determine the concentrations of steroid hormones or their precursors are immunoassays,Citation86–Citation88 fluorescence resonance energy transfer,Citation89 SPR,Citation90 gas chromatography-mass spectrometry (GC/MS),Citation91 and liquid chromatography-mass spectrometry (LC/MS).Citation92–Citation94 A sensitive assay for hormone detection that typically involves a mass spectrometer coupled with either a gas chromatograph or a liquid chromatograph has been developed by Fenlon et al.Citation95 For these hormones, like anabolic steroids, according to the differences in fragmentation caused by collisions of medium energy related to the structure, it is difficult to find a common product ion or neutral loss. Furthermore, not only do most ELISA-like assays lack the sensitivity required to determine >90% of hormone derivatives,Citation94 but also these analytical procedures might introduce artefacts. The detection limits of the above methods range from ng/mL to pg/mL.Citation96 One-dimensional nanostructures such as carbon nanotubes and silicon nanowires (SiNW) have been demonstrated to be sensitive chemical and biological sensors.Citation78 That detection results from the disturbance of charge on the surface of the functionalized nanostructure on which the target molecules are specifically recognized. For instance, the real-time detection of various antigens,Citation97 oligonucleotides,Citation98,Citation99 proteins,Citation100,Citation101 and charged small molecules has been shown to be feasible on devices using nanowire or carbon-nanotube transistors as active transducers. The sensing mechanism in an electrically-based biosensor relies on an altered conductance or threshold voltage induced by the attachment of the charged analytes. Chang et alCitation102 attempted to integrate protein engineering with the sensitive nature of a SiNW-field-effect transistor (FET) in charge disturbance to overcome the intrinsic weakness of SiNW-FET in detecting uncharged analytes. They chose an uncharged steroid, 19-norandrostenedione, as the target analyte. Delta 5-3-ketosteroid isomerase has served as a receptor for steroid recognition because of its well understood enzyme function. The primary concept of the sensing mechanism is based on intramolecular binding of a charged ligand, functioning as a reporter, to mimic the binding of an analyte to a protein. The major driving force favoring this association is generally thought to be the hydrophobic effect that prompts the hydrophobic ligand to bind with the protein. The thermodynamics of protein-ligand binding can be altered by a favorable control of enthalpy and, particularly in this model, the characteristic “entropy-driven” thermodynamic signature of the steroid. The analyte might replace the pre-situated ligand, which becomes thus exposed to the surface of the SiNW and perturbs the charge density and conductance of the nanostructure. In the presence of a steroid, the negatively charged 5-(2-aminoethylamino)-1-naphthalenesulfonic acid (1,5-EDANS) moiety, which presumably occupies the steroid-binding site, is expelled and exposed to the nanowire surface (). The electrical response produced from the 1,5-EDANS moiety is measured and the concentration is calculated accordingly. The sensitivity of this novel nano-bio-device can attain a femtomolar level.Citation102
Figure 4 SiNW-FET platform for the detection of uncharged steroid-related analyte.Citation105
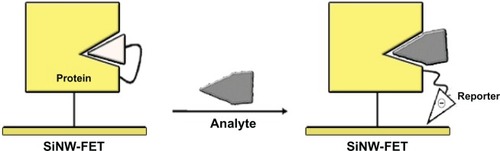
C-reactive protein
Recently a label-free quartz crystal microbalance (QCM) immunosensor for an important biomarker, C-reactive protein (CRP),Citation103–Citation105 related with coronary heart disease, hypertension, and inflammation, has been developed with picomolar sensitivity.Citation106 However, a possible matrix effect caused by serum viscosity might be present, like coloring substances in the case of ELISA.Citation107 One way to avoid it is to dilute samples, including serum and plasma, to the degree at which sample viscosity approaches that of the reaction buffer. Recently, Kim et alCitation107 conspicuously increased the sensor signal, and thus sensitivity of a label-free QCM immunosensor for CRP, by introducing a streptavidin-coated gold nanoparticle during antibody-antigen complexation, based on an indirect competitive assay format. When 200 μL of the modified antibody, having the concentration of 0.250 mg/mL, was added with GNp to the immunosensor system, the frequency shift obtained was 139.8 ± 0.3 Hz. Compared to the frequency shift of 91.1 ± 1.3 Hz found with the addition of the unmodified antibody only, the signal augmentation after GNp binding amounted to 53.4%, which resulted in sensitivity improvement of the immunosensor.
