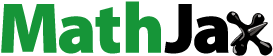
Abstract
Purpose
Sulforaphane is a phytochemically derived organic isothiocyanate 1-isothiocyanato-4-methylsulfinyl-butane present naturally in crucifers, including broccoli and cauliflower. Biochemically, it has been reported to induce the transcription of several antioxidant enzymes. Since such enzymes have been implicated in preventing cataract formation triggered by the intraocular generation of oxy-radical species, the purpose of this investigation was to examine whether it could induce the formation of antioxidant enzymes in the eye lens. Thioredoxin reductase (TrxR) was used as the target of such induction.
Methods
Mice lenses were cultured for an overnight period of 17 hours in medium 199 fortified with 10% fetal calf serum. Incubation was conducted in the absence and presence of sulforaphane (5 μM). Subsequently, the lenses were homogenized in phosphate-buffered saline (PBS), followed by centrifugation. TrxR activity was determined in the supernatant by measuring the nicotinamide adenine dinucleotide phosphate (reduced) (NADPH)-dependent reduction of 5,5′-dithiobis-2-nitrobenzoic acid (DTNB). Non-specific reduction of DTNB was corrected for by conducting parallel determinations in the presence of aurothiomalate. The reduction of DTNB was followed spectrophotometrically at 410 nm.
Results
The activity of TrxR in the lenses incubated with sulforaphane was found to be elevated to 18 times of that observed in lenses incubated without sulforaphane. It was also noticeably higher in the lenses incubated without sulforaphane than in the un-incubated fresh lenses. However, this increase was much lower than that observed for lenses incubated with sulforaphane.
Conclusion
Sulforaphane has been found to enhance TrxR activity in the mouse lens in culture. In view of the protective effect of the antioxidant enzymes and certain nutrients against cataract formation, the findings suggest that it would, by virtue of its ability to enhance the activity of such enzymes, prevent the tissue against oxidative stress that leads to cataract formation. Additional studies with the activities of other antioxidant enzymes such as quinone oxidoreductase and the levels of Nrf2 are in progress.
Introduction
Cataract is one of the leading causes of blindness and visual impairment. Globally, it accounts for over 60% of the total number of individuals suffering from visual impairment.Citation1,Citation2 Its very high prevalence in the tropical and subtropical countries, as well as in the temperate regions with excessive sunlight, strongly suggests that intraocular photochemical generation of superoxide and its derivatization to other reactive oxygen species (ROS), particularly in the aqueous humor and the lens,Citation3–Citation6 constitutes a significant risk factor in its etiology. Such ROS generation is considered incessant, at least during prolonged periods of photopic vision, light, and oxygen acting synergistically in continuing the generation of these entities by cyclic pseudo catalytic reactions propagated in the presence of endogenously available photosensitizers.Citation5 Prevention of the consequent oxidative stress by the application of antioxidants that could effectively scavenge the ROS is, hence, considered a viable means of medically offsetting the onset and progression of this vision-impairing disease.Citation7 Evidence of this hypothesis was initially obtained through in vitro culture experiments referred to above,Citation3–Citation6 as well as through many subsequent in vivo studies where oxidative stress to the lens and consequent cataract formation was induced by the administration of sodium selenite, the induction of diabetes, and feeding animals a high-galactose diet. The administration of various nutritional and metabolic antioxidants, such as bioflavonoids,Citation8,Citation9 vitamin E,Citation10,Citation11 caffeine,Citation2–Citation16 and pyruvate,Citation17 has been shown to prevent cataract formation in most of these experimental animal models. The hypothesis has also been supported by field studies showing that a lower incidence of cataracts correlated with a higher intake of vitamins C and E.Citation18–Citation20 While these antioxidants can protect the lens and other tissues against oxidative stress by scavenging ROS and consequently minimizing the impact of toxic reactions, such as the oxidative inactivation of many _SH enzymes, loss of glutathione and ascorbate, lipid peroxidation and protein cross-linking, etc, the protective effect of such compounds is likely to be short-lived, especially in the eye, where ROS generation is much more overwhelming than in other parts of the body, except portions of skin that are more exposed to light. This limitation in the effectiveness of nutritional antioxidants is also due to their interrupted availability with meals, as well as the variability in the composition of the diet itself and the unstable nature of the antioxidants.
