Abstract
Purpose
Sleep is essential for life and plays a key role for optimal physiology, brain functioning, and health. Evidence suggests a relation between sleep and cerebral white matter integrity. Human studies report that sleep duration shows a U-shaped association with brain functioning. We hypothesized that participants with longer or shorter sleep time in the nighttime period show altered microstructural white matter integrity.
Participants and Methods
Seventy-three young adult participants were evaluated. Sleep-wake cycle parameters were assessed objectively using actigraphy. Diffusion tensor imaging studies were performed to assess white matter integrity using fractional anisotropy and mean, axial, and radial diffusivities. Relations between white matter microstructure indexes and sleep parameters were investigated through tract-based spatial statistics. Participants were grouped according to their nocturnal total sleep time: 27 in the Reference sleep group (6.5–8.0 h), 23 in the Short sleep group (<6.5 h) and 23 in the Long sleep group (>8.0 h).
Results
Compared with the Reference sleep group, participants in the Long sleep group showed lower fractional anisotropy (p < 0.05) and higher radial diffusivity (p < 0.05) values in white matter tracts linked to sleep regulation (corona radiata, body of the corpus callosum, superior longitudinal fasciculus, and anterior thalamic radiation).
Conclusion
This pattern of reduced fractional anisotropy and increased radial diffusivity in the Long sleep group indicates an association between sleep duration and lower integrity of myelin sheaths. Because myelin is continuously remodeled in the brain, nighttime sleep characteristics appear to be a key player for its quality and maintenance.
Introduction
The sleep-wake cycle (SWC) is consolidated by the interaction between homeostatic and circadian processes. The homeostatic process refers to a need for sleep that is accumulated throughout waking and dissipated during sleep. The circadian process is modulated by the internal circadian clock (located in suprachiasmatic nuclei) that is entrained to the light–dark cycle, promoting wake during an active phase of the cycle and allowing sleep during a rest phase of the cycle.Citation1,Citation2 Sleep is essential for life and plays key roles for optimal physiology, brain functioning, and health.Citation3–6
Cerebral white matter is composed of myelinated axons that connect neurons in different brain regions into functional circuits. Myelin is formed by oligodendrocytes and acts as an insulator, which allows high-speed electrical impulse transmission.Citation7 The myelin sheath consists of multiple layers along the axon with small gaps devoid of myelin called nodes of Ranvier.Citation8,Citation9 The nodes are delimited at both sides by the paranode,Citation10 where these myelin layers terminate and form series of paranodal loops tightly attached to the axon.Citation11 Myelination continues until at least the third decade of age in humans.Citation12 Alterations in white matter can disrupt behavior, sensory, motor, and cognitive developmental processes.Citation12–14
Studies in animals have suggested that chronic sleep loss reduces the proliferation of oligodendrocyte precursor cells,Citation15 inhibits the transcription of proteins related to myelination,Citation16 alters node length, and decreases myelin thickness.Citation17 To form layers of myelin, the most external paranodal loop of myelin is continuous with the plasma membrane of the oligodendrocyte.Citation18 De Vivo and BellesiCitation8 proposed that greater sleep restriction causes the outer paranodal loop to detach from the paranodal region and leads to increased node length in the axon followed by retraction into the oligodendrocyte, with consequent myelin sheath thinning.
In humans, white matter integrity is commonly assessed by diffusion tensor imaging (DTI), a magnetic resonance imaging (MRI)-based neuroimaging technique and non-invasive method.Citation19 The DTI parameters most commonly used are fractional anisotropy (FA) and mean diffusivity (MD). Greater FA indicates higher white matter integrity and reduced MD reflects heightened myelination.Citation20 In healthy adults, one night of sleep deprivation was related to decreased FA in the corpus callosum, the thalamus, and the brain stem.Citation21 Compared with self-reported moderate sleep (>6 to ≤8 h), short sleep duration (<6 h sleep) has also been related to increased MD values in the frontal, occipital, parietal, and temporal regions.Citation22 Using actigraphic recordings for objective SWC assessment, total sleep time (TST), lower sleep efficiency and increased duration of wake after sleep onset (WASO) have been associated with lower FA and greater MD in the thalamic radiation, corticospinal tract, uncinate fasciculus, superior and inferior longitudinal fasciculus, inferior fronto-occipital fasciculus, cingulate, and the corpus callosum.Citation23 These findings suggest that sleep may contribute to the formation and maintenance of myelin in the axons and/or affect oligodendrocyte functioning.Citation8
White matter alterations in middle-aged subjects are associated with the risk of dementia.Citation24,Citation25 Moreover, sleep duration shows a U-shaped association with brain functioningCitation26–28 and increases the likelihood of cognitive impairment later in life.Citation29–31 In light of these findings, understanding the relation between white matter integrity and sleep-wake patterns is particularly relevant. In this study, we hypothesized that participants with long or short TST show altered microstructural white matter integrity.
Materials and Methods
Participants
All participants belong to an early infancy cohort of the behavioral and developmental effects of iron-deficiency anemia in infancy. The study design and findings during follow-ups have been published elsewhere.Citation32–34 Briefly, they were healthy term infants (birth weight ≥3.0 kg, without perinatal complications, and free of acute or chronic illnesses). Infants with iron-deficiency anemia at 6, 12, or 18 months of age were considered for neurophysiological evaluations. Those who were clearly non-anemic (venous Hb ≥115 g/L) were randomly invited to the control group. No participant had iron-deficiency anemia at subsequent ages.
