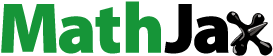
Abstract
By mimicking Nature, we can design and synthesize inorganic smart materials that are reactive to biological tissues. These smart materials can be utilized to design innovative third-generation biomaterials, which are able to not only optimize their interaction with biological tissues and environment, but also mimic biogenic materials in their functionalities. The biomedical applications involve increasing the biomimetic levels from chemical composition, structural organization, morphology, mechanical behavior, nanostructure, and bulk and surface chemical–physical properties until the surface becomes bioreactive and stimulates cellular materials. The chemical–physical characteristics of biogenic hydroxyapatites from bone and tooth have been described, in order to point out the elective sides, which are important to reproduce the design of a new biomimetic synthetic hydroxyapatite. This review outlines the evolving applications of biomimetic synthetic calcium phosphates, details the main characteristics of bone and tooth, where the calcium phosphates are present, and discusses the chemical–physical characteristics of biomimetic calcium phosphates, methods of synthesizing them, and some of their biomedical applications.
Introduction
Biomimetism of synthetic materials for biomedical applications can be carried out at different levels according to the composition, structure, morphology, and bulk and surface chemical–physical properties of the synthetic materials. Synthetic biomaterials should mimic all the characteristics of biological tissues, in order to optimize their interactions, and to mimic biogenic materials in their functionalities. Chemists, biologists, physicists, and engineers interested in material science are amazed by the high degree of sophistication, miniaturization, hierarchical organization, hybridizing, reliability, efficiency, resistance, and adaptability that characterize natural materials.
These properties of biogenic materials, which have been achieved through specific building principles selected by evolution, can only be partially obtained in manmade materials by present synthetic processes. For this reason, Nature is a school for material science. Biomimetism and bioinspiration represent important tools for the design and the synthesis of innovative materials and devices.
Biogenic material morphologies are related to specific strategies for the long-range chemical construction of well-organized architectures from preformed nano- or microcrystalline inorganic building blocks. Actually, many biologic complex structures are obtained by promoting specific links induced by the conformation variability at the nanometer scale of biological macromolecules.
Hydroxyapatite (HA), Ca10(PO4)6(OH)2, is one of the most stable forms of the calcium phosphates and the major inorganic component of bone and teeth in mammals. HA is extensively investigated in numerous interdisciplinary areas, from a better understanding of the formation mechanisms in natural mineralization processes to the applicability as a biomedical or industrial material. The biocompatibility, bioactivity, bioresorbability, osteoconductivity, size dimension, morphology, and surface functionalization represent the physical and chemical properties which should be tailored in synthetic HA crystals to optimize their specific biomedical applications.
The recent trend in biomaterials research is focused on overcoming the limitations of calcium phosphates, hydroxyapatite ceramics (low bioresorbability, surface area, and bioreactivity), and on improving their biological properties by exploring the unique advantages of nanotechnology. Using nanotechnology, it is possible to synthesize inorganic crystals with nanometric dimensions, characterized by a high bioresorbability, surface area, and surface structural disorder which increase crystal bioreactivity. The trend is shifting toward nanotechnology to improve the biological responses of HA, because nano-HA is a constituent of bone.
The use of nano-HA in orthopedics is considered to be very promising, due to its dimensional similarity with the bone crystals. Nanotechnology offers a unique approach to overcome the shortcomings of many conventional materials. From nanomedicine to nanofabrics, this promising technology has encompassed almost all disciplines of human life. Nanostructured materials offer more improved performance than their larger—particle-sized counterparts, due to their large surface to volume ratio and unusual chemical/electronic synergistic effects.
In this review, we discuss the principal characteristics of bone and tooth, where hydroxyapatites are present, chemical–physical characteristics, the methods to synthesize biomimetic hydroxyapatites, and some of their biomedical applications.
Bone
Bone is a complex composite of biopolymer and biomineral in a mineralized matrix. The biopolymer consists of matrix proteins, mostly collagen (type I), with some minor noncollagenous proteins (NCPs; eg, proteoglycans) and minor amounts of lipids and osteogenic factors (eg, bone morphogenetic proteins, BMPs).Citation1–Citation4 Bone is formed by a series of events orchestrated by several types of cells interacting with each other and with the extracellular matrix. The bone cells include 1) osteoblasts, 2) osteoclasts, 3) osteocytes, and 4) bone-living cells. Osteoblasts are responsible for production and mineralization of the bone matrix. Osteoclasts maintain the bone matrix and are responsible for bone resorption. Cell attachment, proliferation, and differentiation are important activities involved in bone formation. The osteoblast (bone-forming) cells attach, proliferate, and differentiate, leading to production of matrix proteins that include collagen (mostly type I), osteopontin (OSP), bone sialoprotein (BSP), osteonectin (ONN), osteocalcin (OSC), fibronectin (FN), and BMPs before mineral deposition.Citation5–Citation8 BMPs and matrix proteins induce bone formation in vitro and in vivo. Collagen, OSP, and ONN have been shown in vitro to nucleate apatite formation and also inhibit or modulate HA crystal growth. As the primary structural support of the mammalian body, bones are constantly being remodeled in response to the applied stresses. This continuous regeneration of bone likely serves to repair stress damage and prevent excessive aging.Citation9 In addition to its structural functions of load bearing, internal organ protection, and muscle support, bone is also important for the tight regulation of calcium ion concentration through the ongoing resorption and formation of new mineral. There are two cell types that are responsible for the formation, removal, and maintenance of bone tissue. Osteoclasts are multinuclear, macrophage-like cells that reabsorb bone.
Important physicochemical properties of bone include 1) interconnecting porosity, 2) biodegradability, 3) bioactivity, 4) osteoconductivity, and 5) osteoinductivity. Pore size ranges from 10 to 50 μm and 100 to 300 nm in cortical bone and 200 to 600 μm in trabecular bone. The size and interconnection of bone porosity are essential for vascularization, diffusion of nutrients, and cells and tissue ingrowths. The bone architecture and composition allow cell attachment, migration, proliferation, and differentiation, promoting bone formation, repair, and regeneration.
To better understand the complex bone architecture, several hierarchical models have been proposed. Weiner and Wagner have identified seven discrete levels of hierarchical organization in bone (), which we describe here.Citation10 In their model, bone is considered as a family of materials with the mineralized collagen fiber as the primary building block for subsequent higher-order architectures.
The structure of bone differs greatly among different locations in the skeleton, but the basic nanoscale structure consisting of mineralized collagen remains the same throughout.Citation10 Mann has also presented a similar structural hierarchy containing six levels.Citation11
The first level of hierarchy consists of the molecular components: water, HA, collagen, and other proteins. The crystals of HA are plate-shaped and are among the smallest known biological crystals (30–50 nm long, 20–25 nm wide, and 1.5–4 nm thick, depending on the study) ().Citation12
Table 1 Characterization of bone crystallites using different analytical methods
In previous studies, apatite needles were observed,Citation13 but other studies suggest that apatite platelets are the dominant morphology and that the apparent needles are most likely to be HA platelike morphology viewed edge-on.Citation14 NCPs are also present but make up 10% or less of the total protein content in the bone matrix. The specific functions of the NCPs are still not completely understood. In addition to influencing crystal nucleation and growth, NCPs also play roles in cell signaling and ion homeostasis.Citation15
The second level is formed by the mineralization of collagen fibrils. This HA-reinforced fibril composite is described by Weiner and Wagner as containing parallel platelike HA crystals with their c-axis aligned with the long axis of the fibril.Citation10,Citation16 HA mineral platelets The location of these crystals in the fibril was demonstrated in a study by Traub et al showing that mineralized collagen fibrils had the same banded pattern as negatively stained collagen fibrils.Citation14 This indicated that mineral is concentrated in the hole zones of the fibril. It was proposed that these were arranged in parallel like a stack of cards within the interstices of the fibril. Olszta et al concluded from electron diffraction studies that that the mineral plates are not quite as ordered as previously assumed.Citation17 This imperfect arrangement of nearly parallel crystals has been supported by recent small-angle X-ray scattering (SAXS) and transmission electron microscopy (TEM) data from Burger et al.Citation18
The third level of hierarchy is composed of arrays of these mineralized collagen fibrils. These fibrils are rarely found isolated, but are rather almost always associated as bundles or other arrangements, often aligned along their long axis.
The fourth level is the patterns of arrays that are formed. These include parallel arrays, woven arrangements, plywoodlike structures, and radial arrays like those found in dentin.Citation19 Cylindrical structures called osteons make up the fifth level. Osteons are formed with significant cellular activity and remodeling; osteoclasts reabsorb bone and form a tunnel, and osteoblasts subsequently lay down lamellae in stacked layers until only a small channel (Haversian canal) is left behind. These channels serve as a conduit for nerves and blood supply to the bone cells.
The sixth level of bone organization is the classification of osseous tissue as either spongy (trabecular or cancellous) or compact (cortical). Cancellous bone is extremely porous (75%–95% porosity), providing space for marrow and blood vessels, but has much lower compressive strength. Cortical bone is the dense outer layer (5%–10% porosity), allowing many of the support functions of bone. Therefore, the mechanical properties of cortical bone represent the benchmark for synthetic bone.Citation20
The seventh level is simply the whole bone on the macroscopic scale, incorporating all of the lower levels of hierarchy. There are 206 bones in the adult human skeleton, the structure of which depends on their location and function.
Tooth
The mammalian body contains numerous mineralized tissues. The tissue with the most robust mechanical properties is enamel. Enamel is the hardest material formed by vertebrates and is the most highly mineralized skeletal tissue present in the body.Citation21 Mature enamel is composed of 95%–97% carbonated HA by weight with <1% organic material. The high degree of mineralization makes enamel a fascinating model for understanding the fundamental mineralization processes and processes that occur within an extracellular matrix. It is distinct from bone in terms of architecture, pathology, and the biological mechanisms mediating its formation. Understanding the biological formation of different mineralized structures could lead to innovative approaches toward engineering novel scaffolds providing new therapeutics. Additionally, unlike other biomineralized tissues, such as bone and dentin, mature enamel is acellular and does not reabsorb or remodel. As a result, enamel regeneration cannot occur in vivo following failure and is therefore an attractive target for future biomimetic and therapeutic approaches.
Enamel formation, or amelogenesis, is a highly regulated process involving precise genetic control as well as protein– protein interactions, protein–mineral interactions, and interactions involving the cell membrane. The mammalian tooth is made up of four distinct structures: enamel, dentin, pulp, and cementum ().Citation22
Figure 2 Hierarchical architecture of mammalian enamel. Enamel (E) is the outermost layer at the crown of the tooth and resides above the dentin (D). The pulp (P) contains nerves and blood vessels, while the cementum (C) is the outermost layer of mineralized tissue surrounding the root of the tooth allowing the tooth to be anchored to the jawbone through the periodontal ligament (PDL). The bulk image depicts the enamel organ, the transition across the dentin–enamel junction, and the dentin below. On the mesoscale level, prismatic enamel consisting of weaving of rods (or prisms) that range from 3 to 5 μm in diameter can be visualized. Upon further magnification, the micrometer scale shows the composition of a single rod. The nanometer scale reveals a highly organized array of individual HA crystallites (~30 nm thick, 60 nm wide, and several millimeters in length), which are preferentially aligned along the c-axis.