We have recently demonstrated that oxidative stress in the lens up-regulates the generation of several toxic microR-NAsCitation21,Citation22 that widely knock out the translation and transcription of antioxidant genes, as well as transcription factors such as Nrf2.Citation23 Therefore, we anticipate that a more stable and consistent anticataractogenic effect can be achieved by the topical administration of compounds that can antagonize such genetic effects, as shown with caffeine.Citation15,Citation16 This is also likely to be achieved by the administration of antisense microRNAs that can overcome the transcriptional and translational limitations imposed by the aberrant production of certain microRNAs. However, success with using such antisense microRNAs could be limited because of their non-specificity and the wider range of their hybridization with sense miRs. Fortuitously, many recent studies have demonstrated that certain phytochemical ingredients that are available nutritionally, such as sulforaphane 1-isothiocyanato-4-methylsulfinylbutane, which is present in many crucifers, including broccoli,Citation24 and curcumin,Citation25 present in turmeric, can up-regulate the transcription of various antioxidant enzymes. However, the mechanism of its action remains only partially understood, though it is purportedly brought about by its ability to up-regulate Nrf2 biogenesis in the cytoplasm, followed by its transport to the nucleus. This increases the probability of its binding to the promoters present in the ARE region of antioxidant genes, enhancing the transcription of several possible antioxidant genes with the consequence of favorable physiologic effects. However, the level of such an enhancement, and its physiologic consequences, is likely to vary among different tissues because of varied cross-talk among various transcriptional pathways.Citation26,Citation27 Therefore, the primary objective of this investigation was to examine whether sulforaphane would indeed exert its transcriptional effect by inducing antioxidant enzymes in the lens. Presently, this was done using the lens organ culture system with mouse lenses by current techniques.Citation13 Thioredoxin reductase (TrxR) was selected as the target enzyme. The results are in accordance with the hypothesis. The activity of this enzyme was substantially elevated in the lenses cultured with sulforaphane in comparison with the lenses cultured without sulforaphane, suggesting the possibility of its use against cataracts. The results are also consistent with the findings that oxidative stress leads to the induction of micro RNAs that inhibit Nrf2 transcription.
Materials
Experiments were conducted using lenses of C56Bl/6 mice, obtained from Harlan Farm Laboratories and used in accordance with Association for Research in Vision and Ophthalmology (ARVO) regulations and approved by the Institutional Animal Care and Use Committee. The weight of the animals was 23 to 25 g. They were fed standard rodent chow and maintained in a 12-hour dark and light cycle. Most of the chemicals were obtained from the Sigma-Aldrich (St Louis, MO, USA). Aurothiomalate, nicotinamide adenine dinucleotide phosphate (reduced) (NADPH), and 5,5′-dithiobis-2-nitrobenzoic acid (DTNB) were obtained as a kit from the Cayman Chemical Company (Ann Arbor, MI, USA). l-Sulforaphane was obtained from Sigma-Aldrich.
Methods
The TrxR activity of the lenses was determined by using the following biochemical reaction while spectrophotometrically monitoring the reduction of DTNB to TNB, with the 2-nitro-5-thiobenzoate derivative ionizing to TNB2–, with a molar absorption coefficient of 13,600:Citation28
Lenses were obtained from the eyes of mice sacrificed by euthanasia with CO2 inhalation. Soon after, the eyes were enucleated and the lenses dissected out by the posterior approach, rinsed briefly with phosphate-buffered saline (PBS), warmed to 37°C, and then transferred to the incubation medium in an incubator maintained at 37°C and gassed with 5% CO2. The incubation period was 17 hours. The weight of the lenses was 6.5 ± 0.5 mg. The incubation medium in the control group consisted of TC-199 containing Earle’s salts and glutamine, without phenol red and modified to contain 10% fetal bovine serum, 1,000 units of penicillin, and 100 μg of streptomycin per 100 mL. In the experimental group, the medium contained, in addition, 5 μM l-sulforaphane, achieved by adding aliquots from a stock solution made in dimethyl sulfoxide (DMSO). An equivalent volume of DMSO without sulforaphane was added to the controls. The lenses were incubated in groups of six, one lens per mL.
