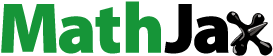
Abstract
Introduction
The peripheral refractive error of the human eye has been hypothesized to be a major stimulus for the development of its central refractive error.
Aim
The purpose of this study was to investigate the changes in the peripheral refractive error across horizontal, vertical and two diagonal meridians in emmetropic and low, moderate and high myopic adults.
Subjects and methods
Thirty-four adult subjects were recruited and aberration was measured using a modified commercial aberrometer. We then computed the refractive error in power vector notation from second-order Zernike terms. Statistical analysis was performed to evaluate the statistical differences in refractive error profiles between the subject groups and across all measured visual field meridians.
Results
Small amounts of relative myopic shift were observed in emmetropic and low myopic subjects. However, moderate and high myopic subjects exhibited a relative hyperopic shift in all four meridians. Astigmatism J0 and J45 had quadratic or linear changes dependent on the visual field meridians. Peripheral Sphero-Cylindrical Retinal Image Blur increased in emmetropic eyes in most of the measured visual fields.
Conclusion
The findings indicate an overall emmetropic or slightly relative myopic periphery (spherical or oblate retinal shape) formed in emmetropes and low myopes, while moderate and high myopes form relative hyperopic periphery (prolate, or less oblate, retinal shape). In general, human emmetropic eyes demonstrate higher amount of peripheral retinal image blur.
Introduction
When simply viewed as an optical instrument, the human eye is imperfect, with defocus, astigmatism and higher order aberrations being common. These optical deficiencies exist for both central and peripheral vision. Central vision has been studied for many years, and clinicians devote themselves to correct them. The importance and potential correction of peripheral refractive errors is not well understood. They influence the peripheral retinal image quality (IQ) and, therefore, also influence peripheral vision. Peripheral vision is important for motion and pattern detection.Citation1,Citation2 Peripheral IQ is highly relevant for fundus imaging.Citation3 Interest in studying the off-axis optical performance and retinal IQ of the human eye has increased dramatically in recent years because some studies suggest that ocular off-axis aberrations influence the development of a central refractive error.Citation4–Citation8 Experiments conducted on different animal species, including primates, demonstrated that eye growth is controlled by local retinal mechanisms.Citation9–Citation11 Some studies suggest that peripheral aberrations, including defocus and astigmatism, may influence the foveal refractive development in human eyes as well.Citation12–Citation15 During regular human visual development, eye growth tends to minimize the image blur on the most part of the retina, known as the “grow to clarity” model.Citation16
Most studies in the field focused on evaluating peripheral refraction and optical aberrations along the horizontal visual field;Citation3,Citation17–Citation20 some also measured refractive changes along the vertical meridian.Citation21,Citation22 These studies demonstrated that refractive error (curvature of field and astigmatism) and IQ differ significantly with retinal eccentricity. Most studies agree that, in the horizontal visual field, hyperopic and emmetropic (Em.) eyes have peripheral refractive errors which are myopic relative to the fovea. In contrast, myopic eyes have less myopia in the peripheral visual field than in the fovea. However, there is controversy regarding the generality of this finding for the vertical meridian.Citation21,Citation22
Based on the theory that ocular growth is controlled by local retinal mechanisms,Citation9 more comprehensive evaluations of peripheral refraction and IQ changes in the full visual field, rather than just horizontal or vertical meridian, are necessary. The purpose of this study was to investigate the changes in peripheral refractive error across horizontal, vertical and two diagonal meridians across the visual field in Em. and low, moderate and high myopic (LM, MM and HM, respectively) adults.
Subjects and methods
This study followed the tenets of the Declaration of Helsinki and was approved by the Western University of Health Sciences Institutional Review Board before study commencement. All subjects gave their written consent to participate in this study after being informed about the nature and possible consequences of study participation.
A total of 34 adult subjects participated in this study. The participants were 8 emmetropes (mean spherical equivalent refraction between −0.50 and +0.50 D, age range 19–33 years) and 26 myopes (between −0.70 and −8.60 D, age range 19–31 years). We sought 80% power to detect a 0.25 D difference in spherical equivalent (SE) between the peripheral and central visual fields with a significance level of 0.05 (two tailed). Assuming the same mean SE values and SD as reported from the literature,Citation23,Citation24 a sample size of 31 (7 emmetropes, and 9 low, 8 moderate and 7 high myopes) was calculated. Astigmatism in all subjects was <1.50 D. We computed the sphero-cylindrical refractive error in power vector notation (M, J0, J45) from second-order Zernike terms. Myopic patients were subgrouped into low myopes (from −0.75 to −3.00 D), moderate myopes (from −3.25 to −6.00 D) and high myopes (>−6.00 D). To preserve a natural viewing condition, no cycloplegic or mydriatic agents were applied. The participants reported no ocular pathology or ocular surgery history. To avoid confounding factors, we measured only their right eyes.