Copyright (c) 2008, MRS Bulletin Reproduced with permission from Tamerler C, Sarikaya M. Molecular biomimetics: genetic synthesis, assembly, and formation of materials using peptides. MRS Bull. 2008;33(5):504–510.
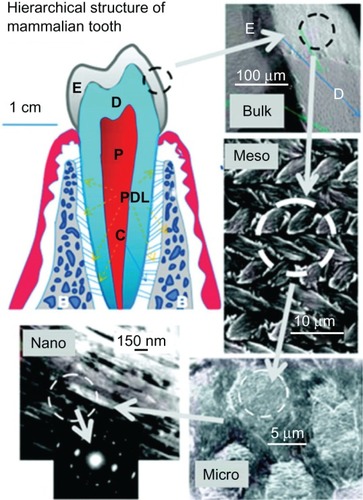
The pulp contains nerves, blood vessels, fibroblasts, and lymphocytes, while the mineralized organs of the tooth include enamel, dentin, and cementum. Enamel makes up the uppermost 1–2 mm of the tooth crown and contains a high mineral content, giving it a high modulus but also making it susceptible to cracking. Dentin lies below the enamel and is tougher, forming the bulk of the tooth and absorbing stresses from enamel, preventing its fracture.Citation23 The composition of dentin is similar to that of bone. The cementum is the mineralized layer that surrounds the root of the tooth covering the dentin layer and some of the enamel layer. The cementum allows the anchoring of the tooth to the alveolar bone (jawbone) through the periodontal ligament (PDL). The primary function of the teeth is mastication of food; however, some species use them to attack prey and for defense. It also faces the lifelong challenge of maintaining robust mechanical properties in a bacteria-filled environment.
The enamel and dentin tissues give rise to a tough, crack-tolerant, and abrasion-resistant tissue through their unique architectures and mineral compositions. Enamel is highly patterned and consists of organized interweaving bundles of crystallites (called rods or prisms). It has a higher reported toughness than that of crystalline HA, indicating that the organization of the crystallites is essential for enamel function.Citation24 Because of the high mineral content and minimal organic, enamel is brittle. Interestingly, the architecture of the enamel crystallites can defect a propagating crack preventing it from reaching the dentin–enamel junction (DEJ), which also has been shown to resist delamination of the tissues, despite their differences in composition.Citation25 The mechanical properties of enamel, dentin, and the DEJ are not completely understood and are a significant matter of research. Understanding the properties of these tissues could serve to motivate further engineering of more robust dental materials as well as to inspire fabrication of nonbiological materials.
Similar to bone, enamel has a complex architecture, which can be broken into several hierarchical levels from the nanoscale to the macroscale.Citation26 On the nanoscale, the protein– protein and protein–mineral interactions in the presence of supersaturated ions create a highly organized array of HA crystallites that grow preferentially along the c-axis.Citation27 The sizes of these crystallites depend on the stage of the mineralization. The crystallites grow primarily in length during the secretory stage and continue to grow in width and thickness during the maturation stage. The assembly of amelogenin has been shown to be crucial for the proper development of enamel crystallites. Disruption of the assembly alters formation on the nanoscale, subsequently affecting larger-length scales and giving rise to a diseased or malformed enamel phenotype.
On the mesoscale level, there are three main structural components: the rod, the interred, and the aprismatic enamel. The main component of enamel on the mesoscale includes rods, which are bundles of aligned crystallites that are ‘woven’ into intricate architectures that are ~3–5 μm in diameter, as seen in .Citation28
Figure 3 Scanning electron microscopy image of enamel-carbonated hydroxyapatite. ‘Spaghetti-shaped nanocrystals’ arranged in bundles oriented along three differentdirections (scale bar 10 μm).
Copyright (c) 1989, Oxford University Press. Reproduced with permission from Lowestan HA, Weiner S. On Biomineralization. New York: Oxford University Press; 1989.
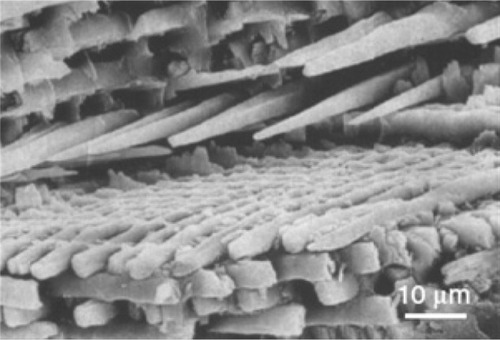
The second structural component of the enamel matrix is the interrod (or interprismatic) enamel, which surrounds and packs between the rods. The difference between the rod and the interrod is the orientation of HA crystals; the rod contains aligned crystallites, whereas the mineral in the interrod is less ordered. These structures coalesce to form the tough tissue of enamel, which can withstand high forces and resist damage by crack defection. The third structure, aprismatic enamel, refers to the structures containing HA crystals that show no mesoscale or macroscale alignment. The macroscale architecture includes specific zones of enamel that have unique characteristics, which contribute to the whole tissue. The enamel adjacent to the DEJ exhibits a gradual transition from dentin to enamel. Aprismatic regions of enamel have been proposed to be primitive areas of the tooth serving as a toughening mechanism due to their flexible nature.Citation28,Citation29 Several authors have identified these aprismatic areas to be located adjacent to the DEJ and at the incisal surface of both deciduous and permanent human enamel.Citation30–Citation32 The Tomes’ process, a unique structure present at the secretory pole of an enamel-forming cell, is responsible for aligned mineral formation in the prismatic enamel. The absence of this process may give rise to the aprismatic zone in the tooth.Citation33
Biogenic hydroxyapatite
The bone mineral was identified as an apatite based on its similarity with the X-ray diffraction profiles of mineral hydroxyapatites and their similarities in composition with each other (principally calcium and phosphate ions).Citation34–Citation37 The mineral of teeth and bones was idealized as stoichiometric calcium hydroxyapatite, Ca10(PO4)6(OH)2.Citation38 However, biologic hydroxyapatites contain minor and trace elements and are therefore not pure HA. The most important minor elements are carbonate (CO3), magnesium (Mg), and sodium (Na). Systematic studies on synthetic carbonate-substituted hydroxyapatites and biologic hydroxyapatites using combined analytical methods (X-ray diffraction, infrared spectroscopy, and chemical analyses)Citation39–Citation42 led to the conclusion that they should be considered as carbonate-hydroxyapatite.Citation43–Citation45 Detailed studies indicated that carbonate ions could be located in the two anionic sites of the apatite structure: in PO43− sites (type B carbonated apatite) and OH− sites (type A carbonated apatite). Bone hydroxyapatites were believed to correspond essentially to type B carbonated apatite, whereas enamel contained both type A and B carbonate. The fraction of type A carbonate in dental enamel was evaluated at about 10% of the total carbonate content using infrared spectroscopy.Citation46 The use of carbonated apatite as a model for biological calcifications of hard tissues of vertebrates is nowadays accepted. The variability of the composition of apatite minerals and their mode of formation however needed further investigation.Citation47
Bone apatite crystals are irregularly shaped platelets of variable lengths and widths (30–45 nm) and thickness (average about 5 nm) oriented with their c-axis parallel to one another and lie along the collagen fibrils.Citation10 Biologic hydroxyapatites of enamel have considerably larger crystal size (about 2000 nm) compared to that of either bone or dentin apatite, as indicated by the well-defined diffraction peaks in the XRD profile of enamel apatiteCitation43 and much broader diffraction peaks of either bone or dentin apatite ().
Figure 4 X-ray diffraction profiles of biologic apatites from A) bone, B) dentin, and C) enamel. The sharper diffraction peaks in C compared to either B or A indicate that enamel apatite crystals are much larger compared to either bone or dentin apatite crystals.
Copyright (c) 2008, American Chemical Society. Reproduced with kind permission from LeGeros RZ. Calcium phosphate-based osteoinductive materials. Chem Rev. 2008;108(11):4742–4753.
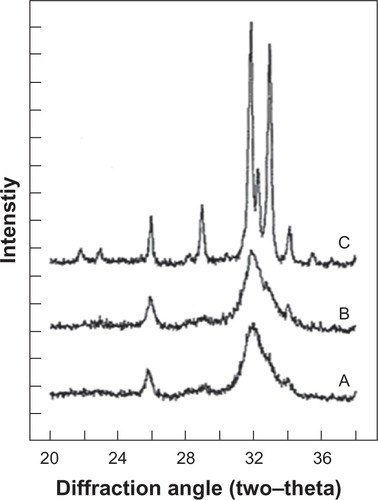
The concentrations of Mg and CO3 in enamel apatite are much lower than those in either dentin or bone apatite.Citation43,Citation48 The mineral phase of some fish enameloids (eg, shark enameloid) has fluoride (F−) ions replacing the hydroxyl (OH−) groups in the apatite structure. Differences in composition affect the lattice parameters of the apatite hexagonal structure (a- and c-axis dimensions), crystal size, and solubility of the biological and synthetic apatites, as demonstrated in the differences in crystallite size and solubility of the biologic apatites in enamel, dentin, and bone. For example, substitution of CO32− (for PO43−) or Mg2+ or Sr2+ (for Ca2+) in the apatite structure causes a decrease in crystallite size and an increase in solubility, while incorporation of fluoride ions (F− for OH− substitution in the apatite structure) causes an increase in crystallite size and decrease in solubility. Detailed information on the chemical composition of the most important human normal calcified tissues is given in .Citation49,Citation50
Table 2 Comparative composition and structural parameters of inorganic phases of adult human calcified tissues
Preparation of biomimetic hydroxyapatite nanocrystals
Synthetic nanohydroxyapatite exhibits good properties as a biomaterial, such as biocompatibility, bioactivity, osteoconductivity, direct bonding to bone, etc, exciting the applications of HA in the fields of bone tissue engineering and orthopedic therapies.Citation51 Many different methodologies have been proposed to prepare nanosized and/or nanocrystalline structures.Citation52–Citation55 These are wet chemical precipitation,Citation56–Citation60 sol–gel synthesis,Citation61–Citation64 coprecipitation,Citation62–Citation67 hydrothermal synthesis,Citation68–Citation70 mechanochemical synthesis,Citation71 microwave processing,Citation72–Citation74 vapor diffusion,Citation75 silica gel template,Citation76 emulsion-based syntheses,Citation77 and several other methods by which nanocrystals of various shapes and sizes can be obtained.Citation78–Citation80 In general, the shape, size, and specific surface area of the apatite nanocrystals appear to be very sensitive to both the reaction temperature and the reactant addition rate. Hydroxyapatites with different stoichiometry and morphology have been prepared and the effects of varying powder synthesis conditions on stoichiometry, crystallinity, and morphology have been analyzed. The effects of varying the concentration of the reagents, the reaction temperature and time, initial pH, aging time, and the atmosphere within the reaction vessel have also been studied.Citation81 In order to optimize their specific biomedical applications, especially drug delivery function, the physical and chemical features that should be tailored in synthetic biomimetic HA are dimensions, porosity, morphology, and surface properties.Citation82,Citation83
It is well known that natural minerals in bone are homogeneous platelike HA crystals with widths of 15–30 nm and lengths of 30–50 nm, and in enamel are rodlike HA crystals with diameters of 25–100 nm and lengths of 100 nm to microns. The study of higher-level biomineralization and biomimetic assembly involves the search for an advanced method so that the synthesis of HA nanocrystals can be accurately controlled.Citation84 Although each of the reported approaches to produce nanosized apatites has both scientific as well as practical relevance, very little attention has been paid to the physical and chemical details involved in the careful control of the particle size distribution and particle shape. Indeed, in the case of particle size distribution, most of the reported ways to synthesize nanosized apatites really produce a particle mixture with a wide size distribution from tens to hundreds of nanometers. For example, the size-controlled synthesis of materials can be achieved by using limited reaction spaces. Nanosized reaction vessels are selected, such as reverse micelles, microemulsions, vesicles, ferritin and viroid cages, lipid bilayer films, bacterial threads, etc.Citation85–Citation87 Among these methods, reverse micelles and microemulsions have been applied to synthesize nano-HA.Citation88 In some cases, special polymers can be used as spatial reaction vessels for production of HA.