In this cross-sectional study in adulthood, 77 young-adults within a narrow age range (22.5 ± 0.2 years) were assessed. Participants filled out the Epworth Sleepiness ScaleCitation35 for daily sleepiness assessment, the Pittsburgh Sleep Quality IndexCitation36 for a subjective measure of sleep quality, and a short questionnaire on sleeping and lifestyle habits. The inclusion criteria consisted of being between the ages of 20 and 30 years and having a regular sleep-work schedule. The exclusion criteria were pregnancy; contraindications to MRI; and history of sleep disorders, major medical and neurological disorders, psychiatric illness, and drug or alcohol dependency or abuse. All participants had actigraphic recordings and DTI studies, but from the initial sample, four were excluded from analysis: three for movement artifacts in the DTI study and one for technical differences in the DTI sequence. Excluded participants were no different in background characteristics.
Participants provided signed informed consent, according to the norms for Human Experimentation, Code of Ethics of the World Medical Association (Declaration of Helsinki, 1995). The original and follow-up protocols were approved and reviewed annually by the Institutional Review Boards of the University of Michigan, Ann Arbor, and the Institute of Nutrition and Food Technology, University of Chile, Santiago.
Actigraphic Recordings
Participants wore waterproof actigraph devices (Actiwatch 2, Philips Respironics, Bend, OR, USA) continuously without removal for 1 week on their non-dominant wrist. Each 24-h interval was divided into nocturnal and diurnal periods. The nocturnal period began with the onset of the first sleep episode after 20:00 h that was followed for at least 30 consecutive min of sustained sleep. This period ended with the transition to the diurnal period, which started with the first wake episode after 06:00 h lasting for at least 30 min of uninterrupted wakefulness. Actigraphic data were digitalized, stored for each successive 1-min interval, and processed on a minute-by-minute basis. We reassessed this first detection of sleep-wake episodes, to avoid the detection of short sleep and wake episodes, which are considered inaccurate and are a main source of errors in actigraphic recordings.Citation37 A locally developed automated procedure generated a new sequence of sleep and wake episodes lasting at least 5-min and incorporated them into the ongoing episode to generate a new sequence.Citation38 The following conventional sleep-wake parameters were assessed: wake-up and sleep onset times, nap episodes, time spent asleep in the nocturnal period (TST), time spent awake in the nocturnal period (WASO), the number of awakenings, mean wake episode duration, latency to the first wake episode, longest wake episode duration and its time placement, and sleep efficiency (proportion of the nocturnal period that is TST). These parameters were assessed in the overall nocturnal period, and WASO and awakenings were also analyzed by halves of the nocturnal period ().Citation39,Citation40 Participants also completed a diary of their SWC that provided data about bedtime, wake-up time, and time of eventual removal of the actigraph. Comparison groups were categorized according to the prior literature on TST: Reference sleep (6.5–8.0 h), Short sleep (<6.5 h), and Long sleep (>8.0 h) groups.Citation41
Figure 1 Illustration of a representative actigraphic nighttime period. Standard parameters of the sleep wake-cycle (eg, wake-up and sleep onset times, duration of sleep and wake episodes) were assessed for the overall nighttime period. Wake episode frequency, duration, distribution, and localization were computed by halves of the nighttime period.
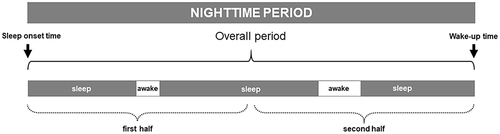
DTI Studies
Studies were performed with a 3-Tesla scanner (Siemens MAGNETOM Skyra System, Siemens Healthcare, Erlangen, Germany). DTI data were acquired using a single shot EPI sequence (TE = 91 ms; TR = 9900 ms; FOV: 256 mm; slices: 72, slice thickness: 2 mm; slice gap: 0 mm; 30 gradient directions with b = 1000 s/mm2 and 1 with b = 0 s/mm2).
Preprocessing was carried out in FMRIB Software Library (FSL, version 5.04).Citation42 The steps were eddy current correction, head motion correction, and brain masking. The diffusion tensor model was then fit at each voxel to obtain maps of FA, MD, axial diffusivity (AD), and radial diffusivity (RD).
Using Tract-Based Spatial Statistics (TBSS, a toolbox of FSL), we performed voxel-wise analysis.Citation43 Running the nonlinear registration (FNIRT), FA images were aligned to the FMRIB58_FA template and affine transformed into Montreal Neurological Institute MNI standard space. FA images were merged, creating a mean FA image, and a skeleton was then generated. A threshold of 0.2 was applied to the FA skeleton to consider only white matter. The aligned FA map of each participant was then projected onto the skeleton. The MD, RD, and AD maps were also projected onto this skeleton.