Subsequent to incubation, the individual lenses were briefly rinsed with 100 μL of PBS warmed to 37°C, blotted gently just enough to remove the adhering liquid, and transferred promptly to microfuge tubes to be frozen at −20°C. In addition to helping in the prevention of the loss of enzyme activities, freezing helped in the visual localization of the mouse lenses in the microfuge tube for its homogenization. An aqueous extract of the lens was then prepared by its homogenization in 0.5 mL of PBS followed by centrifugation at 18,000 relative centrifugal force (RCF) for 12 minutes at 0°C. The protein concentration of the supernatant was determined using the Bradford reagentCitation29 obtained from Bio-Rad (Hercules, CA, USA; catalog #500-000). The basal level of TrxR activity was determined in the freshly isolated un-incubated lenses.
The TrxR activity was determined by adding 10 uL of the lens extract to 190 μL of the assay reagent containing 25 uM NADPH and 500 μM DTNB and following its reduction for 160 seconds, as shown in . The amount of TNB so generated was calculated from ∆OD values, using 13,600 as its molar extinction coefficient. The final value was multiplied by two in view of the production of two moles of TNB generated in the reaction per unit of activity. The reaction was carried out in two cuvettes simultaneously, in the absence and presence of 20 uM sodium aurothiomalate, found adequate to nearly completely inhibit DTNB reduction. The assay reagent was prepared in buffer consisting of 50 mM potassium phosphate, 50 mM KCl, 1 mM ethylenediaminetetraacetic acid (EDTA), and 0.2 mg/mL bovine serum albumin, adjusted to pH 7. The amount of lens protein added to start the reaction was 25 mg.
Figure 1 Effect of sulforaphane on thioredoxin reductase activity of lenses incubated with sulforaphane. Representative computer printout depicting 5,5′-dithiobis-2-nitrobenzoic acid (DTNB) reduction by the lens extract in the absence (□) and presence (○) of aurothiomalate, as described in the text. The lenses had been incubated with 5 μM sulforaphane for 17 hours. A substantially higher rate of reduction in the absence of aurothiomalate with a substantial inhibition in its presence proves elevation of thioredoxin reductase activity caused by sulforaphane, with reference to the data with activity in lenses incubated without sulforaphane as described in . The actual activities as summarized in were calculated by the difference in the two rates, multiplied by two as per stoichiometry of the reaction.
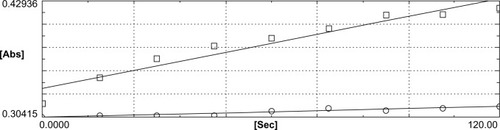
Figure 2 The computer tracing representing the reduction of 5,5′-dithiobis-2-nitrobenzoic acid (DTNB) by the lenses incubated without sulforaphane (controls), determined in the absence (□) and the presence (○) of aurothiomalate. Relative to rates depicted in , the difference in two activities is much less, indicating lower thioredoxin reductase levels in the lenses incubated without sulforaphane.
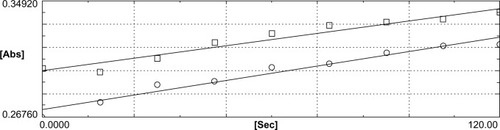
Table 2 Quantitative representation of thioredoxin reductase activity
Results
is representative of the spectrophotometric tracing of the course of the DTNB reduction (∆OD) caused by TrxR activity. As may be noted by the slope of the upper tracing, the rate of DTNB reduction was fairly linear and significantly more prominent and higher with extracts of lenses incubated with sulforaphane. That this reduction is attributable to TrxR was proven by its striking inhibition by aurothiomalate, the slope of reaction being demonstrably much lower in this case. Besides the slope, the substantial difference in the reaction rates between the sample run without (the upper tracing) and with the inhibitor (the lower tracing), which determines the actual enzyme activity, proves the authenticity of the significant elevation of the enzyme in the sulforaphane-treated tissue.