Applications
Synthetic nanohydroxyapatite for orthopedic applications
Calcium phosphate ceramics have been used in orthopedics for more than 30 years—thanks to their excellent biocompatibility—and are still, today, very popular implant materials for diverse orthopedic clinical applications.Citation89 Due to the rapid development of nanotechnology, the potential of nano–calcium phosphates has received considerable attention. Here, we discuss recent achievements of nano–calcium phosphate ceramic matrices, which are utilized for bone implants. Generally, nano-HA has been designed for use in nonloaded parts, due to its unsatisfactory mechanical properties. NanOss® bone void filler from Angstrom Medica (Woburn, MA, USA)Citation90 is considered to be the first nanotechnologic medical device to receive clearance by the US Food and Drug Administration (FDA) in 2005. It is prepared by precipitating nanoparticles of calcium phosphate in an aqueous phase, and the resulting white powder is compressed and heated to form a dense, transparent, and nanocrystalline material. According to the company, NanOss® (Angstrom Medica) duplicates the microstructure, composition, and performance of human bone and possesses high osteoconductivity.
Calcium phosphate bone cements
A new concept in the treatment of bone defects was introduced with bone cements based on calcium phosphates, which have many advantages including their self-setting form, besides excellent biocompatibility and bone-repair properties.Citation91,Citation92 Cement properties, such as setting time, degradation speed, porosity, or mechanical behavior, can be controlled by changing the components of the cement, including type or amount of calcium phosphate and additives.Citation93 Nano–calcium phosphate has been generally selected as the major component because of its accepted improved sinterability, enhanced densification, and better bioactivity than coarser crystals.Citation94 Through some improvements of cements based on nano-HA in terms of rheological, exothermal, and mechanical behavior, etc, some of them are used in clinical treatments, such as Ostim® (aap Implantate AG, Berlin, Germany), an injectable bone matrix in paste form which received CE (Conformité Européenne) approval in 2002; it is composed of synthetic nanoparticulate hydroxyapatite and can be used in metaphyseal fractures and cysts, acetabulum reconstruction, and periprosthetic fractures during hip prosthesis exchange operations, osteotomies, filling cages in spinal column surgery, etc. Moreover, cements based on calcium phosphate can be used as convenient drug carriers for antibiotics, anti-inflammatory drugs, BMPs, or transforming growth factors-beta (TGF-β).Citation95 Unlike other carriers for which drugs are usually adsorbed on the surface, the drugs can be incorporated with the cement throughout the whole material volume, by adding them into one of the two cement phases. This fact can facilitate the release of drugs for more prolonged times. This aspect gives great potential to this type of materials for controlled drug delivery in target sites of the skeletal system.
In the bulk form, many different calcium phosphate ceramics are in the market for the treatment of bone defects as a result of orthopedic removal, bone tumor extraction, complicated fracture, etc.Citation96 In these cases, the site of the bone defect has to be filled with dense or porous bulk biomaterials, which can offer support for growth of new bone on/in it to avoid growth of fibrous tissue. The materials should be biocompatible, best of all, bioactive, and biodegradable. Chemically, synthetic bulk biomaterials are usually based on calcium phosphates. With the rapid development of nanotechnology, nanostructured HA may find use in this field. For example, Cui et alCitation97 developed nano-HA/collagen (NHAC) which mimics the HA nanocrystal-type I collagen nanostructure of natural bone, which can be part of bone metabolism instead of being a permanent implant. Besides, nanocomposites containing HA and chitosan,Citation98 HA–silver composites,Citation99 fluorapatite/collagen composites,Citation100 and nanocrystalline yttria-stabilized, zirconia-reinforced HACitation101 have been developed for some special functions, for instance, antimicrobial activity, improvement of structural stability and cellular responses, and enhancement of strength and toughness. Due to process difficulties and the poor mechanical properties of bulk HA, its applications are currently confined to non-load-bearing implants and porous bodies/scaffolds. Porous 3-D nanocomposites of HA and collagen/polymer mimic natural bone in composition and microstructure and can be employed as a matrix for the tissue engineering of bone.Citation102
HA-coated metallic implants
However, the fracture toughness values of artificial calcium phosphate materials are not as high as those of human cortical bone. Therefore, calcium phosphate materials cannot be used at high-load sites such as femoral and tibial bones. Some biomedical metals like cobalt chromium alloys, titanium, and its alloys are widely used in clinical treatments. However, biomedical metals are usually bioinert, and cannot bond to bone directly in vivo. In order to improve biological properties of biomedical metals, nanostructured HA is generally used as a coating material to accelerate bone growth and enhance bone fixation.Citation103 Different methods of HA coating commercially utilized are pulsed laser deposition and thermal plasma spraying, which can offer a strong link between the metal matrix and the HA coating.Citation104 Other approaches include dip coating, electrophoretic deposition, sol–gel method, etc, which can be used to coat complicated and porous devices along with the incorporation of biologically active substances, such as proteins or antibiotics, into the coating, but with weak bonding force. Because of its advantages over conventional coatings, a biomimetic coating has been suggested.Citation105 The coating is composed of uniform nanocrystalline HA and is demonstrated to be capable of conducting bone formation and promoting direct osseointegration with juxtaposed bone.
Porous HA bioceramics
Porous HA biomimetic in simulating spongy bone morphology (porosity varying from a microporosity >1 μm to a macroporosity ranging from 300 to 2000 μm) has been prepared using various technologies to control pore dimension, shape, distribution, and interconnections. However, HA ceramics processed by high-temperature treatment present a significant reduction of bioreactivity and growth kinetics of new bone due to the lack of resorbability.Citation106 Porous bioceramics with a low degree of crystallinity and an appreciable bioresorbability have been obtained using synthetic methods at lower temperatures.Citation82,Citation107 Colloidal processing,Citation108 starch consolidation,Citation109 gel casting, and foam outCitation110 have allowed to produce bioceramics with a bimodal distribution of the pore size that can be tailored as a function of the sintering conditions. However, the low resorbability of sintered HA ceramics appears useful when they have to be implanted with a defined 3-D form. In these cases, the high porosity of HA ceramic induces bone formation inside the implant increasing HA degradation, but without reaching its complete resorption and allowing the implant to conserve its crystalline architecture.
Different types of calcium phosphate ceramics are available, such as hydroxyapatite, beta-tricalcium phosphate, biphasic calcium phosphate, amorphous calcium phosphate, carbonated apatite, or calcium-deficient HA.Citation111 Porous HA can be synthesized by a hydrothermal method directly from natural sea coralsCitation112 and cattle fish bones,Citation113 in fact, HA phase replaces the aragonite one while preserving its natural porous structure. Coralline apatite could be improved by a sol–gel-derived nanocoating layer to cover meso- and nanopores. This new material can be utilized for bone graft applications where high strength and longevity are required.Citation114,Citation115 The interconnected network of pores promotes bone ingrowth, but also allows bioceramics to be utilized as drug delivery agents, by inserting different bioactive molecules or by filling the macro- and micropores with gelatin, which can act as delivery agent of bioactive molecules.Citation116
Many studies have demonstrated that hydroxyapatite ceramics can be used to deliver steroids, antibiotics, proteins, hormones, and anticancer drugs. Porous ceramics closely mimicking spongy bone morphology have been synthesized by impregnation of cellulosic sponges with poorly crystalline HA water suspension.Citation117
Drug delivery bone grafts
These porous ceramics have been tested as controlled drug delivery bone grafts to evaluate the fundamental parameters that control release kinetics. A theoretical approach, based on the use of the finite element method, was adopted to describe the ibuprofen–lysine and hydrocortisone Na-succinate release kinetics, comparing the numerical results with the experimental ones ().Citation118 An alternative approach to drug delivery function in order to utilize HA ceramic porosity is represented by bone tissue engineering which uses cells seeded onto macropores of these HA scaffolds to promote bone growth and by filling the macro- and micropores with gelatin, which can act as cell nutrient and/or delivery agent of bioactive molecules. When powder bioceramics are used for bone filling applications, they are usually mixed with a polymeric carrier matrix to avoid migration out of the implant region. Both nonabsorbable [poly(methyl methacrylate),Citation119 polyethylene,Citation120 and polysulfone] and biodegradable [poly(lactic acid),Citation121 polyglycolic acid, collagen, cellulose, and starchCitation122,Citation123] polymeric matrices can be used, even if the nonbiodegradability drastically reduces the HA crystal bioactivity.