The microstructural integrity of white matter was indexed by the main parameters of DTI: FA, MD, AD, and RD. Briefly, FA is a quantitative index of the orientation of water diffusion coherence, MD is the average rate of water diffusion, AD measures diffusivity along the primary axis (eigenvalue λ1), and RD is the average diffusivity of the two minor axes (eigenvalues λ2 and λ3), that is, measuring diffusivity perpendicular to the major axis,Citation44 and is related to myelin damage.Citation45
Data Analysis
General linear models were calculated to explore the differences in SWC and DTI indices (FA, MD, RD, and AD) among the groups. We utilized non-parametric permutation-based statistics with the “randomise” tool (p < 0.05) in FSL.Citation46 Five thousand permutations were performed, and threshold-free cluster enhancement (TFCE) was applied to correct for multiple comparisons.Citation47 JHU ICBM-DTI-81 White-Matter Labels and the JHU White-Matter Tractography Atlas were used to identify the location of significant clusters.Citation48,Citation49 All analyses were adjusted for sex and WASO. The Bonferroni correction method was applied. A p-value <0.05 (corrected for multiple comparisons) was considered statistically significant. Statistical analyses were conducted with SAS® Studio (SAS Institute Inc. Cary, NC, USA.).
Results
The mean age of the participants was 22.5 ± 0.2 years and 48% were female. The sleep groups were similar in all background characteristics ().
Table 1 Background Characteristics of Study Participants
Sleep-Wake Parameters
By definition, the groups differed in TST (). Compared with the Reference sleep group, the Short sleep group showed lower sleep efficiency in the overall nocturnal period and greater WASO in the second half of the night. The Long sleep group showed greater WASO and lower sleep efficiency than the Reference group in the overall nocturnal period. The number of wake episodes and WASO were also higher during the second half of the nocturnal period in the Long sleep group compared with the Reference group ().
Table 2 Sleep-Wake Cycle Parameters By Groups
White Matter Integrity and Total Sleep Time
Participants of the Long sleep group showed lower FA () and higher RD values () compared with the Reference sleep group. Of note, not all RD results reached statistical significance. There were significantly lower FA values in the Long sleep group in the body of the corpus callosum and regions of the left hemisphere (). Overlapping but much more restricted clusters were obtained for RD (). There were no other significant differences when comparing the Reference and Short sleep groups, or in MD and AD values in any group comparisons.
Table 3 Cluster of White Matter Tracts With Lower Fractional Anisotropy Values in the Long Versus Reference Sleep Groups
Table 4 Clusters of White Matter Tracts With Higher Radial Diffusivity Values in the Long Versus Reference Sleep Groups
Figure 2 Fractional anisotropy differences between the Long and Reference sleep groups. The red-yellow color indicates the white matter clusters that showed lower fractional anisotropy values (α < 0.05, threshold-free cluster enhancement and family-wise error correction) in the Long sleep group compared with the Reference sleep group (at the indicated coordinates). The mean skeleton of fractional anisotropy is shown in green. The white matter tracts include the superior Corona radiata and the body of the corpus callosum. The coordinates are presented in Montreal Neurological Institute (MNI) template space.
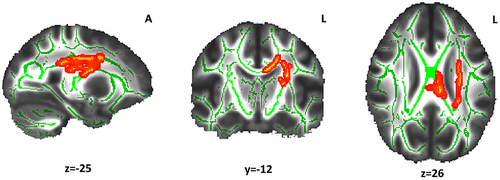
Figure 3 Radial diffusivity differences between the Long and Reference sleep groups. The light-blue color indicates the white matter clusters that showed higher radial diffusivity values (α ≤ 0.05, threshold-free cluster enhancement and family-wise error correction) in the Long sleep group compared with the Reference sleep group (at the indicated coordinates). The mean skeleton of FA is shown in green. The white matter tract includes the body of corpus callosum. Coordinates are presented in Montreal Neurological Institute (MNI) template space.
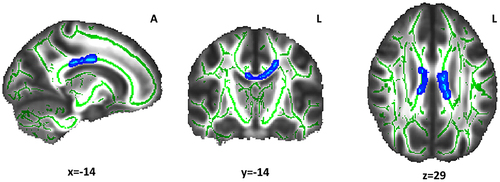
Discussion
This study provides evidence regarding white matter microstructure characteristics in relation to sleep amount in young adults. Compared with the Reference sleep group (6.5–8.0 h), the Long sleep group (>8 h) showed lower white matter integrity. This group also presented reduced sleep consolidation (higher WASO). The pattern of reduced FA and increased RD with longer TST suggests an association between longer sleep and lower integrity of myelin sheaths.