In contrast, as shown in , the rate of DTNB reduction was demonstrably lower in experiments done with lenses incubated without sulforaphane. In particular, the difference in the rates of reaction obtained with samples run without and with the inhibitor is much lower. The slopes of the two reactions are also closer to one another, contrary to that observed with sulforaphane-treated lenses, shown in . The inductive effect of sulforaphane in triggering the induction of TrxR is convincingly demonstrated.
This was also apparent by reference to the DTNB reduction rates obtained with un-incubated lenses, through the processes described above, showing lower enzyme activity than that observed with the lenses incubated even without sulforaphane. This suggests some induction just by moving the lens from its natural habitat to the culture medium as a stress response. This response is highly stimulated if sulforaphane is added to the medium.
summarizes the raw data on the range of absorption changes observed in different experiments. The values represent the ∆OD values per 25 μg of the lens proteins used in each reaction, corrected for the absorption changes observed in the presence of the inhibitor. Conforming to the original tracings represented in –, the mean ∆OD value is at least 18 times higher in the case of lenses incubated with sulforaphane than in the lenses incubated without sulforaphane, providing further evidence of the inductive activity of the compound. A comparison of the values in the lenses incubated in the control medium with the values in the un-incubated lenses points out that incubation in the basal medium itself is slightly stimulatory, representing the probability of a stress response in the tissue once transferred to an artificial medium. Information on the mechanism of this stress remains unknown at present, especially due to the complexity of the medium composition.
Figure 3 Thioredoxin reductase activity in basal lenses. The activity was determined as described in and . The difference in the rates observed in the absence (□) and the presence (○) of aurothiomalate was demonstrably lower than that shown in and , indicating a much lower activity.

Table 1 Absorption changes associated with the reduction of DTNB by thioredoxin reductase activity monitored at 410 nm
summarizes the data yielded when the ∆OD values are converted to micromoles of DTNB reduced, considering that each mole of DTNB reduction represents the formation of two moles of TNB. In conformity with the raw spectrophotometric data, the activities in the sulforaphane-treated lenses again are substantially greater than in the lenses incubated without sulforaphane.
Discussion
It has been previously shown that many plant-derived products, such as bioflavonoids, ascorbate, caffeine, vitamin E, and curcumin protect the lens against oxidative stress and cataract formation in vitro as well as in animal studies.Citation7,Citation15,Citation30 Although the dietary consumption of some of these antioxidants has also been found to be associated with a lower incidence of cataracts in humans,Citation18–Citation20 the medical use of these ingredients, therapeutically or nutritionally, for either cataract prevention or delay, has been limited and not practiced due to several difficulties, as referred to earlier. Surgical removal of the cataractous lens becomes the last resort for restoring vision. However, surgery alone has been unable to eliminate or even reduce the incidence of cataracts for several reasons, including a lack of technical labor and the availability of adequate modern surgically equipped facilities in large parts of the world. Even when facilities are available, subjects continue to live with impaired vision for several years before surgery is recommended and undertaken as a precautionary measure. In addition, cataract surgery is well known to enhance the chances of further loss of vision due to increased risk of vitreous or retinal detachment. Although the possibility of pharmacologically delaying or preventing cataract formation by the use of nutritional antioxidants has been shown in several studies referred to above, their oral or topical pharmacological use is beset with significant limitations. Once administered, orally or even topically, their concentration in the body begins to decline soon after due to their rapid metabolic disposal, as well as their exit through excretory routes.
The possibility of preventing oxidative stress to the lens by the therapeutic application of compounds that can inhibit cataract formation by modulating the phenomenon at the genetic level by offsetting the transcription of aberrant microRNAs under oxidative stress and consequent loss in the genesis of transcription factors and antioxidant enzymes has been little explored so far. However, the usefulness of such an approach is now suggested by reports in the treatment of certain cancers or genetic diseasesCitation31 using antisense micro-RNAs. That such an approach could be useful in cataract prevention is now also strongly indicated by our recent findings demonstrating an up-regulation in the transcription of several species of toxic microRNAs in the lenses of mice given a high-galactose diet – an animal model of sugar-induced oxidative stress and cataract formation and its inhibition by the oral administration of certain antioxidants, such as pyruvate and caffeine.Citation21,Citation22 An up-regulation in the transcription of several microRNAs can induce adverse effects on tissue physiology by several mechanisms, including the inhibition of the antioxidant gene transcription and consequent down-regulation of messenger RNAs (mRNAs) with a consequent decrease in the translation of protective proteins, including the antioxidant enzymes. We have previously reported an up-regulation of at least 25 microRNAs in the galactosemic lenses, known to induce apoptosis and oxidative stress.