We obtained refractive measurements with a commercial Shack–Hartmann wavefront aberrometer (Complete Ophthalmic Analysis System [COAS]; AMO Wavefront Sciences, Inc., Albuquerque, New Mexico) on the participants’ right eyes in a dark room to ensure the largest natural pupil sizes. We chose this instrument, rather than a conventional autorefractor, because it uses a Shack–Hartmann wavefront sensor to obtain a detailed wavefront analysis of the entire entrance pupil, rather than the central limited pupil size analyzed in the autorefractor. This allowed us to obtain data even when the pupil appeared elliptical when viewed obliquely during the measurement of peripheral refractive errors. According to the manufacturer’s specifications, the COAS can measure spherical refractive errors within the range of −15.00 to +7.00 D with an accuracy of ±0.10 D and a repeatability of ±0.05 D. We aligned the participants with the COAS aberrometer using its internal cameras. The cameras are coaxial with the wavefront sensor and are focused using the virtual Purkinje images of light-emitting diodes on the face of the instrument. The COAS aberrometer is a robust and reliable instrument for measuring both lower and higher order aberrations in the central visual fieldCitation25–Citation27 and for measuring peripheral ocular aberrations for elliptical entrance pupils that occur in the off-axis viewing condition.Citation28 We modified the COAS wavefront aberrometer to take measurements in 10° steps out to ±30° of eccentricity along four visual field meridians, that is, horizontal, vertical and two diagonal meridians ().
Figure 1 Experimental setup.
Abbreviations: BS, beam splitter; LS, line of sight; MA, meas urement axis; S, screen; VTs, visual targets.
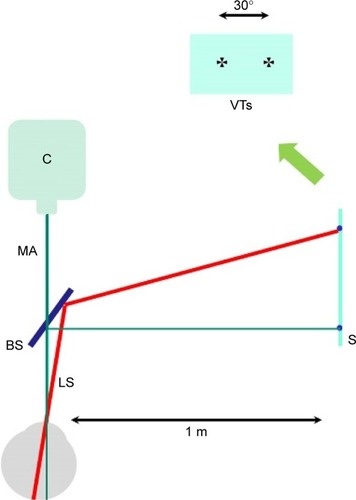
Maltese cross was used in this study as the fixation target. It was projected onto a screen which was placed 1 m away from the subjects. The subjects’ right eyes were able to see the targets through a beam splitter while keeping the left eye covered. For off-axis measurements, a fixation target projected onto the peripheral visual field was used to elicit eyes’ rotation (keeping the head fixed) relative to the aberrometer’s measurement axis. Measurements were taken 1 second after a blink to ensure a smooth and stable tear film. For each gaze position, three measurements were taken after realigning the instrument with the measured eye.
The range of eccentricities accessible in human subjects by our instrument is limited to 30° in both nasal and temporal horizontal visual fields.Citation29 We used the same approach in this study to judge the accessibility of the instrument for other visual field meridians (vertical meridian and two diagonal meridians) and obtained Zernike coefficients up to the sixth order. In our previous article,Citation28 we demonstrated the validity of using commercial software CLAS-2D to analyze off-axis data. We adopted the same analytical approach in this study: using CLAS-2D software to draw an analysis Zernike circle that is concentric with, and surrounds, the elliptical entrance pupil. Computation of the Zernike coefficients ignores the area between the analysis circle and the elliptical entrance pupil.Citation28 The mean ± SD pupil diameter across all subjects was 6.73±0.54 mm (range 5.70–7.75 mm) and the largest pupil was 7.75 mm. We, therefore, used a 7.75 mm circle as a common basis for Zernike analysis of all eyes. We computed the power vector components of the eye’s sphero-cylindrical refractive error (M: the SE, J0: with-the-rule (WTR) and against-the-rule (ATR) astigmatism, J45: oblique astigmatism with axes at 45° and 135°) using the following equations from second-order Zernike terms.Citation30 All results refer to 840 nm wavelength infrared laser light, which was used in the COAS aberrometer. No correction for ocular chromatic aberration was required for differential results that quantify relative changes.
(1) where C is the Zernike coefficient for defocus
, WTR/ATR astigmatism
and oblique astigmatism
, and r is the pupil radius.
Statistical nonparametric sign testCitation31 and linear mixed-model analysis were performed to evaluate the changes in refractive error and amount of peripheral retinal image blur between different refractive error groups. Repeated measures analysis of variance was used to analyze refraction changes across the four meridians. A critical p-value of 0.05 was used to denote statistical significance. Least-square fitting of the data was applied to minimize the sum of squared residuals and the best fit was determined. A triangle-based cubic interpolation method to fit the IQ data across the 60° entire visual field was applied in this study to evaluate the IQ in different sections of the visual field.
Results
gives a summary of the participants’ age and their central refractive error profiles. gives a summary of the major findings of different refraction components (M, J0 and J45) with respect to change as a function of visual field eccentricities across the four meridians.
