Abstract
Sleep and energy balance are essential for health. The two processes act in concert to regulate central and peripheral homeostasis. During sleep, energy is conserved due to suspended activity, movement, and sensory responses, and is redirected to restore and replenish proteins and their assemblies into cellular structures. During wakefulness, various energy-demanding activities lead to hunger. Thus, hunger promotes arousal, and subsequent feeding, followed by satiety that promotes sleep via changes in neuroendocrine or neuropeptide signals. These signals overlap with circuits of sleep-wakefulness, feeding, and energy expenditure. Here, we will briefly review the literature that describes the interplay between the circadian system, sleep-wake, and feeding-fasting cycles that are needed to maintain energy balance and a healthy metabolic profile. In doing so, we describe the neuroendocrine, hormonal/peptide signals that integrate sleep and feeding behavior with energy metabolism.
Overview of the relationship between energy balance and sleep
Sleep and energy balance are essential for health. The two processes act in concert to regulate central and peripheral homeostasis. Epidemiological and experimental evidence have linked insufficient sleep to metabolic disorders.Citation1,Citation2 During sleep, energy is conserved due to suspended activity, movement, and sensory responses, and is redirected to restore and replenish proteins and their assemblies into cellular structures. During wakefulness, various energy-demanding activities lead to hunger. Thus, hunger promotes arousal, and subsequent feeding, followed by satiety that promotes sleep via changes in neuroendocrine or neuropeptide signals. These signals overlap with circuits of sleep-wakefulness, feeding, and energy expenditure. A diurnal rhythm maintained by the internal circadian clock in response to the external environmental cues establishes rhythmicity of the physiological processes and hormonal release. These alterations are associated with the sleep-wake-state changesCitation3,Citation4 and the feeding-fasting cycles.Citation5 Together, they maintain the energy balance that is critical for health.Citation6 Disturbing one of the cycles often results in a cascade of effects, resulting in imbalance contributing to numerous metabolic disorders. Chronic circadian misalignments not only influence sleep but also influence several other systems including the immune system,Citation7 appetitive hormones, and energy balance.Citation8–Citation10 Another promising evidence has emerged from the epidemiological studies using genome-wide associations revealing close linkage between sleep/circadian and metabolism-related genes.Citation11,Citation12 Thus, the circadian rhythmicity, sleep-wake, and feeding behavior are integral to bodily energy homeostasis.
Here, we will briefly review the literature that describes the interplay between the circadian system, sleep-wake, and feeding-fasting cycles that are needed to maintain energy balance and a healthy metabolic profile (). We will also describe the brain centers that integrate these three systems by regulating the autonomic and endocrine signals and discuss their functional interactions. The main focus of this article will be on the interaction between the neuronal and endocrine signals from the gastrointestinal tract and brown adipose tissue (BAT) to influence sleep-wake behavior and energy metabolism. Finally, we will review the causalities and the biological basis of the combined manifestations of sleep disturbances/disorders and briefly discuss the potential therapeutic perspectives.
Interactive regulation of circadian, sleep, and feeding behavior
Sleep/rest and wake/active behavioral states are observed in all living organisms. The state changes are assessed by recording the cortical electrical activity patterns using electroencephalogram (EEG) and skeletal muscle activity using electromyogram in mammals. Wakefulness is defined by low-voltage fast EEG activity and high muscle tone, whereas the non-rapid eye movement (NREM) sleep is defined by high-amplitude low-frequency EEG and decreased muscle tone, and rapid eye movement (REM) sleep is characterized by low-voltage fast EEG activity accompanied by complete loss of muscle tone.Citation5 These assessments preclude sleep assessments in animals that do not have a well-defined cortex. However, researchers have proposed specific behavioral criteria to describe the presence of sleep-like states in several species of fish, reptiles, amphibians, and some invertebrates.Citation13 One common factor that remains constant across all species is the circadian rhythmicity in behavioral state changes.
Since the first description of the circadian “clock” (circadian locomotor output cycles kaput) gene in the suprachiasmatic nuclei (SCN) within the brain,Citation14 other clocks in the peripheral organs associated with energy regulation have been recognized that follow the master clock to maintain physiological rhythmicity.Citation15 Homozygous clock mutant mice exhibit a significant increase in wakefulness by ~2 hours/24 hours independent of light-dark cues.Citation16 These mice also show significantly attenuated diurnal feeding and locomotor rhythms, hyperphagia, and obesity. Most importantly, the mutant mice exhibit hyperglycemia accompanied by insufficient insulin production; a condition associated with type 2 diabetes in humans.Citation17 These characteristics of clock mutant mice provide credence to the fundamental idea of the interactive manifestations of circadian rhythmicity, sleep-wake regulation, and feeding in order to maintain the physiological energy balance.
In the brain, the levels of the high energy molecule, adenosine triphosphate, exhibit a diurnal pattern with highest levels during sleep when the neuronal activity is decreased in the wake-associated areas.Citation18 The hypothalamus serves as a multifunctional center regulating circadian, sleep, and feeding behaviors and also integrates central and peripheral neuroendocrine, endocrine, and peptide signals. The bodily homeostatic mechanisms and sleep homeostasis rely on the complex integrative activity of various neuronal subgroups within hypothalamus.Citation19 Anatomical studies further substantiated by lesion studies describe how the circadian center, the SCN, functionally connects to sleep and feeding centers within hypothalamus. The efferent projections from the SCN target the dorsal and ventral subparaventricular zone (SPZ) with a subset of the axons extending to the dorsomedial nucleus (DMH) in the hypothalamus.Citation20 Thus, the ventral SPZ serves as a relay center and regulates rhythmicity in sleep and feeding as lesions in this area eliminate circadian rhythms of sleep-wakefulness, locomotor activity, and feeding.Citation21,Citation22 Although very few direct axonal inputs from SCN and ventral SPZ have been detected to the sleep-regulating ventrolateral preoptic area (VLPO), a larger contingent of the axons project to the DMH. The DMH sends divergent efferents to the 1) sleep regulation center VLPO; 2) paraventricular nucleus (PVN) containing the neurons synthesizing corticotropin-releasing hormone (CRH) and neurons that mediate preganglionic output to autonomous nervous system; and 3) lateral hypothalamus, an area that contains the hunger-stimulating and wakefulness-promoting peptide orexin (hypocretin) and melanin-concentrating hormone ().Citation19 The DMH also receives inputs from the arcuate nucleus, which is recognized for regulating food intake and energy expenditure by sensing the peripheral energy status through the “satiety hormone” leptinCitation23 and the functionally opposite “hunger peptide” ghrelin.Citation24 The ventromedial nucleus of the hypothalamus (VMH) lies close to the DMH, arcuate nucleus, and third ventricle. It has no known neuroendocrine projections, but is directly involved in feeding behavior and regulating body weight.Citation25,Citation26 Lesions in the VMH region in rats lead to hyperphagia and weight gain.Citation27 Brain-derived neurotrophic factor (BDNF) is a neurotrophin whose expression is regulated by signals from the melanocortin 4 receptor (MC4R).Citation28 Compared with other brain regions, the expression of BDNF is most dense in the VMH, and this region also mediates the ability of BDNF to increase energy expenditure.Citation29 As BDNF regulates sleep onset () along with hedonic food intake, this ventromedial hypothalamic region is likely to closely interact with the overlapping circuits of energy expenditure and sleep regulation.Citation30,Citation31 Thus, the hypothalamus is poised to serve as a center that integrates circadian, sleep-wake regulation, and feeding behavior ().