The protective effect of caffeine against cataract formation has been shown to be due to its direct antioxidant effects, with simultaneous down-regulation of the production to toxic microRNAs that inhibit the production of antioxidant enzymes by directly hybridizing with the corresponding mRNA sequences in their 3′ un-translated regions (3′UTR),Citation32–Citation34 rendering them transnationally inactive. The decrease in the translation of certain transcription factors, such as Nrf2, has a limiting effect on the transcription of antioxidant genes directly in the nucleus.Citation35,Citation36 Its effectiveness in enhancing the transcription of antioxidant enzymes is ascribed to its ability to bind with the promoters in the AREs of antioxidant genes that triggers the transcriptional process. The physiopathological significance of such a down-regulation of Nrf2 is strongly suggested by studies showing substantial enhancement in the susceptibility of Nrf2 knockout (Nrf2–/–) mice to oxidative stress and increased susceptibility to diseases.Citation31,Citation37 Under normal conditions, this protein, transcribed from its mRNA in the cytoplasm, remains essentially locked therein, bound to actin-anchored Keep-1.Citation38,Citation39 Although its origin can be down-regulated under oxidative stress due to enhancement in microRNA transcription, the oxidation of –SHs in the Keep-1 protein under oxidative stress frees Nrf2 from Keep-1, enabling it to penetrate into the nucleus and trigger the antioxidant gene transcription by binding with the promoters in the AREs. The strategy of pharmacologically maintaining its level is thus considered useful for protecting the tissues against oxidative stress and consequent onset of the pathogenetic course.
Sulforaphane, by mechanisms not very well understood, has been shown to up-regulate Nrf2 transcription,Citation40–Citation42 and consequently exert an antioxidant effect in certain cancerous as well as non-cancerous tissues. Therefore, the primary objective of this investigation was to examine whether it can penetrate the lens and enhance the transcription of the antioxidant enzymes. This investigation provides early evidence of this possibility. This hypothesis has been verified by culturing mouse lenses with and without sulforaphane and then measuring the activity of TrxR, a very prominent member of the NADPH-disulfide oxido-reductase class of enzymes, including glutathione reductase. However, we first selected TrxR for this study in view of its activity with a wider spectrum of substrates, such as ubiquinone, lipoic acid and its amide, lipid hydro peroxide, vitamin K3, p53, hydrogen peroxide, dehydroascorbate, ascorbyl radicals, and peroxides, in addition to the protein disulfides.Citation43–Citation49 On the other hand, glutathione reductase is active only with glutathione disulfide (GSSG), without any effect on dehydroascorbate, peroxides, and other substrates referred to above. The ability of TrxR to reduce ascorbyl radicals and dehydroascorbate to ascorbate is highly interesting from the point of view of the physiology of the lens itself. Its concentration in the aqueous and lens is one of the highest in comparison with other tissues,Citation5 maintained by its active transport form the plasma to the aqueous and then in the lens. It is also well known to be effective in preventing oxidative stress in tissue exposed to ROS, under ambient as well as photochemical environs,Citation4 with adequate evidence of its effectiveness in attenuating cataract formation, even in humans, when consumed nutritionally.Citation18–Citation20 Since TrxR is able to catalytically reduce the ascorbyl radical as well as the dehydroascorbate, it is expected to be potentially helpful in minimizing the possible pro-oxidant effect of ascorbate, likely to be exerted in the presence of trace metals.