Table 1 Study participant’s group profiles
Table 2 Summary of refraction profiles along four meridians
Horizontal visual field
In order to show clearly the relative changes of M component at various visual angles, we normalized all the data displayed in by subtracting the central M value from the measurements at each angle (same in , and ). Positive and negative values on the Y axis of indicated relative hyperopic and relative myopic shifts, respectively (same in , and ). In general, Em. and LM subjects showed small myopic peripheral relative M (PRM; <1.00 D). MM and HM subjects exhibited relative hyperopic shifts (). MM eyes have 2.23±0.98 D (mean ± standard error of the mean, same notations in the following text) relative hyperopia in the nasal 30° visual field, and HM eyes show 2.90±0.81 D relative hyperopia (). In the temporal visual field, MM and HM eyes have 1.10±0.35 and 1.39±0.59 D relative hyperopia, respectively, at 30°. Our findings are consistent with previous studies regarding the asymmetry of the spherical power vector component M in the nasal and temporal horizontal periphery:Citation32–Citation35 MM and HM eyes have more hyperopic shift in the nasal than in the temporal peripheral visual field, with an average of 1.3 D difference when compared for a 30° angle (). We found greater intersubject variation in HM eyes than in the other group across all visual field angles. The peripheral relative hyperopic shift is statistically significantly different from the PRM shift in the following comparisons between the two groups at 30° in the temporal visual field and beyond 10° in the nasal visual field: HM vs LM (F=302.46, p=0.0082), HM vs Em. (F=173.82, p=0.016), MM vs LM (F=128.31, p=0.008) and MM vs Em. (F=187.77, p=0.011).
Figure 2 Changes of refractive components as functions of horizontal visual field angle.
Abbreviations: Em., emmetropic; HM, high myopic; LM, low myopic; MM, moderate myopic; SEM, standard error of the mean.
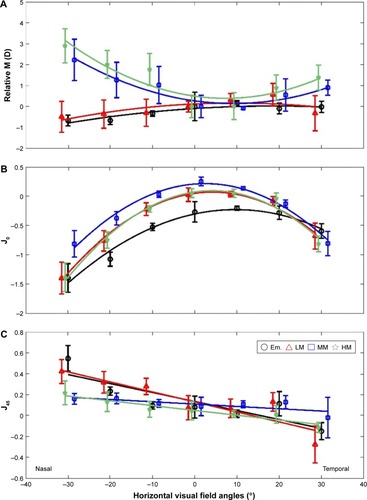
Figure 3 Changes of refractive components as functions of 45° diagonal visual field eccentricities.
Abbreviations: Em., emmetropic; HM, high myopic; LM, low myopic; MM, moderate myopic; SEM, standard error of the mean.
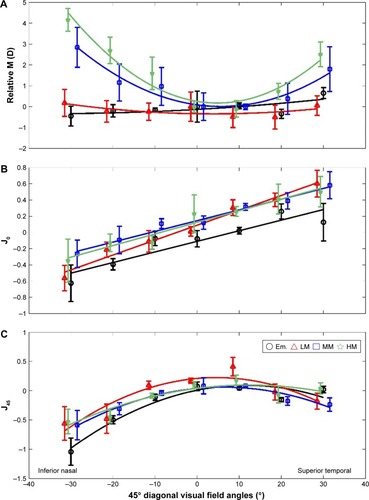
Figure 4 Changes of refractive components as functions of vertical visual field eccentricities.
Abbreviations: Em., emmetropic; HM, high myopic; LM, low myopic; MM, moderate myopic; SEM, standard error of the mean.
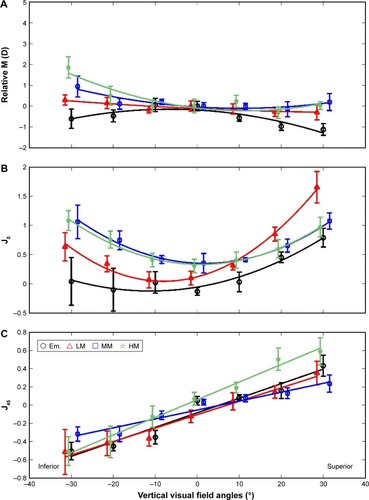
Figure 5 Changes of refractive components as functions of 135° diagonal visual field eccentricities.
Abbreviations: Em., emmetropic; HM, high myopic; LM, low myopic; MM, moderate myopic; SEM, standard error of the mean.