Relative to the Reference sleep group, the Long sleep group showed lower white matter integrity in tracts linked to sleep regulation. In this regard, the corona radiata contains ascending and descending fibers that transmit neural information to and from the cerebral cortex that relates to SWC patterns,Citation50,Citation51 and is also involved with emotional processing,Citation51 attention, and cognitive control.Citation50 This major white matter tract is interconnected in the hemispheres via the corpus callosum.Citation52 This tract—the largest brain pathway to transfer interhemispheric electrical activity—is a key player in the regulation of ultradian rhythms, sleep initiation and maintenance,Citation53 rapid-eye-movement (REM) sleep,Citation54 and sleep electroencephalographic (EEG) activity,Citation55 including slow wave propagation within the deep stage (N3) of non-REM sleep.Citation56,Citation57 Some studies have proposed that the corpus callosum white matter integrity might be sensitive to poor sleep quality.Citation58,Citation59 The superior longitudinal fasciculus is constituted by fibers that connect the frontal lobe to the parietal, temporal, and occipital lobesCitation60 and is involved in the relation between sleep and cognitive performance.Citation61,Citation62 Abnormalities in white matter integrity of this tract have been identified in patients with primary insomniaCitation63,Citation64 and associated with disrupted sleep patterns.Citation58,Citation65 Alterations in the white matter of the anterior thalamic radiation, which connects the thalamus and the frontal lobe,Citation66 have been linked to poor sleep quality,Citation67 sleep deprivation,Citation68 and sleep spindles activity.Citation69
Because sleep fragmentation in the Long sleep group occurred mainly in the second half of the night, we suggest that REM sleepCitation70,Citation71 is involved in the reduced integrity of white matter microstructure.Citation54 Even though higher WASO has already been related to reduce white matter microstructure,Citation23,Citation72 particularly in the corpus callosum,Citation23 the location of sleep interruptions within the nighttime sleep could provide insights regarding the role of specific sleep stages in white matter. Time spent in REM sleep is positively associated with white matter integrity,Citation54 which could be explained by the fact that the proliferation of oligodendrocyte precursor cells, which form new myelin, is related to time spent in this sleep stage.Citation15
Other studies have reported robust relations between long sleep duration and several unhealthy outcomes, including cognitive performance, diabetes mellitus, cardiovascular disease, stroke, coronary heart disease, obesity, and all-cause mortality.Citation65,Citation73,Citation74 It has been proposed that long sleep duration could be secondary or occur in conjunction with factors like age, gender, socioeconomic status, and sleep disorders.Citation75 However, the relations between long sleep and health outcomes remained significant after adjustment of these confounders in large studies.Citation76 To the best of our knowledge, this is the first study to show differences in white matter integrity in young adults who are long sleepers. In our study, the groups were similar in background characteristics, and our results could provide insight into possible mechanisms (white matter integrity) that might help explain these relations.
Long sleep is related to poor sleep quality.Citation77 Although our sleep groups did not show significant differences in the Pittsburgh Sleep Quality Index, it is known that lower self-reported sleep quality in adults is explained mainly by insomnia, anxiety, and depressive symptoms, rather than by actigraphic variables of sleep.Citation78 Moreover, perception of good sleep is related to age and objective measures of increase N2 stage time, higher sleep efficiency, and lower sleep fragmentation.Citation79,Citation80 In this study, the Long sleep group had higher sleep fragmentation in the nocturnal period than the Reference group, which could suggest the fact that sleep quality is a key factor in the maintenance of brain microstructure.
In contrast to other studies,Citation21,Citation65,Citation68 we did not identify differences in white matter indices between the Short and Reference sleep groups. With regard to this result, it is relevant to note that other reports did not find a significant relation between sleep and white matter integrity.Citation58,Citation81 Some studies showing an association between white matter integrity and short sleep duration had been performed by using self-reported sleep data,Citation22,Citation64,Citation82,Citation83 comparing only short and long sleep groups,Citation50 and included participants with mood disordersCitation28,Citation58,Citation84 and wider age ranges or older participants relative to our study.Citation22,Citation23,Citation82,Citation83 Even though our participants are young adults in a narrow age range, with similar characteristics ( and ) and have been assessed with an objective method, our hypothesized differences in white matter microstructure between the Short and Reference sleep groups were not apparent.
Excluding the corpus callosum, the brain regions with lower white matter integrity are located in the left hemisphere, another unexpected result. Some studies suggest a left hemisphere dominance in the waking stateCitation85 such that in situations of increased sleep pressure (sleep restriction/deprivation), a resetting mechanism regulated by prefrontal connectivity in this hemisphere might play a role in brain activity regulation.Citation86 In a study of white matter microstructure of the circadian tract (connecting the suprachiasmatic nucleus and lateral medulla) in the left hemisphere, Koller et alCitation87 found that daytime sleepiness correlated strongly with FA of this pathway. Moreover, lower levels of FA in the left superior longitudinal fasciculus have been related to reduced resistance to sleep deprivation (psychomotor vigilance performance monitoring).Citation62 In light of this information, we suggest that lower white matter integrity in the left hemisphere could relate to more fragmented sleep, greater sleep debt, and, therefore, increased daytime sleepiness. However, it is important to note that increased somnolence was not apparent in the Short sleep group based on the Epworth Sleepiness Scale results.
The limitations of our study include its cross-sectional nature, which precludes inferences regarding causality. We did not include information about other health variables or behaviors (physical activity, substance abuse, inflammation markers, etc.) that could affect white matter composition or sleep-wake characteristics. Regarding the strengths, we highlight the sample size with a narrow age range and the application of appropriate actigraphic recordings and neuroimaging studies (DTI). Our results require replication studies with larger samples.
Conclusion
To conclude, our findings suggest an association between long sleep duration and decreased integrity of white matter microstructure. This group also had increased WASO in the overall period and a higher number of wake episodes in the second half of the night, suggesting lower sleep quality. Taking into account that myelin is continuously remodeled in the brain,Citation8 SWC may be a key factor involved in its formation and maintenance. Further, considering the growing prevalence of sleep problems worldwide, the present study provides support to the relevance of nighttime sleep-wake patterns for healthy brain structure and functioning.
Disclosure
Sussanne Reyes reports grants from Chilean National Fund for Scientific and Technological Development and National Institutes of Health, during the conduct of the study. Carolina de Medeiros Rimkus reports grants from MS International Federation and personal fees from BCTRIMS, outside the submitted work. The author reports no other potential conflicts of interest in this work.
Acknowledgments
We would like to express our gratitude to the individuals whose participation made this study possible. We also thank technicians of the Sleep and Functional Neurobiology Laboratory of INTA, University of Chile, for their contribution during the course of this study. The content is solely the responsibility of the authors and does not necessarily represent the official views of the National Institutes of Health (NIH).