The corollary to this effect is that it also protects the lens against the possible cataractogenic effect of dehydroascorbate exerted by its ability to glycate and denature the lens proteins.Citation50 Since the reducing equivalents are derived from NADPH, the reduction of dehydroascorbate with this enzyme is without any depletion of glutathione (GSH), a primary antioxidant reserve. The NADPH required in these reactions is generated in the hexose-monophosphate oxidative pathway. In addition, TrxR could help in the maintenance of GSH by the NADPH-dependent elimination of lipid peroxides and hydrogen peroxide, its activity being up-regulated in situations generating an excess of these antioxidants.Citation51,Citation52
The pathophysiological changes in the lens associated with cataract formation have also been suggested to involve slowly progressing epigenetic changes similar to that in many other aging tissues.Citation53–Citation55 Such a possibility is indicated by disorganization of the chromatin fibers, associated with the formation of cataracts in diabetic miceCitation56 and its prevention by pyruvate. Therefore, it is interesting to find that the thioredoxin system also inhibits the hypermethylation and deacetylation of the histone moiety of the deoxyribonucleic acid (DNA).Citation57–Citation59
Conclusion
As summarized in the “Results” section, the incubation of lenses with sulforaphane led to a significant enhancement in the activity of TrxR. Its activity rose to about 18 times higher than activity in the lenses incubated without sulforaphane. It was also interesting to note that there was also a small but consistent increase in its activity in the lenses incubated without sulforaphane, compared with the level in the fresh un-incubated lenses, reflecting a stress response in the incubated tissue. This could be attributable to movement of the tissue from its natural habitat in the eye in vivo to an artificial culture medium. This movement also involves a change in the oxygen level in the environment: from a relatively hypoxic atmosphere in vivo to a fully normoxic environment under incubation. In addition, there is an inherent leakage of some constituents from the tissue during its isolation from the eye and during the period of culture. The induction of TrxR activity by sulforaphane is decidedly much stronger. However, whether this compound could be useful in cataract prevention remains to be determined. The beneficial effect of sulforaphane in protecting the lens against cataract formation is also predictable from its preventive effect against lens opacification induced by hydrogen peroxide in vitro.Citation60 It has also been reported to protect against light-induced damage to retinal pigment epithelium in isolated mouse eye cup preparations.Citation61
Disclosure
The authors report no conflicts of interest in this work.
References
- ResnikoffSPascoliniDMariottiSPPokharelGPGlobal magnitude of visual impairment caused by uncorrected refractive errors in 2004Bull World Health Organ200886637018235892
- ThyleforsBNégrelADPararajasegaramRDadzieKYGlobal data on blindnessBull World Health Organ1995731151217704921
- VarmaSDEtsTKRichardsRDProtection against superoxide radicals in rat lensOphthalmic Res19779421431
- VarmaSDKumarSRichardsRDLight-induced damage to ocular lens cation pump: prevention by vitamin CProc Natl Acad Sci19797635043506291017
- VarmaSDChandDSharmaYRKuckJFJrRichardsRDOxidative stress on lens and cataract formation: role of light and oxygenCurr Eye Res1984335576360540
- ZiglerJSJrGooseyJDSinglet oxygen as a possible factor in human senile nuclear cataract developmentCurr Eye Res1984359656690229
- VarmaSDHegdeKROxidative stress and cataract formation: horizons on its medical preventionExpert Rev Ophthalmol20072779801
- VarmaSDMikuniIKinoshitaJHFlavonoids as inhibitors of lens aldose reductaseScience1975188121512161145193
- VarmaSDMizunoAKinoshitaJHDiabetic cataracts and flavonoidsScience1977195205206401544