Figure 2 The neuronal connections between circadian, sleep, and feeding centers within the hypothalamus.
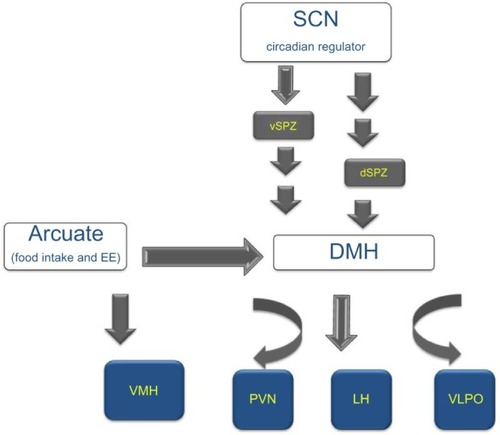
Figure 3 The interaction between peripheral and central signals regulating circadian, sleep, and feeding centers within hypothalamus.
Abbreviations: AgRP, agouti-related protein; BDNF, brain-derived neurotrophic factor; CART, cocaine- and amphetamine-regulated transcript; CRH, corticotropin-releasing hormone; DMH, dorsomedial nucleus; LH, lateral hypothalamus; MCH, melanin-concentrating hormone; NPY, neuropeptide Y; POMC, proopiomelanocortin; PVN, paraventricular/nucleus; VMH, ventromedial hypothalamus; GLP1, glucagon like peptide 1; CCK, Cholecystokinin.
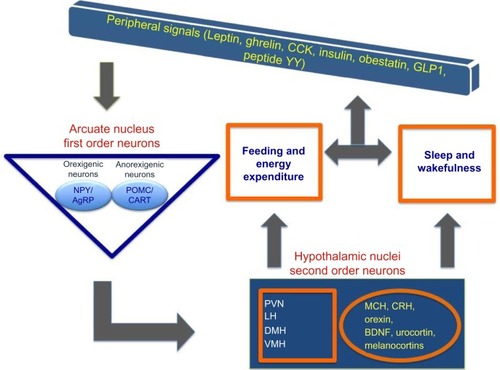
Table 1 Central and peripheral signals involved in energy balance and sleep regulation
Neuronal and endocrine signals from the gastrointestinal tract involved in sleep regulation
Multiple neuronal and endocrine signals have been identified for their direct or indirect roles in integrating sleep and energy balance via feeding mechanisms. Here, we describe the three most recognized peptides, orexin, leptin, and ghrelin, followed by a short summary of others that are included in .
Orexin is important for both sleep-wake regulation as well as feeding and energy balance. Mainly expressed in the perifornical region of the lateral hypothalamus, orexin exists in two forms, the neuropeptide orexin A (a 33 aminoacid peptide with two disulfide bonds) and orexin B (28 amino acid peptide) first described in 1998.Citation32,Citation33 The orexin system has a wide distribution of cognate receptors extending its effects on central as well as peripheral targets, thereby regulating various physiological mechanisms such as feeding, energy metabolism, arousal, onset of REM, reward, and autonomic function.Citation34–Citation38 Clinical studies have underscored the importance of orexin signaling in human pathophysiology, as abnormalities in this system can lead to disorders such as narcolepsy,Citation39 obstructive sleep apnea (OSA),Citation40 excessive daytime sleepiness secondary to traumatic brain injury,Citation41 post-traumatic stress disorder,Citation42 insomnia, and age-related changes in sleep and energy expenditure.Citation43–Citation45 The central administration of orexin dose-dependently increases food intake, waking time, motor activity, and metabolic rate, as well as heart rate and blood pressure in many species (reviewed in SiegelCitation46). Ablations of the orexinergic system lead to sleep disturbances. In 1999, two seminal papers reported that the absence of orexinergic signaling, either due to orexin (peptide) gene knockout (KO) in miceCitation47 or due to orexin receptor 2 mutation in dogs,Citation48 results in elevated wake to rapid eye movement sleep (REMS) transitions, and increased REMS during the active period, mimicking narcolepsy in humans. Human narcoleptic subjects contain low levels of orexin in cerebrospinal fluidCitation49,Citation50 and exhibit loss of orexin neurons.Citation39,Citation51,Citation52 In rats, lesioning orexin neurons also leads to a narcoleptic phenotype.Citation53 Further evidence for the association between orexin neurons and wakefulness is provided from the experiments where selective optogenetic stimulation of orexin neurons reduced latency to wakefulness and increased sleep-to-wake transitions.Citation54
Orexin is also known to link wake-state and feeding behavior. It activates overlapping neural substrates that modulate both arousal and feeding, thereby affecting energy homeostasis. Animal experiments have shown that intracerebral injections of orexin during the light (sleep) period induce feeding behavior in rodents as well as in zebrafishes.Citation32,Citation55,Citation56 A study showed that orexin-deficient mice not only suffer from narcolepsy, but also exhibit metabolic imbalance and are obese due to decreased energy expenditure despite the concomitant reduction in feeding.Citation43 Additionally, orexin neurons act as sensors of energy reserves; high extracellular glucose and leptin induce significant hyperpolarization whereas depleted glucose and ghrelin lead to depolarization of orexin neurons.Citation57–Citation59 The orexinergic (neuropeptide Y, NPY and agouti-related protein [AgRP]) and anorexinergic (proopiomelanocortin cocaine- and amphetamine-regulated transcript) neurons innervate and regulate orexin neurons.Citation60,Citation61 Thus, in concert with the other neuropeptides and hormones including melanin-concentrating hormone (MCH) and CRH ( and ), the orexinergic system serves as an integral part of the hypothalamic neuronal network that regulates feeding behavior and energy homeostasis.Citation62
In addition to orexin, two peptides from the peripheral system, leptin and ghrelin, have gained recognition for their opposing functions in the regulation of appetite, energy homeostasis, and sleep.Citation63 Leptin, an anorectic peptide, is secreted primarily from white adipose tissue and acts on the arcuate nucleus to inhibit NPY/AgRP neurons, and activate the proopiomelanocortin (POMC)/cocaine- and amphetamine-regulated transcript neurons. Ghrelin, secreted by the stomach has an opposite function. In hypothalamus, orexin, leptin, and ghrelin are the key peptides that link sleep-wake behavior to energy homeostasis. The first-order neurons from arcuate nucleus send orexigenic/anorexigenic signals to neurons projecting not only to the lateral hypothalamus as described earlier, but also to other hypothalamic nuclei (). A close association exists between the duration of sleep and the serum levels of leptin and ghrelin. Increasing sleep duration leads to an increase in leptin and a reciprocal decrease in ghrelin; the opposite is seen with short duration sleep.Citation64–Citation66 In controlled laboratory conditions, both acuteCitation67 and chronic partial sleep deprivation decrease serum leptin concentrations.Citation68 This relationship is suggested to be the basis of increased body mass index (BMI) and obesity with sleep loss in humans.Citation64 The common sleep disorder, OSA, is associated with low ghrelin and elevated leptin levels, a condition also observed in obese individuals.Citation69 Leptin is increasingly conceptualized as a biomarker for sleep disorders due to the close relationship between serum leptin levels and sleep durations; however, the topic is still debated due to some inconsistencies in the leptin levels reported in different studies.Citation70 However, in narcoleptic patients with abnormal REMs and excessive daytime sleepiness, the mean 24-hour plasma leptin levels show a significant decrease with the loss of nocturnal acrophase.Citation71 These observations, while establishing a relationship between the plasma levels of leptin or ghrelin with sleep and obesity, are not yet conclusive without a clear understanding of molecular regulations. The discovery of acyl ghrelin in stomach using reverse pharmacology is a noteworthy breakthrough. It is the only protein to be octanylated by ghrelin O-acyltransferase (GOAT).Citation72 Due to this unique post-translational modification, inhibition of ghrelin O-acyltransferase may provide a critical molecular target in developing novel therapeutics for obesity and type 2 diabetes and is unlikely to affect the synthesis of other proteins.