Additional information
Funding
References
- Peirano P, Algarı́n C, Uauy R. Sleep-wake states and their regulatory mechanisms throughout early human development. J Pediatr. 2003;143(4):70–79. doi:10.1067/S0022-3476(03)00404-9
- Pace-Schott EF, Hobson JA. The neurobiology of sleep: genetics, cellular physiology and subcortical networks. Nat Rev Neurosci. 2002;3(8):591–605. doi:10.1038/nrn895
- Fernandez-Mendoza J, Calhoun S, Bixler EO, et al. Insomnia with objective short sleep duration is associated with deficits in neuropsychological performance: a general population study. Sleep. 2010;33(4):459–465. doi:10.1093/sleep/33.4.459
- Medic G, Wille M, Hemels MEH. Short- and long-term health consequences of sleep disruption. Nat Sci Sleep. 2017;9:151–161. doi:10.2147/NSS.S134864
- Barone DA, Krieger AC. The function of sleep. AIMS Neurosci. 2015;2(2):71–90. doi:10.3934/Neuroscience.2015.2.71
- Zhu G, Cassidy S, Hiden H, et al. Exploration of sleep as a specific risk factor for poor metabolic and mental health: a UK biobank study of 84,404 Participants. Nat Sci Sleep. 2021;13:1903–1912. doi:10.2147/NSS.S323160
- Fields DR. Change in the brain’s white matter: the role of the brain’s white matter in active learning and memory may be underestimated. Science. 2010;330(6005):768–769. doi:10.1126/science.1199139
- de Vivo L, Bellesi M. The role of sleep and wakefulness in myelin plasticity. Glia. 2019;67(11):2142–2152. doi:10.1002/glia.23667
- Jarjour AA, Velichkova AN, Boyd A, et al. The formation of paranodal spirals at the ends of CNS myelin sheaths requires the planar polarity protein Vangl2. Glia. 2020;68(9):1840–1858. doi:10.1002/glia.23809
- Bull SJ, Bin JM, Beaumont E, et al. Progressive disorganization of paranodal junctions and compact myelin due to loss of DCC expression by oligodendrocytes. J Neurosci. 2014;34(29):9768–9778. doi:10.1523/JNEUROSCI.0448-14.2014
- Boyle MET, Berglund EO, Murai KK, Weber L, Peles E, Ranscht B. Contactin orchestrates assembly of the septate-like junctions at the paranode in myelinated peripheral nerve. Neuron. 2001;30(2):385–397. doi:10.1016/S0896-6273(01)
- Simmonds DJ, Hallquist MN, Asato M, Luna B. Developmental stages and sex differences of white matter and behavioral development through adolescence: a longitudinal diffusion tensor imaging (DTI) study. Neuroimage. 2014;92:356–368. doi:10.1016/j.neuroimage.2013.12.044
- Wassenaar TM, Yaffe K, van der Werf YD, Sexton CE. Associations between modifiable risk factors and white matter of the aging brain: insights from diffusion tensor imaging studies. Neurobiol Aging. 2019;80:56–70. doi:10.1016/j.neurobiolaging.2019.04.006
- Scholz J, Tomassini V, Johansen-Berg H. Individual differences in white matter microstructure in the healthy brain. In: Johansen-Berg H, Behrens TE, editors. Diffusion MRI: From Quantitative Measurement to in vivo Neuroanatomy. 2nd ed. Elsevier; 2013:301–316. doi:10.1016/B978-0-12-396460-1.00014-7
- Bellesi M, Pfister-Genskow M, Maret S, Keles S, Tononi G, Cirelli C. Effects of sleep and wake on oligodendrocytes and their precursors. J Neurosci. 2013;33(36):14288–14300. doi:10.1523/JNEUROSCI.5102-12.2013
- Cirelli C, Faraguna U, Tononi G. Changes in brain gene expression after long-term sleep deprivation. J Neurochem. 2006;98(5):1632–1645. doi:10.1111/j.1471-4159.2006.04058.x
- Bellesi M, Haswell JD, De Vivo L, et al. Myelin modifications after chronic sleep loss in adolescent mice. Sleep. 2018;41(5):1–11. doi:10.1093/sleep/zsy034
- Dutta DJ, Woo DH, Lee PR, et al. Regulation of myelin structure and conduction velocity by perinodal astrocytes. Proc Natl Acad Sci U S A. 2019;116(25):12574. doi:10.1073/pnas.1908361116
- Alexander AL, Hurley SA, Samsonov AA, et al. Characterization of cerebral white matter properties using quantitative magnetic resonance imaging stains. Brain Connect. 2011;1(6):423–446. doi:10.1089/brain.2011.0071
- Kullmann S, Schweizer F, Veit R, Fritsche A, Preissl H. Compromised white matter integrity in obesity. Obes Rev. 2015;16:273–281. doi:10.1111/obr.12248
- Elvsåshagen T, Norbom LB, Pedersen P, et al. Widespread changes in white matter microstructure after a day of waking and sleep deprivation. PLoS One. 2015;10(5):e0127351. doi:10.1371/journal.pone.0127351
- Yaffe K, Nasrallah I, Hoang TD, et al. Sleep duration and white matter quality in middle-aged adults. Sleep. 2016;39(9):1743–1747. doi:10.5665/sleep.6104