Silicon carbide grafts
Following the biomimetic approach, inspiring to nature, natural wood templates have been selected as a starting point to obtain open-pore geometries with high surface area and microstructure allowing cell ingrowth and reorganization and providing the necessary space for vascularization.Citation124,Citation125 The alternation of fiber bundles and channel-like porous areas makes the wood an elective material to be used as template in starting the development of new bone substitute biomaterials by an ideal biomimetic hierarchic structure.Citation126 Particularly, Si/SiC wood-derived structures have been optimized to be employed as bioinert bone scaffolds. During the last decade, biomorphic silicon carbide (BioSiC) prepared by Si vapor or by Si-melted reactive infiltration of carbon templates, previously obtained by pyrolysis of different kinds of wood, has received great attention.Citation126–Citation128 The reason for this is not only due to wood’s good thermomechanical properties, but also to its large availability, renewability, low cost, and very low environmental impact.Citation129 The morphological characteristics of BioSiC closely resemble that of the different kinds of wood utilized for its preparation. In fact, the macroscopic organization and structural hierarchy arrangement of cells and channels in coniferous, oak, bamboo, and many other woods appear greatly different till the nano-/micro-size level.Citation130
BioSiC is a siliconized carbon material produced by reactive infiltration of molten Si into a carbon template. It is obtained from wood pyrolysis and represents a novel kind of porous ceramic composed of elongated tubular cells with a diameter of a few to hundreds of micrometers, preferentially aligned with the axis of the tree trunk. BioSiC cannot be prepared by traditional technologies in porous ceramics manufacturing; it represents a new generation of light, tough, and strong material for biomedical applications. Because of good biocompatibility and biological response, the porous material BioSiC is considered to be a bone filler and substitute in orthopedic, odontology, dental, and maxillofacial implantation. BioSiC has been used as a support of suspended particles to realize a nickel/silicon carbide nanocomposite by electrodeposition and heat treatment ().Citation125,Citation131,Citation132
Figure 6 Scanning electron microscopy image of wood silicon carbide. Transversal cross-sectioned sample A) before and B) after electrochemically assisted biomimetic hydroxyapatite/collagen surface deposition. Copyright (c) 2010, Wiley-VCH Verlag GmbH & Co. KGaA. Reproduced with permission from Lelli M, Foltran I, Foresti E, et al. Biomorphic silicon carbide coated with an electrodeposition of nanostructured hydroxyapatite/collagen as biomimetic bone filler and scaffold. Adv Eng Mater. 2010;12(8):B348–B355.
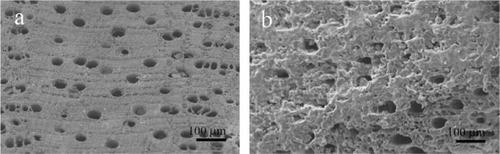
However, all these BioSiC ceramic structures derived from the conversion of wood exhibit a biomimetism limited to inner morphology and organized structure, but their chemical composition and surface bioactivity are far from the biomimetic concept which could be carried out not only as structure, morphology, bulk, but also like surface chemical– physical properties and bioreactivity.
An innovative hybrid biomaterial, like bone substitute and bone tissue engineering scaffold, with the possibility of synergistically joining the porous bioinspired morphology and mechanical property of BioSiC has been recently synthesized.Citation133 BioSiC exhibiting the surface bioactivity of a nanostructured hydroxyapatite/collagen biomimetic coating has been recently obtained by the electrochemically assisted hydroxyapatite/collagen coating deposition ().
Hydroxyapatite bone scaffolds characterized by highly organized hierarchical structures have been recently obtained by chemically transforming native woods through a sequence of thermal and hydrothermal processes. The whole chemical conversion has been carried out through five chemical steps from native wood to porous hydroxyapatite: 1) Pyrolysis of ligneous raw materials to produce carbon templates characterized by the natural complex anisotropic pore structure. 2) Carburization process by vapor or liquid calcium permeation to yield calcium carbide. 3) Oxidation process to transform calcium carbide into calcium oxide. 4) Carbonation by hydrothermal process under CO2 pressure for the further conversion into calcium carbonate. 5) Phosphatization process through hydrothermal treatment to achieve the final hydroxyapatite phase ().
Figure 7 A processing scheme to convert wood hierarchical structures to new biomimetic hydroxyapatite scaffolds. Copyright (c) 2009, Royal Society of Chemistry. Tampieri A, Sprio S, Ruffini A, Celotti G, Lesci IG, Roveri N. From wood to bone: multi-step process to convert wood hierarchical structures into biomimetic hydroxyapatite scaffolds for bone tissue engineering. J Mater Chem. 2009;19:4973–4980.
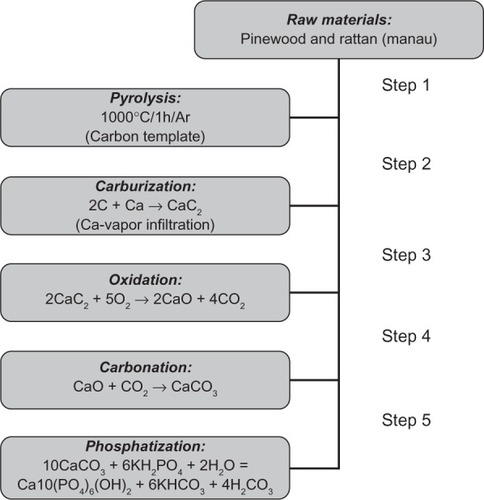
The five steps of the phase transformation process have been set up in order to achieve total phase conversion and purity, maintaining the original native microstructure. An innovative biomimetic apatite hierarchically structured in parallel fastened hollow microtubules has been synthesized, structurally characterized, and proposed as new inorganic biomorphic scaffold providing a biomimetic nanostructure surface for fascinating bone engineering applications.Citation134 The SEM image reported in demonstrates that the structure anisotropy typical of the native woods was preserved on the macroscale, exhibiting, in the case of rattan, pore sizes in the range 100–300 μm, revealing an ordered fastening of parallel microtubes 100–150 μm long and 15–30 μm wide with a hollow core of about 10–25 μm in diameter, organized like the cell morphology of the natural wood used as the starting template for its synthesis. SEM image reported in of the newly formed HA surface morphology shows typical needlelike nuclei grown on the surface, proving the concomitant occurrence of a dissolution/precipitation process at submicron level, in agreement with hypotheses previously reported. This surface nanostructured morphology of the unidirectional, fastened hollow HA microtubules allows biological systems like cells to utilize biomorphic scaffolds on the micrometer level which are also biomimetic for composition and structure on the nanometer scale.
Figure 8 Detailed scanning electron microscopy images of pinewood-derived hydroxyapatite. A) Microstructure of wood-derived parallel fastened hydroxyapatite microtubes. B) Typical needlelike HA nuclei grown on the microtube surface; the inset shows a higher magnification of B. Copyright (c) 2009, Royal Society of Chemistry. Tampieri A, Sprio S, Ruffini A, Celotti G, Lesci IG, Roveri N. From wood to bone: multi-step process to convert wood hierarchical structures into biomimetic hydroxyapatite scaffolds for bone tissue engineering. J Mater Chem. 2009;19:4973–4980.
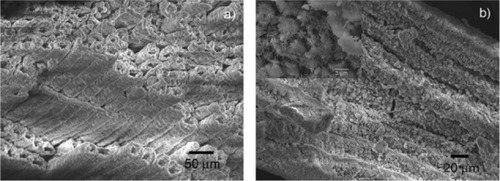
Synthetic nanohydroxyapatite for drug delivery
Over the past few decades, the rise of modern pharmaceutical technology and the amazing growth of the biotechnology industry have revolutionized the approach to drug delivery systems development.Citation135 During the past three decades, complex formulations that control the rate and period of drug delivery and that target specific areas of the body for treatment have become increasingly common.
Addressing this complexity, coupled with the explosion of new and potential treatments resulting from discoveries of bioactive molecules and gene therapies, pharmaceutical research is facing challenges in not only the development of new treatments, but also the mechanisms through which we administer them.Citation136–Citation139
To optimize the benefits, the design of a controlled release system requires simultaneous considerations of several factors,Citation140 such as the chemical–physical properties of the drug, the route of administration,Citation141 the nature of the delivery vehicle, the mechanism of drug release, the potential for targeting, and biocompatibility. Because of the extensive interdependency of those factors, it is not easy to establish a sequential process for designing a controlled drug delivery system,Citation142 which can bring both therapeutic and commercial value to health care products.Citation143 Many drug delivery technology companies enjoy a good return on their investments in the form of increased revenues and market share, and it is a very fast-growing segment of the economy.Citation138,Citation139 It is clear that significant advances will only be made through multidisciplinary teams that utilize the latest advances in the biological, chemical, physical, and engineering sciences.
Drug delivery technologies are classified according to the route through which a drug is administered into the body. In the oral route, a drug is taken by mouth into the gastrointestinal (GI) tract to be absorbed. Other important routes include intravenous injection, intramuscular injection, subcutaneous injection, pulmonary, ocular, buccal (through the internal wall of the cheeks), sublingual (under the tongue), nasal, vaginal, rectal, transdermal, and implanted (inside a body cavity). Highly sophisticated drug delivery systems are being developed to exert their effects after they are administered into the body, in particular, into the systemic circulation. They usually consist of particles in the size range of nanometers that have unique combinations of capacities such as controlled release and biological site-specific targeting.
Furthermore, one of the most interesting things is the implantable drug delivery systems (IDDS). These techniques have the advantage of maintaining a steady release of drug to the specific site of action so that they are safer and more reliable. IDDS can be classified into three major categories: biodegradable or nonbiodegradable implants, implantable pump systems, and the newest atypical class of implants.Citation144 Biodegradable and nonbiodegradable implants are available as monolithic systems or reservoir systems. The release kinetics of drugs from such systems depend on both the solubility and diffusion coefficient of the drug in the polymer, the drug load, as well as the in vivo degradation rate of the polymer in the case of the biodegradable systems.Citation145 The third IDDS system, the atypical class, includes recently developed systems such as ceramic hydroxyapatite antibiotic systems used in the treatment of bone infections, intraocular implants for the treatment of glaucoma, and transurethral implants utilized in the treatment of impotence. The major advantages of these systems include targeted local delivery of drugs at a constant rate, fewer drugs required to treat the disease state, minimization of possible side effects, and enhanced efficacy of treatment. Also, these forms of delivery systems are capable of protecting drugs which are unstable in vivo and that would normally require frequent dosing intervals. Due to the development of such sustained-release formulations, it is now possible to administer unstable drugs once a week to once a year that in the past required frequent daily dosing.
Preliminary studies using these systems have shown superior effectiveness over conventional methods of treatment. However, some of the most recently discovered implants are in the early developmental stages, and more rigorous clinical testing is required prior to their use in standard practice.Citation145
Hydroxyapatite is known for its binding capability to a wide variety of molecules.Citation146,Citation147 The interaction between proteins and hydroxyapatite surfaces plays an important role in many applications, namely medicine, pharmacy, nanodevices, biosensors, and bioengineering.Citation148 In particular, in the biomaterials field, its study is fundamental to learn more about the biomineralization process in vivo,Citation149 to test the material performance in biological environment,Citation150 or to check its ability as inorganic carrier for biomolecules.Citation151,Citation152 Although protein adsorption on solid surfaces has been widely studied for decades, its mechanisms are still far from being fully understood. This is because adsorption of protein on a solid surface is a complicated process consisting of many events, such as conformational changes in protein molecules and coadsorption of ions. In particular, the protein conformational change, which results in entropic gain, is thought to be important for driving forces to the protein surface adsorption.Citation153 In evaluating the interaction of HA with serum and BMPs,Citation154–Citation156 the interaction of HA with myoglobinCitation157 and bovine and human serum albuminsCitation158 has been recently clearly elucidated. Actually, these proteins can be considered as model proteins because of their well-known structure and properties.