There are several other mediators of metabolism as listed in that regulate both energy metabolism and sleep. Sleep disorders such as OSA are linked to specific mutations in genes coding for neuroendocrine molecules or hormones. For example, a MC4R deficiency has been associated with OSA, and also with metabolic disorders along with obesity.Citation73,Citation74 The MC4R is expressed largely in the hypothalamus and is closely involved in appetite regulation, autonomic, and endocrine functions, as well as in insulin resistance.Citation75,Citation76
In humans, sleep loss has also been suggested to influence the choice of food, increasing preference for high-fat food facilitating weight gain.Citation77,Citation78 The feeding pattern and diet contents are also known to regulate the sleep quality and quantity due to the resulting influence on plasma levels of endocrine peptide.Citation79 For example, a high-fat diet increases sleepiness in humans.Citation80 Similarly, bariatric surgeries that impact the diet and associated endocrine factors can alter sleep in obese OSA patients. In a study of severely obese patients with OSA who underwent gastric bypass, the Epworth sleepiness score for the group fell from 14 (severe sleepiness) preoperatively to 5 (normal) at 1 month after surgery, a time when weight loss is expected to be just 10–12 pounds.Citation81 Although the association between sleep dysregulation, feeding, and energy metabolism is gaining much attention, the exact mechanisms and the interactions between various molecules need further investigation.
Neuronal and endocrine signals from BAT involved in sleep regulation
Known for its production of heat from uncoupling of mitochondrial oxidative phosphorylation, BAT is highly differentiated, and is uniquely characterized by the presence of uncoupling protein (UCP) 1 in large quantities. On activation, UCP1 allows rapid dissipation of chemical energy in the form of heat via the mitochondrial mechanism of maintaining proton flow across its inner membrane.Citation82 The energy transfer and this direct conversion from food to heat are under the control of the sympathetic nervous system and the release of norepinephrine. Brown fat plays a critical role in maintaining metabolic homeostasis by regulating energy expenditure and glucose disposal. The acute ability of this tissue to generate heat by non-shivering thermogenesis has been the focus of many studies over the past decade for its possible homeostatic and thermoregulatory role in small mammals as well as in humans.Citation83–Citation86 Although white fat deposition increases with a defect in BAT in small animals,Citation85 in humans, a higher BMI is associated with decreased BAT.Citation87,Citation88 It has been estimated that roughly 60 g of BAT can contribute up to 20% of total daily heat production in humans,Citation89 implying an important functional role in temperature regulation. Although our understanding of the physiological and functional roles of BAT is still relatively limited, it is known that it can be rapidly activated by cold exposureCitation90 and has a key role in overall energy balance regulation.Citation91 Systems that regulate energy balance and have a homeostatic role are often linked to the neural circuits that modulate sleep and sleep quality. Here, we focus on the interaction of sleep and temperature regulation via BAT.
It has been reported that activated BAT generates sleep-promoting signals, which are essential for restorative sleep after a prolonged period of wakefulness.Citation92 Sleep, particularly slow wave sleep, can be increased by specifically activating BAT thermogenesis.Citation93 The importance of the role of UCP1 in thermoregulatory role of BAT is further established in the studies from UCP1 KO mice. In response to sleep deprivation, an elevated UCP1 mRNA expression is observed in BAT along with increased NREM, REM, and slow wave activity.Citation92 Compared with wild type, the rebound changes that are characteristic of homeostatic sleep are significantly reduced in the UCP1 KO mice.Citation92 Similarly, chronic sleep deprivation in rats upregulates UCP1 gene expression in BAT.Citation94 Since UCP1 mediates heat production, these findings highlight the role of thermogenic activity of the BAT in increased sleep after sleep loss.
A number of neuroendocrine signals integrate to trigger these signals via BAT. Neuropeptides such as leptin, tumor necrosis factor, and interleukins 1 and 6 are present in adipose tissue and potentially carry sleep-promoting signals to the brain.Citation95–Citation97 However, the precise mechanism or a direct impact on the level of thermogenic effect in BAT is poorly understood. There is a close interaction between hypothalamic thermosensitivity and somnogenic mechanisms.Citation98 The increase in core body temperature as a result of heat production from BAT and the brain can affect these hypothalamic systems, in turn altering sleep. For example, temperature-sensing transient receptor potential vanilloid 4 channels are also found in these adipose tissues and are highly sensitive to subtle changes in temperature.Citation99,Citation100 Additionally, extensive sensory afferent innervation links interscapular BAT extending to the hypothalamus including PVN, and to other areas such as periaqueductal gray, parabrachial nuclei, and raphe nuclei.Citation101,Citation102 There is evidence to suggest that these innervations are temperature sensitive and prevent excessive heating of the tissue by a negative feedback loop.Citation103 Recent selective interscapular BAT deafferentation experiments in mice provide some evidence of significant sensory denervation in BAT; animals with disrupted innervation also show impaired recovery sleep response suggesting a neuronal regulation of sleep via BAT.Citation92 The increase in sleep typically seen in ambient temperatures (35°C) in wild-type mice is significantly diminished in the UCP1 KO mice in warmer environment, independent of differences in food intake and energy expenditure.Citation92 Since increased lipolysis is a sleep-promoting signal, the impaired lipolytic response in UCP1-deficient mice could explain their sleep deficiency in the warm environment. However, there is a significant time lag between the sleep-promoting and temperature effects of BAT activation, suggesting that an enhancement of sleep is not due to elevated body temperature.Citation104 Together, these findings indicate the role of metabolic activity of BAT, which in turn works in cohesion with promoting optimal sleep.
Most research on establishing the link between obesity and sleep has focused on OSA. Significant weight loss and reduction in BAT has been reported in a rat model of sleep apnea,Citation105 while a reduction in UCP1 expression in BAT was seen in a mouse model of OSA.Citation106 Reduced function of the orexin system resulting in the disordered sleep patterns of narcolepsy is also characterized by disruptions in thermoregulation and in energy homeostasis, along with obesity propensity.Citation107–Citation109 In rats, neuronal activation of orexin projections from the perifornical lateral hypothalamus increases BAT thermogenesis, indicating that orexin could significantly mediate the overall tone of BAT thermogenesis, thereby regulating body temperature during sleep and wakefulness.Citation110,Citation111 Impaired BAT function is a possible common link between inadequate sleep and metabolic disorders. Overall, metabolic status is tightly regulated by a complex signaling mechanism, which also adjusts the sleep-wake activity in order to maintain constant homeostasis. Feeding signals and others related to metabolism, activity, and thermoregulation interact with sleep circuits. Signals arising from BAT are part of this signaling mechanism and are likely to alter sleep and wakefulness.