- CreightonMORossWMStewart-DeHaanPJSanwalMTrevithickJRModeling cortical cataractogenesis VII: Effects of vitamin E treatment on galactose-induced cataractsExp Eye Res1985402132223979462
- CreightonMOTrevithickJRCortical cataract formation prevented by vitamin E and glutathioneExp Eye Res1979296689693544285
- VarmaSDHegdeKRPrevention of oxidative damage to lens by caffeineJ Ocul Pharmacol Ther201026737720148663
- VarmaSDHegdeKRKovtunSUV-B-induced damage to the lens in vitro: prevention by caffeineJ Ocul Pharmacol Ther20082443944418788993
- VarmaSDHegdeKRKovtunSInhibition of selenite-induced cataracts by caffeineActa Ophthalmol2010887e245e24920977689
- VarmaSDKovtunSHegdeKEffectiveness of topical caffeine in cataract prevention: studies with galactose cataractMol Vis2010162626263321179241
- KronschlägerMLöfgrenSYuZTalebizadehNVarmaSDSöderbergPCaffeine eye drops protect against UV-B cataractExp Eye Res2013113263123644096
- ZhaoWDevamanoharanPSHeneinMAliAHVarmaSDDiabetes-induced biochemical changes in rat lens: attenuation of cataractogenesis by pyruvateDiabetes Obes Metab2000216517411220552
- RobertsonJMDonnerAPTrevithickJRVitamin E intake and risk of cataracts in humansAnn N Y Acad Sci19895703723822629606
- JacquesPFThe potential preventive effects of vitamins for cataract and age-related macular degenerationInt J Vitam Nutr Res19996919820510389028
- TanAGMitchellPFloodVMAntioxidant nutrient intake and the long-term incidence of age-related cataract: the Blue Mountains Eye StudyAm J Clin Nutr2008871899190518541583
- VarmaSDKovtunSHegdeKYinJRamnathJEffect of high sugar levels on miRNA expression. Studies with galactosemic mice lensesMol Vis2012181609161822736950
- VarmaSDKovtunSProtective effect of caffeine against high sugar-induced transcription of microRNAs and consequent gene silencing: a study using lenses of galactosemic miceMol Vis20131949350023441122
- BoettlerUVolzNTellerNInduction of antioxidative Nrf2 gene transcription by coffee in humans: depending on genotype?Mol Biol Rep2012397155716222314914
- ZhangYTalalayPChoCGPosnerGHA major inducer of anticar-cinogenic protective enzymes from broccoli: isolation and elucidation of structureProc Natl Acad Sci U S A199289239924031549603
- HyberstonBMGaoBBoseSKMcCordJMOxidative stress in health and disease: the therapeutic potential of Nrf2 activationMol Aspects Med20113223424622020111
- TurpaevKTKeap1-Nrf2 signaling pathway: mechanism of regulation and role in protection of cells against toxicity caused by xenobiotics and electrophilesBiochemistry (Mosc)20137811112623581983
- KorcsmárosTFarkasIJSzalayMSUniformly curated signaling pathways reveal tissue-specific cross-talks and support drug target discoveryBioinformatics2010262042205020542890
- EllmanGLTissue sulfhydryl groupsArch Biochem Biophys195982707713650640
- BradfordMMA rapid and sensitive method for the quantitation of microgram quantities of protein utilizing the principle of protein-dye bindingAnal Biochem197672248254942051
- SaraswatMMuthennaPSuryanarayanaPPetrashJMReddyGBDietary sources of aldose reductase inhibitors: prospects for alleviating diabetic complicationsAsia Pac J Clin Nutr20081755856519114390
- WangVWuWMicroRNA-based therapeutics for cancerBio Drugs20092311523
- BartelDPMicroRNAs: genomics, biogenesis, mechanism, and functionCell200411628129714744438
- AmbrosVThe functions of animal microRNASNature200443135035515372042
- ChangTCMendellJTMicroRNAs in vertebrate physiology and human diseaseAnnu Rev Genomics Hum Genet2007821523917506656
- SangokoyaCTelenMJChiJTMicroRNA miR-144 modulates oxidative stress tolerance and associates with anemia severity in sickle cell diseaseBlood20101164338434820709907
- NarasimhanMPatelDVedpathakDRathinamMHendersonGMahimainathanLIdentification of novel microRNAs in post-transcriptional control of Nrf2 expression and redox homeostasis in neural, SH-SY5Y cellsPLoS One2012712e5111