It is an attractive goal to develop new biomimetic apatite nanocrystals for potential use in bone implantation, which in addition function as a local targeted delivery system for anticancer and antimetastatic drugs with controlled release properties. For example, the application of such a material in the chemotherapeutic treatments of osteosarcoma could result in tumor inhibition accompanied by low levels of systemic toxicity. As drug carriers, calcium phosphate nanoparticles have some advantageous properties.Citation159 They are dissolved at low pH (around 4), eg, in lysosomes after the cellular intakeCitation160 or in the environment of solid tumors, thereby releasing incorporated drugs or biomolecules. Their size can easily be controlled by stabilizing agents, such as polymers or nucleic acids. The nanoparticles can be made to fluoresce by the incorporation of lanthanide ions,Citation161,Citation162 and they can also act as carriers for different drugs, eg, insulin,Citation163 cisplatin,Citation164 or ceramide.Citation165 Liu et al have shown by in vivo experiments that calcium phosphate nanoparticles, carrying a suicide gene, can treat nasopharyngeal carcinoma.Citation166
The adsorption and release of bioactive molecules are strongly affected not only by the chemical properties of the drug molecule, but also by the chemical and structural characteristics of the HA substrates. The adsorption and release of cisplatin, alendronate, and di(ethylenediamineplatinum) medronate have been investigated using two biomimetic synthetic hydroxyapatite nanocrystal materials with either plate-shaped or needle-shaped morphologies and with different physical and chemical surface properties.Citation167 These bioactive molecules were chosen in order to compare the behavior of metal-based drugs to that of a classical organic drug (alendronate), evaluating the effect of the overall charge of the drug molecule in influencing the drug affinity for apatite nanocrystals with variable structural and chemical properties. The HA surface area and surface charge (Ca/P ratio), as well as the charge on the adsorbed molecules and their mode of interaction with the HA surface, influence the adsorption and release kinetics of the three drugs investigated. The results demonstrated that HA nanocrystals and antitumor drugs can be selected in such a way that the bioactivity of the drug–HA conjugate could be tailored for specific therapeutic applications.
The adsorption of two different platinum complexes with cytotoxic activity, {ethylenediamine platinum(II)}-2-amino-1-hydroxyethane-1,1-diyl-bisphosphonate (A) and bis-{ethylenediamine platinum(II)}medronate (B), on the synthesized biomimetic nanocrystals has been investigated.Citation168 Both complexes contain a geminal bisphosphonate but, in addition, complex A contains a charged ammonium group. This structural difference dramatically affects the affinity of A and B toward HA nanocrystals, complex A having a greater affinity for calcium phosphate nanocrystals. The release profiles of the platinum complexes from the HA nanoparticles follow an inverted trend (complex B > complex A) when compared with the adsorption process. Most probably, the less effective desorption in the case of complex A could be due to the aminic group present on the bisphosphonate which remains anchored to the HA matrix, coordinating and holding some of the Pt(en) residues. Unmodified and HA-adsorbed Pt complexes were tested for their cytotoxicity toward human cervix carcinoma cells (HeLa). The HA-loaded Pt complexes were more cytotoxic than the unmodified compounds A and B, and their cytotoxicity was comparable to that of PtCl2(en), thus indicating a common active species. The above results demonstrate that HA nanocrystals and antitumor drugs can be conjugated in such a way that they yield a smart bone filler delivery system, acting both as bone substitutes and as platinum drug releasing agents with the final goal of locally inhibiting the tumor regrowth and reducing the systemic toxicity. The one described here can not only ensure a prolonged release of active species but also improve the performance of the unmodified drug. Moreover, these results suggest the possibility of using the chemical–physical differences of HA nanocrystals, above all degree of crystallinity, crystal size, and surface area, in order to strongly tailor the Pt complex release kinetics. Considering the biomimetic apatite nanocrystal’s functionalization effects, an attracting goal could be to obtain a drug delivery process characterized by a stimuli-responsive kinetic. This aim induces to surface functionalize HA nanocrystals with different linking agents, such as bisphosphonates, to anchor biologically active molecules which can be released breaking the linkage as a consequence of external stimuli or internal chemical factors, such as pH and ionic force variation due to physiological or pathological biological process.
Biomimetic nanohydroxyapatite coating
In medical devices such as ceramic-coated metallic implants or drug-eluting stents, mechanical strength can only be achieved with metals, which lack the required biocompatibility. Surface treatments to improve that lack have been extensively studied. One of the most promising techniques in the production of composite coatings is the electrochemically assisted deposition onto a metallic surface. This method not only allows overcoming the difficulty of depositing protein component by plasma spray or physical vapor deposition, but also allows controlling the coating process easily.
Recently, hydroxyapatite and collagen/hydroxyapatite biomimetic nanostructured coatings have been applied on conductive material surface, like titanium, by an electrochemically assisted deposition in order to improve the surface bioactivity.Citation169 In preparing the electrolyte, proper amounts of Ca(NO3)2 and NH4H2PO4 were dissolved in distilled water to make 42 mM of [Ca2+] and 25 mM of [PO43−]. This electrolyte mixture was kept at room temperature and a current of 34 mA was applied. The main reactions occurring at the Ti cathode when current passes through the electrodes are the following:
During the electrochemical process, the pH increases up to about 9.0–10.0 around the cathode, leading the precipitation of a mineral apatitic phase on the cathode electrode.Citation170–Citation172 It is possible to obtain also calcium–phosphate/collagen coating on titanium surface with cell containing slightly acidic collagen molecules (soluble type I collagen was added to the electrolyte at the concentration of 0.012%, w/v) and Ca2+ and PO43− ions aqueous solution. In such a process, the collagen/calcium phosphate composite formation involves the self-assembly of collagen molecules into reconstituted fibrils during the contemporary crystallization of calcium phosphate mineral on the electrode surface.Citation169 In an electrochemical cell composed of two electrodes, the nucleation–crystallization of carbonate-hydroxyapatite and the collagen molecules self-assembling of collagen fibers take place simultaneously for effect of the pH raising on the titanium electrode surface, allowing the formation of a nanostructured hydroxyapatite/collagen biomimetic coating.Citation170
In order to well visualize the collagen fibrils electrochemically deposited on the cathode, the collagen fibrils– hydroxyapatite nanocrystals hybrid coating can be decalcified in a 10 wt% EDTA solution for 24 h before being examined by SEM ().
Figure 9 Scanning electron microscopy image of collagen fibrils–hydroxyapatite nanocrystals hybrid coating after decalcification in a 10-wt% EDTA solution for 24 h. Scale bar is 5 μm.
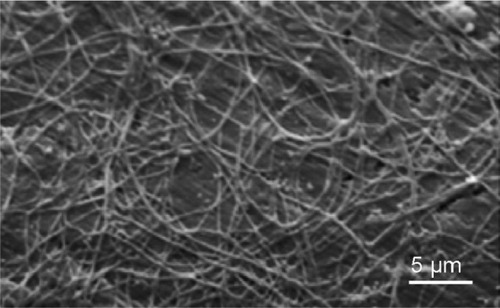
The electrochemically assisted deposition of biomimetic hydroxyapatite/collagen coating on ceramic and metallic prosthesis opens many opportunities to optimize the bone–prosthesis interface, improving bone conductivity and prosthesis immobilization. However, the interaction of blood with the surface biomaterials utilized for artificial organs and biomedical device preparation is strictly related to the coagulation mechanism and thrombus formation. These collateral effects could be prevented by surface functionalizing the electrodeposited biomimetic apatitic coating with an appropriate anticoagulant at exemplum. Heparin which is the major clinical anticoagulant is a linear, unbranched, highly sulfonated polymer that consists of 1,4-linked pyranosyluronic acid and glucosamine units. The nanostructured apatite coating functionalization by heparin has been performed carrying out the hydroxyapatite electrochemical deposition in the presence of low concentration of heparin in the electrolytic solution. According to this method, it is possible to obtain on the metallic prosthesis an electrochemically assisted deposition of a coating of nanostructured hydroxyapatite functionalized with heparin revealing a strong anticoagulant activity.Citation173
Biomimetic hydroxyapatite for teeth remineralization
By a nanotechnological ‘bottom-up’ process, biomimetic carbonate-hydroxyapatite nanocrystals (CHAs) can aggregate into microsized crystal clusters, whose dimensions increase prolonging maturation time in mother solution at constant temperature and stirring.Citation174
This synthetic process to prepare biomimetic nanostructured microsized crystal clusters starts with the synthesis of CHAs having a nearly stoichiometric in bulk Ca/P molar ratio of about 1.6–1.7 and containing 4 ± 1 wt% of carbonate ions replacing prevalently phosphate groups. TEM images of these synthetic CHA microcrystals showing a nanostructure surface morphology are reported in .
Powder X-ray diffraction patterns of these nanocrystals () show characteristic diffraction maxima of an apatite single phase. The broadening of the diffraction maxima present in the X-ray diffraction patterns indicates a relatively low degree of crystallinity, very close to the one determined by the X-ray diffraction pattern of deproteined dentine natural carbonate-hydroxyapatite (). The same similarity can be observed from the comparison of the FTIR spectra of synthesized CHA nanocrystals and natural apatite of deproteined dentine.
Figure 11 Powder X-ray diffraction patterns of biomimetic carbonate-hydroxyapatite microcrystals (red) compared with the powder X-ray diffraction patterns of dentine apatite (blue).
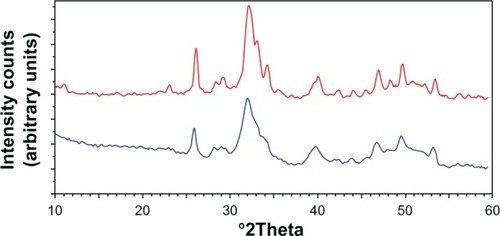
CHA nanocrystal and CHA crystal microclusters represent an excellent biomaterial as bone filler and substitute in orthopedic, maxillofacial, and dental surgery.Citation175 Actually, biomimetic carbonate-hydroxyapatite nanostructured microcrystals are successfully utilized as active agents in some oral care products such as toothpastes and mouth-washes. Biomimetic hydroxyapatite micro/nanocrystals were demonstrated to be able to remineralize the surfaces of enamel and exposed dentine. The dentine remineralizing effect of synthetic biomimetic CHA nanometric crystal has been studied in vitro by scanning electron microscopy positioning CHA nanocrystals slurry solution onto slices of dentine. Biomimetic hydroxyapatite nanocrystals were demonstrated to be able to remineralize the surfaces of the dentine previously etched by orthophosphoric acid application and able to progressively reduce the number of dentine tubules in few minutes till quickly regenerating a new layer of mineralized tissue. This remineralization effect of CHA has encouraged the design and preparation of toothpastes, exhibiting remineralizing effect and ability to contrast dentine hipersensibility.Citation176,Citation177 Scanning electron microscopic analysis allows investigation of the morphology of both demineralized enamel and the features observed after remineralization procedures induced by biomimetic CHA nanocrystals in in vitro application ().Citation178,Citation179 Biomimetic nanostructured CHA microcrystals produce an apatite-coating deposition on the enamel surface. This coating is much less crystalline than native enamel apatite and consists of a new apatitic mineral deposition which progressively fills the scratches and pits.