Integration of metabolic signals with sleep regulation: neurobiology and neurochemistry
The homeostatic regulatory system works in close association with the peripheral metabolic signals to modulate energy balance. The central orexinergic and anorexinergic molecules such as NPY, orexin, histamine, cholecystokinin, and CRH influence the sympathetic activity to intercapsular BAT.Citation112–Citation118 Similarly, the circulating hormones that act as signals of energy surplus or surfeit activate hypothalamic neuronal populations that regulate sleep homeostasis.Citation113 The central melanocortin system is important in sensing and interacting with peripheral, neural, and endocrine cues such as insulin, leptin, and ghrelin, which then influence downstream mediators of energy balance.Citation76,Citation113
Along with their established role in altering appetite and energy utilization, these neuronal and endocrine signals also interact with sleep-wake behavior. Further, their cyclical high/low profiles are often well synchronized with the sleep-wake timing within a 24-hour period. Several recent studies have revealed that the bodily energy balance maintained by such synchronized systems is disturbed by changing the sleep-wake durations and timing within the 24-hour period. The major impact of shorter sleep durations on the feeding and energy imbalance leads to higher incidences of insulin resistance, glucose intolerance, and result in metabolic syndrome.Citation114,Citation115 The quality of sleep is also an important factor in maintaining energy balance. Sufficient slow wave sleep is needed to trigger a number of peripheral and central effects such as release of growth hormone and prolactin, and inhibiting corticotropic and thyrotropic activity which in turn regulate blood pressure, heart rate, and sympathetic nerve activity.Citation115,Citation119
Deficiencies in sleep are associated with obesity, yet little is known about how chronic sleep restriction influences energy expenditure. There is substantial literature to suggest that there are changes in satiety and hunger hormones during sleep loss; however, the mechanisms by which sleep loss contributes to weight gain are likely to be more complex.Citation120,Citation121 In a report from human subjects in which the effect of 5 days of reduced sleep was investigated, significant weight gain was observed in spite of changes in the levels of ghrelin, leptin, and peptide YY that signal excess energy stores suggestive of decreased sensitivity to the satiety hormones during sleep loss.Citation8
An elevated food demand is likely to be a physiological adaptation to provide the resources to sustain prolonged wakefulness during sleep loss. However, in the current obesogenic environment, weight gain is plausible because energy intake is in excess of the required energy demands during sleep loss. This could be because sleep loss can alter brain mechanisms involved in non-homeostatic food intake (eg, mood, comfort, reward, and reduced eating restraint).Citation77,Citation114,Citation122 A recent study in humans using functional magnetic resonance imaging and food-desire tasks demonstrated that the brain regions affected by sleep loss also affect appetitive food desire and enhance the response to hedonic food.Citation77,Citation122 They confirm that lack of sleep diminishes activity in appetite evaluation centers in the brain and further triggers an increase in craving for a high-calorie diet.Citation77 Sleep loss also consistently increases food intake in humans, mainly at night. Sleep and circadian systems are highly integrated; lack of sleep also leads to a delay in circadian timing and thus a change in the circadian timing of meals, especially breakfast.Citation123,Citation124 A recent Drosophila study involving time-restricted feeding showed a direct effect on caloric intake, sleep quality, and cardiovascular profile to further indicate this interesting interplay between circadian cycle, feeding-fasting, and the overall metabolic health.Citation125 These reports strongly implicate the circadian periodicity of metabolic pathways in sleep loss-related weight gain.
A study comparing sleep deprivation (4 hours/2 nights) vs sleep extension (10 hours/2 nights) reported a panel of endocrine changes that indicate metabolic imbalance. The individuals with sleep debt were found to have lower morning insulin levels, higher glucose, elevated ghrelin-to-leptin ratio, and an increase in appetite for high carbohydrate food.Citation65 Other reports including a large population study show a direct link between circulating leptin and ghrelin levels with sleep restriction.Citation64,Citation126 In general, curtailed sleep is accompanied by elevated cortisol during the day.Citation115 Chronic lack of sleep is also associated with an aberrant carbohydrate tolerance and impaired glucose tolerance, thus increasing the risk for type 2 diabetes. Central and peripheral alterations due to excessive glucocorticoids may contribute to aging and related deficits in memory.Citation127,Citation128 shows the key mediators of these pathways and their integration at the central nervous system having a direct two-way communication with sleep and metabolic parameters. Thus, sleep duration has a multitude of effects on both endocrine and metabolic functions, and the neuroendocrine regulation of appetite and food consumption is altered by sleep restriction and may favor the development of obesity.
Therapeutic perspectives: potential pharmacological targets for the treatment of sleep disorders, especially those linked to obesity and metabolic disorders
It is widely recognized that short sleep duration is associated with elevated BMI in many epidemiological studies. As discussed earlier, several pathways link sleep deprivation to energy imbalance, including increased food intake, decreased energy expenditure, and changes in levels of appetite-regulating hormones including leptin and ghrelin. One cannot ignore the contribution of multimedia (eg, television viewing, computer, and Internet), which aggravates sedentary behavior and increases caloric intake. Electronic devices including e-Readers have been recently shown to directly affect circadian cycle and alter sleep and alertness, the following day.Citation129 In addition, shift-work, long working hours, and increased time commuting to and from work can contribute to obesity and related metabolic disorders, because of their strong link to shorter sleep times. Consequently, the first and foremost consideration in treating the metabolic disorders is the evaluation of the life style and its potential impact on energy imbalance.
The hormone melatonin, normally secreted from the pineal gland at night, serves as the signal of darkness in the organism and plays a pivotal role in the physiological regulation of circadian rhythms, including sleep. There is a growing body of evidence suggesting a link between disturbances in melatonin production and impaired insulin, glucose, lipid metabolism, and antioxidant capacity.Citation130,Citation131 Several studies support that melatonin can prevent hyperadiposity in animal models of obesity. In obese rats, melatonin treatment ameliorated abdominal obesity, hyperinsulinemia, hypercholesterolemia, hyperglycemia, hyperbetalipoproteinemia, and glycosuria.Citation132 Melatonin is one of the several system targets for sleep disorders. Ramelteon is a selective melatonin receptor type 1 (MT1) and melatonin receptor type 2 (MT2) receptors agonist and is commonly used for insomnia.Citation133 However, no published studies have systematically evaluated its effects on metabolic pathways. Sleep aids directed toward GABAA receptors, such as zolpidem, eszopiclone, and zaleplon, are commonly used despite a paucity of published reports on the drug’s effects on metabolic processes. However, the orexin peptides and their receptors, by virtue of their involvement in multiple physiological processes such as the regulation of sleep/wakefulness state, and energy homeostasis are proving to be good targets for drug development. The use of small-molecule orexin receptor antagonists as novel therapies for the treatment of insomnia is increasingly being discussed.Citation134 Recently, a dual receptor antagonist, suvorexant was approved by the US Food and Drug Administration for treating insomnia and is being marketed by Merck Pharmaceuticals as Belsomra.Citation135,Citation136 Increased levels of signaling via the orexin peptide/receptor system enhance sleep and may protect against obesity. Thus, orexin agonists and antagonists are thought to be promising avenues toward the treatment of sleep disorders as well as for targeting eating disorders.