Conclusion
Clinicians, food technologists, and environmentalists all have an interest in generally increased sensitivity and limits of detection for a range of analytes. While the precise demands to meet today’s requirements may be modest in these respects, few would contest the longer term benefits of reliable detection of trace amounts of various diagnostic indicators, additives, or contaminants.
As chemical analysis becomes simpler and more widely available, we can expect more accurate diagnosis and prognosis for different diseases. Nanosensors, capable of providing data through unique technology, could find wide application in monitoring our personal health, the food we eat, and our environmental health. The performance of nano-biochemical sensors is excellent in terms of sensitivity, selectivity, linearity, stability, response time, and reproducibility compared to the traditional biosensors. Nanoparticle labeling procedure is very simple, and the biochemical activity of the labeled compound is almost unaffected. This new approach is critically useful in preventing interference between chemically related analytes.
The use of core-shell NPs for the biocatalytic transformation of an enzyme could form the basis for the fabrication of an optical sensor for redox compounds. Moreover, the low Kmapp value recorded when enzymes were immobilized on nano-biosensors indicates high affinity of sensors to analytes, which is interesting and promising for biosensor electrode construction.
Disclosure
The authors report no conflicts of interest in this work.
References
- MurphyCJJanaNRControlling the aspect ratio of inorganic nanorods and nanowiresAdv Mater20021480
- SunYXiaYShape-controlled synthesis of gold and silver nanoparticlesScience2002298217612481134
- BalasubramanianBKraemerKLRedingNASkomskiRDucharmeSSellmyerDJSynthesis of monodisperse TiO(2)-paraffin core shell nanoparticles for improved dielectric propertiesACS Nano2010441893190020359188
- van DijkMATchebotarevaALOrritMAbsorption and scattering microscopy of single metal nanoparticlesPhys Chem Chem Phys20068348616871337
- LingJChengZHYuanFLLight scattering signals from nanoparticles in biochemical assay, pharmaceutical analysis and biological imagingTrends Analyt Chem2009284
- ThanhNTRosenzweigZDevelopment of an aggregation-based immunoassay for anti-protein A using gold nanoparticlesAnal Chem20027471624162812033254
- JiangZLSunSJLiangAHLiuCJA new immune resonance scattering spectral assay for trace fibrinogen with gold nanoparticle labelAnal Chim Acta2006571120017723439
- JiangZHuangWLiJLiangAZhangSChenBNanogold catalysis-based immunoresonance-scattering spectral assay for trace complement component 3Clin Chem200854111612317998268
- ClarkLCLyonsCElectrode systems for continous monitoring in cardiovascular surgeryAnn N Y Acad Sci196210215551582
- IannielloRMYacynyehAMCarbon nanotube/chitosan/gold nanoparticles-based glucoseAnal Chem1981532090
- WangYWanzhiWXiaoyingLXiandongZCarbon nanotube/chitosan/gold nanoparticles-based glucose biosensor prepared by a layer-by-layer techniqueMater Sci Eng C20092915054
- YuJYuDZhaoTZengBDevelopment of amperometric glucose biosensor through immobilizing enzyme in a Pt nanoparticles/mesoporous carbon matrixTalanta20087451586159118371821
- MeissamNMotlaghKAboozarTPreparation of silver hexacyanoferrate nanoparticles and its application for the simultaneous determination of ascorbic acid, dopamine and uric acidTalanta20108051657166420152393
- SoundappaTHsuanTShen-MingChen TEasy modification of glassy carbon electrode for simultaneous determination of ascorbic acid, dopamine and uric acidBiosens Bioelectron20092482712271519162467