Figure 12 Scanning electron microscopy images of enamel surface after application of A) ortophosphoric acid and B) CHA-contained toothpaste.
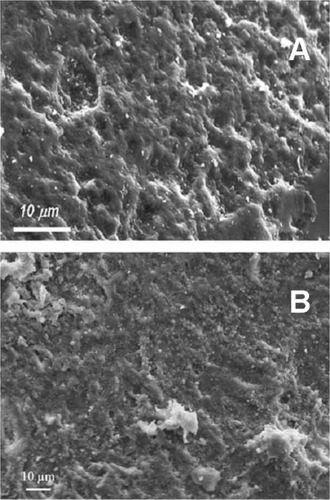
Only recently, the development of nanotechnologies has opened new opportunities in obtaining cheap hydroxyapatite micro/nanoparticles by the ‘bottom-up’ methods. These hydroxyapatites are surface nanostructured and have higher surface area and consequently higher reactivity, allowing them to bind to enamel and dentine apatite producing a biomimetic coating on enamel, contrasting plaque formation, and sealing dentine tubules, and annulling hypersensibility.
Conclusions
Biomimetic hydroxyapatite nano- and microcrystals can be synthesized to exhibit excellent properties like biocompatibility, bioactivity, osteoconductivity, and direct bonding to bone, exciting new applications in the fields of bone tissue engineering and in orthopedic and odontoiatric therapies. Biogenic materials are nucleated in defined nano-/microdimensional sites inside the biological environments in which chemistry can be spatially controlled. The spatial delimitation is essential to biological mechanisms for controlling the size, shape, and structural organization of biomaterials. This strategy employing natural material genesis has attracted a lot of attention in designing bioinspired materials. In the past, industrial hydroxyapatite has been submitted to milling to improve hydroxyapatite surface area and reactivity. Recently, the development of nanotechnology has opened new opportunities in obtaining hydroxyapatite micro/nanoparticles by the ‘bottom-up’ methods. These hydroxyapatites are nanostructured and have higher surface area and consequently higher reactivity. Biomineral morphogenesis is related to specific strategies for the long-range chemical construction of well-organized architectures from preformed nano- or microcrystalline inorganic building blocks. Biosystems reveal a high level of integration of three fundamental aspects: the nano-/micro- ‘spatial confinement’ of biochemical reactions, the inorganic and organic ‘hybridization’ compounds, and the ‘hierarchy’ from nano- to macroscale, in order to produce a biomaterial that is able to exhibit the appropriate chemical-physical properties at any different scale level. These aspects have been translated into synthetic methods and strategies for the laboratory construction of materials organized across a range of length scale.
Biomedical applications, especially new bone formation and drug delivery function, are optimized by the physical and chemical features which could be tailored in synthetic biomimetic HA. The surface functionalization of HA nanocrystals with bioactive molecules enables them to transfer information and to act selectively on the biological environment and cell activity representing a main challenge for innovative biomaterials opening interesting future developments.
However, the high degree of sophistication, miniaturization, hierarchical organization, hybridizing, reliability, efficiency, resistance, and adaptability of biogenic hydroxyapatites have been achieved through specific building principles selected by evolution, and these characteristics can be only partially obtained in man-made materials by present synthetic processes. For this reason, nature is a school for material science representing important tools for the design and the synthesis of the innovative materials and devices in the future.
Acknowledgments
We thank the University of Bologna (funds for selected research topics) and the Interuniversity Consortium for Research on Chemistry of Metals in Biological Systems (CIRCMSB). In honor of Prof. Alberto Ripamonti on his 80th birthday.
Disclosure
The authors state no conflict of interest and have received no payment in the preparation of this manuscript.
References
- BoskeyALPaschalisEBone EngineeringDaviesJEToronto, CanadaEm Squared, Inc200044
- CowlesEADeRomeMEPastizzoGBraileyLLGronowiczGAMineralization and the expression of matrix proteins during in vivo bone developmentCalcif Tissues Int19986217482
- MarksSCHermeyDCBilezikianJPRaiszLGRodanGAThe Principles of Bone BiologyNew York, NYAcademic Press19963
- ReddiAHSymbiosis of biotechnology and biomaterials: applications in tissue engineering of bone and cartilageJ Cell Biochem19945621921957829580
- UristMRBone: formation by autoinductionScience19651506988938995319761
- UristMRSilvermanBFBuringKDubucFLRosenbergJThe bone induction principleClin Orthop Relat Res1967532432834870495
- TermineJDKleinmanHKWhitsonSWConnKMMcGarveyMLMartinGROsteonectin, a bone-specific protein linking mineral to collagenCell1981261 Pt 1991057034958
- WozneyJMThe bone morphogenic protein family and osteogenesisMol Reprod Dev19923221601671637554
- ManolagasSCBirth and death of bone cells: basic regulatory mechanisms and implications for the pathogenesis and treatment of osteoporosisEndocr Rev200021211513710782361
- WeinerSWagnerSDThe material bone: structure-mechanical function relationsAnnu Rev Mater Sci1998281271298
- MannSBiomineralization Principles and Concepts in Bioinorganic Materials ChemistryOxford, UKOxford University Press2001
- PalmerLCNewcombCJKaltzSRSpoerkeEDStuppSIBiomimetic systems for hydroxyapatite mineralization inspired by bone and enamelChem Rev2008108114754478319006400
- Fernandez-MoranHEngstromAElectron microscopy and X-ray diffraction of boneBiochim Biophys Acta195723226026413412720
- TraubWAradTWeinerSThree-dimensional ordered distribution of crystals in turkey tendon collagen fibersProc Natl Acad Sci U S A19898624982298262602376
- FengJQWardLMLiuSLoss of DMP1 causes rickets and osteomalacia and identifies a role for osteocytes in mineral metabolismNat Genet200638111310131517033621
- SchmidtWJÜber die Orientierung der Kristallite im ZahnschmelzNaturwissenschaften19362423361
- OlsztaMJChengXJeeSSBone structure and formation: a new perspectiveMat Sci Eng R Rep2007583–577116
- BurgerCZhouHWWangHLateral packing of mineral crystals in bone collagen fibrilsBiophys J20089541985199218359799
- WeinerSAddadiLDesign strategies in mineralized biological materialsJ Mater Chem199775689702
- RhoJYKuhn-SpearingLZiouposPMechanical properties and the hierarchical structure of boneMed Eng Phys1998202921029679227
- RobinsonCKirkhamJShoreRDental Enamel Formation to DestructionBoca Raton, FLCRC Press1995
- TamerlerCSarikayaMMolecular biomimetics: genetic synthesis, assembly, and formation of materials using peptidesMRS Bull2008335504510
- Ten CateAROral Histology Development, Structure, and Function4th edSt. Louis, MOMosby1994
- WhiteSNLuoWPaineMLFongHSarikayaMSneadMLBiological organization of hydroxyapatite crystallites into a fibrous continuum toughens and controls anisotropy in human enamelJ Dent Res200180132132611269723
- ImbeniVKruzicJJMarshallGWMarshallSJRitchieROThe dentin-enamel junction and the fracture of human teethNat Mater20054322923215711554
- PaineMLWhiteSNLuoWFongHSarikayaMSneadMLRegulated gene expression dictates enamel structure and tooth functionMatrix Biol2001205–627329211566262
- WenHBMoradian-OldakJFinchamAGDose-dependent modulation of octacalcium phosphate crystal habit by amelogeninsJ Dent Res200079111902190611145363
- LowestanHAWeinerSOn BiomineralizationNew York, NYOxford University Press1989
- WangRZWeinerSStrain-structure relations in human teeth using Moiré fringesJ Biomech19983121351419593206
- WhittakerDKStructural variations in the surface zone of human tooth enamel observed by scanning electron microscopyArch Oral Biol19822753833926956250
- BoydeAHandbook of Microscopic AnatomyOkscheAVollrathVBerlin, GermanySpringer-Verlag1989
- KodakaTNakajimaFHigashiSStructure of the so-called ‘prism-less’ enamel in human deciduous teethCaries Res19892352902962766312
- GarantPOral Cells and TissuesChicago, ILQuintessence Publishing Co., Inc2003
- LeGerosRZCalcium phosphate-based osteoinductive materialsChem Rev2008108114742475319006399
- de JongWFThe mineral substance in boneRec Trav Chim Pays-Bas192645445448
- BeeversCAMcIntyreDBThe atomic structure of fluorapatite and its relation to that of tooth and bone mineralMineral Mag194627254259
- McConnellDThe crystal chemistry of carbonate apatities and their relationship to the composition of calcified tissuesJ Dent Res1952311536314917817
- KayMIYoungRAPosnerASCrystal structure of hydroxyapatiteNature19642041050105214243377
- LeGerosRZLeGerosJPTrautzORKleinESpectral properties of carbonate in carbonate-containing apatitesDev Appl Spectrosc19707B3
- LeGerosRZLeGerosJPTrautzORShirraWPConversion of monetite, CaHPO4, to apatites: effects of carbonate on the crystallinity and the morphology of the apatite crystallitesAdv X-ray Anal1971145766
- LeGerosRZSilverstoneLMDaculsiGKerebelLMIn vitro caries-like lesion formation in F-containing tooth enamelJ Dent Res19836221381446571867
- LeGerosRZTrautzORLeGerosJPKleinEShirraWPApatite crystallites: effects of carbonate on morphologyScience196715537681409141117839613
- LeGerosRZApatites in biological systemsProg Crystal Growth Charact19814145
- ElliottJCStructure and Chemistry of Apatites and Other Calcium OrthophosphatesAmsterdam, The NetherlandsElsevier1994
- ReyCRenugopalakrishnanVCollinsBGlimcherMJFourier transform infrared spectroscopic study of the carbonate ions in bone mineral during agingCalcif Tissue Int19914942512581760769
- ElliottJCHolcombDWYoungRAInfrared determination of the degree of substitution of hydroxyl by carbonate ions in human dental enamelCalcif Tissue Int19853743723753930033
- EichertDDrouetCSfihiaHReyCCombesCNanocrystalline apatite-based biomaterials: synthesis, processing and characterizationKendallJBBiomaterials Research AdvancesNew York, NYNova Science Publishers201093143