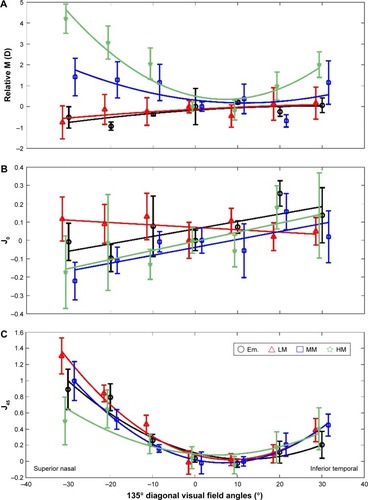
The astigmatic power vector J0 showed quadratic changes across the horizontal visual field (). With an increasing visual field angle, we observed an increased negative J0, which indicated more ATR astigmatism toward the horizontal periphery. The central J0 component in different groups was in the range from −0.23±0.68 to +0.33±0.58 D. It increased to an average of −1.21±0.45 and −0.78±0.51 D at 30° in the nasal and temporal horizontal fields, respectively. Although the Em. eyes have the least relative ATR increase and the HM eyes have the most relative ATR increase from the center to the horizontal periphery, the differences are not statistically significant (F=3.14, p=0.16). We also identified a nasal–temporal asymmetry in the J0 profiles as follows: more ATR astigmatism was observed in the nasal visual field than in the temporal visual field (F=101.52, p=0.0002, 0.42 D difference at 30° visual field angle). There was higher intersubjective variation in the periphery (0.87±0.21 D) than in the center (0.35±0.08 D; ).
The astigmatic power vector J45 was found, using least-square fitting, to be related linearly to the horizontal field angles, and it showed a maximum change of <0.5 D across the visual field. The change of J45 as a function of the visual field angle showed negative slopes for all groups. There were no significant differences between the slopes of any two groups (all p>0.05), and these slopes were not significantly different from zero. The slope values varied from −0.002 D/degree (D/Deg.) in moderate myope group to −0.01 D/Deg. in low myope group ().
45° Diagonal visual field
Consistent with the findings of the PRM profiles for MM and HM subjects across the horizontal meridian, PRM also showed a hyperopic shift across the 45° diagonal visual field in these two subject groups. High myopes had more hyperopic shift in the periphery than moderate myopes (). There were significantly larger hyperopic shift in high myopes than in moderate myopes at the inferior nasal (IN) 20° and 30° (F=181.27, p=0.003). In addition, an asymmetric profile was also observed with larger amounts of hyperopic PRM shift in the IN visual field than in the superior temporal (ST) visual field. High myopes exhibited 4.16±0.53 D hyperopic shift at the 30° IN visual field and 2.70±0.60 D hyperopic shift at the 30° ST visual field, with 1.46 D difference between IN and ST visual fields. Moderate myopes showed 2.92±0.95 and 1.91±1.07 D hyperopic shifts at the 30° IN and ST, respectively, with 1.00 D difference ().
Unlike the myopic shift profiles of PRM in low myopes and emmetropes across the horizontal visual field, the changes of PRM in these two groups showed flat profiles across the 45° diagonal visual field with a variation of <1.0 D in Em. and 0.60 D in LM subject groups. There were no significant differences of PRM at any visual field angle between the two groups (F=0.52, p=0.752; ).
J0 showed a linear function across the 45° diagonal visual field from the IN to the ST quadrant with positive slopes in all groups. Increased ATR astigmatisms were observed toward the IN visual field. In contrast, increased WTR astigmatisms were found toward the ST visual field. There were no significant differences in the slopes of the linear J0 variation profiles between the four groups (F=21.06, p=0.855). They had an average slope value of 0.015±0.006 D/Deg. The changes of J0 were within 1.00 D across the entire 60° visual field except for the LM group, which had a J0 variation of 1.30 D (−0.60 D at the IN 30° and 0.70 D at the ST 30°; ).
The power vector component J45 exhibited a quadratic change with visual field eccentricities across the 45° diagonal meridian for all four subjects groups. More negative values of J45 were found in the IN and the ST visual fields than in the central J45. Although the groups showed slightly different patterns, there were no significant differences of the J45 profile (F=4.76, p=0.16; ). IN–ST asymmetry existed in J45 profiles with more negative values of J45 in the IN visual field (−0.87±0.16 D at 30° IN) than in the ST visual field (−0.21±0.087 D at 30° ST). Greater between-subjects variation occurred at larger off-axis eccentricities ().
Vertical visual field
Myopic PRM was observed in the Em. group across the vertical visual field, while moderate and high myopes tended to have hyperopic peripheries (). This was consistent with the findings of PRM profiles in the horizontal and 45° diagonal meridians. PRM varied in a small range of 0.50 D for the low myopes. LM subjects tended to have a hyperopic shift in the inferior visual field (, blue symbols). When comparing the amount of hyperopic shift of PRM in MM and HM subjects, more hyperopic shift was found in high myopes (F=172.43, p=0.0045). There was a 1.94±0.87 D hyperopic shift of PRM in the inferior 30° visual field in HM group. This was 1.0 D larger than that in the MM group (). The asymmetry of PRM profiles in the inferior and superior visual fields was also noticeable. There were more hyperopic shifts toward the inferior visual field than toward the superior visual field in the MM and HM groups, and there were more myopic shifts toward the superior quadrant than toward the inferior field for the Em. group. The Em. subjects had −1.32±0.65 D myopic shift in the superior visual field and −0.64±0.41 D myopic shift in the inferior visual field. Larger amounts of between-subjects variations were also present with increasing visual field eccentricities (0.52±0.32 D at 30° inferior vs 0.23±0.17 D at the center; ).