- SofroniewMVPriestleyJVConsolazioneAEckensteinFCuelloACCholinergic projections from the midbrain and pons to the thalamus in the rat, identified by combined retrograde tracing and choline acetyltransferase immunohistochemistryBrain Res1985329122132232983837
- PanfiliGManziPCompagnoneDScarcigliaLPalleschiGRapid assay of choline in foods using microwave hydrolysis and a choline biosensorJ Agric Food Chem20004883403340710956124
- ShimomuraTItohTMizukamiFOnoMAmperometric determination of choline with enzyme immobilized in a hybrid mesoporous membraneTalanta200978121722019174228
- DaiHChiYWangYWeiMChenGBiocompatible electrochemiluminescent biosensor for choline based on enzyme/titanate/chitosan composite modified electrodeBiosens Bioelectron20102561414141919945840
- WangJMusamaehMCarbon nanotube/teflon composite electrochemical sensors and biosensorsAnal Chem20037592075207912720343
- WhiteAHandlerPSmithELHillRLLehmanIRPrinciples of Biochemistry9th edNew York, NYMcGraw-Hill1978
- HanJAdsorption and electrochemical oxidation behaviour of NADH at a clean platinum electrodeJ Electroanal Chem1980110295302
- KotzianPJankuTKalcherKVytrasKCatalytic activity of iron hexacyanoosmate (II) towards hydrogen peroxide and nicotinamide adenine dinucleotide and its use in amperometric biosensorsAnal Chim Acta2007599228729317870292
- TangLZengGShenGHighly sensitive sensor for detection of NADH based on catalytic growth of Au nanoparticles on glassy carbon electrodeAnal Bioanal Chem20093936716771684
- GopalanARagupathyDKimHTManeshKMLeeKPPd (core)-Au (shell) nanoparticles catalyzed conversion of NADH to NAD+ by UV-vis spectroscopy – a kinetic analysisSpectrochim Acta A Mol Biomol Spectrosc200974367868419717334
- ValdenMLaiXGoodmanDWOnset of catalytic activity of gold clusters on titania with the appearance of nonmetallic propertiesScience19982815383164716509733505
- ArtissJDKarcherRECavanaghKTA liquid-stable reagent for lactic acid levelsAm J Clin Pathol200011413914310884809
- SuzukMAkagumaHChemical cross-talk in flow-type integrated enzyme sensorsSens Actuators B Chem200064136141
- ParraACaseroEVazquezLParienteFLorenzoEDesign and characterization of a lactate biosensor based on immobilized lactate oxidase onto gold surfacesAnal Chim Acta2006555308315
- MarzoukSAMCosofretVVBuckRPYangHCascioWEHassanSSMA conducting salt-based amperometric biosensor for measurement of extracellular lactate accumulation in ischemic myocardiumAnal Chem199769264626529230678
- HaccounJPiroBTranLDDangLAPhamMCReagentless amperometric detection of L-lactate on an enzyme-modified conducting copolymer poly(5-hydroxy-1,4-naphthoquinone-co-5-hydroxy-3-thioacetic acid-1,4-naphthoquinone)Biosens Bioelectron2004191325132915046766
- PalmisanoFRizziRCentonzeDZamboninPGSimultaneous monitoring of glucose and lactate by an interference and cross-talk free dual electrode amperometric biosensor based on electropolymerized thin filmsBiosens Bioelectron200015910531539
- SumanSSinghalRSharmaALMalthotraBDPundirCSDevelopment of a lactate biosensor based on conducting copolymer bound lactate oxidaseSens Actuators B Chem2005107768772
- TrojanowiczMGeschkeOKrawczykTKVCammannKBiosensors based on oxidases immobilized in various conducting polymersSens Actuators B Chem199528191199
- ParkTMIwuohaEISmythMRFreaneyRMcShaneAJSol-gel based amperometric biosensor incorporating an osmium redox polymer as mediator for detection of L-lactateTalanta199744697397818966828
- LowinsohnDBertottiMFlow injection analysis of blood L-lactate by using a Prussian Blue-based biosensor as amperometric detectorAnal Biochem200736526026517449005
- PalmisanoFQuintoMRizziRZamboninPGFlow injection analysis of L-lactate in milk and yogurt by on-line microdialysis and amperometric detection at a disposable biosensorAnalyst2001126686687011445953