- EnomotoAItohKNagayoshiEHigh sensitivity of Nrf2 knockout mice to acetaminophen hepatotoxicity associated with decreased expression of ARE-regulated drug metabolizing enzymes and antioxidant genesToxicol Sci20015916917711134556
- ZangDDMechanistic studies of the Nrf2-Keap1 signaling pathwayDrug Metab Rev20063876978917145701
- LeeJMJohnsonJAAn important role of Nrf2-ARE pathway in the cellular defense mechanismJ Biochem Mol Biol20043713914315469687
- FaheyJWTalalayPAntioxidant functions of sulforaphane: a potent inducer of Phase II detoxication enzymesFood Chem Toxicol19993797397910541453
- NguyenTNioiPPickettCBThe Nrf2-antioxidant response element signaling pathway and its activation by oxidative stressJ Biol Chem2009284132911329519182219
- Guerrero-BeltránCECalderón-OliverMPedraza-ChaverriJChirinoYIProtective effect of sulforaphane against oxidative stress: recent advancesExp Toxicol Pathol20126450350821129940
- MustacichDPowisGThioredoxin reductaseBiochem J2000346Pt 11810657232
- ArnérESHolmgrenAPhysiological functions of thioredoxin and thioredoxin reductaseEur J Biochem20002676102610911012661
- ArnérESFocus on mammalian thioredoxin reductase: important sele-noproteins with versatile functionsBiochim Biophys Acta2009179049552619364476
- MayJMMendirattaSHillKEBurkRFReduction of dehydroascorbate by the selenoenzyme thioredoxin reductaseJ Biol Chem19972723622607226109278416
- CenasNNivinskasHAnuseviciusZSarlauskasJLedererFArnérESInteractions of quinones with thioredoxin reductase: a challenge to the antioxidant role of the mammalian selenoproteinJ Biol Chem20042792583259214604985
- DregerHWestphalKWellerANrf2-dependent upregulation of antioxidative enzymes: a novel pathway for proteasome inhibitor-mediated cardioprotectionCardiovasc Res20098335436119351736
- ElavarasanJVelusamyPGanesanTRamakrishnanSKRajasekaranDPeriandavanKHesperidin-mediated expression of Nrf2 and upregulation of antioxidant status in senescent rat heartJ Pharm Pharmacol2012641472148222943178
- NagarajRHMonnierVMProtein modification by the degradation products of ascorbate: formation of a novel pyrrole from the Maillard reaction of L-threose with proteinsBiochim Biophys Acta1995125375847492603
- ReddyPGBhuyanDKBhuyanKCLens-specific regulation of thi-oredoxin-1 gene, but not thioredoxin-2, upon in vivo photochemical oxidative stress in the Emory mouseBiochem Biophys Res Commun199926534534910558869
- MoonSFernandoMRLouMFInduction of thioltransferase and thi-oredoxinthioredoxin reductase systems in cultured porcine lenses under oxidative stressInvest Ophthalmol Vis Sci2005463783378916186363
- ZhouPLuoYLiuXFanLLuYDown-regulation and CpG island hypermethylation of CRYAA in age-related nuclear cataractFASEB J2012264897490222889833
- PalsamyPAyakiMElanchezhianRShinoharaTPromoter demethylation of Keap1 gene in human diabetic cataractous lensesBiochem Biophys Res Commun201242354254822683333
- BhargavanBChhunchhaBFatmaNKuboEKumarASinghDPEpi-genetic repression of LEDGF during UVB exposure by recruitment of SUV39H1and HDAC1 to the Sp1-responsive elements within LEDGF promoter CpG islandEpigenetics2013826828023386123
- HegdeKRVarmaSDCataracts in experimentally diabetic mouse: morphological and apoptotic changesDiabetes Obes Metab2005720020415715894
- MyzakMCHardinKWangRDashwoodRHHoESulforaphane inhibits histone deacetylase activity in BPH-1, LnCaP and PC-3 prostate epithelial cellsCarcinogenesis20062781181916280330
- OckerMSchneider-StockRHistone deacetylase inhibitors: signaling towards p21cip1/waf1Int J Biochem Cell Biol2007391367137417412634
- HsuAWongCPYuZWilliamsDEDashwoodRHHoEPromoter de-methylation of cyclin D2 by sulforaphane in prostate cancer cellsClin Epigenetics20113322303414
- LiuHSmithAJLottMCSulforaphane can protect lens cells against oxidative stress: implications for cataract preventionInvest Ophthalmol Vis Sci2013545236524823812493
- TanitoMMasutaniHKimYCNishikawaMOhiraAYodoiJSulforaphane induces thioredoxin through the antioxidant-responsive element and attenuates retinal light damage in miceInvest Ophthalmol Vis Sci20054697998715728556