J0 across the vertical meridian also exhibited a quadratic function with an increase in off-axis angles (). But unlike the J0 changes across the horizontal meridian, WTR astigmatisms were found toward the vertical periphery. The variation of J0 across the visual field was within the range of 1.00 D for all subject groups except for the LM group, which showed an increase of 1.80±0.76 D WTR astigmatism in the superior visual field toward 30° periphery. The asymmetry of the J0 profile in the two quadrants was more noticeable in the Em. and LM groups. More WTR astigmatism appeared in the superior visual field than in inferior visual field (30°: F=76.38, p=0.002; ).
J45 showed a linear relationship with the change in vertical visual field angles (). Positive slopes were found, with an average value of 0.02±0.008 D/Deg., regardless of the foveal refractions. There were no significant differences in the slopes. From inferior 30° to superior 30°, J45 changed 1.15 D in the HM group, while the change was 0.87±0.07 D for all the other groups ().
135° Diagonal visual field
PRM showed hyperopic shifts in the periphery of the 135° visual field for MM and HM subjects (F=103.34, p=0.0072; ). HM subjects exhibited more hyperopic shifts than MM subjects (F=32.78, p=0.017). In the superior nasal (SN) quadrant, PRM shifted to 4.12±0.71 and 1.41±0.62 D for high and moderate myopes, respectively. In the inferior temporal (IT) quadrant, the hyperopic shifts were 2.03±0.31 and 1.17±0.49 D for these two groups, respectively. This also indicated an SN–IT asymmetry of the PRM profiles for the two groups: moderate to high myopes tended to have more hyperopic shifts in the SN visual field. There were no noticeable myopic or hyperopic shifts to the periphery across this meridian in Em. and LM subjects (F=5.07, p=0.321). But these groups tended to exhibit slight myopic shifts in the superior visual fields. The PRM values varied in a range of 0.70 D for these two groups across the entire 60° oblique visual field ().
J0 exhibited a linear dependence of the visual field angles across the 135° diagonal meridian for all subject groups (). Except the LM group, all groups demonstrated a positive slope, although it was relatively flat (slope value =0.006±0.001 D/Deg.). From the SN to the IT visual field, J0 astigmatism varied in a small range of 0.50 D in the study groups. There were increases in the between-subjects measurement variances (). The J45 astigmatism component showed a quadratic relationship with the increase in visual field angle toward the SN and the IT peripheries (). However, there were no statistical differences of these quadratic patterns between the different study groups (F=54.69, p=0.23). A noticeable SN–IT asymmetry in the J45 shift was found, and there were larger amounts of J45 in the SN quadrant than in the IT quadrant (on an average of 0.97±0.63 and 0.36±0.25 D at the 30° SN and IT periphery, respectively). Increased between-subjects variances were also observed when the measurements were taken at larger off-axis angles ().
Peripheral IQ
The results described above show that the spherical component M and the astigmatic power vectors vary across the entire visual field. If IQ is a driving force for myopia progression as suggested previously,Citation4–Citation8 it is important to determine the combined effects of M, J0 and J45. To determine the peripheral IQ which combines the optical effects of spherical and astigmatic power vector components, we analyzed the total sphero-cylindrical image blur on the peripheral retina across the entire visual field. We calculated this total Sphero-Cylindrical Retinal Image Blur (SCRIB) using the following equation:
(2)
We use a triangle-based cubic interpolation methodCitation21 to fit the SCRIB data across the 60° entire visual field. Em. eyes show an increased amount of SCRIB in all peripheral eccentricities (all p<0.05) with exceptions of far periphery (30°) at the IT and ST visual fields (both p>0.05). The greatest SCRIB increase, 1.38±0.71 D, was found at 30° nasal periphery (). All myopia groups show increased image blur in the nasal, superior nasal and superior visual fields (all p<0.05), but with much lower amount (about 1/2) compared with emmetropes (). The SCRIB decrease in T, IT, and I visual field (VF) of myopic subjects. A nearly one-third diopter smaller retinal image blur was noticed at 30° in IT visual field compared with the center. The best IQ, in terms of SCRIB, was achieved between 10° and 20° in the temporal visual field of myopic subjects ().
Figure 6 Relative SCRIB data (peripheral SCRIB – central SCRIB) fitted across 60° visual field using a triangle-based cubic interpolation method in (A) emmetropia, (B) low myopia, (C) moderate myopia and (D) high myopia.