- TurnerAPFKarubeIWisonGSBiosensors:fundamentals and applicationsOxford, UKOxford Science1987
- RahmanMMShiddikyMJRahmanMAShimYBA lactate biosensor based on lactate dehydrogenase/nictotinamide adenine dinucleotide (oxidized form) immobilized on a conducting polymer/multiwall carbon nanotube composite filmAnal Biochem200938415916518851940
- CaplanLAPesceAJClinical Chemistry: theory, analysis, correlationSt Louis, MOMosby1989
- AvramogluRBasicianoKAdeliHKLipid and lipoprotein dysregulation in insulin resistant statesClin Chim Acta200636811916480697
- VijayalakshmiATarunashreeYBaruwatiBEnzyme field effect transistor (ENFET) for estimation of triglycerides using magnetic nanoparticlesBiosens Bioelectron2008231708171418356036
- ReddyRRKChadhaABhattacharyaEPorous silicon based potentiometric triglyceride biosensorBiosens Bioelectron20011631331711390219
- BucoloGDavidHQuantitative determination of serum triacylglycerols by use of enzymesClin Chem1973194764824703655
- FossatiPPrencipeLSerum triglycerides determined colorimetrically with an enzyme that produces hydrogen peroxideClin Chem198228207720806812986
- MendezAJCabezaCHsiaSLA flurometric method for the determination of triglycerides in the nanomolar quantitiesAnal Biochem19861563863893766939
- McGowanMWArtissJDStrandberghDRZakBA peroxidase-coupled method for the colorimetric determination of serum triglycerideClin Chem1983295385426825269
- SolankiPRDhandCKaushikAAnsariAASoodKNMalhotraBDNanostructured cerium oxide film for triglyceride sensorSens Actuators B Chem2009141551556
- WeiASunaXWWangJXEnzymatic glucose biosensor based on ZnO nanorod array grown by hydrothermal decompositionAppl Phys Lett200689123902
- WangJFSunXWWeiAZinc oxide nanocomb biosensor for glucose detectionAppl Phys Lett200688233106
- AnsariAASinghRSumanaGMalhotraBDSol-gel derived nanostructured zinc oxide film for sexually transmitted disease sensorAnalyst2009134997100219381396
- LiuYLYangYHYangFHLiuMZShenLGYuQRNanosized flower-like ZnO synthesized by a simple hydrothermal method and applied as matrix for horseradish peroxidase immobilization for electro-biosensingJ Inorg Biochem2005992046205316095710
- YuJJuHPreparation of porous titania sol-gel matrix for immobilization of horseradish peroxidase by a vapor deposition methodAnal Chem2002743579358312139071
- RadiAEBerbelXMLatesVMartyJLLabel-free impedimetric immunosensor for sensitive detection of ochratoxin ABiosens Bioelectron2009241888189219013783
- PfohlLAPetkovaBTChernozemskyINCastegnaroMBalkan endemic nephropathy and the associated urinary tract tumors: review on etiological causes and the potential role of mycotoxinsFood Addit Contam20021928230211834078
- Kuiper-GoodmanTMycotoxins and phytotoxins in perspectiveMiragliaMvan EgmondHBreraCGilbertJMycotoxins and Phycotoxins – developments in chemistry, toxicology and food safetyFort Collins, COAlaken199825
- ZimmerliBDickROchratoxin A in table wine and grape-juice: occurrence and risk assessmentFood Addit Contam19961366556688871123
- AnsariAAKaushikASolankiPRMalhotraBDSol-gel derived nanoporous cerium oxide film for application to cholesterol biosensorElectrochem Commun20081012461268
- KaushikASolankiPRAnsariAAAhmadSMalhotraBDChitosan – iron oxide nanobiocomposite based immunosensor for ochratoxin-AElectrochem Commun20081013641368
- AnsariAAKaushikASolankiPRMalhotraBDNanostructured zinc oxide platform for mycotoxin detectionBioelectrochemistry201077758119648064
- ChuaKSSerum urea analysis using the Beckman BUN AnalyzerJ Clin Pathol197629517519820726
- TaufikMYusuffMHarunaOAMuhamadNMEffect of mixing urea with humic acid and acid sulphate soil on ammonia loss, exchangeable ammonium and available nitrateAm J Environ Sci200955588591
- CarlssonJPehrsonBResponses in urea and true protein of milk to different scheme protien feedingActa Vet Scand19943567778209822