In summary, epidemiological studies and empirical evidence from well-crafted human and animal experiments have begun to converge upon the close association between sleep-wake and feeding-fasting cycles that maintain energy balance. The hypothalamus is increasingly being recognized for its unifying role in maintaining energy balance. Research continues to explore and understand neuropeptide-specific populations within hypothalamus, their connectivity and their selective contributions that will allow identification of precise therapeutic targets for combined treatment of sleep and metabolic imbalances.
Acknowledgments
This work was supported by the Department of Veterans Affairs Medical Research Service Merit Award, NINDS 079866, and NIMH 39683. We would like to thank Dr Colleen M Novak for critically reading this review.
Disclosure
The authors report no conflicts of interest in this work.
References
- ReutrakulSVan CauterEInteractions between sleep, circadian function, and glucose metabolism: implications for risk and severity of diabetesAnn NY Acad Sci2014131115117324628249
- KimTWJeongJHHongSCThe impact of sleep and circadian disturbance on hormones and metabolismInt J Endocrinol2015201559172925861266
- CzeislerCAKlermanEBCircadian and sleep-dependent regulation of hormone release in humansRecent Prog Hormone Res19995497130 discussion 130–132
- DijkDJLockleySWIntegration of human sleep-wake regulation and circadian rhythmicityJ Appl Physiol200292285286211796701
- BrownREBasheerRMcKennaJTStreckerREMcCarleyRWControl of sleep and wakefulnessPhysiol Rev20129231087118722811426
- LaposkyADBassJKohsakaATurekFWSleep and circadian rhythms: key components in the regulation of energy metabolismFEBS Lett2008582114215117707819
- WrightKPJrDrakeALFreyDJInfluence of sleep deprivation and circadian misalignment on cortisol, inflammatory markers, and cytokine balanceBrain BehavImmun2015472434
- MarkwaldRRMelansonELSmithMRImpact of insufficient sleep on total daily energy expenditure, food intake, and weight gainProc Natl Acad Sci U S A2013110145695570023479616
- McHillAWMelansonELHigginsJImpact of circadian misalignment on energy metabolism during simulated nightshift workProc Natl Acad Sci U S A201411148173021730725404342
- NguyenJWrightKPJrInfluence of weeks of circadian misalignment on leptin levelsNat Sci Sleep2010291823616693
- LockeAEKahaliBBerndtSIGenetic studies of body mass index yield new insights for obesity biologyNature2015518753819720625673413
- ShunginDWinklerTWCroteau-ChonkaDCNew genetic loci link adipose and insulin biology to body fat distributionNature2015518753818719625673412
- CampbellSSToblerIAnimal sleep: a review of sleep duration across phylogenyNeurosci Biobehav Rev1984832693006504414
- KingDPZhaoYSangoramAMPositional cloning of the mouse circadian clock geneCell19978946416539160755
- ReppertSMWeaverDRCoordination of circadian timing in mammalsNature2002418690193594112198538
- NaylorEBergmannBMKrauskiKThe circadian clock mutation alters sleep homeostasis in the mouseJ Neurosci200020218138814311050136
- TurekFWJoshuCKohsakaAObesity and metabolic syndrome in circadian Clock mutant miceScience (New York, NY)2005308572410431045
- DworakMMcCarleyRWKimTKalinchukAVBasheerRSleep and brain energy levels: ATP changes during sleepJ Neurosci201030269007901620592221
- SaperCBStaying awake for dinner: hypothalamic integration of sleep, feeding, and circadian rhythmsProg Brain Res200615324325216876579
- ChouTCScammellTEGooleyJJGausSESaperCBLuJCritical role of dorsomedial hypothalamic nucleus in a wide range of behavioral circadian rhythmsJ Neurosci20032333106911070214627654
- AbrahamsonEEMooreRYLesions of suprachiasmatic nucleus efferents selectively affect rest-activity rhythmMol Cell Endocrinol20062521–2465616793198
- LuJZhangYHChouTCContrasting effects of ibotenate lesions of the paraventricular nucleus and subparaventricular zone on sleep-wake cycle and temperature regulationJ Neurosci200121134864487411425913
- HouseknechtKLBaileCAMatteriRLSpurlockMEThe biology of leptin: a reviewJ Anim Sci1998765140514209621947
- KojimaMKangawaKGhrelin: structure and functionPhysiol Rev200585249552215788704
- MillingtonGWTungYCHewsonAKO’RahillySDicksonSLDifferential effects of alpha-, beta- and gamma(2)-melanocyte-stimulating hormones on hypothalamic neuronal activation and feeding in the fasted ratNeuroscience2001108343744511738258
- SatohNOgawaYKatsuuraGPathophysiological significance of the obese gene product, leptin, in ventromedial hypothalamus (VMH)-lesioned rats: evidence for loss of its satiety effect in VMH-lesioned ratsEndocrinology199713839479549048594
- GrundmannSJPankeyEACookMMWoodALRollinsBLKingBMCombination unilateral amygdaloid and ventromedial hypothalamic lesions: evidence for a feeding pathwayAm J Physiol Regul Integr Comp Physiol20052883R702R70715699361
- XuBGouldingEHZangKBrain-derived neurotrophic factor regulates energy balance downstream of melanocortin-4 receptorNat Neurosci20036773674212796784
- WangCBombergEBillingtonCJLevineASKotzCMBrain-derived neurotrophic factor (BDNF) in the hypothalamic ventromedial nucleus increases energy expenditureBrain Res20101336667720398635
- FaragunaUVyazovskiyVVNelsonABTononiGCirelliCA causal role for brain-derived neurotrophic factor in the homeostatic regulation of sleepJ Neurosci200828154088409518400908
- RiosMNew insights into the mechanisms underlying the effects of BDNF on eating behaviorNeuropsychopharmacology201136136836921116262
- SakuraiTAmemiyaAIshiiMOrexins and orexin receptors: a family of hypothalamic neuropeptides and G protein-coupled receptors that regulate feeding behaviorCell19989245735859491897
- de LeceaLKilduffTSPeyronCThe hypocretins: hypothalamus-specific peptides with neuroexcitatory activityProc Natl Acad Sci U S A19989513223279419374
- BurdakovDKarnaniMMGonzalezALateral hypothalamus as a sensor-regulator in respiratory and metabolic controlPhysiol Behav201312111712423562864
- de LeceaLHuertaRHypocretin (orexin) regulation of sleep-to-wake transitionsFront Pharmacol201451624575043
- KarnaniMBurdakovDMultiple hypothalamic circuits sense and regulate glucose levelsAm J Physiol Regul Integr Comp Physiol20113001R47R5521048078
- MahlerSVSmithRJMoormanDESartorGCAston-JonesGMultiple roles for orexin/hypocretin in addictionProg Brain Res20121987912122813971
- NixonJPMavanjiVButterickTABillingtonCJKotzCMTeskeJASleep disorders, obesity, and aging: the role of orexinAgeing Res Rev201520637325462194