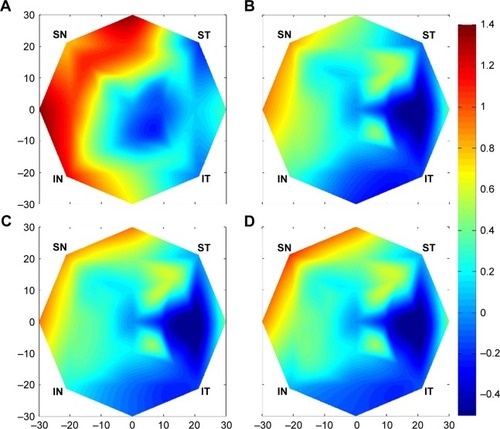
Discussion
Most of the previous studies on peripheral optics of the human eye were conducted only along the horizontal and vertical visual fields.Citation29,Citation33,Citation36–Citation40 These studies show that the refraction varies differently along the nasal and temporal visual fields. Our study confirmed that a myopic eye along the horizontal visual field is generally less myopic (or relatively hyperopic) in its periphery. As pointed out in previous studies,Citation29,Citation40 the magnitude of spherical power vector component M varies in proportion to the foveal refractive error. We found in our study that, along the horizontal meridian, moderate and high myopes showed higher amounts of relative hyperopic refractive error compared to low myopes and emmetropes. As expected, the astigmatism (mainly the J0 component) increased dramatically (quadratically as a function of the visual field angle) and exhibited more ATR astigmatism into the horizontal periphery. This would deteriorate the peripheral retinal IQ considerably.
Our finding suggests that the conventional approach to generalize the characteristics of peripheral refraction and retinal IQ solely based on findings in the horizontal visual field is questionable since the real eyes do not generally exhibit rotational symmetry.Citation41,Citation42 The few existing studies which extended peripheral refraction measurements to other peripheral positions in addition to horizontal meridian found different amounts of peripheral refractive errors for other meridians.Citation34,Citation39,Citation43,Citation44 For example, Seidemann et alCitation39 conducted photorefraction measurements across 22° of the visual field of 18 myopic subjects. Their results show relative peripheral myopia across the retina, except for the superior visual field in which they found relative hyperopia. Mathur et al,Citation43 using aberrometry in nine myopia subjects, found relative peripheral myopia across the retina in all their subjects. Atchison et al,Citation32 by performing autorefraction measurements along both horizontal and vertical meridians, found that myopic eyes showed relative hyperopia along the horizontal meridian but relative myopia along the vertical meridian. Consistent with Atchison et al, Berntsen et alCitation44 reported myopic children have relative hyperopia in horizontal periphery and relative myopia in vertical periphery. A cross-sectional study conducted by Ehsaei et al reported that myopic eyes show a relative hyperopic shift in the periphery along all four measured meridians.Citation34
The conflicting findings in these studies may, partly, be caused by the variation of subjects’ myopia severity from study to study. We found that MM and HM subjects have relative hyperopic periphery in all four meridians. The data reveal that a higher amount of central myopia is associated with more peripheral relative hyperopic shift. gives an illustration of the differences in PRM patterns of the four groups in this study. Em. eyes exhibited a relative myopic shift in five out of eight octants. LM eyes exhibited an LM shift in three out of eight octants and a hyperopic shift in one octant. All the other octants in these two subject groups showed close to zero PRM, which means no relative hyperopic or myopic defocus shift. In contrast, MM to HM eyes did not exhibit any myopic PRM patterns. All eight octants in these two subject groups showed a hyperopic shift. The amount of this hyperopic shift increased with the amount of their central myopia.
Figure 7 PRM patterns in different subject groups.
Abbreviations: Em., emmetropic; HM, high myopic; LM, low myopic; MM, moderate myopic; PRM, peripheral relative M.