- NikolelisDPSiontorouCOBilayer lipid membranes for flow injection monitoring of acetylcholine, urea, and penicillinAnal Chem1995675369944
- YangMYangYLiuYShenGYuRPlatinum nanoparticles-doped sol-gel/carbon nanotubes composite electrochemical sensors and biosensorsBiosens Bioelectron2006211125113115885999
- ChenJChiuSComposite hydrogel membrane for urease immobilization to enhance urea hydrolysis rateEnzyme Microb Technol20002635936710713208
- SrivastavaPKKayasthaAMSrinivasanKCharacterization of gelatin-immobilized pigeonpea urease and preparation of a new urea biosensorBiotechnol Appl Biochem200134556211483155
- BarhoumiHMaarefARammahMInsulator semiconductor structures coated with biodegradable latexes as encapsulation matrix for ureaseBiosens Bioelectron2005202318232315797333
- ThorntonDByrneMJFlynnAJohnsonDBStudies on the immobilization of trypsin on sandBiochem Soc Trans197421360
- YoneyamaKFujinoYOsakaTSatohIAmperometric sensing system proposed urease immobilization on porous glass beadsActuators200176152157
- SekiAIkedaSKuboIKarubeIBiosensors based on light-addressable potentiometric sensors for urea, penicillin.and glucoseAnal Chim Acta1998373913
- SeoMLKimJSLeeSSIron oxide-chitosan nano biocomposite for urea sensorJ Chem Soc199337937
- KeckLDSkeelesJKMcNewRWAntibody detection in matched chicken sera and egg-yolk samples by commercial enzyme-linked immunosorbent assay kits for Newcastle disease virus, infectious bronchitis virus, infectious bursal disease virus, and avian reovirusAvian Dis19933738258288257378
- OisjoenFSchneidermanJFPrietoAstalan AKalabukhovAJohanssonCWinklerDA new approach for bioassays based on frequency- and time-domain measurements of magnetic nanoparticlesBiosens Bioelectron20102551008101319822413
- FengJShanGMaquieiraAFunctionalized europium oxide nanoparticles used as a fluorescent label in an immunoassay for atrazineAnal Chem20037552825286
- LiZFRuckensteinEWater-soluble poly (acrylic acid) grafted luminescent fluorescent silicon nanoparticles and their use as biological staining labelsNano Lett2004414651467
- GoldmanERMauroJConjugation of luminescent quantum dots with antibodies using an engineered adaptor protein to provide new reagents for fluoroimmunoassaysAnal Chem20027484184711866065
- LiXWangLZhouCGuanTLiJZhangYPreliminary studies of application of CdTe nanocrystals and dextran-Fe3O4 magnetic nanoparticles in sandwich immunoassayClinica Chimica Acta2007378168174
- CuiYWeiQParkHLieberCMNanowire nanosensors for highly sensitive and selective detection of biological and chemical speciesScience20012931289129211509722
- ChenMHChuangYJTsengFGSelf-masked high-aspect-ratio polymer nanopillarsNanotechnology2008195050530119942765
- WangGParkHYLipertRJPorterMDMixed monolayers on gold nanoparticle labels for multiplexed surface-enhanced Raman scattering based immunoassaysAnal Chem200981239643965019874000
- KnauerMIvlevaNPLiuXNiessnerRHaischCSurface-enhanced Raman scattering-based label-free microarray readout for the detection of microorganismsAnal Chem20108272766277220196561
- BeermannJNovikovSMLeossonKBozhevolnyiSISurface enhanced Raman imaging: periodic arrays and individual metal nanoparticlesOpt Express200917151269819654675
- HanXXKitahamaYItohTWangCXZhaoBOzakiYProteinmediated sandwich strategy for surface-enhanced Raman scattering: application to versatile protein detectionAnal Chem20098193350335519361230
- SongCWangZZhangRYangJTanXCuiYHighly sensitive immunoassay based on Raman reporter-labeled immuno-Au aggregates and SERS-active immune substrateBiosens Bioelectron200925482683119765972
- ChonHLeeSSonSWOhCHChooJHighly sensitive immunoassay of lung cancer marker carcinoembryonic antigen using surface-enhanced Raman scattering of hollow gold nanospheresAnal Chem20098183029303419301845