- Kocevska D, Tiemeier H, Lysen TS, et al. The prospective association of objectively measured sleep and cerebral white matter microstructure in middle-aged and older persons. Sleep. 2019;42(10):1–8. doi:10.1093/sleep/zsz140
- Bangen KJ, Delano-Wood L, Deoni SCL, et al. Decreased myelin content of the fornix predicts poorer memory performance beyond vascular risk, hippocampal volume, and fractional anisotropy in nondemented older adults. Brain Imaging Behav. 2021;15(5):2563–2571. doi:10.1007/s11682-021-00458-z
- Mak E, Dounavi ME, Low A, et al. Proximity to dementia onset and multi-modal neuroimaging changes: the prevent-dementia study. Neuroimage. 2021;229:117749. doi:10.1016/j.neuroimage.2021.117749
- Lo JC, Groeger JA, Cheng GH, Dijk DJ, Chee MW. Self-reported sleep duration and cognitive performance in older adults: a systematic review and meta-analysis. Sleep Med. 2016;17:87–98. doi:10.1016/j.sleep.2015.08.021
- Ramos AR, Dong C, Rundek T, et al. Sleep duration is associated with white matter hyperintensity volume in older adults: the Northern Manhattan Study. J Sleep Res. 2014;23(5):524–530. doi:10.1111/jsr.12177
- Verkooijen S, Stevelink R, Abramovic L, et al. The association of sleep and physical activity with integrity of white matter microstructure in bipolar disorder patients and healthy controls. Psychiatry Res Neuroimaging. 2017;262:71–80. doi:10.1016/j.pscychresns.2017.01.013
- Liang Y, Qu LB, Liu H. Non-linear associations between sleep duration and the risks of mild cognitive impairment/dementia and cognitive decline: a dose–response meta-analysis of observational studies. Aging Clin Exp Res. 2019;31(3):309–320. doi:10.1007/s40520-018-1005-y
- Xu W, Tan CC, Zou JJ, Cao XP, Tan L. Sleep problems and risk of all-cause cognitive decline or dementia: an updated systematic review and meta-Analysis. J Neurol Neurosurg Psychiatry. 2019;236–244. doi:10.1136/jnnp-2019-321896
- Sabia S, Fayosse A, Dumurgier J, et al. Association of sleep duration in middle and old age with incidence of dementia. Nat Commun. 2021;12(1):2289. doi:10.1038/s41467-021-22354-2
- Algarín C, Karunakaran KD, Reyes S, et al. Differences on brain connectivity in adulthood are present in subjects with iron deficiency anemia in infancy. Front Aging Neurosci. 2017;9:54. doi:10.3389/fnagi.2017.00054
- Lozoff B, Castillo M, Clark KM, Smith JB, Sturza J. Iron supplementation in infancy contributes to more adaptive behavior at 10 years of age. J Nutr. 2014;144(11):838–846. doi:10.3945/jn.113.182048.adulthood
- Doom JR, Gahagan S, East PL, Encina P, Delva J, Lozoff B. Adolescent internalizing, externalizing, and social problems following iron deficiency at 12–18 months: the role of maternal responsiveness. Child Dev. 2020;91(3):e545–e562. doi:10.1111/cdev.13266
- Johns MW. A new method for measuring daytime sleepiness: the Epworth sleepiness scale. Sleep. 1991;14(6):540–545. doi:10.1093/sleep/14.6.540
- Buysse DJ, Reynolds CF, Monk TH, Berman SR, Kupfer DJ. The Pittsburgh Sleep Quality Index: a new instrument for psychiatric practice and research. Psychiatry Res. 1989;28(2):193–213. doi:10.1016/0165-1781(89)
- Ancoli-Israel S, Cole R, Alessi C, Chambers M, Moorcroft W, Pollak CP. The role of actigraphy in the study of sleep and circadian rhythms. Sleep. 2003;26(3):342–392. doi:10.1093/sleep/26.3.342
- Reyes S, Algarín C, Bunout D, Peirano P. Sleep/wake patterns and physical performance in older adults. Aging Clin Exp Res. 2013;25(2):175–181. doi:10.1007/s40520-013-0028-7
- Weikel JC, Wichniak A, Ising M, et al. Ghrelin promotes slow-wave sleep in humans. Am J Physiol Endocrinol Metab. 2003;284(2):E407–E415. doi:10.1152/ajpendo.00184.2002
- Peirano P, Algarín C, Garrido M, Lozoff B. Iron deficiency anemia in infancy is associated with altered temporal organization of sleep states in childhood. Pediatr Res. 2007;62(6):715–719. doi:10.1203/PDR.0b013e3181586aef
- Du M, Liu M, Liu J. The association between sleep duration and the risk of mortality in the Chinese older adults: a national cohort study. J Clin Sleep Med. 2021;17(9):1821–1829. doi:10.5664/jcsm.9278
- Smith SM, Jenkinson M, Woolrich MW, et al. Advances in functional and structural MR image analysis and implementation as FSL. Neuroimage. 2004;23(Suppl 1):208–219. doi:10.1016/j.neuroimage.2004.07.051
- Smith SM, Jenkinson M, Johansen-Berg H, et al. Tract-based spatial statistics: voxelwise analysis of multi-subject diffusion data. Neuroimage. 2006;31(4):1487–1505. doi:10.1016/j.neuroimage.2006.02.024
- Alexander AL, Lee JE, Lazar M, Field AS. Diffusion tensor imaging of the brain. Neurotherapeutics. 2007;4(3):316–329. doi:10.1016/j.nurt.2007.05.011