- PeyronCFaracoJRogersWA mutation in a case of early onset narcolepsy and a generalized absence of hypocretin peptides in human narcoleptic brainsNat Med20006999199710973318
- AhmedWATsutsumiMNakataSA functional variation in the hypocretin neuropeptide precursor gene may be associated with obstructive sleep apnea syndrome in JapanLaryngoscope2012122492592922302634
- BaumannCRTraumatic brain injury and disturbed sleep and wakefulnessNeuromolecular Med201214320521222441999
- StrawnJRPyne-GeithmanGJEkhatorNNLow cerebrospinal fluid and plasma orexin-A (hypocretin-1) concentrations in combat-related posttraumatic stress disorderPsychoneuroendocrinology20103571001100720116928
- HaraJBeuckmannCTNambuTGenetic ablation of orexin neurons in mice results in narcolepsy, hypophagia, and obesityNeuron200130234535411394998
- FadelJRJolivaltCGReaganLPFood for thought: the role of appetitive peptides in age-related cognitive declineAgeing Res Rev201312376477623416469
- FronczekRBaumannCRLammersGJBassettiCLOvereemSHypocretin/orexin disturbances in neurological disordersSleep Med Rev200913192218819824
- SiegelJMHypocretin (orexin): role in normal behavior and neuropathologyAnnu Rev Psychol20045512514814744212
- ChemelliRMWillieJTSintonCMNarcolepsy in orexin knockout mice: molecular genetics of sleep regulationCell199998443745110481909
- LinLFaracoJLiRThe sleep disorder canine narcolepsy is caused by a mutation in the hypocretin (orexin) receptor 2 geneCell199998336537610458611
- MignotELammersGJRipleyBThe role of cerebrospinal fluid hypocretin measurement in the diagnosis of narcolepsy and other hypersomniasArch Neurol200259101553156212374492
- NishinoSRipleyBOvereemSLammersGJMignotEHypocretin (orexin) deficiency in human narcolepsyLancet20003559197394010615891
- ThannickalTCMooreRYNienhuisRReduced number of hypocretin neurons in human narcolepsyNeuron200027346947411055430
- CrockerAEspanaRAPapadopoulouMConcomitant loss of dynorphin, NARP, and orexin in narcolepsyNeurology20056581184118816247044
- GerashchenkoDBlanco-CenturionCGrecoMAShiromaniPJEffects of lateral hypothalamic lesion with the neurotoxin hypocretin-2-saporin on sleep in Long-Evans ratsNeuroscience2003116122323512535955
- AdamantidisARZhangFAravanisAMDeisserothKde LeceaLNeural substrates of awakening probed with optogenetic control of hypocretin neuronsNature2007450716842042417943086
- YokoboriEKojimaKAzumaMStimulatory effect of intracerebroventricular administration of orexin A on food intake in the zebrafish, Danio rerioPeptides20113271357136221616109
- HaynesACJacksonBOverendPEffects of single and chronic intracerebroventricular administration of the orexins on feeding in the ratPeptides19992091099110510499428
- BurdakovDGerasimenkoOVerkhratskyAPhysiological changes in glucose differentially modulate the excitability of hypothalamic melanin-concentrating hormone and orexin neurons in situJ Neurosci20052592429243315745970
- GonzalezJAJensenLTDoyleSEDeletion of TASK1 and TASK3 channels disrupts intrinsic excitability but does not abolish glucose or pH responses of orexin/hypocretin neuronsEur J Neurosci2009301576419508695
- SchoneCVennerAKnowlesDKarnaniMMBurdakovDDichotomous cellular properties of mouse orexin/hypocretin neuronsJ Physiol2011589Pt 112767277921486780
- ZhengHCorkernMMCrousillacSMPattersonLMPhiferCBBerthoudHRNeurochemical phenotype of hypothalamic neurons showing Fos expression 23 h after intracranial AgRPAm J Physiol Regul Integr Comp Physiol20022826R1773R178112010760
- EliasCFSaperCBMaratos-FlierEChemically defined projections linking the mediobasal hypothalamus and the lateral hypothalamic areaJ Comp Neurol199840244424599862320
- NovakCMLevineJACentral neural and endocrine mechanisms of non-exercise activity thermogenesis and their potential impact on obesityJ Neuroendocrinol2007191292394018001322
- ShintaniMOgawaYEbiharaKGhrelin, an endogenous growth hormone secretagogue, is a novel orexigenic peptide that antagonizes leptin action through the activation of hypothalamic neuropeptide Y/Y1 receptor pathwayDiabetes200150222723211272130
- TaheriSLinLAustinDYoungTMignotEShort sleep duration is associated with reduced leptin, elevated ghrelin, and increased body mass indexPLoS Med200413e6215602591
- SpiegelKTasaliEPenevPVan CauterEBrief communication: sleep curtailment in healthy young men is associated with decreased leptin levels, elevated ghrelin levels, and increased hunger and appetiteAnn Intern Med20041411184685015583226
- LittmanAJVitielloMVFoster-SchubertKSleep, ghrelin, leptin and changes in body weight during a 1-year moderate-intensity physical activity interventionInt J Obes2007313466475
- MullingtonJMChanJLVan DongenHPSleep loss reduces diurnal rhythm amplitude of leptin in healthy menJ Neuroendocrinol200315985185412899679
- SpiegelKLeproultRVan CauterEImpact of sleep debt on physiological rhythmsRev Neurol200315911 Suppl6S1116S2014646794
- HarschIAKonturekPCKoebnickCLeptin and ghrelin levels in patients with obstructive sleep apnoea: effect of CPAP treatmentEur Respir J200322225125712952256
- PanWKastinAJLeptin: a biomarker for sleep disorders?Sleep Med Rev201418328329024080454
- KokSWMeindersAEOvereemSReduction of plasma leptin levels and loss of its circadian rhythmicity in hypocretin (orexin)-deficient narcoleptic humansJ Clin Endocrinol Metab200287280580911836325
- YangJZhaoTJGoldsteinJLBrownMSInhibition of ghrelin O-acyltransferase (GOAT) by octanoylated pentapeptidesProc Natl Acad Sci U S A200810531107501075518669668
- LoosRJLindgrenCMLiSCommon variants near MC4R are associated with fat mass, weight and risk of obesityNat Genet200840676877518454148
- PillaiSNandalikeKKogelmanYMuzumdarRBalkSJArensRSevere obstructive sleep apnea in a child with melanocortin-4 receptor deficiencyJ Clin Sleep Med20141019910124426828
- ElmquistJKEliasCFSaperCBFrom lesions to leptin: hypothalamic control of food intake and body weightNeuron199922222123210069329
- CowleyMAPronchukNFanWDinulescuDMColmersWFConeRDIntegration of NPY, AGRP, and melanocortin signals in the hypothalamic paraventricular nucleus: evidence of a cellular basis for the adipostatNeuron199924115516310677034
- GreerSMGoldsteinANWalkerMPThe impact of sleep deprivation on food desire in the human brainNat Commun20134225923922121
- DingesDFChughDPhysiologic correlates of sleep deprivationKinneyJMTuckerHNPhysiology, stress and malnutrition: Functional correlates, nutritional interventionNew YorkLippincott-Raven1997127
- PanossianLAVeaseySCDaytime sleepiness in obesity: mechanisms beyond obstructive sleep apnea–a reviewSleep201235560561522547886