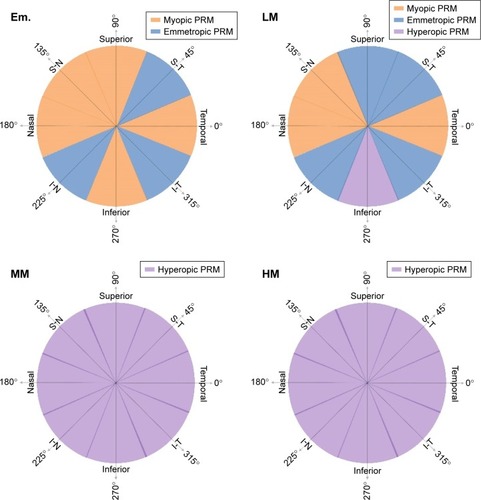
It is readily observed that patterns of PRM are associated with the refractive states of central vision (). Although previous studies have suggested that PRM may influence the development of a central refractive error,Citation4–Citation8,Citation12–Citation15 the causal–effect relationship remains undetermined. This means that it is still unclear whether hyperopic PRM is a driving force for the refractive error development or is simply a result of the myopia progression.Citation29,Citation45 A recent study, using three-dimensional magnetic resonance imaging to investigate the changes in eye dimensions and retinal shape with the degree of myopia, suggested that eyes’ oblateness decreased with an increase in myopia.Citation41 Our results confirm that, as myopia increases, more hyperopic PRM is found across the entire visual field in all meridians. Therefore, the shape of the retina is relatively more prolate, or less oblate, in moderate and high myopes. Data in this study also suggest that emmetropes and low myopes, in contrast, tend to have an overall spherical or some degree of oblate retinal shapes. These findings are in agreement with the results from another cross-sectional study of peripheral refraction in myopes and emmetropes.Citation34 An overall larger amount of image blur in the periphery of Em. eyes in this study may indicate the causation of myopia onset and progression based “grow to clarity” theory.Citation16
Off-axis astigmatism increased considerably with visual field eccentricities.Citation29 Although a number of animal studies using chicken model suggested that imposed astigmatic error does not play an important role during emmetropization,Citation46,Citation47 some other studies showed that astigmatism could influence the development of myopiaCitation48,Citation49 or emmetropization process.Citation5 Thus, it is still unclear whether off-axis astigmatism influences refractive error development. Our findings of quadratic field dependence of the J0 to horizontal and vertical eccentricities, and those of quadratic field dependence of the J45 to 45° and 135° diagonal visual field eccentricities are consistent with Seidel’s theory.Citation50 According to this theory, the M component (defocus) of the refraction shows quadratic field dependence. This was also apparent in our data, especially for MM to HM subjects, across all the four meridians in the entire visual field.
Conclusion
This study examined the peripheral refractive error changes in all four major meridians of the human eye with detailed subgrouping based on the amount of their central myopic refractive error. The findings indicate an overall Em. or slightly relative myopic periphery (spherical or oblate retinal shape) formed in emmetropes and low myopes, while moderate and high myopes form relative hyperopic periphery (prolate, or less oblate, retinal shape). In general, human Em. eyes demonstrate higher amount of peripheral retinal image blur.
Disclosure
The authors report no conflicts of interest in this work.
References
- FankhauserFEnochJMThe effects of blur upon perimetric thresholds. A method for determining a quantitative estimate of retinal contourArch Ophthalmol19626824025113891538
- WangYZThibosLNOblique (off-axis) astigmatism of the reduced schematic eye with elliptical refracting surfaceOptom Vis Sci19977475575629293525
- WilliamsDRArtalPNavarroRMcMahonMJBrainardDHOff-axis optical quality and retinal sampling in the human eyeVision Res199636110311148762715
- CharmanWNAberrations and myopiaOphthalmic Physiol Opt200525428530115953113
- KeeCSHungLFQiao-GriderYRoordaASmithEL3rdEffects of optically imposed astigmatism on emmetropization in infant monkeysInvest Ophthalmol Vis Sci20044561647165915161822
- MarcosSBarberoSLlorenteLThe sources of optical aberrations in myopic eyesInvest Ophthalmol Vis Sci20024313 Abstract 1510
- ThornFHeJThomSHeldRGwiazdaJThe vision of myopic children: how wavefront aberrations alter the image of school book textMyopia 2000: Proceedings of the VIII International Conference in MyopiaBoston2000
- WildsoetCStructural correlates of myopiaRosenfieldMGilmartinBMyopia and NearworkNew York, NYButterworth-Heinemann19983156
- WallmanJWinawerJHomeostasis of eye growth and the question of myopiaNeuron20044344746815312645
- HodosWKuenzelWJRetinal-image degradation produces ocular enlargement in chicksInvest Ophthalmol Vis Sci19842566526596724835
- DietherSSchaeffelFLocal changes in eye growth induced by imposed local refractive error despite active accommodationVision Res19973766596689156210
- SchmidGFRetinal steepness vs myopic shift in children (Abstract)Optom Vis Sci20041223
- WallineJJonesLAMuttiDOZadnikKA randomized trial of the effects of rigid contact lenses on myopia progressionArch Ophthalmol2004122121760176615596577
- HoogerheideJRemptFHoogenboomWPAcquired myopia in young pilotsOphthalmologica197116342092155127164