- Winklewski PJ, Sabisz A, Naumczyk P, Jodzio K, Szurowska E, Szarmach A. Understanding the physiopathology behind axial and radial diffusivity changes-what do we know? Front Neurol. 2018;9:92. doi:10.3389/fneur.2018.00092
- Winkler AM, Ridgway GR, Webster MA, Smith SM, Nichols TE. Permutation inference for the general linear model. Neuroimage. 2014;92:381–397. doi:10.1016/j.neuroimage.2014.01.060
- Smith SM, Nichols TE. Threshold-free cluster enhancement: addressing problems of smoothing, threshold dependence and localisation in cluster inference. Neuroimage. 2009;44(1):83–98. doi:10.1016/j.neuroimage.2008.03.061
- Mori S, Oishi K, Jiang H, et al. Stereotaxic white matter atlas based on diffusion tensor imaging in an ICBM template. Neuroimage. 2008;40(2):570–582. doi:10.1016/j.neuroimage.2007.12.035
- Mori S, Oishi K, Faria AV. White matter atlases based on diffusion tensor imaging. Curr Opin Neurol. 2009;22(4):362–369. doi:10.1097/WCO.0b013e32832d954b
- Khalsa S, Hale JR, Goldstone A, et al. Habitual sleep durations and subjective sleep quality predict white matter differences in the human brain. Neurobiol Sleep Circadian Rhythm. 2017;3:17–25. doi:10.1016/j.nbscr.2017.03.001
- Yang Y, Zhu DM, Zhang C, et al. Brain structural and functional alterations specific to low sleep efficiency in major depressive disorder. Front Neurosci. 2020;14:50. doi:10.3389/fnins.2020.00050
- Gage NM, Baars BJ. The brain. In: Fundamentals of Cognitive Neuroscience. Academic Press; 2018:17–52. doi:10.1016/B978-0-12-803813-0.00002-7
- Melloni EMT, Poletti S, Dallaspezia S, et al. Changes of white matter microstructure after successful treatment of bipolar depression. J Affect Disord. 2020;274:1049–1056. doi:10.1016/j.jad.2020.05.146
- Altendahl M, Cotter DL, Staffaroni AM, et al. REM sleep is associated with white matter integrity in cognitively healthy, older adults. PLoS One. 2020;15(7):e0235395. doi:10.1371/journal.pone.0235395
- Corsi-Cabrera M, Ondarza R, Martínez-Gutiérrez V. Role of corpus callosum in interhemispheric coherent activity during sleep. Clin Neurophysiol. 2006;117(8):1826–1835. doi:10.1016/j.clinph.2006.05.008
- Ingram DG, Churchill SS. Sleep problems in children with agenesis of the corpus callosum. Pediatr Neurol. 2017;67:85–90. doi:10.1016/j.pediatrneurol.2016.10.002
- Avvenuti G, Handjaras G, Betta M, et al. Integrity of corpus callosum is essential for the cross-hemispheric propagation of sleep slow waves: a high-density EEG study in split-brain patients. bioRxiv. 2019;40(29):5589–5603. doi:10.1101/756676
- Li C, Schreiber J, Bittner N, et al. White matter microstructure underlies the effects of sleep quality and life stress on depression symptomatology in older adults. Front Aging Neurosci. 2020;12:578037. doi:10.3389/fnagi.2020.578037
- McMahon M, Malneedi Y, Worthy DA, Schnyer DM. Rest-activity rhythms and white matter microstructure across the lifespan. Sleep. 2021;44(6):zsaa266. doi:10.1093/sleep/zsaa266
- Wycoco V, Shroff M, Sudhakar S, Lee W. White matter anatomy. What the radiologist needs to know. Neuroimaging Clin N Am. 2013;23(2):197–216. doi:10.1016/j.nic.2012.12.002
- Zhu Y, Wang L, Xi Y, et al. White matter microstructural properties are related to inter-individual differences in cognitive instability after sleep deprivation. Neuroscience. 2017;365:206–216. doi:10.1016/j.neuroscience.2017.09.047
- Cui J, Tkachenko O, Gogel H, et al. Microstructure of frontoparietal connections predicts individual resistance to sleep deprivation. Neuroimage. 2015;106:123–133. doi:10.1016/j.neuroimage.2014.11.035
- Sanjari Moghaddam H, Mohammadi E, Dolatshahi M, et al. White matter microstructural abnormalities in primary insomnia: a systematic review of diffusion tensor imaging studies. Prog Neuropsychopharmacology Biol Psychiatry. 2021;105:110132. doi:10.1016/j.pnpbp.2020.110132
- Cai W, Zhao M, Liu J, Liu B, Yu D, Yuan K. Right arcuate fasciculus and superior longitudinal fasciculus abnormalities in primary insomnia. Brain Imaging Behav. 2019;13(6):1746–1755. doi:10.1007/s11682-019-00160-1
- Grumbach P, Opel N, Martin S, et al. Sleep duration is associated with white matter microstructure and cognitive performance in healthy adults. Hum Brain Mapp. 2020;41(15):4397–4405. doi:10.1002/hbm.25132
- George K, Das M. J. Neuroanatomy, Thalamocortical Radiations. StatPearls; 2019. http://www.ncbi.nlm.nih.gov/pubmed/31536305. Accessed August 2, 2022.