- WellsASReadNWUvnas-MobergKAlsterPInfluences of fat and carbohydrate on postprandial sleepiness, mood, and hormonesPhysiol Behav19976156796869145937
- VarelaJEHinojosaMWNguyenNTResolution of obstructive sleep apnea after laparoscopic gastric bypassObes Surg200717101279128218000730
- CannonBNedergaardJBrown adipose tissue: function and physiological significancePhysiol Rev200484127735914715917
- NedergaardJBengtssonTCannonBUnexpected evidence for active brown adipose tissue in adult humansAm J Physiol Endocrinol Metab20072932E444E45217473055
- BlessingWMohammedMOotsukaYHeating and eating: brown adipose tissue thermogenesis precedes food ingestion as part of the ultradian basic rest-activity cycle in ratsPhysiol Behav2012105496697422115948
- HamannAFlierJSLowellBBDecreased brown fat markedly enhances susceptibility to diet-induced obesity, diabetes, and hyperlipidemiaEndocrinology1996137121298536614
- SymondsMEPopeMSharkeyDBudgeHAdipose tissue and fetal programmingDiabetologia20125561597160622402988
- VijgenGHBouvyNDTeuleGJIncrease in brown adipose tissue activity after weight loss in morbidly obese subjectsJ Clin Endocrinol Metab2012977E1229E123322535970
- VijgenGHBouvyNDTeuleGJBransBSchrauwenPvan Marken LichtenbeltWDBrown adipose tissue in morbidly obese subjectsPLoS One201162e1724721390318
- OuelletVLabbeSMBlondinDPBrown adipose tissue oxidative metabolism contributes to energy expenditure during acute cold exposure in humansJ Clin Investig2012122254555222269323
- SymondsMEHendersonKElvidgeLThermal imaging to assess age-related changes of skin temperature within the supraclavicular region co-locating with brown adipose tissue in healthy childrenJ Pediatrics20121615892898
- van Marken LichtenbeltWDSchrauwenPImplications of nonshivering thermogenesis for energy balance regulation in humansAm J Physiol Regul Integr Comp Physiol20113012R285R29621490370
- SzentirmaiEKapasLIntact brown adipose tissue thermogenesis is required for restorative sleep responses after sleep lossEur J Neurosci201439698499824372950
- DewasmesGLoosNDelanaudSDewasmesDGeloenAActivation of brown adipose tissue thermogenesis increases slow wave sleep in ratNeurosci Lett2003339320721012633889
- KobanMSwinsonKLChronic REM-sleep deprivation of rats elevates metabolic rate and increases UCP1 gene expression in brown adipose tissueAm J Physiol Endocrinol Metab20052891E68E7415727948
- KapasLHongLCadyABSomnogenic, pyrogenic, and anorectic activities of tumor necrosis factor-alpha and TNF-alpha fragmentsAm J Physiol19922633 Pt 2R708R7151357984
- SintonCMFitchTEGershenfeldHKThe effects of leptin on REM sleep and slow wave delta in rats are reversed by food deprivationJ Sleep Res19998319720310476006
- HoganDMorrowJDSmithEMOppMRInterleukin-6 alters sleep of ratsJ Neuroimmunol20031371–2596612667648
- McGintyDSzymusiakRHypothalamic regulation of sleep and arousalFront Biosci20038s1074s108312957869
- YeLKleinerSWuJTRPV4 is a regulator of adipose oxidative metabolism, inflammation, and energy homeostasisCell201215119611023021218
- WatanabeHVriensJSuhSHBenhamCDDroogmansGNiliusBHeat-evoked activation of TRPV4 channels in a HEK293 cell expression system and in native mouse aorta endothelial cellsJ Biol Chem200227749470444705112354759
- BartnessTJVaughanCHSongCKSympathetic and sensory innervation of brown adipose tissueInt J Obes201034Suppl 1S36S42
- VaughanCHBartnessTJAnterograde transneuronal viral tract tracing reveals central sensory circuits from brown fat and sensory denervation alters its thermogenic responsesAm J Physiol Regul Integr Comp Physiol20123029R1049R105822378771
- OsakaTKobayashiANambaYTemperature- and capsaicin-sensitive nerve fibers in brown adipose tissue attenuate thermogenesis in the ratPflugers Arch1998437136429817783
- SzentirmaiLKÉBrown adipose tissue at the intersection of sleep and temperature regulationTemperature2014111617
- MartinezDVasconcellosLFde OliveiraPGKonradSPWeight loss and brown adipose tissue reduction in rat model of sleep apneaLipids Health Dis200872618671859
- FioriCZMartinezDBaronioDDownregulation of uncoupling protein-1 mRNA expression and hypoadiponectinemia in a mouse model of sleep apneaSleep Breath201418354154824337908
- HaraJYanagisawaMSakuraiTDifference in obesity phenotype between orexin-knockout mice and orexin neuron-deficient mice with same genetic background and environmental conditionsNeurosci Lett2005380323924215862893
- KokSWOvereemSVisscherTLHypocretin deficiency in narcoleptic humans is associated with abdominal obesityObes Res20031191147115412972686
- MochizukiTKlermanEBSakuraiTScammellTEElevated body temperature during sleep in orexin knockout miceAm J Physiol Regul Integr Comp Physiol20062913R533R54016556901
- FeldmannHMGolozoubovaVCannonBNedergaardJUCP1 ablation induces obesity and abolishes diet-induced thermogenesis in mice exempt from thermal stress by living at thermoneutralityCell Metab20099220320919187776
- TuponeDMaddenCJCanoGMorrisonSFAn orexinergic projection from perifornical hypothalamus to raphe pallidus increases rat brown adipose tissue thermogenesisJ Neurosci20113144159441595522049437
- YoshimatsuHEgawaMBrayGAEffects of cholecystokinin on sympathetic activity to interscapular brown adipose tissueBrain Res199259722983031473000
- EliasCFAschkenasiCLeeCLeptin differentially regulates NPY and POMC neurons projecting to the lateral hypothalamic areaNeuron199923477578610482243
- LeproultRVan CauterERole of sleep and sleep loss in hormonal release and metabolismEndocr Dev201017112119955752
- Van CauterEHolmbackUKnutsonKImpact of sleep and sleep loss on neuroendocrine and metabolic functionHormone Res200767Suppl 12917308390
- YasudaTMasakiTKakumaTDual regulatory effects of orexins on sympathetic nerve activity innervating brown adipose tissue in ratsEndocrinology200514662744274815746258
- EgawaMYoshimatsuHBrayGAPreoptic area injection of corticotropin-releasing hormone stimulates sympathetic activityAm J Physiol19902594 Pt 2R799R8062221147
- EgawaMYoshimatsuHBrayGANeuropeptide Y suppresses sympathetic activity to interscapular brown adipose tissue in ratsAm J Physiol19912602 Pt 2R328R3341996720
- Van CauterEPlatLCopinschiGInterrelations between sleep and the somatotropic axisSleep19982165535669779515
- BadmanMKFlierJSThe gut and energy balance: visceral allies in the obesity warsScience200530757171909191415790843
- BatterhamRLCohenMAEllisSMInhibition of food intake in obese subjects by peptide YY3-36New Engl J Med20033491094194812954742