- LeGerosRZCalcium Phosphates in Oral Biology and MedicineNew York, NYKarger1991
- DorozhkinSVEppleMBiological and medical significance of calcium phosphatesAngew Chem Int Ed Engl200241173130314612207375
- DaculsiGBoulerJMLeGerosRZAdaptive crystal formation in normal and pathological calcifications in synthetic calcium phosphate and related biomaterialsInt Rev Cytol19971721291919102393
- DorozhkinSVNanosized and nanocrystalline calcium orthophosphatesActa Biomater20106371573419861183
- SchmidtHKNanoparticles for ceramic and nanocomposite processingMol Cryst Liq Cryst2000353165179
- CushingBLKolesnichenkoVLO’ConnorCJRecent advances in the liquidphase syntheses of inorganic nanoparticlesChem Rev200410493893394615352782
- WangXZhuangJPengQLiYA general strategy for nanocrystal synthesisNature2005437705512112416136139
- MaoYParkTJZhangFZhouHWongSSEnvironmentally friendly methodologies of nanostructure synthesisSmall2007371122113917554768
- WangJShawLLMorphology-enhanced low-temperature sintering of nanocrystalline hydroxyapatiteAdv Mater2007191723642369
- HuangJBestSMBonfieldWIn vitro assessment of the biological response to nano-sized hydroxyapatiteJ Mater Sci Mater Med200415444144515332614
- LiouSCChenSYLeeHYBowJSStructural characterization of nano-sized calcium deficient apatite powdersBiomaterials200425218919614585706
- GanesanKEppleMCalcium phosphate nanoparticles as nuclei for the preparation of colloidal calcium phytateNew J Chem200832813261330
- ZhangYLuJA simple method to tailor spherical nanocrystal hydroxyapatite at low temperatureJ Nanopart Res200794589594
- SunWChuCWangJZhaoHComparison of periodontal ligament cells responses to dense and nanophase hydroxyapatiteJ Mater Sci Mater Med200718567768317143736
- ChaiCSBen-NissanBBioactive nanocrystalline sol–gel hydroxyapatite coatingsJ Mater Sci Mater Med199910846546915348113
- Ben-NissanBChoiAHSol–gel production of bioactive nanocoatings for medical applications. Part 1. An introductionNanomedicine (Lond)20061331131917716161
- ChoiAHBen-NissanBSol–gel production of bioactive nanocoatings for medical applications. Part 2. Current research and developmentNanomedicine (Lond)200721516117716190
- RusuVMNgCHWilkeMTierschBFratzlPPeterMGSize-controlled hydroxyapatite nanoparticles as self-organized organic–inorganic composite materialsBiomaterials200526265414542615814140
- López-MacipeAGómez-MoralesJRodríguez-ClementeRNanosized hydroxyapatite precipitation from homogeneous calcium/citrate/phosphate solutions using microwave and conventional heatingAdv Mater19981014953
- TasACSynthesis of biomimetic Ca-hydroxyapatite powders at 37 degrees C in synthetic body fluidsBiomaterials200021141429143810872772
- GuoXGoughJEXiaoPLiuJShenZFabrication of nanostructured hydroxyapatite and analysis of human osteoblastic cellular responseJ Biomed Mater Res A20078241022103217377965
- ChaudhryAAHaqueSKelliciSInstant nano-hydroxyapatite: a continuous and rapid hydrothermal synthesisChem Commun (Camb)2006212286228816718331
- SuchanekWLShukPByrappaKRimanRETenHuisenKSJanasVFMechanochemical–hydrothermal synthesis of carbonated apatite powders at room temperatureBiomaterials200223369971011771690
- YeonKCWangJNgSCMechanochemical synthesis of nanocrystalline hydroxyapatite from CaO and CaHPO4Biomaterials200122202705271211545304
- LiuJLiKWangHZhuMXuHYanHSelf-assembly of hydroxyapatite nanostructures by microwave irradiationNanotechnology20051618287
- RameshbabuNRaoKPKumarTSSAccelerated microwave processing of nanocrystalline hydroxyapatiteJ Mater Sci2005402363196323
- SivaRamaKrishnaDSiddharthanASeshadriSKSampath KumarTSA novel route for synthesis of nanocrystalline hydroxyapatite from eggshell wasteJ Mater Sci Mater Med20071891735174317483877
- IafiscoMGomez MoralesJHernandez-HernandezMAGarcia RuizJMRoveriNBiomimetic carbonate-hydroxyapatite nanocrystals prepared by vapor diffusionAdv Eng Mater2010127B218B223
- IafiscoMMarchettiMGomez MoralesJHernandez-HernandezMAGarcia RuizJMRoveriNSilica gel template for calcium phosphates crystallizationCryst Growth Des200991149124921
- PhillipsMJDarrJALuklinskaZBRehmanISynthesis and characterization of nanobiomaterials with potential osteological applicationsJ Mater Sci Mater Med2003141087588215348525
- LayrollePLebugleACharacterization and reactivity of nanosized calcium phosphate prepared in anhydrous ethanolChem Mater199461119962004
- FerrazMPMonteiroFJManuelCMHydroxyapatite nanoparticles: a review of preparation methodologiesJ Appl Biomater Biomech20042748020803440
- YeFGuoHZhangHBiomimetic synthesis of oriented hydroxyapatite mediated by nonionic surfactantsNanotechnology2008192424560510.1088/0957-4484/19/24/24560521825817
- KoutsopoulosSSynthesis and characterization of hydroxyapatite crystals: a review study on the analytical methodsJ Biomed Mater Res200262460061212221709
- TampieriACelottiGLandiEFrom biomimetic apatites to biologically inspired compositesAnal Bioanal Chem2005381356857615696277
- RoveriNPalazzoBIafiscoMThe role of biomimetism in developing nanostructured inorganic matrices for drug deliveryExpert Opin Drug Deliv20085886187718712996
- CaiYTangRCalcium phosphate nanoparticles in biomineralization and biomaterialsJ Mater Chem2008183237753787
- IngertDPileniMPLimitations in producing nanocrystals using reverse micelles as nanoreactorsAdv Funct Mater2001112136139
- WongKKWMannSBiomimetic synthesis of cadmium sulfide-ferritin nanocompositesAdv Mater1996811928932
- DouglasTYoungMHost-guest encapsulation of materials by assembled virus protein cagesNature19983936681152155
- BaralSSchoenPSilica-deposited phospholipid tubules as a precursor to hollow submicron-diameter silica cylindersChem Mater199352145147
- StuppSIBraunPVMolecular manipulation of microstructures: biomaterials, ceramics, and semiconductorsScience19972775330124212489271562
- PaulWSharmaCPNanoceramic matrices: biomedical applicationsAm J Biochem Biotechnol2006224148
- ConstantzBRIsonICFulmerMTSkeletal repair by in situ formation of the mineral phase of boneScience19952675205179617997892603
- BrownWEChowLCA new calcium phosphate setting cementJ Dent Res198362672
- GinebraMPDriessensFCPlanellJAEffect of the particle size on the micro and nanostructural features of a calcium phosphate cement: a kinetic analysisBiomaterials200425173453346215020119
- HanYLiSWangXChenXSynthesis and sintering of nanocrystalline hydroxyapatite powders by citric acid sol–gel combustion methodMater Res Bull20043912532
- GinebraMPTraykovaTPlanellJACalcium phosphate cements as bone drug delivery systems: a reviewJ Control Release2006113210211016740332
- RuegerJMBone substitution materials. Current status and prospectsOrthopade199827272799530663
- CuiFZLiYGeJSelf-assembly of mineralized collagen compositesMater Sci Eng R Rep2007571–6127
- ZhangYZhangMCalcium phosphate/chitosan composite scaffolds for controlled in vitro antibiotic drug releaseJ Biomed Mater Res200262337838612209923
- ShirkhanzadehMAzadeganMFormation of carbonate apatite on calcium phosphate coatings containing silver ionsJ Mater Sci Mater Med19989738539115348866
- YoonBHKimHWLeeSHStability and cellular responses to fluorapatite-collagen compositesBiomaterials200526162957296315603790
- GuoHKhorKABoeyYCMiaoXLaminated and functionally graded hydroxyapatite/yttria stabilized tetragonal zirconia composites fabricated by spark plasma sinteringBiomaterials200324466767512437961
- WeiGMaPXStructure and properties of nano-hydroxyapatite/polymer composite scaffolds for bone tissue engineeringBiomaterials200425194749475715120521
- ClemensJAKleinCPVriesdeRCRozingPMde GrootKHealing of large (2 mm) gaps around calcium phosphate-coated bone implants: a study in goats with a follow-up of 6 monthsJ Biomed Mater Res19984033413499570063
- GuYWKhorKACheangPIn vitro studies of plasma-sprayed hydroxyapatite/Ti-6Al-4V composite coatings in simulated body fluid (SBF)Biomaterials20032491603161112559820
- BigiABoaniniEBracciBNanocrystalline hydroxyapatite coatings on titanium: a new fast biomimetic methodBiomaterials200526194085408915664635
- Rodríguez-LorenzoLMVallet-RegíMFerreiraJMFabrication of hydroxyapatite bodies by uniaxial pressing from a precipitated powderBiomaterials200122658358811219723
- TampieriACelottiGSprioSDelcoglianoAFranzeseSPorosity-graded hydroxyapatite ceramics to replace natural boneBiomaterials200122111365137011336309
- TadicDBeckmannFSchwarzKEppleMA novel method to produce hydroxyapatite objects with interconnecting porosity that avoids sinteringBiomaterials200425163335334014980428
- Rodríguez-LorenzoLMVallet-RegíMFerreiraJMGinebraMPAparicioCPlanellJAHydroxyapatite ceramic bodies with tailored mechanical properties for different applicationsJ Biomed Mater Res200260115916611835171
- PadillaSRománJVallet-RegíMSynthesis of porous hydroxyapatites by combination of gelcasting and foams burn out methodsJ Mater Sci Mater Med200213121193119715348665
- HabrakenWJWolkeJGJansenJACeramic composites as matrices and scaffolds for drug delivery in tissue engineeringAdv Drug Deliv Rev2007594–523424817478007
- RoyDMLinnehanSKHydroxyapatite formed from coral skeletal carbonate by hydrothermal exchangeNature19742474382202224149289
- BattistellaEMeleSPietronaveSTransformed cuttle-fish bone scaffolds for bone tissue engineeringAdv Mater Res201089–914752
- Vallet-RegìMRàmilaADel RealRPPèrez-ParienteJA new property of MCM-41: drug delivery systemChem Mater2001132308331
- Ben-NissanBNatural bioceramics: from coral to bone and beyondCurr Opin Solid State Mater Sci200374–5283288