- EnglandBGParsonsGHPossleyRMMcConnellDSMidgleyARUltrasensitive semiautomated chemiluminescent immunoassay for estradiolClin Chem20024891584158612194939
- TaiebJBenattarCBirrASLindenbaumALimitations of steroid determination by direct immunoassayClin Chem200248358358511861459
- HungerfordNLSortaisBSmartCGAnalysis of anabolic steroids in the horse: development of a generic ELISA for the screening of 17alpha-alkyl anabolic steroid metabolitesJ Steroid Biochem Mol Biol20059631733416040239
- MacaraIGLanniganDANovel biosensors for the detection of estrogen receptor ligandsJ Steroid Biochem Mol Biol20059623524415985367
- ToshieKTTomofumFTadashiOHX531, a retinoid X receptor antagonist, inhibited the 9-cis retinoic acid-induced binding with steroid receptor coactivator-1 as detected by surface plasmom resonanceJ Steroid Biochem Mol Biol20059430330915857749
- VenturaRRoigMPerezBDetection of the administration of 17beta-nortestosterone in boars by gas chromatography/mass spectrometryRapid Commun Mass Spectrom2008221863187018481350
- QinFZhaoYYSawyerMBLiXFHydrophilic interaction liquid chromatography-tandem mass spectrometry determination of estrogen conjugates in human urineAnal Chem2008803404341118348576
- PozoOJEenooPVThuyneWVDeventerKDelbekeFTDirect quantification of steroid glucuronides in human urine by liquid chromatography-electrospray tandem mass spectrometryJ Chromatogr A2008118310811818258241
- GeislerJEkseDHelleHDuongNKLonningPEAn optimized, highly sensitive radioimmunoassay for the simultaneous measurement if estrone estradiol and estrone sulfate in the ultra-low range in human plasma samplesJ Steroid Biochem Mol Biol2008109909518242079
- FenlonKAJohnsonACTylerCRHillEMGas-liquid chromatography-tandem mass spectrometry methodology for the quantitation of estrogenic contaminants in bile of fish exposed to wastewater treatment works effluents and from wild populationsJ Chromatogr A20101217111211819932485
- Al-DujailiEADevelopment and validation of a simple and direct ELISAmethod for the determination of conjugated (glucuronide) and non-conjugated testosterone excretion in urineClin Chim Acta200636417217916098502
- PalSAlociljaECDownesPFNanowire labeled direct-charge transfer biosensor for detecting Bacillus speciesBiosens Bioelectron2007222329233617320373
- SoHMWonKKimYHSingle walled carbon nanotube biosensors using aptamers as molecular recognition elementsJ Am Chem Soc2005127119061190716117506
- KimDSJeongYTParkHJAn FET-type charge sensor for highly sensitive detection of DNA sequenceBiosens Bioelectron200420697415142578
- BestemanGLeeJOWiertzFGMHeeringHADekkerCEnzyme-coated carbon nanotubes as single-molecule biosensorsNano Lett20033727730
- PatolskyFLieberCMNanowire nanosensorsMater Today200582028
- ChangKSChenCCSheuJTLiY-KDetection of an uncharged steroid with a silicon nanowire field-effect transistorSens Actuators B Chem2009138148153
- GabayCKushnerINAcute-phase proteins and other systemic responses to inflammationN Engl J Med19993404484549971870
- RifaiNRidkerPMHigh-sensitivity C-reactive protein: a novel and promising marker of coronary heart diseaseClin Chem20014740341111238289
- WolfMJunckerDMichelBHunzikerPEmmanuelDSimultaneous detection of C-reactive protein and other cardiac markers in human plasma using micromosaic immunoassays and self-regulating microfluidic networksBiosens Bioelectron2004191193120215046750
- KimNParkISKimWYSalmonella detection with a direct-binding optical grating coupler immunosensorSens Actuators B Chem2007121606615
- KimNKimYJChoDKGold nanoparticle-based signal augmentation of quartz crystal microbalance immunosensor measuring C-reactive proteinCurr Appl Phys201010412271230