- BenedictCBrooksSJO’DalyOGAcute sleep deprivation enhances the brain’s response to hedonic food stimuli: an fMRI studyJ Clin Endocrinol Metab2012973E443E44722259064
- NaismithSLHickieIBTerpeningZCircadian misalignment and sleep disruption in mild cognitive impairmentJ Alzheimer’s Dis201438485786624100124
- WrightKPJrHullJTHughesRJRondaJMCzeislerCASleep and wakefulness out of phase with internal biological time impairs learning in humansJ Cogn Neurosci200618450852116768357
- GillSLeHDMelkaniGCPandaSTime-restricted feeding attenuates age-related cardiac decline in DrosophilaScience201534762271265126925766238
- SchmidSMHallschmidMJauch-CharaKBornJSchultesBA single night of sleep deprivation increases ghrelin levels and feelings of hunger in normal-weight healthy menJ Sleep Res200817333133418564298
- MirescuCPetersJDNoimanLGouldESleep deprivation inhibits adult neurogenesis in the hippocampus by elevating glucocorticoidsProc Natl Acad Sci U S A200610350191701917517135354
- BackhausJJunghannsKBornJHohausKFaaschFHohagenFImpaired declarative memory consolidation during sleep in patients with primary insomnia: Influence of sleep architecture and nocturnal cortisol releaseBiolPsychiatry2006601213241330
- ChangAMAeschbachDDuffyJFCzeislerCAEvening use of light-emitting eReaders negatively affects sleep, circadian timing, and next-morning alertnessProc Natl Acad Sci U S A201511241232123725535358
- RobevaRKirilovGTomovaAKumanovPMelatonin-insulin interactions in patients with metabolic syndromeJ Pineal Res2008441525618078448
- NishidaSMetabolic effects of melatonin on oxidative stress and diabetes mellitusEndocrine200527213113616217126
- VinogradovaIAnisimovVMelatonin prevents the development of the metabolic syndrome in male rats exposed to different light/dark regimensBiogerontology201314440140923784085
- Pandi-PerumalSRSrinivasanVSpenceDWRamelteon: a review of its therapeutic potential in sleep disordersAdv Ther200926661362619568703
- XuTRYangYWardRGaoLLiuYOrexin receptors: multi-functional therapeutic targets for sleeping disorders, eating disorders, drug addiction, cancers and other physiological disordersCell Signal201325122413242323917208
- WinrowCJRengerJJDiscovery and development of orexin receptor antagonists as therapeutics for insomniaBr J Pharmacol2014171228329323731216
- RoeckerAJCoxCDColemanPJOrexin receptor antagonists: new therapeutic agents for the treatment of insomniaJ Med Chem Epub2015915
- CastanedaTRJurgensHWiedmerPObesity and the neuroendocrine control of energy homeostasis: the role of spontaneous locomotor activityJ Nutr200513551314131915867332
- SzentirmaiEKruegerJMObestatin alters sleep in ratsNeurosci Lett20064041–222222616806691
- ZhangJVRenPGAvsian-KretchmerOObestatin, a peptide encoded by the ghrelin gene, opposes ghrelin’s effects on food intakeScience2005310575099699916284174
- ShemyakinAKapasLL-364,718, a cholecystokinin-A receptor antagonist, suppresses feeding-induced sleep in ratsAm J Physiol Regul Integr Comp Physiol20012805R1420R142611294763
- LittleTJHorowitzMFeinle-BissetCRole of cholecystokinin in appetite control and body weight regulationObes Rev20056429730616246215
- BenedictCBarclayJLOttVOsterHHallschmidMAcute sleep deprivation delays the glucagon-like peptide 1 peak response to breakfast in healthy menNutr Diabetes20133e7823797385
- MadsbadSThe role of glucagon-like peptide-1 impairment in obesity and potential therapeutic implicationsDiabObes Metab2014161921
- KarraEChandaranaKBatterhamRLThe role of peptide YY in appetite regulation and obesityJ Physiol2009587Pt 1192519064614
- AhnaouADrinkenburgWHNeuromedin U(2) receptor signaling mediates alteration of sleep-wake architecture in ratsNeuropeptides201145216517421296417
- HeldKAntonijevicIMurckHKuenzelHSteigerANeuropeptide Y (NPY) shortens sleep latency but does not suppress ACTH and cortisol in depressed patients and normal controlsPsychoneuroendocrinology200631110010716112814
- AntonijevicIAMurckHBohlhalterSFrieboesRMHolsboerFSteigerANeuropeptide Y promotes sleep and inhibits ACTH and cortisol release in young menNeuropharmacology20003981474148110818263
- DyzmaMBoudjeltiaKZFarautBKerkhofsMNeuropeptide Y and sleepSleep Med Rev201014316116520122859
- AdamantidisAde LeceaLSleep and metabolism: shared circuits, new connectionsTrends Endocrinol Metab2008191036237018938086
- RomanovaIVMikhrinaALParticipation of Agouti related peptide in machanisms of wakefulness-sleep cycle regulationFiziol Chel20133962430
- ChastretteNCespuglioRInfluence of proopiomelanocortin-derived peptides on the sleep-waking cycle of the ratNeurosci Lett19856233653703005923
- ChastretteNCespuglioRJouvetMProopiomelanocortin (POMC)-derived peptides and sleep in the rat. Part 1 – Hypnogenic properties of ACTH derivativesNeuropeptides199015261741981927
- MiedaMHasegawaEKisanukiYYSintonCMYanagisawaMSakuraiTDifferential roles of orexin receptor-1 and -2 in the regulation of non-REM and REM sleepJ Neurosci201131176518652621525292
- WillieJTChemelliRMSintonCMYanagisawaMTo eat or to sleep? Orexin in the regulation of feeding and wakefulnessAnnu Rev Neurosci20012442945811283317
- ZhangXvan den PolANThyrotropin-releasing hormone (TRH) inhibits melanin-concentrating hormone neurons: implications for TRH-mediated anorexic and arousal actionsJ Neurosci20123293032304322378876
- UchidaMSuzukiMShimizuKEffects of urocortin, corticotropin-releasing factor (CRF) receptor agonist, and astressin, CRF receptor antagonist, on the sleep-wake pattern: analysis by radiotelemetry in conscious ratsBiol Pharm Bull200730101895189717917258
- StengelATacheYCRF and urocortin peptides as modulators of energy balance and feeding behavior during stressFront Neurosci201485224672423
- KonadhodeRRPelluruDShiromaniPJNeurons containing orexin or melanin concentrating hormone reciprocally regulate wake and sleepFront Syst Neurosci2014824425620917
- SteigerASleep and the hypothalamo-pituitary-adrenocortical systemSleep Med Rev20026212513812531148
- RichardDHuangQTimofeevaEThe corticotropin-releasing hormone system in the regulation of energy balance in obesityInt J Obes Relat Metab Disord200024Suppl 2S36S3910997606
- MongrainVHernandezSAPradervandSSeparating the contribution of glucocorticoids and wakefulness to the molecular and electrophysiological correlates of sleep homeostasisSleep20103391147115720857860
- de KloetADKrauseEGSolomonMBAdipocyte glucocorticoid receptors mediate fat-to-brain signalingPsychoneuroendocrinology20155611011925808702