- MuttiDOSholtzRIFriedmanNEZadnikKPeripheral refraction and ocular shape in childrenInvest Ophthalmol Vis Sci20004151022103010752937
- WildsoetCFActive emmetropization – evidence for its existence and ramifications for clinical practiceOphthalmic Physiol Opt19971742792909390372
- WangYZThibosLNLopezNSalmonTBradleyASubjective refraction of the peripheral field using contrast detection acuityJ Am Optom Assoc199667105845898942130
- BerntsenDAMuttiDOZadnikKValidation of aberrometry-based relative peripheral refraction measurementsOphthal Physiol Opt20082818390
- RadhakrishnanHCharmanWNRefractive changes associated with oblique viewing and reading in myopes and emmetropesJ Vis2007785
- JaekenBArtalPOptical quality of emmetropic and myopic eyes in the periphery measured with high-angular resolutionInvest Ophthalmol Vis Sci20125373405341322511633
- MathurAAtchisonDAScottDHOcular aberrations in the peripheral visual fieldOpt Lett200833886386518414558
- OsuagwuULSuheimatMAtchisonDAPeripheral aberrations in adult hyperopes, emmetropes and myopesOphthalmic Physiol Opt201737215115928211176
- CollinsMJWildsoetCFAtchisonDAMonochromatic aberrations and myopiaVision Res1995359115711637610577
- LiLChengGPMNgALKChanTCYJhanjiVWangYInfluence of refractive status on the higher-order aberration pattern after small incision lenticule extraction surgeryCornea201736896797228628503
- ThibosLNHongXClinical applications of the Shack-Hartmann aberrometerOptom Vis Sci1999761281782510612402
- ChengXHimebaughNLKollbaumPSThibosLNBradleyAValidation of a clinical Shack-Hartmann aberrometerOptom Vis Sci200380858759512917578
- ChengXHimebaughNLKollbaumPSThibosLNBradleyATest-retest reliability of clinical Shack-Hartmann measurementsInvest Ophthalmol Vis Sci200445135136014691194
- ShenJThibosLNMeasuring ocular aberrations and image quality in peripheral vision with a clinical wavefront aberrometerClin Exp Optom200992321222219462503
- ShenJClarkCASoniPSThibosLNPeripheral refraction with and without contact lens correctionOptom Vis Sci201087964265520601913
- ThibosLNWheelerWHornerDPower vectors: an application of Fourier analysis to the description and statistical analysis of refractive errorOptom Vis Sci19977463673759255814
- SiegelSNonparametric Statistics for the Behavioral SciencesNew York, NYMcGraw-Hill1956
- AtchisonDAPritchardNSchmidKLPeripheral refraction along the horizontal and vertical visual fields in myopiaVision Res2006468–91450145816356528
- AtchisonDAScottDHCharmanWNHartmann-Shack technique and refraction across the horizontal visual fieldJ Opt Soc Am A Opt Image Sci Vis200320696597312801164
- EhsaeiAMallenEAChisholmCMPaceyIECross-sectional sample of peripheral refraction in four meridians in myopes and emmetropesInvest Ophthalmol Vis Sci201152107574758521873675
- FerreeCRandGHardyCRefraction for the peripheral field of visionArch Ophthalmol19315717731
- LuFMaoXQuJXuDHeJCMonochromatic wavefront aberrations in the human eye with contact lensesOptom Vis Sci200380213514112597328
- LundstromLUnsboPGustafssonJOff-axis wave front measurements for optical correction in eccentric viewingJ Biomed Opt200510303400216229646
- MuttiDOHavesJRMitchellGLRefractive error, axial length, and relative peripheral refractive error before and after the onset of myopiaInvest Ophthalmol Vis Sci20074862510251917525178
- SeidemannASchaeffelFGuiraoALopez-GilNArtalPPeripheral refractive errors in myopic, emmetropic, and hyperopic young subjectsJ Opt Soc Am A Opt Image Sci Vis200219122363237312469730
- AtchisonDAHigher order aberrations across the horizontal visual fieldJ Biomed Opt20061133402616822075
- PopeJMVerkicharlaPKSepehrbandFSuheimatMSchmidKLAtchisonDAThree-dimensional MRI study of the relationship between eye dimensions, retinal shape and myopiaBiomed Opt Express2017852386239528663880
- VerkicharlaPKMathurAMallenEAPopeJMAtchisonDAEye shape and retinal shape, and their relation to peripheral refractionOphthalmic Physiol Opt201232318419922486366
- MathurAAtchisonDACharmanWNMyopia and peripheral ocular aberrationsJ Vis2009910p15
- BerntsenDAMuttiDOZadnikKStudy of Theories about Myopia Progression (STAMP) design and baseline dataOptom Vis Sci2010871182383220935586
- AtchisonDARosenRThe possible role of peripheral refraction in development of myopiaOptom Vis Sci20169391042104427560691
- McLeanRCWallmanJSevere astigmatic blur does not interfere with spectacle lens compensationInvest Ophthalmol Vis Sci200344244945712556368
- SchmidKWildsoetCFNatural and imposed astigmatism and their relation to emmetropization in the chickExp Eye Res19976458378479245915
- FultonABHansenRMPetersenRAThe relation of myopia and astigmatism in developing eyesOphthalmology19828942983027099549
- IrvingELCallenderMGSivakJGInducing ametropias in hatchling chicks by defocus–aperture effects and cylindrical lensesVision Res1995359116511747610578
- SmithGAtchisonDAThe Eye and Visual Optical InstrumentsCambridgeCambridge University Press1997601