- TampieriACelottiGLandiEPorous phosphate-gelatin composite as bone graft with drug delivery functionJ Mater Sci Mater Med200314762362715348425
- MaXPBiomimetic materials for tissue engineeringAdv Drug Deliv Rev200860218419818045729
- PalazzoBSidotiMCRoveriNControlled drug delivery from porous hydroxyapatite grafts: an experimental and theoretical approachMater Sci Eng C2005252207213
- PeñaJVallet-RegíMSan RománJTiO2-polymer composites for biomedical applicationsJ Biomed Mater Res19973511291349104705
- WangMKokuboTBonfieldWBioceramicsOxford, UKPergamon1996
- KikuchiMSatoKSuetsuguYTanakaJBioceramicsNew YorkWorld Scientific1998
- BakosDSoldánMHernández-FuentesIHydroxyapatite-collagen-hyaluronic acid compositeBiomaterials199920219119510022789
- CherngATakagiSChowLCEffects of hydroxypropyl methylcellulose and other gelling agents on the handling properties of calcium phosphate cementJ Biomed Mater Res19973532732779138061
- LiXFanTLiuZDingJGuoQZhangDSynthesis of hierarchical pore structure of biomorphic manganese oxide derived from woodsJ Eur Ceram Soc2006261636573664
- ZimmermanAFPalumboGAustKTErbUMechanical properties of nickel silicon carbide nanocompositesMater Sci Eng A20023281–2137146
- SinghMMartinez-FernandezJde Arellano-LopezAREnvironmentally conscious ceramics (ecoceramics) from natural wood precursorsCurr Opin Solid State Mater Sci200373247254
- SinghMSalemJAMechanical properties and microstructure of biomorphic silicon carbide ceramics fabricated from wood precursorsJ Eur Ceram Soc20022214–1527092717
- de Arellano LopezARMartinez-FernandezJGonzalezPDominguezCFernandez-QueroVSinghMBiomorphic SiC: a new engineering ceramic materialInt J Appl Ceram Technol2004115667
- Varela-FeriaFMMartinez-FernandezJde Arellano-LópezARSinghMLow density biomorphic silicon carbide: microstructure and mechanical propertiesJ Eur Ceram Soc20062214–1527192725
- ZhuSZhangDLiZFurukawaHChenZPrecision replication of hierarchical biological structures by metal oxides using a sonochemical methodLangmuir200824126292629918489191
- Burzy skaLRudnikEKozaJBłaLSzyma skiWElectrodeposition and heat treatment of nickel/silicon carbide compositesSurf Coatings Technol20082021225452556
- SochaRPNowakPLaajalehtoKVäyrynenJParticle-electrode surface interaction during nickel electrodeposition from suspensions containing SiC and SiO2particlesColloids Surf A Physicochem Eng Asp20042351–34555
- LelliMFoltranIForestiEBiomorphic silicon carbide coated with an electrodeposition of nanostructured hydroxyapatite/collagen as biomimetic bone filler and scaffoldAdv Eng Mater2010128B348B355
- TampieriASprioSRuffiniACelottiGLesciIGRoveriNFrom wood to bone: multi-step process to convert wood hierarchical structures into biomimetic hydroxyapatite scaffolds for bone tissue engineeringJ Mater Chem20091949734980
- ParkKControlled Drug Delivery: Challenges and StrategiesWashington (DC)American Chemical Society1997
- BreimerDDFuture challenges for drug deliveryJ Control Release1999621–23610518628
- JulianoRLControlled Delivery of Drugs: and Overview and ProspectusNew YorkOxford University Press1990
- BraydenDJControlled release technologies for drug deliveryDrug Discov Today200382197697814643159
- HughesGANanostructure-mediated drug deliveryNanomedicine200511223017292054
- KooOMRubinsteinIOnyukselHRole of nanotechnology in targeted drug delivery and imaging: a concise reviewNanomedicine20051319321217292079
- LinCCMettersATHydrogels in controlled release formulations: network design and mathematical modelingAdv Drug Deliv Rev20065812–131379140817081649
- LecomteFSiepmannJWaltherMMacRaeRJBodmeierRpH-Sensitive polymer blends used as coating materials to control drug release from spherical beads: elucidation of the underlying mass transport mechanismsPharm Res20052271129114116028014
- AcharyaGParkKMechanisms of controlled drug release from drug-eluting stentsAdv Drug Deliv Rev200658338740116546289
- Szymura-OleksiakJSlosarczykACiosAThe kinetics of pentoxifylline release in vivo from drug-loaded hydroxyapatite implantsCeram Int2001277767772
- DashAKCudworthGC2ndTherapeutic applications of implantable drug delivery systemsJ Pharmacol Toxicol Methods19984011129920528
- PalazzoBWalshDIafiscoMAmino acid synergetic effect on structure, morphology and surface properties of biomimetic apatite nanocrystalsActa Biomater2009541241125219083277
- KandoriKOdaSFukusumiMMorisadaYSynthesis of positively charged calcium hydroxyapatite nano-crystals and their adsorption behavior of proteinsColloids Surf B Biointerfaces200973114014519515538
- LatourRAMolecular simulation of protein-surface interactions: benefits, problems, solutions, and future directionsBiointerphases200833FC2FC1219809597
- Hernández-HernándezARodríguez-NavarroABGómez-MoralesJJiménez-LopezCNysYGarcía-RuizJMInfluence of model globular proteins with different isoelectric points on the precipitation of calcium carbonateCryst Growth Des20088514951502
- DeeKCPuleoDABiziosRAn Introduction to Tissue-Biomaterial InteractionsHobokenWiley-Liss2003
- LiuTYChenSYLiuDMLiouSCOn the study of BSA-loaded calcium-deficient hydroxyapatite nano-carriers for controlled drug deliveryJ Control Release2005107111212115982777
- PietronaveSIafiscoMLocarnoDRimondiniLMaria PratMFunctionalized nanomaterials for diagnosis and therapy of cancerJ Appl Biomater Biomech200972778920799167
- KondoAMiharaJComparison of adsorption and conformation of hemoglobin and myoglobin on various inorganic ultrafine particlesJ Colloid Interface Sci1996177121422110479434
- BoixTGómez-MoralesJTorrent-BurguésJMonfortAPuigdomènechPRodríguez-ClementeRAdsorption of recombinant human bone morphogenetic protein rhBMP-2m onto hydroxyapatiteJ Inorg Biochem20059951043105015833327
- WassellDTHallRCEmberyGAdsorption of bovine serum albumin onto hydroxyapatiteBiomaterials19951696977027578773
- BarrougAFastrezJLemaitreJRouxhetPAdsorption of succinylated lysozyme on hydroxyapatiteJ Colloid Interface Sci199718913742
- IafiscoMPalazzoBFaliniGAdsorption and conformational change of myoglobin on biomimetic hydroxyapatite nanocrystals functionalized with alendronateLangmuir20082494924493018373380
- IafiscoMSabatinoPLesciIGPratMRimondiniLRoveriNConformational modifications of serum albumins adsorbed on different kinds of biomimetic hydroxyapatite nanocrystalsColloids Surf B Biointerfaces201081127428420692819
- EppleMGanesanKHeumannRApplication of calcium phosphate nanoparticles in biomedicineJ Mater Chem20102011823
- EwenceAEBootmanMRoderickHLCalcium phosphate crystals induce cell death in human vascular smooth muscle cells: a potential mechanism in atherosclerotic plaque destabilizationCirc Res20081035e28e3418669918
- DoatAPelleFGardantNLebugleASynthesis of luminescent bioapatite nanoparticles for utilization as a biological probeJ Solid State Chem20041774–511791187
- Padilla MondejarSKovtunAEppleMLanthanide-doped calcium phosphate nanoparticles with high internal crystallinity and with a shell of DNA as fluorescent probes in cell experimentsJ Mater Chem2007173941534159
- RamachandranRPaulWSharmaCPSynthesis and characterization of PEGylated calcium phosphate nanoparticles for oral insulin deliveryJ Biomed Mater Res B Appl Biomater2009881414818946870
- ChengXKuhnLChemotherapy drug delivery from calcium phosphate nanoparticlesInt J Nanomedicine20072466767418203433
- KesterMHeakalYFoxTCalcium phosphate nanocomposite particles for in vitro imaging and encapsulated chemotherapeutic drug delivery to cancer cellsNano Lett20088124116412119367878
- LiuTTangAZhangGCalcium phosphate nanoparticles as a novel nonviral vector for efficient transfection of DNA in cancer gene therapyCancer Biother Radiopharm200520214114915869447
- PalazzoBIafiscoMLaforgiaMBiomimetic hydroxyapatite-drug nanocrystals as potential bone substitutes with antitumor drug delivery propertiesAdv Funct Mater2007171321802188
- IafiscoMPalazzoBMarchettiMSmart delivery of antitumoral platinum complexes from biomimetic hydroxyapatite nanocrystalsJ Mater Chem2009194483858392
- FanYDuanKWangRA composite coating by electrolysis-induced collagen self-assembly and calcium phosphate mineralizationBiomaterials200526141623163215576136
- ManaraSPaolucciFPalazzoBElectrochemically assisted deposition of biomimetic hydroxyapatite-collagen coatings on titanium plateInorg Chim Acta2008361616341645
- LuXZhaoZLengYCalcium phosphate crystal growth under controlled atmosphere in electrochemical depositionJ Cryst Growth20052843–4506516
- YousefpourMAfisharAYangXNano-crystalline growth of electrochemically deposited apatite coating on pure titaniumJ Electroanal Chem2006589196105
- PalazzoBManaraSLelliMElectrochemically assisted deposition of nanostructured hydroxyapatite coatings with drug-activated surfaceMC8: Advancing Materials by Chemical Design2007 Jul 2–5University College LondonUK
- GazzanigaGRoveriNRimondiniLPalazzoBIafiscoMGualandiPBiologically active nanoparticles of a carbonate-substituted hydroxyapatite process for their preparation and compositions incorporating the same WO20071376062007
- RoveriNPalazzoBTissue, Cell and Organ EngineeringWeinheimWiley-VCH2006
- RoveriNBattistellaEBianchiCLSurface enamel remineralization: biomimetic apatite nanocrystals and fluoride ions different effectsJ Nanomaterials2009200974638310.1155/2009/746383.
- RimondiniLPalazzoBIafiscoMThe remineralizing effect of carbonate-hydroxyapatite nanocrystals on dentineMater Sci Forum2007539–543602605
- RoveriNBattistellaEFoltranISynthetic biomimetic carbonate-hydroxyapatite nanocrystals for enamel remineralisationAdv Mater Res200847–50821824
- RoveriNForestiELelliMLesciIGRecent advancements in preventing teeth health hazard: the daily use of hydroxyapatite instead of fluorideRecent Pat Biomed Eng200923197215