- Sun H, Xue B, Peng M, et al. Abnormal neurite orientation dispersion and density imaging of white matter in children with primary nocturnal enuresis. NeuroImage Clin. 2020;28:102389. doi:10.1016/j.nicl.2020.102389
- Voldsbekk I, Groote I, Zak N, et al. Sleep and sleep deprivation differentially alter white matter microstructure: a mixed model design utilising advanced diffusion modelling. bioRxiv. 2020. doi:10.1101/2020.08.24.259432
- Gaudreault P-O, Gosselin N, Lafortune M, et al. The association between white matter and sleep spindles differs in young and older individuals. Sleep. 2018;41:9. doi:10.1093/sleep/zsy113
- Yetton BD, McDevitt EA, Cellini N, Shelton C, Mednick SC. Quantifying sleep architecture dynamics and individual differences using big data and Bayesian networks. PLoS One. 2018;13(4):e0194604. doi:10.1371/journal.pone.0194604
- Colten HR, Altevogt BM, eds. Section 2 - Sleep Physiology. In: Sleep Disorders and Sleep Deprivation: An Unmet Public Health Problem: National Academies Press; 2006. 33–54. doi:10.17226/11617
- Baillet M, Dilharreguy B, Pérès K, Dartigues JF, Mayo W, Catheline G. Activity/rest cycle and disturbances of structural backbone of cerebral networks in aging. Neuroimage. 2017;146:814–820. doi:10.1016/j.neuroimage.2016.09.051
- Jike M, Itani O, Watanabe N, Buysse DJ, Kaneita Y. Long sleep duration and health outcomes: a systematic review, meta-analysis and meta-regression. Sleep Med Rev. 2018;39:25–36. doi:10.1016/j.smrv.2017.06.011
- Reyes S, Algarín C, Lozoff B, Peigneux P, Peirano P. Sleep and motor sequence learning consolidation in former iron deficient anemic adolescents. Sleep Med. 2019;64:116–122. doi:10.1016/j.sleep.2019.05.023
- Covassin N, Singh P. Sleep duration and cardiovascular disease risk: epidemiologic and experimental evidence. Sleep Med Clin. 2016;11(1):81–89. doi:10.1016/j.jsmc.2015.10.007
- Liu Y, Wheaton AG, Chapman DP, Croft JB. Sleep duration and chronic diseases among us adults age 45 years and older: evidence from the 2010 behavioral risk factor surveillance system. Sleep. 2013;36(10):1421–1427. doi:10.5665/sleep.3028
- Andreasson A, Axelsson J, Bosch JA, Balter LJ. Poor sleep quality is associated with worse self-rated health in long sleep duration but not short sleep duration. Sleep Med. 2021;88:262–266. doi:10.1016/j.sleep.2021.10.028
- Johnson DA, Javaheri S, Guo N, et al. Objective measures of sleep apnea and actigraphy-based sleep characteristics as correlates of subjective sleep quality in an epidemiologic study: the Jackson Heart Sleep Study. Psychosom Med. 2020;82(3):324–330. doi:10.1097/PSY.0000000000000778
- Åkerstedt T, Schwarz J, Gruber G, Lindberg E, Theorell-Haglöw J. The relation between polysomnography and subjective sleep and its dependence on age – poor sleep may become good sleep. J Sleep Res. 2016;25(5):565–570. doi:10.1111/jsr.12407
- Lok R, Chawra D, Hon F, Ha M, Kaplan KA, Zeitzer JM. Objective underpinnings of self-reported sleep quality in middle-aged and older adults: the importance of N2 and wakefulness. Biol Psychol. 2022;170:108290. doi:10.1016/j.biopsycho.2022.108290
- Zitser J, Anatürk M, Zsoldos E, et al. Sleep duration over 28 years, cognition, gray matter volume, and white matter microstructure: a prospective cohort study. Sleep. 2020;43(5):1–7. doi:10.1093/sleep/zsz290
- Sexton CE, Zsoldos E, Filippini N, et al. Associations between self-reported sleep quality and white matter in community-dwelling older adults: a prospective cohort study. Hum Brain Mapp. 2017;38(11):5465–5473. doi:10.1002/hbm.23739
- Amorim L, Magalhães R, Coelho A, et al. Poor sleep quality associates with decreased functional and structural brain connectivity in normative aging: a MRI multimodal approach. Front Aging Neurosci. 2018;10:375. doi:10.3389/fnagi.2018.00375
- Benedetti F, Melloni EMT, Dallaspezia S, et al. Night sleep influences white matter microstructure in bipolar depression. J Affect Disord. 2017;218:380–387. doi:10.1016/j.jad.2017.05.008
- Casagrande M, Bertini M. Night-time right hemisphere superiority and daytime left hemisphere superiority: a repatterning of laterality across wake-sleep-wake states. Biol Psychol. 2008;77(3):337–342. doi:10.1016/j.biopsycho.2007.11.007
- Borragán G, Guerrero-Mosquera C, Guillaume C, Slama H, Peigneux P. Decreased prefrontal connectivity parallels cognitive fatigue-related performance decline after sleep deprivation. An optical imaging study. Biol Psychol. 2019;144:115–124. doi:10.1016/j.biopsycho.2019.03.004
- Koller K, Rafal RD, Mullins PG. Circadian circuits in humans: white matter microstructure predicts daytime sleepiness. Cortex. 2020;122:97–107. doi:10.1016/j.cortex.2019.01.011